Abstract
Alzheimer’s disease (AD) is a chronic, progressive brain degenerative disease that is common in the elderly. So far, there is no effective treatment. The multi-target-directed ligands (MTDLs) strategy has been recognised as the most promising approach due to the complexity of the pathogenesis of AD. Herein, novel salicylic acid–donepezil–rivastigmine hybrids were designed and synthesised. The bioactivity results exhibited that 5a was a reversible and selective eqBChE inhibitor (IC50 = 0.53 μM), and the docking provided the possible mechanism. Compound 5a also displayed potential anti-inflammatory effects and significant neuroprotective effect. Moreover, 5a exhibited favourable stabilities in artificial gastrointestinal solution and plasma. Finally, 5a demonstrated potential cognitive improvement in scopolamine-induced cognitive dysfunction. Hence, 5a was a potential multifunctional lead compound against AD.
Introduction
Alzheimer’s disease (AD) is a chronic and progressive neurodegenerative disease of the brain that commonly occurs in the elderlyCitation1. So far, more than 55 million people worldwide are estimated to have dementia, and by 2050, the figure will rise to 139 millionCitation2. Currently, there is no known cure for AD or alters the disease process in the brainCitation3.
Although many efforts have been made to study AD, the exact pathogenesis is still not clear. Several abnormal brain changes (including amyloid-β (Aβ) aggregations, levels of acetylcholine (ACh) decreased and neuroinflammation) have been suggested to serve as important roles in the pathogenesis of AD. Thus, the multi-target-directed ligands (MTDLs) approach has been recognised and developed as the most promising approach due to the complex pathogenesisCitation4–6.
Based on the cholinergic hypothesis, three AChE inhibitors (donepezil, galanthamine, and rivastigmine) have been marketed to treat AD by recovering ACh levels. While, long-term clinical uses indicate that the above drugs can only locally relieve the symptoms of AD, and the inhibition of peripheral AChE leads to nausea, dizziness, and vomitingCitation7. Furthermore, as AD progressed, AChE levels in the AD brains sharply decreased by 90%, while the levels of BuChE increased to 165% compared with normal levels, indicating that BuChE takes over AChE to hydrolyse ACh in progressive ADCitation8. Therefore, inhibition of BuChE could be a potential anti-AD approach.
The amyloid hypothesis states that the production and accumulation of Aβ is the key pathogenesis leading to ADCitation9. Aβ oligomeric aggregates show remarkable neurotoxicity. Moreover, Aβ is able to enter mitochondria where it increases ROS production and induces oxidative stress, and further damages biological macromolecules such as lipids, DNA, and proteins. Furthermore, neuroinflammation is also a major pathogenesis in AD patients and is associated with astrocytes, microglia, and inflammatory factorsCitation10. Investigations have shown that ADf patients have significantly increased levels of proinflammatory cytokines (such as IL-6, TNF-α, and IL-1β) in serum and brain, which further increase Aβ deposition and lead to cognitive dysfunction and neuron lossCitation11,Citation12. Hence, anti-inflammation is an effective treatment for AD.
Salicylic acid (SA) is a non-steroidal anti-inflammatory drug found mainly in vegetables and fruits. The study shows that SA exhibits weak anticholinesterase activity (IC50 = 346.0 μM)Citation13. As we all known, 1-benzylpiperidine of donepezil is the key pharmacophore, which has been applied for the development of novel ChEs inhibitorsCitation4,Citation14. In our previous work, 1-benzylpiperidine has been introduced into SA to develop as BuChE inhibitorCitation15. In addition, rivastigmine is an FDA-approved dual AChE/BuChE inhibitor and the carbamate fragment is the pharmacophore, and many selective BuChE inhibitors have been developed by introducing carbamate fragmentCitation16–19. Therefore, based on the SA skeleton, novel SA–donepezil–rivastigmine hybrids were designed and synthesised by the simultaneous introduction of benzylpiperidine and carbamate fragments (), hoping the optimised compounds possess multiple properties including BuChE inhibition, anti-inflammation, and neuroprotective effects.
Results and discussion
Chemistry
The synthetic route of target compounds 5a–5h is described in Scheme 1. Briefly, the starting materials 1a–1b (2,4-dihydroxybenzoic acid 1a; 2,5-dihydroxybenzoic acid 1b) were reacted with 4-benzylpiperidine (2) in the presence of EDCI and HOBT in THF to gain compounds 3a and 3b. Finally, compound 3a or 3b was reacted with excessive amounts of N,N-disubstituted carbamoyl chlorides (4a–4e), respectively, to afford target compounds 5a–5h.
