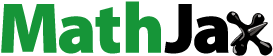
Abstract
Recent studies on biphenyl-containing compounds, a type of PD-1/PD-L1 blocker which binds to PD-L1 and induces dimerisation, have focussed on its immune function. Herein, 10 novel biphenyl derivatives were designed and synthesised. The results of the CCK-8 showed that compounds have different anti-tumour activities for tumour cells in the absence of T cells. Particularly, 12j–4 can significantly induce the apoptosis of MDA-MB-231 cells (IC50 = 2.68 ± 0.27 μM). In further studies, 12j–4 has been shown to prevent the phosphorylation of AKT by binding to cytoplasmic PD-L1, which induces apoptosis in MDA-MB-231 cells through non-immune pathways. The inhibition of AKT phosphorylation restores the activity of GSK-3β, ultimately resulting in the degradation of PD-L1. Besides, in vivo study indicated that 12j–4 repressed tumour growth in nude mice. As these biphenyls exert their anti-tumour effects mainly through non-immune pathways, they are worthy of further study as PD-L1 inhibitors.
Graphical Abstract
10 novel biphenyl derivatives were designed and synthesised.
12j–4 can cause the apoptosis of MDA-MB-231 cells with an IC50 value of 2.68 ± 0.27 μM in the absence of immune cells.
12j–4 prevents the phosphorylation of AKT by binding to cytoplasmic PD-L1 to induce apoptosis through non-immune pathways.
HIGHLIGHTS
Introduction
Triple-negative breast cancer (TNBC) refers to a subtype of breast cancer that is negative for oestrogen receptor (ER), progesterone receptor (PR), and human epidermal growth factor receptor 2 (HER2)Citation1. Compared with other breast cancers, TNBCs are more likely to express the programmed death ligand-1 (PD-L1) in the tumour microenvironment (TME)Citation2,Citation3. TNBC is highly heterogeneous, and a long-standing lack of effective therapies, with malignant and aggressive behaviour, contributes to a poor prognosis. Thus, TNBC has always been considered as one of the most unmanageable breast cancer types worth in-depth studyCitation4.
On the other hand, PD-L1 is highly expressed in many malignant tumours and, along with programmed death-1 (PD-1), is part of the immune checkpointCitation5. The CD274 gene encodes human PD-L1. The entire protein structure consists of 290 amino acids and belongs to the type I transmembrane glycoprotein, which weighs 40 kDaCitation6. Its microscopic framework consists of an extracellular region, a hydrophobic spanning region, and a cytoplasmic regionCitation7,Citation8. In the TME, the tumour cells will be marked as “safety” to achieve immune escape after PD-L1 is recognised by PD-1 presented on the T cells surfaceCitation9,Citation10.
When tumour cells express PD-L1 in a way that is not normal, they can avoid being watched and attacked by the immune system. Presently, monoclonal antibodies targeting PD-1/PD-L1 have demonstrated significant efficacy. These antibody drugs function by blocking the interaction between PD-L1 expressed on the surface of tumour cells and PD-1 present on the surface of immune cells, resulting in immune system reactivation and restoration of the immune cell-mediated killing of tumour cellsCitation11,Citation12. However, clinical outcomes have exposed several therapeutic antibodies’ disadvantages, including their lack of oral bioavailability, expensive production, immune-related adverse events, and poor uptake into tumour tissues, which hinder the clinical use of PD-1/PD-L1 antibody drugsCitation13.
In comparison with antibodies, there is mounting evidence that small molecule compounds often have improved physiological properties, such as better tissue and tumour penetration, higher oral bioavailability, and more tolerable half-livesCitation14–24. Biphenyl derivatives, such as BMS202, BMS-1166, and NSC13728 can induce PD-L1 dimerisation and enhance the stability of the PD-L1 proteinCitation25–27.
In 2015, the patent published by Bristol-Myers Squibb mentioned a class of small molecular inhibitors targeting PD-1/PD-L1 with a biphenyl skeleton, that is, BMS series compounds, which act to induce PD-L1 protein dimerisation to block the binding of PD-1 and PD-L1. Among them, BMS202 has the strongest blocking ability, and the IC50 value is as low as a nanomole, which is 18 nMCitation28. At the same time, current research shows that PD-L1 dimerisation will trigger the activation of downstream signalling pathways. Biphenyl-containing compounds have been reported to be moderately cytotoxic to tumour cells in the absence of immune cells such as T cells, for example, BMS202Citation29. PD-L1 endocytosis and nuclear transfer induce gasdermin C expression, transforming tumour cell apoptosis into pyroptosis, and promoting tumour necrosisCitation30. A variety of cancer cell-intrinsic PD-L1 causes a decrease in AKT phosphorylationCitation31,Citation32. These all lead to the occurrence of tumour cell death. All studies are based on quitting the T cell, but the signalling pathways of how PD-L1 inhibitors lead to cytotoxicity have yet to be well-known in detailCitation29,Citation33,Citation34.
Recently, our research group designed and synthesised 10 biphenyl compounds that can target PD-L1 and induce PD-L1 dimerisation to inhibit the PD-1/PD-L1 axis. During the compound screening, some compounds showed strong cytotoxicity to tumour cells, implying that these compounds appear to have non-immune pathway antitumour activity in addition to blocking PD-1/PD-L1 binding through immune pathways. However, at present, the research focuses more on the antitumour effects of biphenyl compounds through immune pathways, while the research on their non-immune pathways is less concerned. In order to demonstrate the antitumour effects of biphenyl compounds through non-immune pathways, we carried out assays by HTRF, CCK-8, wound healing methods, etc. Particularly, we found that, among these biphenyl-containing compounds, a compound (12j-4) can effectively block the PD-1/PD-L1 axis at 33.52 ± 4.81 nM concentration by HTRF assay and inhibit the obvious effect on the viability and migration of MDA-MB-231 cells, as well as on PD-L1 expression and apoptosis via AKT-mTOR, AKT-GSK-3β, and Caspase 3-PARP intracellular pathways, respectively. It is worth noting that strong anti-tumour activity, mainly via a non-immune pathway, was discovered in the case of PD-L1-highly expressed cancers.
Experimental section
Synthesis of compounds
Reagents and solvents were obtained from commercial sources and used without further purification. The compound (BMS-202) as the positive control was purchased from Topscience Co. Ltd. (Shanghai, China). All the reactions were monitored by thin-layer chromatography (TLC) using silica gel GF/UV254. Flash chromatography was performed using silica gel (300 − 400 mesh). The 1H and 13C NMR spectra were recorded on a Bruker AV-400 or a Bruker AV-600 spectrometer, with TMS as an internal standard. The purity of compounds was determined by HPLC (high-performance liquid chromatography) analysis and confirmed to be more than 95%. HPLC analyses were performed with the Shimadzu LC-20A using a C18 column (InertSustain C18, 4.6 × 250 mm, 5 μm). UV detection was conducted at 254 nm. The mobile gradient phases consisted of B (pure water) with 0.1% trifluoroacetic acid and A (100% acetonitrile) with a 1.0 ml/min flow rate. Mass spectra were measured on an Agilent 6210 TOF LC/MS (Santa Clara, CA).
Homogeneous time-resolved fluorescence (HTRF) assay
The HTRF PD1/PD-L1 binding assay (Cisbio, cat. no. 64PD1PEG) is designed to evaluate the blockage of the interaction between PD-L1 and PD-1 by compounds. The activity of the compounds was determined by the 665 nm/620 nm signal ratio, which was inversely proportional. In brief, according to the PD1/PD-L1 binding kit manufacturer’s guidelines, 2 µL of the compound, 4 µL of Tag1-PD-L1 protein, and 4 μL of Tag2-PD1 protein were added to the 384-well plates. Then, dispense 10 µL of pre-mixed Anti-Tag1 Eu Cryptate reagent and Anti-Tag2 XL665 antibody and incubate at room temperature for 1 h. Finally, the well plate is read on an HTRF-compatible reader to get the absorption value at the right wavelength from the reading.
Molecular docking
Molecular graphics manipulations and docking were performed using Discovery Studio 4.0 (DS) software. The docking simulation was performed using the CDOCKER module of DS. The PD-L1 crystal structure (PDB ID: 5J89) was taken from the Protein Data Bank. The prepared ligands module of DS is used to process the compounds. After the docking is completed, the view interaction module is used to study the interactions between the compound and the receptor.
Cell culture and inhibitors
MDA-MB-231, MCF-7, HepG2, SK-OV-3, A549, HCC827, H1299, PC-3, A375, AGS, HT-29, 786-O, K562, BE(2)-M17, SK-N-SH, SH-SY5Y, SK-N-AS, IMR32, and MRC-5 were purchased from the Cell Bank, Shanghai Institutes for Biological Sciences, Chinese Academy of Sciences. All cell lines were cultured in DMEM or RPMI-1640 (Thermo Scientific, Waltham, MA) supplemented with 10% foetal bovine serum (Thermo Scientific) and 1% Penicillin-Streptomycin Solution (Thermo Scientific). All cells were cultured at 37 °C in a humidified 5% CO2 atmosphere. For experiments, tumour cells were cultured in 6-well plates at a density of 2 × 105 cells/well. GSK-3β inhibitor (CAS: 187325–53-7) and paclitaxel (CAS: 33069–62-4) were purchased from Med Chem Express company (MCE, Monmouth Junction, NJ). BMS202 (CAS: 1675203–84-5) was purchased from TOPSCIENCE (Singapore). Cyclophosphamide (CTX, CAS: 50–18-0) was bought from Merk company (Rahway, NJ).
Cell viability assay
With paclitaxel and BMS-202 as positive controls, the cytotoxicity of compounds against tumour cells in vitro was determined by the CCK-8 method. According to the growth rates of different cell lines, cells were seeded in 96-well plates at an appropriate density and placed in a cell culture incubator at 37 °C and 5% CO2 for 8 h, then exposed to the test compounds for 60 h (all compounds used in biological experiments were dissolved in dimethyl sulfoxide (DMSO) (10 mM) as a stock solution). 10 μL of cell counting kit-8 (CCK-8) (Dojindo, CK04) was added to each well and incubated for 0.5–1 h. Each well’s OD value was measured at 450 nm using a microplate reader (SYNERGYH1, Bio-Tek, Winooski, VT). Cell viability was normalised to control.
Colony formation assay
MDA-MB-231 cells were seeded in a 6-well plate at a density of 500 cells/well in triplicate and then treated with different concentrations of 12j-4 or dimethyl sulfoxide (DMSO) for 24 h. The fresh medium was changed every three days. After two weeks, the colonies were washed twice with PBS, fixed with 4% paraformaldehyde for 15 min, and stained with 1% crystal violet. The Image J software was used to count the clones with more than 500 cells in a group formation.
Wound healing assay
MDA-MB-231 cells were seeded in a 12-well plate at a density of 5 × 104 cells/well in triplicate. After the cells were overgrown, a scratch was made in each well with the 10 μL tip, followed by cleaning up the floating cell clumps and treating them with different concentrations of the 12j-4 or BMS-202 or DMSO. Every 24 h, images were taken under the microscope for analysis.
Flow cytometry analysis
MDA-MB-231 cells were seeded in a 6-well plate at 1 × 105 cells/well. After 20 h, the medium was changed, and different compounds were added to each well. The cells were incubated for 48 h at 37 °C, 5% CO2. Cells were trypsinised, washed with PBS, resuspended and incubated in PI/Annexin-V solution (BD, 556547) for flow cytometry (Cytoflex S, Beckman Coulter, Brea, CA) for apoptosis analysis. Data were analysed by FlowJo software (BD Biosciences, Franklin Lakes, NJ). All groups have three groups for parallel testing.
Western blot
Protein was extracted from compound-treated cells. Protein was separated by electrophoresis of the sodium dodecyl sulphate-polyacrylamide gel (SDS-PAGE) and transferred to the polyvinylidene fluoride membrane (PVDF). The membranes were incubated overnight at 4 °C with primary antibodies after blocking in 5% BSA/TBST buffer for 2 h. The following primary antibodies were used at 1:1000: PD-L1 (ABCAM, cat. ab213524), AKT (CST, cat. 4691 T), p-AKT (beyotime, cat. AF1546), mTOR (CST, cat. 2972S), p-mTOR (CST, cat. 2971S), Bcl-2 (HuaBio, cat. HN0921), Caspase 3 (CST, cat. 9662S), c-Caspase 3 (beyotime, cat. AC033), PARP (CST, cat. 9542 T), c-PARP (CST, cat. 9541 T), GAPDH (beyotime, cat. AF1186), and β-actin (CST, cat. 4970 T).
Immunofluorescence and immunofluorescence colocalisation
Cells were cultured on a confocal dish (cat. BS-20-GJM, Biosharp, Tallin, Estonia) and washed twice with pre-warmed PBS. For cytoplasmic membrane PD-L1 staining, cells were fixed with 5% PFA (Paraformaldehyde) for 15 min, followed by 0.5% triton (PFA + Triton X-100) for 10 min. For cell membrane PD-L1 staining, cells were fixed with 5% PFA for 15 min only. Cells were blocked with 1% BSA and then incubated with the PD-L1 antibody for 1 h at room temperature. Primary antibody: Anti-human PD-L1 (ABCAM, cat. ab213524). Secondary Antibody: Anti-Rabbit Alexa Fluor 568 (ABCAM, cat. Ab175470). For immunofluorescence, images were acquired using a fluorescence microscope (Olympos, Tokyo, Japan; CKX3) and analysed by Image J. For immunofluorescence colocalisation, images were acquired using a confocal microscope (Zeiss; LSM 880) and analysed by ZEN software.
In vivo animal model assays
Female BALB/c nude mice were purchased from Shanghai SLAC Laboratory Animal Co., Ltd. This experiment was approved by the animal ethics committee of the Zhejiang University of Technology (20210719055). MDA-MB-231 cells within 10 generations were suspended in a PBS and Matrigel (Corning, cat. 356234) solution (PBS: Matrigel = 1:1) and adjusted to a density of 3 × 107 cells/mL. These cells were then subcutaneously injected into the axilla of female BALB/c nude mice at a volume of 100 μL per injection. After tumour growth reached approximately 200 mm3, intraperitoneal treatment with compounds dissolved in normal saline (including 30% PEG400) was initiated every 2 days. Tumour volume was monitored using Vernier callipers, and the study was concluded once the control group tumours reached 1500 mm3. All mice were sacrificed by dislocating their cervical spine, followed by tumour removal and subsequent weighing. The tumour volume and tumour inhibition rate were calculated according to the following formula:
Statistical analysis
Data in bar or line graphs are presented as the means ± SD or means ± SEM of at least three independent experiments. Comparisons were carried out with the Pearson R test or the unpaired Student’s t-test (*p < 0.05, **p < 0.01, ***p < 0.001).
Results and discussion
Synthesis of biphenyl-containing small molecule compounds
The synthesis of the critical intermediates 5a–d is outlined in Scheme S1 (to see supporting information for details of the synthetic procedure). The synthesis of compounds 13h-1–3, 13b-4–6, 7b-2, 7b-4, and 12j-3–4 is depicted in Scheme 1. The starting material, 2,4-Dihydroxy-benzaldehyde (6 u) or 5-Chloro-2,4-dihydroxy-benzaldehyde (6v), was reacted with intermediates 5a–d to afford intermediates 7a–f, which were reacted with different chloromethyl-aryl derivatives in the presence of Cs2CO3 to yield intermediates 8a–i. Intermediates 8a–i were directly reacted with appropriate amines (R3–H) in the presence of NaBH3CN and acetic acid to give rise to the compounds 13h-1–3, 13b-4–6, 7b-2, 7b-4, and 12j-3–4. In addition, the 1H NMR spectra of the intermediates are exhibited in Figure S3. 1H NMR and 13C NMR spectra of the final compounds were also shown in the supporting materials (Figure S4).
Synthesis of intermediates 7a − f
To the mixture of compounds 5a–d (1.0 eq), K2CO3 (1.3 eq), 2,4-Dihydroxy-benzaldehyde (6u), or 5-Chloro-2,4-dihydroxy-benzaldehyde (6v) (1.2 eq), and DMF (5.0 vol) were added at room temperature. The mixture was stirred at 60 °C for 2 h and monitored by TLC. The reaction mixture was cooled to room temperature, and EA and water were added. The aqueous phase was extracted once with ethyl acetate. The organic phase was collected, dried with anhydrous sodium sulphate, washed with brine, and evaporated under reduced pressure. The residue was purified by silica gel column chromatography (ethyl acetate/petroleum ether, 1:10) to afford intermediates 7a–f.
4-(2'-Fluoro-2-methoxy-biphenyl-3-ylmethoxy)-2-hydroxy-benzaldehyde (7a)
Off-white solid (817 mg, yield 43%). 1H NMR (400 MHz, Chloroform-d): δ 11.50 (s, 1H), 9.75 (d, J = 0.6 Hz, 1H), 7.54 − 7.44 (m, 3H), 7.38 (dddd, J = 9.1, 7.5, 4.1, 2.1 Hz, 2H), 7.30 − 7.17 (m, 3H), 6.67 (dd, J = 8.7, 2.3 Hz, 1H), 6.60 (d, J = 2.3 Hz, 1H), 5.25 (s, 2H), and 3.46 (s, 3H).