Biological activity
Inhibition effects of target compounds on AChE and BuChE
The eeAChE (from electric eel)/eqBuChE (from equine serum) inhibitory activity of compounds 5a–5h were evaluated through Ellman’s methodCitation20,Citation21. Donepezil and rivastigmine were also evaluated as control compounds. The results are displayed in , overall speaking, 5a–5h demonstrated significant AChE/BuChE inhibitory potency compared with the starting material 1a and 1b, suggesting that the introduction of carbamate fragments into SA skeleton could improve AChE/BuChE inhibitory effects. 5a–5f exhibited significant eqBuChE inhibitory activity (IC50 values ranging from 0.53 μM to 13.1 μM) and potent eeAChE inhibitory activity (IC50 was ranging from 6.3 μM to 22.3 μM). The introduction of carbamate fragment increased the AChE/BuChE inhibition. 5a with dimethylcarbamoyl fragment indicated good eeAChE inhibitory potency (IC50 = 7.8 μM). When the dimethylcarbamate fragment of 5a was replaced with the N-ethyl-N-methylcarbamate fragment to obtain 5b, the eeAChE inhibitory capacity was 6.3 μM, when changing dimethylcarbamoyl fragment of 5a with diisopropylcarbamoyl fragment to get 5c (IC50 = 7.1 μM), no significant change in eeAChE inhibitory potency was produced. In contrast, when the dimethylcarbamoyl fragment of 5a was displaced with 1-pyrrolidinecarbonyl and 4-morpholinecarbonyl fragment to obtain 5d and 5e, respectively, the inhibitory capacity of eeAChE sharply reduced to 22.3 μM and 18.3 μM, respectively, suggesting that 1-pyrrolidinecarbonyl and 4-morpholinecarbonyl fragment negatively affected the eeAChE inhibitory potency. Furthermore, compound 5a showed excellent eqBuChE inhibitory potency with IC50 value of 0.53 μM, when the dimethylcarbamoyl fragment of 5a was replaced with N-ethyl-N-methylcarbamoyl fragment to get 5b, the eqBuChE inhibitory potency significantly decreased to 2.6 μM, when the dimethylcarbamoyl fragment of 5a was replaced with diisopropylcarbamoyl, 1-pyrrolidinecarbonyl, and 4-morpholinecarbonyl fragment to afford compounds 5c–5e, the eqBuChE sharply reduced to 10.8 μM, 8.7 μM, and 13.1 μM, respectively. Further, the position of carbamate fragment also influenced the inhibitory potency of eeAChE and eqBuChE. When the carbamate fragment at 4-position was displaced with carbamate fragment at 5-position to afford compounds 5f–5h, it showed significant eeAChE inhibitory capacity (IC50 values ranging from 4.9 μM to 8.2 μM) and weak eqBuChE inhibitory activity (IC50 was ranging from 13.5 μM to 16.7 μM). Compared with compound 5a, compound 5f showed significant eeAChE inhibitory capacity (IC50 = 5.2 μM) and weak eqBuChE inhibitory capacity (IC50 = 16.3 μM). 5g demonstrated a potential eeAChE inhibition capacity (IC50 = 4.9 μM) and a sharp decrease in eqBuChE to 13.5 μM compared to 5b. Compound 5h showed similar eeAChE inhibitory potency (IC50 = 8.2 μM) and eqBuChE inhibitory capacity (IC50 = 16.7 μM) compared with compound 5c, respectively. Totally speaking, 5a was a promising eqBuChE inhibitor, deserving further investigation.
Table 1. The inhibitory capacity of AChE/BuChE by 5a–5h, rivastigmine and donepezil.
The reversibility of BuChE inhibition by 5a
To determine whether 5a was a reversible BuChE inhibitor, we evaluated the recovery capability of eqBuChE inhibitors inhibition after dilution to 0.1 × IC50, rivastigmine and donepezil were also evaluated as control compoundsCitation22. As exhibited in , the eqBuChE activity restored to 2.7% when donepezil was diluted to 0.1 × IC50, while no significant recovery of eqBuChE activity occurred when rivastigmine and 5a was diluted to 0.1 × IC50, respectively. Further, the recovery capability of BuChE inhibitory effect after dilution was monitored by time. As indicated in , donepezil at 0.1 × IC50 recovered BuChE activity to 101.3% at 120 min, and rivastigmine at 0.1 × IC50 gradually restored BuChE activity to 68.7% at 120 min, suggesting that donepezil was a reversible huAChE inhibitor and rivastigmine was a pseudo-irreversible huAChE inhibitor. Under the same conditions, the 0.1 × IC50 5a gradually restored BuChE activity to 63.9% at 120 min, which was similar with rivastigmine. Therefore, the data showed that 5a was a pseudo-irreversible BuChE inhibitor.
Docking study
The possible binding action of 5a with huBChE (PDB code: 4tpk) was carried out by molecular dockingCitation20,Citation23,Citation24. In the 5a−huBuChE complex (), the benzene ring of SA formed one π–π interaction (5.0 Å) with key residue Phe329, the benzene ring of 4-benzylpiperidine formed one π–π interaction (5.8 Å) with key amino acid residue Phe329. The benzene ring of 4-benzylpiperidine formed two π–π interactions (3.8 Å and 4.8 Å, respectively) with key amino acid residue Trp231. Furthermore, hydrophobic interactions were also found between 5a and residues Trp82, Phe329, His438, Trp231, Leu286, Gly117, Val288, and Trp430. Thus, the multi-interactions of huBuChE−5a complex might provide possible mechanism for the high BuChE inhibitory capability.
Anti-inflammation of 5a
5a was chosen to evaluate the anti-inflammation property through testing the production of IL-1β, IL-6, and NO in LPS-induced BV-2 cellsCitation25,Citation26. First of all, the cytotoxicity of 5a was determined through the CCK-8 assay. As demonstrated in , compound 5a did not produce significant cytotoxicity on BV-2 cells at 10 μM.
Figure 4. The cell viability was determined using CCK-8 assay. The results were indicated as the mean ± SD.
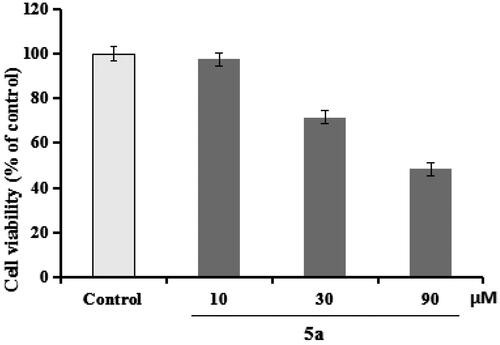
Evaluation of the production of IL-6, IL-1β, and NO in LPS-stimulated BV-2 cells
Compound 5a was selected to evaluate the anti-inflammation property, and the positive drug donepezil was also tested as comparison purpose. As displayed in , when BV-2 cells were treated with LPS (1 μg/mL), the level of IL-6 significantly elevated to 1805.2 pg/mL (p < 0.01) compared with normal group (100.5 pg/mL), when treating with 5a (1, 3, and 9 μM), the levels of IL-6 remarkably declined to 456.2 pg/mL, 505.3 pg/mL, and 971.1 pg/mL, respectively. Furthermore, shows that the levels of IL-1β significantly elevated to 302.7 pg/mL (p < 0.01) after the addition of 1 μg/mL LPS, when treating with 5a (1, 3, and 9 μM), the levels of IL-1β remarkably declined to 67.2 pg/mL, 90.9 pg/mL, and 163.4 pg/mL, respectively. Moreover, as demonstrated in , when BV-2 cells were treated with LPS (1 μg/mL), the release volume of NO remarkably elevated to 31.9 μM (p < 0.01), when treating with 5a (1, 3, and 9 μM), the release volume of NO remarkably declined. The data showed that 5a exhibited good anti-inflammatory activity compared with the positive drug donepezil.
Figure 5. (A) Effects of compounds 5a and donepezil on the production of IL-6; (B) effects of compound 5a and donepezil on the production of IL-1β; (C) effects of compound 5a and donepezil on NO release. The results were expressed as the mean ± SD. *p < 0.05, **p < 0.01 vs. model group; ##p < 0.01 vs. control.
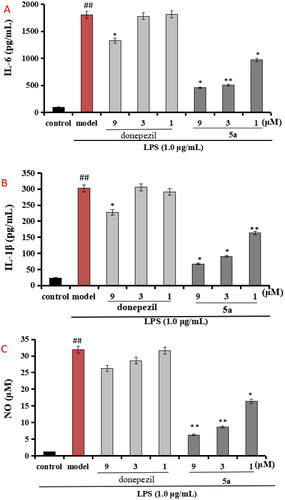
Neuroprotective effects of 5a
The CCK-8 assay was applied to assess the neuroprotective effect of 5a on Aβ25-35-induced injury in PC12 cell, and donepezil was also tested as comparison purposeCitation27,Citation28. shows that compound 5a shows no obvious cytotoxicity on PC12 cells at 30 μM. In addition, as demonstrated in , donepezil showed potent neuroprotective effect at 9 μM, and when PC12 cells were exposed to 25 μM Aβ25-35 aggregates, the cell viability was reduced to 46.7% (p < 0.01). When treatment with 5a (1, 3, and 9 μM), the PC12 cells viability improved to 61.7% (p < 0.05), 68.6% (p < 0.01), and 83.3% (p < 0.01), respectively. The data demonstrated that compound 5a presented remarkable neuroprotective effects on Aβ25-35-induced PC12 cell injury compared with donepezil.
Prediction of drug-likeness
The QikProp module from Schröginger molecular modelling software was chosen to calculate the ADME property of 5aCitation4. The predictive indicators include molecular weight, hydrogen bond donors, hydrogen bond acceptors, polar surface area, hydrophilicity, permeability, and human oral absorption parameters. As demonstrated in Table S1, compound 5a complied with both Lipinski’s rule of five. To sum up, compound 5a demonstrated good drug-like properties.
Stability studies of compound 5a
Based on the above data, 5a was chosen to evaluate the stabilities by artificial gastrointestinal solution, blood plasma, and rat liver microsomesCitation29. As demonstrated in , the residual amount of 5a was 99.5%, 95.6%, 97.5%, and 110.6% after 8 h incubation in blank gastric juice, artificial gastric juice, blank intestinal juice, and artificial intestinal juice, respectively. The data suggested that 5a was stable in artificial intestinal and gastric fluids.
Moreover, shows that compound 5a displayed moderate stability in rat liver microsomes (t1/2 = 19.20 min) and favourable stability in blood plasma (t1/2 = 3.25 h).
Effects of compound 5a on scopolamine-induced cognitive dysfunction in mice
The effects of 5a on scopolamine-induced cognitive dysfunction in mice were accessed by the step-down passive avoidance taskCitation21,Citation22. First, the safety profile of 5a was determined by Kunming mice (18–22 g, eligibility certification no. SCXK-Xiang2019-0004) at doses of 500 and 250 mg/kg through intragastric administration. The results showed that the mice did not present abnormal behaviour at the dose of 500 mg/kg.