4-(2'-Fluoro-2-methyl-biphenyl-3-ylmethoxy)-2-hydroxy-benzaldehyde (7d)
Off-white solid (242 mg, yield 41%). 1H NMR (400 MHz, Chloroform-d): δ 11.53 (s, 1H), 9.76 (s, 1H), 7.48 (t, J = 8.4 Hz, 2H), 7.43 − 7.36 (m, 1H), 7.35 − 7.29 (m, 2H), 7.28 − 7.21 (m, 2H), 7.17 (s, 1H), 6.67 (dd, J = 8.6, 2.3 Hz, 1H), 6.60 (d, J = 2.3 Hz, 1H), 5.18 (s, 2H), 2.21 (d, J = 1.5 Hz, 3H).
Synthesis of intermediates 8a − i
To the mixture of compounds 7a–f (1.0 eq), Cs2CO3 (1.3 eq), NaI (0.5 eq), different chloromethyl-aryl derivatives (1.1 eq), and DMF (5.0 vol) were added at room temperature. The mixture was stirred at 60 °C for 2 h and monitored by TLC. The mixture was quenched with saturated aqueous NaCl, and the precipitate was filtered and dried to obtain intermediates (8a − i) as white solids. Intermediates 8a-i were used directly for the next step without purification.
Synthesis of compounds 13h-1–3, 13b-4–6, 7b-2, 7b-4, and 12j-3–4
N-[2–(5-Cyano-pyridin-3-ylmethoxy)-4-(2'-fluoro-2-methoxy-biphenyl-3-ylmethoxy)-benzyl]-D-serine (13h-1)
To a solution of intermediate 8a (46.8 mg, 0.1 mmol, 1.0 eq) and D-serine (21.0 mg, 0.2 mmol, 2.0 eq) in MeOH (2 ml) were added two drops of acetic acid. After the mixture was stirred at room temperature for 30 min, sodium cyanoborohydride (12.6 mg, 0.2 mmol, 2.0 eq) was added. The mixture was stirred overnight at room temperature until TLC indicated the completion of the reaction. The solvent was evaporated to give a residue, which was purified by Prep TLC to afford compound 13h-1 as an off-white solid (12 mg, 21%). mp 154 − 157 °C. Purity: 95.20% by HPLC. 1H NMR (500 MHz, Chloroform-d): δ 8.89 − 8.86 (m, 1H), 8.68 (s, 1H), 8.16 (s, 1H), 7.45 − 7.33 (m, 4H), 7.28 (s, 1H), 7.24 − 7.11 (m, 3H), 6.61 − 6.54 (m, 2H), 5.09 (d, J = 26.1 Hz, 4H), 4.23 (s, 2H), 3.89 (s, 2H), 3.45 (s, 1H), 3.38 (s, 3H). 13C NMR (101 MHz, Chloroform-d) δ 171.12, 161.09, 160.94, 158.49, 157.50, 156.04, 152.08, 151.72, 138.72, 132.68, 131.98, 131.62 (d, J = 3.2 Hz), 129.73, 129.63, 129.53 (d, J = 8.0 Hz), 129.34, 125.66, 124.16, 124.07 (d, J = 3.5 Hz), 116.52, 115.82, 115.60, 109.93, 107.14, 100.12, 66.89, 65.36, 62.21, 61.51, 60.37, 53.39.
Compounds 13h-2–3, 13b-4–6, 7b-2, 7b-4, and 12j-3––4 were prepared similarly as described for compound 13h-1 by reacting 8b–i with appropriate amines.
N-{2-[5-Chloro-2-(3-cyano-benzyloxy)-4-(2'-fluoro-2-methoxy-biphenyl-3-ylmethoxy)-benzylamino]-ethyl}-acetamide (13h-2)
It was obtained as an off-white solid (23 mg, yield 27%). mp 87 − 91 °C. Purity: 96.81% by HPLC. 1H NMR (600 MHz, Chloroform-d): δ 7.74 (td, J = 1.7, 0.8 Hz, 1H), 7.66 (dp, J = 5.8, 1.6 Hz, 2H), 7.58 (dd, J = 7.7, 1.8 Hz, 1H), 7.53 (t, J = 7.8 Hz, 1H), 7.44 − 7.38 (m, 2H), 7.36 − 7.29 (m, 2H), 7.24 (td, J = 7.5, 1. 2 Hz, 2H), 7.18 (ddd, J = 10.0, 8.7, 1.2 Hz, 1H), 6.68 (s, 1H), 6.59 (s, 1H), 5.28 (s, 2H), 5.10 (s, 2H), 3.79 (s, 2H), 3.48 (s, 3H), 3.35 (q, J = 5.6 Hz, 2H), 2.78 (dd, J = 6.7, 4.9 Hz, 2H), 1.96 (s, 3H). 13C NMR (101 MHz, Chloroform-d) δ 173.37, 159.69 (d, J = 246.9 Hz), 156.14, 155.79, 155.76, 137.68, 132.44, 132.05, 131.99, 131.53 (d, J = 3.3 Hz), 131.12, 129.77, 129.68, 129.49, 129.44, 129.19, 125.71, 125.55, 124.54, 124.22 (d, J = 3.6 Hz), 118.66, 115.84, 115.62, 114.77, 113.05, 112.65, 99.27, 69.41, 65.73, 61.86, 48.30, 46.57, 37.32, 22.81.
3-{4-Chloro-5–(2'-fluoro-2-methoxy-biphenyl-3-ylmethoxy)-2-[(2-hydroxy-ethylamino)-methyl]-phenoxymethyl}-benzonitrile (13h-3)
It was obtained as an off-white solid (19 mg, yield 31%). mp 77 − 80 °C. Purity: 96.81% by HPLC. 1H NMR (600 MHz, Chloroform-d): δ 7.76 (d, J = 1.7 Hz, 1H), 7.69 (d, J = 7.7 Hz, 1H), 7.65 (dt, J = 7.8, 1.4 Hz, 1H), 7.57 (dd, J = 7.6, 1.7 Hz, 1H), 7.53 (t, J = 7.8 Hz, 1H), 7.45 − 7.38 (m, 2H), 7.34 − 7.29 (m, 2H), 7.27 − 7.21 (m, 2H), 7.21 − 7.15 (m, 1H), 6.70 (s, 1H), 5.28 (s, 2H), 5.13 (s, 2H), 3.86 (s, 2H), 3.69 (t, J = 5.1 Hz, 2H), 3.48 (s, 3H), 2.84 (t, J = 5.1 Hz, 2H). 13C NMR (101 MHz, Chloroform-d) δ 159.69 (d, J = 246.9 Hz), 156.15, 155.81, 155.67, 137.54, 132.38, 132.19, 132.03, 131.54 (d, J = 3.3 Hz), 131.17, 129.79, 129.70 (d, J = 8.1 Hz), 129.50, 129.40, 129.24, 125.72, 125.56, 124.49, 124.22 (d, J = 3.5 Hz), 118.72, 115.84, 115.61, 114.73, 113.35, 112.55, 99.34, 69.47, 65.79, 61.84, 57.48, 49.21, 46.70.
N-{2–(3-Cyano-benzyloxy)-4-[3–(2,3-dihydro-benzo[1,4]dioxin-6-yl)-2-methoxy-benzyloxy]-benzyl}-L-serine (13b-4)
It was obtained as an off-white solid (22 mg, yield 34%). mp 134 − 136 °C Purity: 97.35% by HPLC. 1H NMR (400 MHz, Chloroform-d) δ 7.76 − 7.66 (m, 2H), 7.53 (d, J = 7.5 Hz, 1H), 7.45 (t, J = 7.6 Hz, 1H), 7.38 − 7.29 (m, 3H), 7.18 − 7.10 (m, 2H), 7.06 (dd, J = 8.3, 2.1 Hz, 1H), 6.92 (d, J = 8.3 Hz, 1H), 6.60 (d, J = 8.1 Hz, 1H), 6.55 (d, J = 2.1 Hz, 1H), 5.13 (s, 2H), 5.05 (s, 2H), 4.32 (s, 6H), 3.94 (s, 2H), 3.69 (s, 1H), 3.51 (s, 1H), 3.40 (s, 3H). 13C NMR (101 MHz, Chloroform-d) δ 161.25, 157.67, 155.55, 143.34, 143.04, 137.81, 134.21, 131.86, 131.74, 131.38, 131.28, 130.81, 129.82, 129.64, 128.55, 124.30, 122.09, 118.76, 117.69, 117.19, 112.49, 111.66, 106.75, 100.38, 69.17, 65.58, 64.45, 64.37, 60.98, 29.72.
N-(2-{5-Chloro-2-(3-cyano-benzyloxy)-4-[3–(2,3-dihydro-benzo [1,4] dioxin-6-yl)-2-methoxy-benzyloxy]-benzylamino}-ethyl)-acetamide (13b-5)
It was obtained as an off-white solid (19 mg, yield 31%). mp 103 − 106 °C. Purity: 98.31% by HPLC. 1H NMR (400 MHz, Chloroform-d) δ 7.76 (d, J = 1.7 Hz, 1H), 7.71 − 7.64 (m, 2H), 7.59 − 7.46 (m, 2H), 7.35 − 7.29 (m, 2H), 7.20 (t, J = 7.6 Hz, 1H), 7.15 (d, J = 2.1 Hz, 1H), 7.06 (dd, J = 8.4, 2.1 Hz, 1H), 6.94 (d, J = 8.4 Hz, 1H), 6.74 (s, 1H), 6.14 (s, 1H), 5.26 (s, 2H), 5.12 (s, 2H), 4.35 (s, 4H), 3.77 (s, 2H), 3.50 (s, 3H), 3.34 (q, J = 5.6 Hz, 2H), 2.76 (t, J = 5.8 Hz, 2H), 1.97 (s, 3H). 13C NMR (101 MHz, Chloroform-d) δ 170.41, 155.41, 155.20, 153.87, 143.40, 143.14, 138.15, 134.18, 131.82, 131.36, 131.31, 131.27, 130.98, 130.63, 129.82, 129.63, 128.41, 124.64, 122.03, 121.50, 118.63, 117.69, 117.28, 114.79, 112.84, 99.74, 69.19, 66.04, 64.49, 64.46, 61.29, 47.86, 47.64, 39.05, 23.26.
N-{2-[4-[3–(2,3-Dihydro-benzo [1,4] dioxin-6-yl)-2-methoxy-benzyloxy]-2-(pyridin-3-ylmethoxy)-benzylamino]-ethyl}-acetamide (13b-6)
It was obtained as an off-white solid (14 mg, yield 26%). mp 98 − 101 °C. Purity: 95.81% by HPLC. 1H NMR (600 MHz, Chloroform-d) δ 8.74 − 8.71 (m, 1H), 8.60 (dd, J = 4.9, 1.6 Hz, 1H), 7.79 (dt, J = 7.9, 2.0 Hz, 1H), 7.43 (dd, J = 7.6, 1.7 Hz, 1H), 7.38 − 7.31 (m, 2H), 7.25 − 7.15 (m, 2H), 7.14 (d, J = 2.1 Hz, 1H), 7.10 (dd, J = 8.3, 2.1 Hz, 1H), 6.94 (d, J = 8.4 Hz, 1H), 6.68 (d, J = 2.3 Hz, 1H), 6.65 (dd, J = 8.3, 2.3 Hz, 1H), 6.47 (s, 1H), 5.15 (d, J = 14.7 Hz, 4H), 4.33 (s, 4H), 3.88 (s, 2H), 3.47 (s, 3H), 3.37 (q, J = 5.5 Hz, 2H), 2.84 (t, J = 5.5 Hz, 2H), 1.94 (s, 3H). 13C NMR (101 MHz, Chloroform-d) δ 172.84, 161.45, 157.66, 155.61, 149.34, 148.83, 143.37, 143.06, 135.90, 134.32, 132.89, 131.98, 131.39 (d, J = 2.5 Hz), 129.83, 128.58, 124.37, 123.86, 122.12, 117.71, 117.20, 111.86, 106.48, 100.39, 67.83, 65.69, 64.46, 64.39, 61.05, 47.80, 46.83, 36.55, 22.81.
3-[2-[(2-Hydroxy-ethylamino)-methyl]-5-(2,2',5'-trifluoro-biphenyl-3-ylmethoxy)-phenoxymethyl]-benzonitrile (7b-2)
It was obtained as an off-white solid (12 mg, yield 26%). mp 74 − 76 °C. Purity: 97.21% by HPLC. 1H NMR (600 MHz, Chloroform-d) δ 7.76 (d, J = 1.7 Hz, 1H), 7.71 (d, J = 7.6 Hz, 1H), 7.66 − 7.62 (m, 1H), 7.59 − 7.50 (m, 2H), 7.40 − 7.36 (m, 1H), 7.30 (d, J = 8.3 Hz, 1H), 7.26 (d, J = 7.7 Hz, 1H), 7.13 (dddd, J = 23.1, 8.9, 7.0, 4.0 Hz, 3H), 6.66 − 6.58 (m, 2H), 5.17 (d, J = 3.8 Hz, 4H), 4.05 (s, 2H), 3.74 (t, J = 4.8 Hz, 2H), 2.94 (t, J = 5.0 Hz, 2H). 13C NMR (101 MHz, Chloroform-d) δ 159.45, 159.24, 157.92, 157.27, 155.45, 138.22, 133.34, 131.75, 131.48, 131.36, 130.62, 130.06 (d, J = 4.1 Hz), 129.59, 128.73 (d, J = 3.5 Hz), 125.54 (d, J = 15.3 Hz), 125.33 (d, J = 4.5 Hz), 119.34, 117.92 (d, J = 25.1 Hz), 116.89 (dd, J = 25.1, 8.7 Hz), 116.33 (dd, J = 24.0, 8.6 Hz), 112.79, 105.83, 100.54, 68.86, 63.86 (d, J = 3.9 Hz), 59.91, 50.09, 47.82.
5-[2-[(2-Hydroxy-ethylamino)-methyl]-5-(2,2',5'-trifluoro-biphenyl-3-ylmethoxy)-phenoxymethyl]-nicotinonitrile (7b-4)
It was obtained as an off-white solid (17 mg, yield 32%). mp 69 − 71 °C. Purity: 96.53% by HPLC. 1H NMR (600 MHz, Chloroform-d) δ 8.93 − 8.90 (m, 1H), 8.86 − 8.83 (m, 1H), 8.17 (s, 1H), 7.56 (t, J = 7.2 Hz, 1H), 7.41 − 7.36 (m, 1H), 7.29 (q, J = 5.7, 3.7 Hz, 2H), 7.20 − 7.08 (m, 3H), 6.68 − 6.61 (m, 2H), 5.20 (d, J = 13.8 Hz, 4H), 4.04 (s, 2H), 3.76 − 3.73 (m, 2H), 2.95 (t, J = 5.1 Hz, 2H). 13C NMR (101 MHz, Chloroform-d) δ 160.41, 160.24, 157.96, 157.14, 155.49, 151.92, 151.86, 138.67, 133.48, 132.79, 132.39, 130.21 (d, J = 4.1 Hz), 128.92 (d, J = 3.4 Hz), 125.40 (d, J = 4.5 Hz), 125.30, 124.41, 117.89 (d, J = 23.0 Hz), 117.20, 116.58, 115.31 (d, J = 12.9 Hz), 110.07, 109.15 (d, J = 20.8 Hz), 106.43, 100.50, 66.71, 64.01 (d, J = 3.7 Hz), 58.19, 49.43, 47.28.
N-{2-[2-(3-Cyano-benzyloxy)-4-(2'-fluoro-2-methyl-biphenyl-3-ylmethoxy)-benzylamino]-ethyl}-acetamide (12j-3)
It was obtained as an off-white solid (13 mg, yield 22%). mp 69 − 72 °C. Purity: 97.33% by HPLC. 1H NMR (600 MHz, Chloroform-d): δ 7.76 (d, J = 1.9 Hz, 1H), 7.66 (ddt, J = 13.9, 7.8, 1.4 Hz, 2H), 7.54 (t, J = 7.8 Hz, 1H), 7.45 (dd, J = 7.5, 1.6 Hz, 1H), 7.39 (dddd, J = 8.3, 7.1, 5.1, 1.9 Hz, 1H), 7.33 − 7.21 (m, 5H), 7.17 (ddd, J = 9.6, 8.2, 1.2 Hz, 1H), 6.64 (dd, J = 8.3, 2.3 Hz, 1H), 6.59 (d, J = 2.3 Hz, 1H), 6.36 (s, 1H), 5.13 (s, 2H), 5.08 (s, 2H), 3.86 (s, 2H), 3.37 (q, J = 5.6 Hz, 2H), 2.81 (t, J = 5.7 Hz, 2H), 2.20 (d, J = 1.5 Hz, 3H), 1.96 (s, 3H). 13C NMR (101 MHz, Chloroform-d) δ 170.36, 159.64 (d, J = 245.7 Hz), 159.46, 157.09, 138.50, 136.68, 135.45, 134.94, 131.68, 131.63 (d, J = 3.6 Hz), 131.25, 130.82, 130.45, 129.59, 129.31, 129.23, 128.61, 125.72, 124.11 (d, J = 3.6 Hz), 121.06, 118.66, 115.53 (d, J = 22.4 Hz), 112.83, 105.60, 100.78, 69.20, 68.68, 48.26, 47.75, 39.09, 23.26, 15.85 (d, J = 2.4 Hz).