The in vivo effects of 5a were determined through the step-down passive avoidance task. As demonstrated in , when mice was given scopolamine at a dose of 2.0 mg/kg, the step-down latency remarkably reduced to 42.1 s (p < 0.01). When treatment with donepezil at a dose of 2.0 mg/kg, the step-down latency sharply increased to 144.3 s (p < 0.01). When treating with 5a (1.23, 2.45, and 4.9 mg/kg), the step-down latency elevated to 140.1 s (p < 0.01), 77.8 s (p < 0.01), and 105.2 s (p < 0.01), respectively, suggesting that 5a produced a precognitive effect on scopolamine-induced cognitive dysfunction in mice at a dose of 1.23 mg/kg.
Conclusions
To date, there are no effective drugs capable of halting or reversing the progression of AD. As the complex pathogenesis of AD, MTDLs approach is regarded as the potential treatment. Thus, novel SA–donepezil–rivastigmine hybrids were designed and synthesised by the simultaneous introduction of benzylpiperidine and carbamate fragments. The results demonstrated that 5a was a reversible and selective eqBChE inhibitor (IC50 = 0.53 μM). 5a demonstrated potential anti-inflammation property by inhibiting the level of IL-6, IL-1β, and NO production, and significant neuroprotective effect on Aβ25-35-induced PC12 cell injury. Moreover, 5a exhibited favourable stabilities in artificial gastrointestinal fluids, liver microsomes, and plasma. Finally, 5a produced a precognitive effect on scopolamine-induced cognitive dysfunction in mice. All in all, 5a was a potential multi-targeted anti-AD compound.
Experimental
Chemistry
All the chemicals and solvents were from commercial companies. The reactions were monitored by TLC, and crude products were purified by column chromatography. The HR-MS was afforded from a mass spectrometer (Waters Xevo G2-XS-Qtof, Milford, MA). The 1H NMR and 13C NMR spectra of compounds were afforded on a Varian INOVA at 400 and 100 MHz, respectively.
The synthesis of compounds 3a and 3b was presented in supporting information
General preparation procedures of the target compounds 5a–5h
3a or 3b (2.0 mmol) and K2CO3 (3.5 mmol) were added to CH3CN solution (10 mL). Under ice-water bath conditions, N,N-disubstituted carbamoyl chlorides (4a–4e) (3.2 mmol) were added to the reaction. The reaction solution was reacted for 15–18 h under reflux conditions. When the reaction was complete, the solvent was evaporated to dryness under reduced pressure. Water (30 mL) was added to the residue and extraction was carried out using CH2Cl2 (2 × 30 mL). The combined organic phases were washed with saturated sodium chloride (50 mL) and dried with anhydrous sodium sulphate. The solvent was dried under reduced pressure to afford the crude product, which was further purified by column chromatography using petroleum ether/ethyl acetate (50:1) as an eluent to gain the target compounds 5a–5h.
4-(4-Benzylpiperidine-1-carbonyl)-1,3-phenylene bis(dimethylcarbamate) (5a)
3a was reacted with dimethylcarbamoyl chloride (4a) according to the above general preparation to gain target derivative 5a. Light yellow oil, 50.6% yield and 96.7% HPLC purity. 1H NMR (400 MHz, CDCl3) δ 7.28 (t, J = 7.2 Hz, 2H, 2 × Ar-H), 7.19 (t, J = 7.2 Hz, 2H, 2 × Ar-H), 7.13 (s, 1H, Ar-H), 7.11–7.09 (m, 2H, 2 × Ar-H), 7.02 (d, J = 7.2 Hz, 1H, Ar-H), 4.70 (d, J = 12.8 Hz, 1H, 1/2 PhCH2), 3.61 (d, J = 12.8 Hz, 1H, 1/2 PhCH2), 3.06 (s, 3H, NCH3), 3.02–2.93 (m, 10H, 3 × NCH3 + 1/2 NCH2), 2.88 (t, J = 13.2 Hz, 1H, 1/2 CH2), 2.66–2.63 (m, 1H, 1/2 NCH2), 2.54–2.52 (m, 2H, NCH2), 2.06–2.03 (m, 1H, CH), 1.75 (d, J = 10.8 Hz, 2H, CH2), 1.58 (d, J = 12.8 Hz, 1H, 1/2 CH2). 13C NMR (100 MHz, CDCl3) δ 166.4, 154.1, 152.2, 129.1, 128.3, 127.5, 126.4, 126.1, 118.5, 47.5, 43.0, 42.0, 38.6, 36.8, 36.7, 36.5, 32.5, 31.8. HR-ESI-MS: Calcd. for C25H31N3O5 [M + H]+: 454.2297, found: 454.2349.
4-(4-Benzylpiperidine-1-carbonyl)-1,3-phenylene bis(ethyl(methyl)carbamate) (5b)
3a was reacted with N-ethyl-N-methylcarbamoyl chloride (4b) according to the above general preparation to gain target derivative 5b. Light yellow oil, 43.8% yield and 96.5% HPLC purity. 1H NMR (400 MHz, CDCl3) δ 7.28 (t, J = 7.2 Hz, 2H, 2 × Ar-H), 7.19 (t, J = 7.2 Hz, 2H, 2 × Ar-H), 7.11 (d, J = 7.2 Hz, 3H, 3 × Ar-H), 7.03–7.00 (m, 1H, Ar-H), 4.70 (d, J = 12.8 Hz, 1H, 1/2 PhCH2), 3.61 (d, J = 12.8 Hz, 1H, 1/2 PhCH2), 3.46–3.34 (m, 5H, 2 × NCH2 + 1/2 NCH2), 3.03 (s, 3/2 H, 1/2 NCH3), 2.97 (s, 3H, NCH3), 2.95 (s, 3/2 H, 1/2 NCH3), 2.87 (t, J = 12.8 Hz, 1H, 1/2 NCH2), 2.65–2.61 (m, 1H, 1/2 CH2), 2.54–2.50 (m, 2H, NCH2), 1.96–1.94 (m, 1H, CH), 1.74 (d, J = 11.6 Hz, 2H, CH2), 1.57 (d, J = 12.8 Hz, 1H, 1/2 CH2), 1.21–1.14 (m, 6H, 2 × CH3). 13C NMR (100 MHz, CDCl3) δ 166.4, 153.8, 153.2, 152.2, 148.2, 139.9, 129.1, 128.3, 127.5, 126.4, 126.1, 118.6, 116.9, 116.7, 116.6, 53.5, 47.5, 44.2, 43.0, 42.0, 38.3, 38.2, 34.3, 33.9, 33.8, 32.6, 31.7, 13.2, 12.6, 12.4. HR-ESI-MS: Calcd. for C27H35N3O5 [M + H]+: 482.2610, found: 482.2648.
4-(4-Benzylpiperidine-1-carbonyl)-1,3-phenylene bis(diisopropylcarbamate) (5c)
3a was reacted with diisopropylcarbamoyl chloride (4c) according to the above general preparation to gain target derivative 5c. Light yellow oil, 32.9% yield and 96.6% HPLC purity. 1H NMR (400 MHz, CDCl3) δ 7.27 (t, J = 7.6 Hz, 2H, 2 × Ar-H), 7.19 (t, J = 7.2 Hz, 2H, 2 × Ar-H), 7.15–7.08 (m, 5H, 5 × Ar-H), 4.68 (d, J = 12.8 Hz, 1H, 1/2 PhCH2), 4.14–3.83 (m, 5H, 1/2 PhCH2 + 4 × NCH), 3.70 (t, J = 12.8 Hz, 1H, 1/2 NCH2), 2.97–2.82 (m, 1H, 1/2 NCH2), 2.71–2.58 (m, 1H, 1/2 CH2), 2.53–2.41 (m, 2H, NCH2), 1.87–1.82 (m, 1H, CH), 1.72 (d, J = 10.8 Hz, 2H, CH2), 1.60 (d, J = 11.6 Hz, 1H, 1/2 CH2), 1.33 (d, J = 6.4 Hz, 12H, 4 × CH3), 1.24 (d, J = 6.4 Hz, 12H, 4 × CH3). 13C NMR (100 MHz, CDCl3) δ 166.0, 153.4, 140.0, 129.7, 129.0, 128.3, 126.0, 122.9, 122.8, 120.3, 47.5, 46.7, 46.6, 43.1, 43.0, 42.0, 38.4, 31.6, 21.7, 21.6, 21.5, 21.3, 20.7, 20.6, 20.5. HR-ESI-MS: Calcd. for C33H47N3O5 [M + H]+: 566.3549, found: 566.3602.
4-(4-Benzylpiperidine-1-carbonyl)-1,3-phenylene bis(pyrrolidine-1-carboxylate) (5d)
3a was reacted with 1-pyrrolidinecarbonylchloride (4d) according to the above general preparation to gain target derivative 5d. Light yellow oil, 33.2% yield and 96.5% HPLC purity. 1H NMR (400 MHz, CDCl3) δ 7.28 (t, J = 7.2 Hz, 2H, 2 × Ar-H), 7.19 (t, J = 6.4 Hz, 2H, 2 × Ar-H), 7.11 (d, J = 7.2 Hz, 3H, 3 × Ar-H), 7.04 (d, J = 8.0 Hz, 1H, Ar-H), 4.70 (d, J = 12.8 Hz, 1H, 1/2 PhCH2), 3.60 (d, J = 12.8 Hz, 1H, 1/2 PhCH2), 3.53 (t, J = 6.8 Hz, 2H, NCH2), 3.46 (t, J = 6.8 Hz, 2H, NCH2), 3.43–3.40 (m, 1H, 1/2 NCH2), 3.38 (t, J = 6.8 Hz, 4H, 2 × NCH2), 2.92–2.83 (m, 1H, 1/2 NCH2), 2.68–2.62 (m, 1H, 1/2 CH2), 2.55–2.52 (m, 2H, NCH2), 1.97–1.85 (m, 9H, 4 × CH2 + CH), 1.76–1.74 (m, 2H, CH2), 1.58 (d, J = 12.8 Hz, 1H, 1/2 CH2). 13C NMR (100 MHz, CDCl3) δ 166.6, 152.4, 148.6, 139.9, 129.1, 128.3, 126.1, 118.4, 112.8, 48.0, 46.5, 46.4, 25.8, 25.5, 24.9. HR-ESI-MS: Calcd. for C29H35N3O5 [M + H]+: 506.2610, found: 506.2641.