N1-[2-Benzyloxy-4-(2'-fluoro-2-methyl-biphenyl-3-ylmethoxy)-benzyl]-ethane-1,2-diamine (12j-4)
It was obtained as an off-white solid (11 mg, yield 18%). mp 83 − 85 °C Purity: 96.73% by HPLC. 1H NMR (400 MHz, Chloroform-d) δ 7.46 − 7.34 (m, 6H), 7.32 (dd, J = 5.8, 2.7 Hz, 1H), 7.30 − 7.20 (m, 5H), 7.16 (t, J = 9.0 Hz, 1H), 6.66 − 6.58 (m, 2H), 5.06 (d, J = 18.3 Hz, 4H), 4.04 (s, 2H), 3.10 (d, J = 22.0 Hz, 4H), 2.17 (s, 3H), 1.86 (s, 2H). 13C NMR (101 MHz, Chloroform-d) δ 160.99, 159.65 (d, J = 245.8 Hz), 158.06, 136.65, 136.23, 135.47, 134.73, 132.33, 131.65, 130.47, 129.27, 129.23, 129.19, 129.06, 128.76, 128.60, 128.30, 127.56, 125.70, 124.07, 115.63, 115.41, 105.94, 100.71, 70.37, 69.12, 46.40, 44.86, 36.90, 15.79. HRMS (ESI): the exact mass calculated for C30H31FN2O2 [M + H]+ 471.2442 was found at 471.2448.
In vitro evaluation of target compounds for inhibiting PD-1/PD-L1 interaction
The activities of compounds 13h-1–3, 13b-4––6, 7b-2, 7b-4, and 12j-3–4 as inhibitors of the PD-1/PD-L1 interaction were evaluated by the well-established homogeneous time-resolved fluorescence (HTRF) assay. The biochemical data are presented as IC50 values and listed in . Results show that compounds 13b-4–6 exhibited weak inhibitory activities against the PD-1/PD-L1 interaction, with IC50 values of more than 1 μM. On the contrary, compounds 13h-1–3, 7b-2, 7b-4, and 12j-3–4 demonstrated excellent inhibitory potency with IC50 values of less than 1 μM. In particular, compound 12j-4 possessed the strongest inhibitory activities with an IC50 value of 33.52 ± 4.81 nM, which was worthy of further examination in vitro and in vivo.
Table 1. The inhibitory activities of compounds 13h-1–3, 13b-4–6, 7b-2, 7b-4, and 12j-3–4 on the PD-1/PD-L1 interaction.
Molecular docking study of compound 12j-4 with the PD-L1 dimer
Molecular docking studies were carried out using Discovery Studio software, and the binding mode of 12j-4 to the PD-L1 dimer was explored. As shown in , the fluorine atom in the 12j-4 structure generates a halogen interaction with B-Ile116. The biphenyl moiety and central phenyl ring generate π-π stacking interactions with B-Tyr56 and A-Tyr56, respectively. Concurrently, the benzene ring of the side chain also produces a π-π stacking interaction with B-Ala18. In addition, the ethylenediamine structure in the side chain produces an electrostatic interaction and a hydrogen bond with B-Asp122 and A-Gln66, respectively. In summary, these interactions enable compound 12j-4 to bind well with PD-L1 dimers.
Figure 1. Detailed interactions of compound 12j-4 (the green stick) with the PD-L1 dimer. The crystal structure of the dimeric PD-L1 protein was taken from PDB ID: 5J89. Chain A is a solid cyan ribbon, while chain B is a solid magenta ribbon. The green dashed lines represent H-Bond interactions. The magenta dashed line represents hydrophobic interactions. The orange dashed lines represent electrostatic interactions. The cyan-dashed line represented halogen interactions.

In vitro anti-tumour activity evaluation of novel synthesised compounds by the CCK-8 assay
On the basis of the above completed assays, we have found that, among 10 novel synthesised biphenyl derivatives (13h-1–3, 13b-4––6, 7b-2, 7b-4, and 12j-3–4), 13h-1, 13h-3, and 12j-4 showed good performance in blocking PD-1/PD-L1 interaction by HTRF method (), of which 12j-4 can interact very well with PD-L1 dimer by docking study () and HTRF method. In order to examine the ability of these new compounds against tumour cells in the non-immune system (in the absence of immune cells, such as T cells), their anti-tumour proliferation activity was conducted by the CCK-8 assay on 18 kinds of cancer cells (MDA-MB-231, MCF-7, HepG2, SK-OV-3, A549, HCC827, H1299, PC-3, A375, AGS, HT-29, 786-O, K562, BE(2)-M17, SK-N-SH, SH-SY5Y, SK-N-AS, IMR32) and 1 normal cells (MRC-5). Initially, tested cells were treated with 4 µM of each compound for 60 h, and progressive cell growth inhibition was observed. As shown in and Figure S1, all compounds presented different anti-proliferative activities for all tested cancer cells, in particular, compound 12j-4 did stronger effect on half of tested cancer cells. In order to understand the anti-proliferative mechanism of compounds in the absence of immune cells, one PD-L1 high-expressing strain and one PD-L1 low-expressing strain were selected for further CCK-8 assay from breast cancer (MDA-MB-231 (high), MCF-7 (low),) and lung cancer cell lines (HCC-827 (high), A549 (low)), respectively, and the IC50 values of 13h-1, 13h-3, and 12j-4 for these four kinds of cancer cells were obtained, with BMS-202 as a positive control. As observed in , 12j-4 exhibited the best anti-proliferation activity against cancer cell lines: MDA-MB-231, MCF-7, HCC827, and A549 in the second trial, with IC50 values of 2.68 ± 0.27 μM, 4.71 ± 0.44 μM, 3.24 ± 0.09 μM, and 7.79 ± 0.69 μM, respectively (). While three compounds exhibited moderate cytotoxicity against normal MRC-5 cells with IC50 values around 10 μM, which were more than twice as large as those against MDA-MB-231 cells in and , in particular, that of 12j-4 is 3.83-fold. Taken together, these results suggest that 12j-4 showed potent cytotoxicity against four tested cancer cells, especially MDA-MB-231 cells with the strongest inhibitory activity and best selectivity for MDA-MB-231 cells. This suggests that 12j-4 should be explored as a promising MDA-MB-231 cell inhibitor. What’s more, the observation of the greater inhibitory impact of 12j-4 on breast cancer and lung cancer cell lines exhibiting high PD-L1 expression compared to their low PD-L1 expression counterparts, along with the weaker inhibitory effects of 13h-1 and 13h-3 on cell lines with high PD-L1 expression, suggests that the anti-tumour activity of these small-molecule compounds may be related to the expression of the PD-L1 protein.
Table 2. IC50 values (μM) for the MDA-MB-231, MCF-7, A549, HCC827, and MRC-5 cell lines treated with 12j-4, 13h-1, and 13h-3.
Compound 12j-4 inhibits the proliferation and migration of MDA-MB-231 cells
Then, we investigated the effect of compound 12j-4 on proliferation and migration in MDA-MB-231 cells via a colony formation assay and a wound healing assay. The clone formation experiment indicated that, compared to BMS-202, the 12j-4 treated group’s formation rate was much lower (, Figure S2(A)). At a concentration of 1 µM, which was lower than the IC50 and did not possess cytotoxicity for MDA-MB-231 cells, the MDA-MB-231 cells’ proliferation rate reached to 40%, while BMS-202 showed scarce inhibition activity under the same condition. Few colonies can be observed in the paclitaxel group.
Figure 2. Viability of MDA-MB-231 cells treated with 4 μM of compounds (A) 13h-1–3, 13b-4–6, 7b-2, 7b-4, and 12j-3–4, and (B) MDA-MB-231, (C) A549, (D) HCC-827, (E) MCF-7, and (F) MRC-5 treated with compounds 12j-4, 13h-1, and 13h-3 with 0, 1, 2, 4, 8, 16, and 32 μM for 60 h, respectively. Cell viability was determined using the Cell Counting Kit-8 assay. Positive control: paclitaxel and BMS202. Negative control: 1% DMSO. Data are expressed as mean ± SEM (n = 3). *p < 0.05, **p < 0.01, ***p < 0.001.

MDA-MB-231 cells possess the characteristics of strong metastasis and invasion ability. The wound healing assay showed that 12j-4 could significantly inhibit the migration of MDA-MB-231 cells. As shown in and S2(B), the migration inhibition rate of 12j-4 at 1 μM on MDA-MB-231 cells reached 50%, while BMS-202 can hardly inhibit the cancer cells’ migration. These results are in agreement with the experimental results described earlier. Interestingly, the migration inhibitory activity of 12j-4 remained stable at around 50% over time.
Compound 12j-4 induced MDA-MB-231 apoptosis
Next, we examined whether 12j-4 can induce apoptosis in MDA-MB-231 cells by flow cytometry assay. Results showed that the ability of 12j-4 to induce apoptosis is weaker than that of paclitaxel but stronger than that of BMS202 (, Figure S2(C)). The apoptotic ratio exceeded 40.42% when the compound concentration reached 4 μM, which was about fourfold that of the negative control group. And, paclitaxel induces approximately 60% tumour cell apoptosis under the condition of a 1 μM intervention for 48 h. As for another positive control, BMS-202, its ability to trigger apoptosis of tumour cells at the concentration of 4 µM is significantly weak, only slightly stronger than that in the 1 μM-12j-4 group.