4-(4-Benzylpiperidine-1-carbonyl)-1,3-phenylene bis(morpholine-4-carboxylate) (5e)
3a was reacted with 4-morpholinecarbonyl chloride (4e) according to the above general preparation to gain target derivative 5e. Light yellow oil, 28.6% yield and 96.6% HPLC purity. 1H NMR (400 MHz, CDCl3) δ 7.28 (t, J = 7.2 Hz, 2H, 2 × Ar-H), 7.20 (t, J = 7.6 Hz, 2H, 2 × Ar-H), 7.12 (d, J = 6.4 Hz, 3H, 3 × Ar-H), 7.03 (d, J = 8.4 Hz, 1H, Ar-H), 4.70 (d, J = 12.8 Hz, 1H, 1/2 PhCH2), 3.73–3.50 (m, 17H, 4 × OCH2 + 1/2 PhCH2 + 4 × NCH2), 3.27 (t, J = 4.8 Hz, 1H, 1/2 NCH2), 2.88–2.86 (m, 1H, 1/2 CH2), 2.67–2.59 (m, 1H, 1/2 NCH2), 2.55 (d, J = 6.0 Hz, 2H, NCH2), 1.91–1.88 (m, 1H, CH), 1.77 (d, J = 11.2 Hz, 2H, CH2), 1.59 (d, J = 12.8 Hz, 1H, 1/2 CH2). 13C NMR (100 MHz, CDCl3) δ 166.1, 152.9, 152.5, 151.9, 139.7, 129.1, 128.3, 127.7, 126.6, 126.1, 118.7, 116.6, 66.6, 53.5, 47.6, 47.2, 45.0, 44.9, 44.2, 43.0, 42.1, 32.6, 31.8. HR-ESI-MS: Calcd. for C29H35N3O7 [M + H]+: 538.2509, found: 538.2565.
2-(4-Benzylpiperidine-1-carbonyl)-1,4-phenylene bis(dimethylcarbamate) (5f)
3b was reacted with dimethylcarbamoyl chloride (4a) according to the above general preparation to gain target derivative 5f. Light yellow oil, 34.3% yield and 96.8% HPLC purity. 1H NMR (400 MHz, CDCl3) δ 7.27 (t, J = 7.2 Hz, 2H, 2 × Ar-H), 7.20 (t, J = 8.8 Hz, 2H, 2 × Ar-H), 7.13–7.10 (m, 4H, 4 × Ar-H), 4.67 (d, J = 11.6 Hz, 1H, 1/2 PhCH2), 3.65 (d, J = 13.6 Hz, 1H, 1/2 PhCH2), 3.07–2.96 (m, 13H, 4 × NCH3 + 1/2 NCH2), 2.91–2.87 (m, 1H, 1/2 NCH2), 2.64 (dt, J1 = 10.8 Hz, J2 = 2.0 Hz, 1H, 1/2 CH2), 2.54–2.52 (m, 2H, NCH2), 1.85–1.82 (m, 1H, CH), 1.75 (d, J = 10.8 Hz, 2H, CH2), 1.60 (d, J = 11.6 Hz, 1H, 1/2 CH2). 13C NMR (100 MHz, CDCl3) δ 165.8, 154.4, 154.1, 148.3, 139.9, 130.2, 129.1, 128.3, 126.1, 123.7, 122.9, 120.4, 53.5, 47.5, 43.1, 43.0, 42.0, 38.3, 38.2, 36.8, 36.5, 32.4, 31.7. HR-ESI-MS: Calcd. for C25H31N3O5 [M + H]+: 454.2297, found: 454.2330.
2-(4-Benzylpiperidine-1-carbonyl)-1,4-phenylene bis(ethyl(methyl)carbamate) (5g)
3b was reacted with N-ethyl-N-methylcarbamoyl chloride (4b) according to the above general preparation to gain target derivative 5g. Light yellow oil, 39.7% yield and 97.1% HPLC purity. 1H NMR (400 MHz, CDCl3) δ 7.27 (t, J = 7.2 Hz, 2H, 2 × Ar-H), 7.23–7.17 (m, 2H, 2 × Ar-H), 7.14–7.18 (m, 4H, 4 × Ar-H), 4.68 (d, J = 12.8 Hz, 1H, 1/2 PhCH2), 3.65 (d, J = 13.2 Hz, 1H, 1/2 PhCH2), 3.45–3.38 (m, 5H, 2 × NCH2 + 1/2 NCH2), 3.04 (s, 3/2 H, 1/2 NCH3), 2.97 (s, 3H, NCH3), 2.94 (s, 3/2 H, 1/2 NCH3), 2.88 (t, J = 12.8 Hz, 1H, 1/2 NCH2), 2.65–2.59 (m, 1H, 1/2 CH2), 2.55–2.49 (m, 2H, NCH2), 1.76–1.69 (m, 3H, CH + CH2), 1.59 (d, J = 12.4 Hz, 1H, 1/2 CH2), 1.24–1.09 (m, 6H, 2 × CH3). 13C NMR (100 MHz, CDCl3) δ 165.8, 153.9, 153.6, 148.4, 148.3, 139.9, 130.2, 129.1, 128.5, 128.3, 126.1, 123.7, 122.9, 122.8, 120.4, 47.5, 47.4, 44.1, 43.1, 43.0, 41.9, 34.3, 33.9, 32.5, 31.7, 13.2, 12.5. HR-ESI-MS: Calcd. for C27H35N3O5 [M + H]+: 482.2610, found: 482.2702.
2-(4-Benzylpiperidine-1-carbonyl)-1,4-phenylene bis(diisopropylcarbamate) (5h)
3b was reacted with diisopropylcarbamoyl chloride (4c) according to the above general preparation to gain target derivative 5h. Light yellow oil, 23.6% yield and 96.5% HPLC purity. 1H NMR (400 MHz, CDCl3) δ 7.27 (t, J = 7.6 Hz, 2H, 2 × Ar-H), 7.23–7.15 (m, 2H, 2 × Ar-H), 7.13–7.08 (m, 2H, 2 × Ar-H), 7.07–7.05 (m, 1H, Ar-H), 7.03–6.98 (m, 1H, Ar-H), 4.68 (d, J = 12.0 Hz, 1H, 1/2 PhCH2), 4.06–3.88 (m, 5H, 1/2 PhCH2 + 4 × NCH), 3.65–3.61 (d, J = 8.4 Hz, 1H, 1/2 NCH2), 2.93–2.80 (m, 1H, 1/2 NCH2), 2.59–2.56 (m, 1H, 1/2 CH2), 2.54–2.51 (m, 2H, NCH2), 1.85–1.79 (m, 1H, CH), 1.73 (d, J = 10.0 Hz, 2H, CH2), 1.58 (d, J = 13.2 Hz, 1H, 1/2 CH2), 1.30–1.24 (m, 24H, 8 × CH3). 13C NMR (100 MHz, CDCl3) δ 166.5, 153.0, 152.0, 139.9, 129.1, 128.4, 127.3, 126.6, 126.1, 118.7, 117.0, 112.2, 111.0, 47.6, 46.9, 46.2, 43.1, 38.3, 32.7, 32.3, 31.7, 21.6, 21.3, 20.7, 20.5. HR-ESI-MS: Calcd. for C33H47N3O5 [M + H]+: 566.3549, found: 566.3588.
Supplemental Material
Download PDF (932 KB)Acknowledgements
This work was financially supported by the Science and Technology Project of Henan Province (No. 2121023111000); The Special Project of Nanyang Normal University (2020ZX015 and 2021CX002); The Fundamental Research Funds for Hainan University (KYQD(ZR)23002).
Disclosure statement
The authors declare no competing financial interest.
Additional information
Funding
References
- Scheltens P, De Strooper B, Kivipelto M, Holstege H, Chételat G, Teunissen CE, Cummings J, van der Flier WM. Alzheimer’s disease. Lancet. 2021;397(10284):1577–1590.
- World Alzheimer Report 2019: attitudes to dementia. Alzheimer’s Disease International; 2019. p. 1–13. https://www.alz.co.uk/research/world-report-2019.
- Cummings J, Lee G, Zhong K, Fonseca J, Taghva K. Alzheimer’s disease drug development pipeline: 2021. Alzheimers Dement. 2021;7(1):e12179.
- Sang Z, Wang K, Dong J, Tang L. Alzheimer’s disease: updated multi-targets therapeutics are in clinical and in progress. Eur J Med Chem. 2022;238:114464.
- Zhang P, Xu S, Zhu Z, Xu J. Multi-target design strategies for the improved treatment of Alzheimer’s disease. Eur J Med Chem. 2019;176:228–247.
- de Freitas Silva M, Dias KST, Gontijo VS, Ortiz CJC, Viegas C Jr. Multi-target directed drugs as a modern approach for drug design towards Alzheimer’s disease: an update. Curr Med Chem. 2018;25(29):3491–3525.
- Anand P, Singh B. A review on cholinesterase inhibitors for Alzheimer’s disease. Arch Pharm Res. 2013;36(4):375–399.
- Li Q, Yang H, Chen Y, Sun H. Recent progress in the identification of selective butyrylcholinesterase inhibitors for Alzheimer’s disease. Eur J Med Chem. 2017;132:294–309.
- Hardy J, Selkoe DJ. The amyloid hypothesis of Alzheimer’s disease: progress and problems on the road to therapeutics. Science. 2002;297(5580):353–356.
- Cheignon C, Tomas M, Bonnefont-Rousselot D, Faller P, Hureau C, Collin F. Oxidative stress and the amyloid beta peptide in Alzheimer’s disease. Redox Biol. 2018;14:450–464.
- Ozben T, Ozben S. Neuro-inflammation and anti-inflammatory treatment options for Alzheimer’s disease. Clin Biochem. 2019;72:87–89.
- Liu P, Wang Y, Sun Y, Peng G. Neuroinflammation as a potential therapeutic target in Alzheimer’s disease. Clin Interv Aging. 2022;17:665–674.