Figure 3. Inhibition of the proliferation and migration of MDA-MB-231 cells with compound 12j-4. (A) The clone formation rates of MDA-MB-231 cells were treated with 12j-4 for 24 h at a concentration of 1 μM. (B) Migration ability of MDA-MB-231 cells treated with 12j-4 (1 μM) for 24 h and 48 h. Positive control: paclitaxel and BMS202. Negative control: 1% DMSO. Data are expressed as mean ± SEM (n = 3). *p < 0.05, **p < 0.01, ***p < 0.001.

Figure 4. 12j-4 induced apoptosis in MDA-MB-231 cells. (A) The apoptosis of MDA-MB-231 cells was evaluated by flow cytometry after they were treated with 12j-4 for 48 h at a concentration of 4 μM. (B) The expression of Bcl-2, Caspase 3, cleaved-Caspase 3, PARP, and cleaved-PARP proteins was detected by Western blot in MDA-MB-231 cells treated with compound 12j-4 for 48 h at a concentration of 1, 2, and 4 μM, respectively. Data are expressed as mean ± SEM (n = 3). *p < 0.05, **p < 0.01, ***p < 0.001.

All of these results suggested that the compound 12j-4 has a strong interaction and inhibitory effect on tumour cells in the absence of immune cells such as T cells. And then, the mechanism leading to the appearance of 12j-4 against tumour cells was further explored in the next assay.
By Western blot analysis, we confirmed the expression of apoptosis-related proteins. The results indicated that under the intervention of 12j-4, the apoptosis pathway was awakened in MDA-MB-231 cells. The expression of Bcl-2, Caspase-3, and PARP proteins was decreased in the presence of 12j-4. Relatively, c-Caspase-3 and c-PARP proteins’ levels significantly increased (). In brief, 12j-4 demonstrates potent anti-tumour activity through various mechanisms, such as suppressing migration, inhibiting invasion, and inducing apoptosis in the absence of immune cell involvement. Recent investigations have highlighted the intrinsic modulatory function of PD-L1 in promoting tumour cell viability, apoptosis resistance, and metastasisCitation35–37. Consequently, we were motivated to investigate the potential of the small molecule 12j-4 to modulate the expression level of PD-L1 in MDA-MB-231 cells with the aim of eliciting a tumour-inhibitory effect.
Compound 12j-4 downregulates PD-L1 expression
PD-L1 is the ligand of the immune checkpoint PD-1, which can help tumour cells escape immune surveillance through immune pathways. In non-immune pathways, the PD-L1 protein also plays a critical role in tumour cell growth and metabolismCitation38,Citation39. Recent research has revealed that biphenyl small molecule compounds, exemplified by the BMS series, can bind to PD-L1 and trigger its dimerisation. Certain compounds have demonstrated the capability to promote PD-L1 degradationCitation40. This evidence suggests that the biphenyl-based compound 12j-4 may possess targeted regulation abilities over PD-L1. Through a Western blot assay, PD-L1 has been demonstrated to be down-regulated in MDA-MB-231 cells after treatment with 12j-4 for 48 h (). Along with it, as shown in , PD-L1 mRNA decreased. However, even if the mRNA is decreased, its level cannot correspond to the PD-L1 protein dropped. Further study found that PD-L1’s decrement can be reversed by a GSK-3β inhibitor (). Due to this finding, the location of PD-L1 degradation was explored by determining whether to rupture the cell membranes via an immunofluorescence assay. Results in demonstrate that treatment of tested cells with 12j-4 compound significantly reduces cytoplasmic PD-L1 fluorescence after disrupting the cell membrane with 0.5% Triton X-100. However, this effect can be reversed by GSK-3β inhibitors. We observed the accumulation of PD-L1 protein in the cytoplasm in the GSK-3β inhibitor group, which could be due to the degradation of multivesicular bodies and lysosomes Citation41. Furthermore, in the group without Triton X-100 treatment (), we observed that the change in PD-L1 protein levels on the cell membrane was significantly lower than that in the cytoplasm. The reversal effect of the GSK-3β inhibitor on the down-regulation of PD-L1 expression by 12j-4 was insignificant.
Figure 5. The expression level of PD-L1 is regulated by compound 12j-4. MDA-MB-231 cells were treated with 12j-4 for 48 h at a concentration of 1, 2, and 4 μM, respectively. BMS202 was used as a positive drug. (A) The expression of the PD-L1 protein and (B) PD-L1 mRNA was detected by Western blot and qPCR, respectively. The expression of PD-L1 protein was detected by (C) Western blot after MDA-MB-231 cells were treated with or without 12j-4 (4 μM) combined with GSK-3β inhibitor (40 nM) for 48 h. Data are expressed as mean ± SEM (n = 3). *p < 0.05, **p < 0.01, ***p < 0.001.

Figure 6. Representative immunofluorescence images of PD-L1 (red) and nucleic (blue) foci in MDA-MB-231 cells treated with or without 12j-4 (4 μM) combined with GSK-3β inhibitor (40 nM) for 48 h. (A) PD-L1 protein expressed in organelle membranes with cell membrane ruptured; (B) PD-L1 protein expressed in organelle membranes with cell membrane non-ruptured.

These data demonstrated the ability of 12j-4 to diminish the expression level of PD-L1 in MDA-MB-231 cells significantly. However, this degradation predominantly affects the cytoplasmic fraction of PD-L1 rather than the membrane-associated form. The results in have implicated GSK-3β in the degradation of PD-L1, yet the mechanism underlying 12j-4-mediated upregulation of GSK-3β activity remains unclear and requires further elucidation. Notably, the activity of GSK-3β is reported to be modulated by the PI3K/AKT signalling pathwayCitation42, which regulates vital cellular processes such as proliferation and apoptosis. In addition, the activity of this pathway is upregulated by tumour-associated PD-L1, suggesting a plausible mechanistic basis for the observed effects of 12j-4.
Fluorescence co-localization of 12j-4/PD-L1
PD-L1 protein is distributed in the cell membrane, nucleus, and organelles such as the endoplasmic reticulum and Golgi apparatus. Like the positive control drug BMS-202, compound 12j-4 targeted the PD-L1 protein and induced PD-L1 dimerisation. We have verified by HTRF experiments that 12j-4 can block the PD-1/PD-L1 axis at the protein level. Also, at the cellular level, it is necessary to demonstrate that 12j-4 could accurately hit the target by fluorescence colocalisation experiments.
In order to determine the location of 12j-4 and PD-L1 and the interaction between the two molecules, we coupled fluorescein isothiocyanate (FITC) to 12j-4 () and then added it to the cell culture medium to observe whether 12j-4-FITC and PD-L1 were co-located. In this experiment, we still use the method with 0.5% Triton X-100 to break the membrane to control the PD-L1 antibody to stain PD-L1 protein on the cell membrane or in the cytoplasm.
Figure 7. The location of 12j-4/PD-L1 and the interaction between both molecules. (A) The structure of 12j-4-FITC. (B) PD-L1 protein expressed in organelle membranes with cell membrane ruptured; (C) PD-L1 protein expressed in organelle membranes with cell membrane non-ruptured. Representative immunofluorescence images of PD-L1 (red), nucleic (blue), and 12j-4 (green) foci in MDA-MB-231 cells treated with 12j-4-FITC (4 μM) for 48 h.

As shown in , in the ruptured group, 12j-4-FITC has a wide distribution range, majoring in the red fluorescence region and minoring in the blue fluorescence region, and almost no region exists alone. The overlapping area of red and green fluorescence on the cytoplasmic membrane may be on the Golgi apparatus or the lysosome, implying that the compound 12j-4-FITC recognised the PD-L1 protein and absorbed it. The PD-L1 protein in the overlapping region of red, green, and blue fluorescence is thought to be the result of internalising nuclear transfer induced by 12j-4-FITC.
While in the non-ruptured group (), PD-L1 protein was mainly displayed on the cell membranes, and 12j-4-FITC was widely distributed and hardly overlapped with the blue fluorescence region (nucleus). The position specified by the white arrow means the area where red and green fluorescence is highly overlapped on the cell membrane, which 12j-4-FITC recognises and binds to the PD-L1 protein.
PD-L1 protein dimerisation inhibits the AKT-mTOR pathway
It has been reported that the dimerisation of PD-L1 protein is directly related to AKT phosphorylation, which in turn affects the activation of the AKT-mTOR pathwayCitation32. Through fluorescence co-localization assay (), PD-L1 and p-AKT are proven to be contacted directly in MDA-MB-231 cells. We can find multiple regions where the fluorescence of the two proteins overlaps (white arrows). What’s more, it can be observed that p-AKT (the green spot) significantly decreased in the presence of 12j-4 compared to the control.