- Blacklock CJ, Lawrence JR, Wiles D, Malcolm EA, Gibson IH, Kelly CJ, Paterson JR. Salicylic acid in the serum of subjects not taking aspirin. Comparison of salicylic acid concentrations in the serum of vegetarians, non-vegetarians, and patients taking low dose aspirin. J Clin Pathol. 2001;54(7):553–555.
- Li Q, He S, Chen Y, Feng F, Qu W, Sun H. Donepezil-based multi-functional cholinesterase inhibitors for treatment of Alzheimer’s disease. Eur J Med Chem. 2018;158:463–477.
- He Y, Rao Z, Liu Z, Mi J, Yang J, Zhou Y, Yin Z, Chen P, Liu W, Zeng N, et al. Design, synthesis and evaluation of salicylic acid–donepezil hybrids as functional agents for the treatment of Alzheimer’s disease. Med Chem Res. 2023;32(2):355–368.
- Zhang H, Wang Y, Wang Y, Li X, Wang S, Wang Z. Recent advance on carbamate-based cholinesterase inhibitors as potential multifunctional agents against Alzheimer’s disease. Eur J Med Chem. 2022;240:114606.
- Sang Z, Wang K, Bai P, Wu A, Shi J, Liu W, Zhu G, Wang Y, Lan Y, Chen Z, et al. Design, synthesis and biological evaluation of novel O-carbamoyl ferulamide derivatives as multi-target-directed ligands for the treatment of Alzheimer’s disease. Eur J Med Chem. 2020;194:112265.
- Bajda M, Łątka K, Hebda M, Jończyk J, Malawska B. Novel carbamate derivatives as selective butyrylcholinesterase inhibitors. Bioorg Chem. 2018;78:29–38.
- Yamazaki DAS, Rozada AMF, Baréa P, Reis EC, Basso EA, Sarragiotto MH, Seixas FAV, Gauze GF. Novel arylcarbamate-N-acylhydrazones derivatives as promising BuChE inhibitors: design, synthesis, molecular modeling and biological evaluation. Bioorg Med Chem. 2021;32:115991.
- Sang Z, Wang K, Han X, Cao M, Tan Z, Liu W. Design, synthesis, and evaluation of novel ferulic acid derivatives as multi-target-directed ligands for the treatment of Alzheimer’s disease. ACS Chem Neurosci. 2019;10(2):1008–1024.
- Sang Z, Qiang X, Li Y, Yuan W, Liu Q, Shi Y, Ang W, Luo Y, Tan Z, Deng Y. Design, synthesis and evaluation of scutellarein-O-alkylamines as multifunctional agents for the treatment of Alzheimer’s disease. Eur J Med Chem. 2015;94:348–366.
- Sang Z, Wang K, Shi J, Cheng X, Zhu G, Wei R, Ma Q, Yu L, Zhao Y, Tan Z, et al. Apigenin-rivastigmine hybrids as multi-target-directed ligands for the treatment of Alzheimer’s disease. Eur J Med Chem. 2020;187:111958.
- Dighe SN, Deora GS, De la Mora E, Nachon F, Chan S, Parat M-O, Brazzolotto X, Ross BP. Discovery and structure–activity relationships of a highly selective butyrylcholinesterase inhibitor by structure-based virtual screening. J Med Chem. 2016;59(16):7683–7689.
- Košak U, Brus B, Knez D, Žakelj S, Trontelj J, Pišlar A, Šink R, Jukič M, Živin M, Podkowa A, et al. The magic of crystal structure-based inhibitor optimization: development of a butyrylcholinesterase inhibitor with picomolar affinity and in vivo activity. J Med Chem. 2018;61(1):119–139.
- Tian C, Qiang X, Song Q, Cao Z, Ye C, He Y, Deng Y, Zhang L. Flurbiprofen-chalcone hybrid Mannich base derivatives as balanced multifunctional agents against Alzheimer’s disease: design, synthesis and biological evaluation. Bioorg Chem. 2020;94:103477.
- Yang Z, Song Q, Cao Z, Yu G, Liu Z, Tan Z, Deng Y. Design, synthesis and evaluation of flurbiprofen–clioquinol hybrids as multitarget-directed ligands against Alzheimer’s disease. Bioorg Med Chem. 2020;28(7):115374.
- Xu P, Wang H, Li Z, Yang Z. Triptolide attenuated injury via inhibiting oxidative stress in Amyloid-Beta25-35-treated differentiated PC12 cells. Life Sci. 2016;145:19–26.
- Xie X, Wang HT, Li CL, Gao XH, Ding JL, Zhao HH, Lu YL. Ginsenoside Rb1 protects PC12 cells against β-amyloid-induced cell injury. Mol Med Rep. 2010;3(4):635–639.
- Sang Z, Bai P, Ban Y, Wang K, Wu A, Mi J, Hu J, Xu R, Zhu G, Wang J, et al. Novel donepezil–chalcone–rivastigmine hybrids as potential multifunctional anti-Alzheimer’s agents: design, synthesis, in vitro biological evaluation, in vivo and in silico studies. Bioorg Chem. 2022;127:106007.