Figure 8. Blocking of the AKT/mTOR pathway by 12j-4 in MDA-MB-231 cells. (A) Representative immunofluorescence images of PD-L1 (red), p-AKT (green), and nucleic (blue) foci in MDA-MB-231 cells treated with 12j-4 (4 μM) for 24 h. (B) Detection of the expression of AKT, p-AKT, mTOR, and p-mTOR proteins by Western blot in MDA-MB-231 cells treated with compound 12j-4 for 48 h at a concentration of 1, 2, and 4 μM, respectively. Data are expressed as mean ± SEM (n = 3). *p < 0.05, **p < 0.01, ***p < 0.001.

Furthermore, as shown in , the inhibition of the AKT-mTOR pathway was verified by Western blot. Experimental results indicate that in MDA-MB-231, the expression of AKT did not change significantly under the condition of 12j-4 intervention for 48 h, while its phosphorylated form, p-AKT1 (Ser473), was significantly inhibited. The expression of AKT type I phosphorylated form decreased with the increase in the concentration of compound 12j-4, and the inhibition rate reached 90% at 4 μM. The phosphorylation of the mTOR protein downstream of AKT was also severely inhibited. The expression of the phosphorylated form of mTOR decreased with the increase in the concentration of compound 12j-4, and the inhibition rate reached 40% at 4 μM. The activation of the AKT-mTOR pathway explained the occurrence of apoptosis and the activation of the apoptosis pathway under the intervention of 12j-4 in MDA-MB-231 cells. Not only that, inhibition of AKT I phosphorylation also releases the ability of GSK-3β. Studies have shown that inhibition of the AKT-mTOR pathway indirectly affects the transcriptional stage of the B7-H1 gene, ultimately leading to a decrease in PD-L1 protein expression. Taken together, inhibition of the AKT-mTOR pathway explains the occurrence of decreased PD-L1 protein expression.
Collectively, these results demonstrated that the compound 12j-4 could induce tumour cell apoptosis without an immune system. 12j-4 binds to the PD-L1 protein in the cytoplasm via the non-immune pathway, causing the tumour cell to undergo apoptosis and less migration. Also, 12j-4 regulates the expression of PD-L1 by inhibiting the phosphorylation of AKT.
Compound 12j-4 suppresses the growth of MDA-MB-231 in the mouse model
The aforementioned results demonstrated the significant inhibition of survival and migration of MDA-MB-231 cells by 12j-4 in vitro via non-immune pathways. To further investigate the anti-tumour effect of the small molecular compound in vivo, an MDA-MB-231 tumour-bearing nude mouse model was established and the assay was divided into four groups: a control group, 12j-4 high/low dose groups, and a cyclophosphamide (CTX) group. Following nine consecutive administrations, the control group exhibited an average tumour volume of 1265.15 mm3, whereas the tumour growth of the 12j-4-treated group was significantly impeded, with inhibition rates of 39.04% (10 mg/kg) and 62.54% (20 mg/kg) (as shown in ). This result indicated that the compound 12j-4 has the ability to inhibit tumour growth in nude mice mainly through a non-immune pathway.
Figure 9. Anti-tumour effect of 12j-4 in nude mice. (A) 12j-4 inhibited tumour growth as indicated by the tumour volume. (B) Body weight curve of mice during treatment. (C) Tumour weight. Data are expressed as mean ± SEM (n = 3). *p < 0.05, **p < 0.01, ***p < 0.001.

On the basis of the above experiments, we found that compound 12j-4 can bind to PD-L1 protein and induce dimerisation of PD-L1 protein, thus blocking the downstream AKT/mTOR signal pathway and promoting the degradation of PD-L1 protein. A scheme for the proposed molecular mechanism of 12j-4 is depicted in .
Conclusions
In this study, we assessed the inhibitory activity of ten small molecular compounds (7b-2, 7b-4, 12j-3–4, 13b-4–6, and 13h-1–3) against 18 different tumour cell lines, in addition to their effect on the PD1/PD-L1 interaction. Of note, compound 12j-4 demonstrated the lowest IC50 for PD1/PD-L1 blocking and exhibited a superior anti-tumour effect on breast cancer MDA-MB-231 cells, characterised by high PD-L1 expression. Further investigation into the anti-tumour activity of 12j-4 revealed that 12j-4 effectively inhibited the clone formation and migration of MDA-MB-231 cells. Besides, 12j-4 significantly induced apoptosis, as evidenced by the down-regulation of the anti-apoptotic protein Bcl-2 and an increase in the levels of cleaved-Caspase3 and cleaved-PARP in the absence of T cells. Mechanistically, it was observed that 12j-4 bound with the PD-L1 protein and induced PD-L1 dimerisation. This led to preventing PD-L1 from activating the AKT/mTOR signal, reducing the level of AKT phosphorylation, and restoring GSK-3β activity. Ultimately, this promoted the degradation of PD-L1 in the cytoplasm. To confirm the in vitro results, nude mice xenograft models of MDA-MB-231 cells were established. As we expected, 12j-4 effectively inhibited tumour growth in nude mice without relying on the immune system. The biphenyl compounds were proved to have the potential to be the prototype of therapeutics as PD-L1 inhibitors to treat cancers via the immune and non-immune systems.
This study presents a novel insight into the mechanism underlying the inhibition of cancer cell proliferation and apoptosis induction by biphenyl compounds in the absence of immune cells. Notably, this is the first report to elucidate this mechanism. Our findings reveal a promising avenue for developing PD-L1 targeted immunotherapy drugs, which involve using small molecules to regulate the endogenous signal of PD-L1, thereby restoring anti-tumour immunity and inhibiting tumour growth. This strategy is particularly significant for patients with low or depleted immune cell activity in the TME, as it may enhance their response to immune checkpoint-blocking therapy.
Author contributions
The work was done through the contributions of all the authors. All authors have approved the final version of the manuscript.
Supplemental Material
Download PDF (2.1 MB)Disclosure statement
No potential conflict of interest was reported by the author(s).
Additional information
Funding
References
- Bianchini G, Balko JM, Mayer IA, Sanders ME, Gianni L. Triple-negative breast cancer: challenges and opportunities of a heterogeneous disease. Nat Rev Clin Oncol. 2016;13(11):674–690.
- Emens LA. Breast cancer immunotherapy: facts and hopes. Clin Cancer Res. 2018;24(3):511–520.
- Li X, Li MH, Lian Z, Zhu H, Kong L, Wang P, Yu J. M. Prognostic role of programmed death ligand-1 expression in breast cancer: a systematic review and meta-analysis. Target Oncol. 2016;11(6):753–761.
- Bianchini G, De Angelis C, Licata L, Gianni L. Treatment landscape of triple-negative breast cancer – expanded options, evolving needs. Nat Rev Clin Oncol. 2022;19(2):91–113.
- Dong H, Zhu G, Tamada K, Chen L. B7-H1, a third member of the B7 family, co-stimulates T-cell proliferation and interleukin-10 secretion. Nat Med. 1999;5(12):1365–1369.
- Fagerberg L, Hallström BM, Oksvold P, Kampf C, Djureinovic D, Odeberg J, Habuka M, Tahmasebpoor S, Danielsson A, Edlund K, et al. Analysis of the human tissue-specific expression by genome-wide integration of transcriptomics and antibody-based proteomics. Mol Cell Proteomics. 2014;13(2):397–406.
- Kataoka K. Genetic alterations involving PD-L1/PD-L2 in human malignancies. Gan to Kagaku Ryoho. 2019;46:841–844.
- Wang SD, Bajorath J, Flies DB, Dong HD, Honjo T, Chen LP. Molecular modeling and functional mapping of B7-H1 and B7-DC uncouple costimulatory function from PD-1 interaction. J Exp Med. 2003;197(9):1083–1091.
- Aghbash PS, Eslami N, Shamekh A, Entezari-Maleki T, Baghi HB. SARS-CoV-2 infection: the role of PD-1/PD-L1 and CTLA-4 axis. Life Sci. 2021;270:119124.
- Zuazo M, Arasanz H, Granda MJG, Bocanegra AI, Fernández-Hinojal G, Gato-Cañas M, Aguillo MM, Marín BH, Teijeira L, Ruiz IM, et al. Functional systemic CD4 immunity is required for clinical responses to PD-L1/PD-1 blockade therapy. Ann Oncol. 2019;30:v504.
- Wang T, Cai S, Cheng Y, Zhang W, Wang M, Sun H, Guo B, Li Z, Xiao Y, Jiang S, et al. Discovery of small-molecule inhibitors of the PD-1/PD-L1 axis that promote pd-l1 internalization and degradation. J Med Chem. 2022;65(5):3879–3893.
- Zhan MM, Hu XQ, Liu XX, Ruan BF, Xu J, Liao C. From monoclonal antibodies to small molecules: the development of inhibitors targeting the PD-1/PD-L1 pathway. Drug Discov Today. 2016;21(6):1027–1036.
- Naidoo J, Page DB, Li BT, Connell LC, Schindler K, Lacouture ME, Postow MA, Wolchok JD. Toxicities of the anti-PD-1 and anti-PD-L1 immune checkpoint antibodies. Ann Oncol. 2016;27(7):1362.
- Ansell SM, Lesokhin AM, Borrello I, Halwani A, Scott EC, Gutierrez M, Schuster SJ, Millenson MM, Cattry D, Freeman GJ, et al. PD-1 blockade with nivolumab in relapsed or refractory Hodgkin’s lymphoma. N Engl J Med. 2015;372(4):311–319.
- Antonia SJ, Villegas A, Daniel D, Vicente D, Murakami S, Hui R, Kurata T, Chiappori A, Lee KH, de Wit M, et al. Overall survival with durvalumab after chemoradiotherapy in stage III NSCLC. N Engl J Med. 2018;379(24):2342–2350.
- Glazar DJ, Johnson M, Farinhas J, Steuer CE, Saba NF, Bonomi M, Chung CH, Enderling H. Early response dynamics predict treatment failure in patients with recurrent and/or metastatic head and neck squamous cell carcinoma treated with cetuximab and nivolumab. Oral Oncol. 2022;127:105787.
- Motzer RJ, Escudier B, McDermott DF, George S, Hammers HJ, Srinivas S, Tykodi SS, Sosman JA, Procopio G, Plimack ER, et al. Nivolumab versus everolimus in advanced renal-cell carcinoma. N Engl J Med. 2015;373(19):1803–1813.
- Muro K, Chung HC, Shankaran V, Geva R, Catenacci D, Gupta S, Eder JP, Golan T, Le DT, Burtness B, et al. Pembrolizumab for patients with PD-L1-positive advanced gastric cancer (KEYNOTE-012): a multicentre, open-label, phase 1b trial. Lancet Oncol. 2016;17(6):717–726.
- Neal JW, Kundu P, Tanaka T, Enquist I, Patel S, Balestrini A, Wang J, Newsom-Davis T, Goto Y, Pavlakis N, et al. CONTACT-01: a phase III, randomized study of atezolizumab plus cabozantinib versus docetaxel in patients with metastatic non-small cell lung cancer (mNSCLC) previously treated with PD-L1/PD-1 inhibitors and platinum-containing chemotherapy. JCO. 2021;39(15_suppl):TPS9134–TPS9134.
- Reck M, Rodríguez-Abreu D, Robinson AG, Hui R, Csőszi T, Fülöp A, Gottfried M, Peled N, Tafreshi A, Cuffe S, et al. Pembrolizumab versus chemotherapy for PD-L1-positive non-small-cell lung cancer. N Engl J Med. 2016;375(19):1823–1833.
- Rittmeyer A, Barlesi F, Waterkamp D, Park K, Ciardiello F, von Pawel J, Gadgeel SM, Hida T, Kowalski DM, Dols MC, OAK Study Group, et al. Atezolizumab versus docetaxel in patients with previously treated non-small-cell lung cancer (OAK): a phase 3, open-label, multicentre randomised controlled trial (vol 389, pg 255, 2016). Lancet. 2017;389(10066):255–265.
- Robert C, Schachter J, Long GV, Arance A, Grob JJ, Mortier L, Daud A, Carlino MS, McNeil C, Lotem M, et al. Pembrolizumab versus ipilimumab in advanced melanoma. N Engl J Med. 2015;372(26):2521–2532.
- Zhao PF, Li L, Jiang XY, Li Q. Mismatch repair deficiency/microsatellite instability-high as a predictor for anti-PD-1/PD-L1 immunotherapy efficacy. J Hematol Oncol. 2019;12(1):54.
- Zhou C, Wang Z, Sun Y, Cao L, Ma Z, Wu R, Yu Y, Yao W, Chang J, Chen J, et al. Sugemalimab versus placebo, in combination with platinum-based chemotherapy, as first-line treatment of metastatic non-small-cell lung cancer (GEMSTONE-302): interim and final analyses of a double-blind, randomised, phase 3 clinical trial. Lancet Oncol. 2022;23(2):220–233.
- Awadasseid A, Wu YL, Zhang W. Advance investigation on synthetic small-molecule inhibitors targeting PD-1/PD-L1 signaling pathway. Life Sci. 2021;282:119813.
- Lim KH, Suresh B, Park JH, Kim YS, Ramakrishna S, Baek KH. Ubiquitin-specific protease 11 functions as a tumor suppressor by modulating Mgl-1 protein to regulate cancer cell growth. Oncotarget. 2016;7(12):14441–14457.
- Lin X, Lu X, Luo G, Xiang H. Progress in PD-1/PD-L1 pathway inhibitors: from biomacromolecules to small molecules. Eur J Med Chem. 2020;186:111876.
- Chupak LS, Zheng X. Preparation of compounds useful as immunomodulators. 2015.
- Liu L, Yao Z, Wang S, Xie T, Wu G, Zhang H, Zhang P, Wu Y, Yuan H, Sun H, et al. Syntheses, biological evaluations, and mechanistic studies of benzo[c][1,2,5]oxadiazole derivatives as potent pd-l1 inhibitors with in vivo antitumor activity. J Med Chem. 2021;64(12):8391–8409.
- Hou J, Zhao R, Xia W, Chang C-W, You Y, Hsu J-M, Nie L, Chen Y, Wang Y-C, Liu C, et al. PD-L1-mediated gasdermin C expression switches apoptosis to pyroptosis in cancer cells and facilitates tumour necrosis. Nat Cell Biol. 2020;22(10):1264–1275.
- Wang M, Ma X, Zhou K, Mao H, Liu J, Xiong X, Zhao X, Narva S, Tanaka Y, Wu Y, et al. Discovery of pyrrole-imidazole polyamides as pd-l1 expression inhibitors and their anticancer activity via immune and nonimmune pathways. J Med Chem. 2021;64(9):6021–6036.
- Zhang D, Reyes RM, Osta E, Kari S, Gupta HB, Padron AS, Kornepati AVR, Kancharla A, Sun X, Deng Y, et al. Bladder cancer cell-intrinsic PD-L1 signals promote mTOR and autophagy activation that can be inhibited to improve cytotoxic chemotherapy. Cancer Med. 2021;10(6):2137–2152.
- Lu Y, Zhong W, Liu Y, Chen W, Zhang J, Zeng Z, Huang H, Qiao Y, Wan X, Meng X, et al. Anti-PD-L1 antibody alleviates pulmonary fibrosis by inducing autophagy via inhibition of the PI3K/Akt/mTOR pathway. Int Immunopharmacol. 2022;104:108504.
- Yu J, Qin B, Moyer AM, Nowsheen S, Tu X, Dong H, Boughey JC, Goetz MP, Weinshilboum R, Lou Z, et al. Regulation of sister chromatid cohesion by nuclear PD-L1. Cell Res. 2020;30(7):590–601.
- Almozyan S, Colak D, Mansour F, Alaiya A, Al-Harazi O, Qattan A, Al-Mohanna F, Al-Alwan M, Ghebeh H. PD-L1 promotes OCT4 and Nanog expression in breast cancer stem cells by sustaining PI3K/AKT pathway activation. Int J Cancer. 2017;141(7):1402–1412.
- Chen C, Li S, Xue J, Qi M, Liu X, Huang Y, Hu J, Dong H, Ling K. PD-L1 tumor-intrinsic signaling and its therapeutic implication in triple-negative breast cancer. JCI Insight. 2021;6(8):e131458.
- Clark CA, Gupta HB, Sareddy G, Pandeswara S, Lao S, Yuan B, Drerup JM, Padron A, Conejo-Garcia J, Murthy K, et al. Tumor-intrinsic pd-l1 signals regulate cell growth, pathogenesis, and autophagy in ovarian cancer and melanoma. Cancer Res. 2016;76(23):6964–6974.
- Dong P, Xiong Y, Yue J, Hanley SJB, Watari H. Tumor-intrinsic PD-L1 signaling in cancer initiation, development and treatment: beyond immune evasion. Front Oncol. 2018;8:386.
- Hudson K, Cross N, Jordan-Mahy N, Leyland R. The extrinsic and intrinsic roles of PD-L1 and its receptor PD-1: implications for immunotherapy treatment. Front Immunol. 2020;11:568931.
- Cheng B, Xiao Y, Xue M, Cao H, Chen J. Recent advances in the development of PD-L1 modulators: degraders, downregulators, and covalent inhibitors. J Med Chem. 2020;63(24):15389–15398.
- Cotton AD, Nguyen DP, Gramespacher JA, Seiple IB, Wells JA. Development of antibody-based PROTACs for the degradation of the cell-surface immune checkpoint protein PD-L1. J Am Chem Soc. 2021;143(2):593–598.
- Liu R, Chen Y, Liu G, Li C, Song Y, Cao Z, Li W, Hu J, Lu C, Liu Y, et al. PI3K/AKT pathway as a key link modulates the multidrug resistance of cancers. Cell Death Dis. 2020;11(9):797.