Abstract
ATPase family AAA domain-containing protein 2 (ATAD2) has been emerging as a hot anti-cancer drugable target due to its oncogenic epigenetic modification closely associated with cancer cells proliferation, apoptosis, migration and drug resistance. In this study, we design a series of theophylline derivatives as novel ATAD2 inhibitors through fragment-based screening and scaffold growth strategy. A novel potent ATAD2 inhibitor (compound 19f) is discovered with an IC50 value of 0.27 μM against ATAD2, which adopts a combination of classic and atypical binding mode. Additionally, compound 19f could impede ATAD2 activity and c-Myc activation, induced significant apoptosis, and illustrated an anti-migration effect in BT-549 cells. Collectively, these results provide new enlightenment for the development of novel potent ATAD2 inhibitors for triple-negative breast cancer (TNBC) treatment.
Introduction
ATPase family AAA domain-containing protein 2 (ATAD2) contains a bromodomain and an ATPase domain, is an important epigenetic regulator in histone modification, identified as a coactivator of several oncogenic transcription factors, including c-Myc and E2F transcription factor 2 (E2F2) et al.Citation1,Citation2 In our previous review, ATAD2 plays an essential role in cancer chromatin remodelling, DNA replication, DNA damage, and DNA repair, and is associated with cancer cell proliferation, migration, autophagy, and cell cycle regulationCitation3. ATAD2 is recruited to the promoter of BRCA1, enhancing the efficacy of DNA-damaging chemotherapy agents and radiationCitation4. ATAD2 could directly bind to the histone acetylation site of chromatin, elevating chromatin accessibility and histone dynamics which is beneficial to oncogenic gene expressionCitation5. ATAD2 forms a complex with SOX10 to promote melanoma phenotypes through chromatin remodelingCitation6. Additionally, ATAD2 is proven to be a marker and driver of cell proliferation in ovarian cancer, and MYBL2-ATAD2 proliferative signalling axis is confirmed by Liu et al.Citation7 Due to its critical and extensive regulatory role, ATAD2 has emerged as a promising drug target for cancer treatment.
Some ATAD2 inhibitors have been discovered over the past few years for cancer treatment (). In our previous work, a novel ATAD2 inhibitor (1, AM879) was discovered by a structure-based virtual screening synergy with a biochemical analysis strategy. Structurally, the cyano group of AM879 is very important for the affinity of ATAD2/AM879 by initiating a hydrogen network with key conserved Asn1064 and Tyr1021. AM879 shows potent inhibitory activity against ATAD2 with an IC50 value of 3.5 μM and acceptable antiproliferative activity with an IC50 value of 2.43 μM in MDA-MB-231 cells. AM879 could suppress the expression of c-Myc, and induce significant apoptosis and autophagyCitation8. Another new ATAD2 inhibitor (2) is reported by Robert J. Watson, which is identified by high-throughput screening and hit qualification. A novel binding mode is observed in the X-ray structure of ATAD2 with 2, there is no hydrogen interaction initiated by Asn1064. By contrast, three key hydrogen interactions are initiated by residues Arg1007, Lys1011, and Asp1014 from the ZA loopCitation9. A structural derivative (3, GSK232) of 2 displays an improvement activity against ATAD2 with an IC50 value of 0.032 μM in an atypical binding mode, but no more cellular activity was observedCitation10. 3-methylquinolinone derivatives are identified as potent ATAD2 inhibitors, the first reported 2 digits nanomolar selective ATAD2 inhibitor is GSK8814 (4) with an IC50 value of 0.059 μM, which is used as a cell-permeable chemical probe for ATAD2 BromodomainCitation11. AZ4347 (5) is a recently reported potent ATAD2 inhibitor with antiproliferative activity in a range of breast cancer modelsCitation12. Another potent ATAD2 inhibitor is BAY-850 (6) identified by a DNA-encoded library screen, which could specifically induce ATAD2 bromodomain dimerisation and prevented interactions with acetylated histonesCitation13. Although several potent and selective ATAD2 inhibitors have been reported, most lack appreciable cellular activity and druggability, especially in inhibiting tumour growth, which limits the clinical application of ATAD2 inhibitors. The discovery of new chemical frameworks is very important for improving cell penetration and antitumor activity of ATAD2 inhibitors.
Figure 1. Several representative ATAD2 inhibitors. The pink group indicates the classical acetyl group analog unit initiating hydrogen with ASN1064 and the blue groups indicate non-classical ZA loop region binding units.
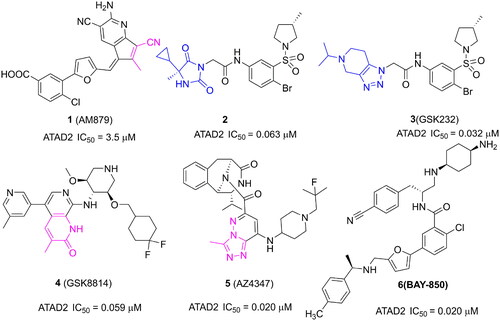
In this study, a series of theophylline derivatives are designed and synthesised through fragment-based screening and scaffold growth strategy. Compound 19f is identified as a potent ATAD2 inhibitor with an IC50 value of 0.27 μM, which could significantly inhibit BT-549 cells proliferation with an IC50 value of 5.43 μM. In addition, compound 19f was substantiated to impede ATAD2 activity and c-Myc activation, induced significant apoptosis, and showed anti-migration ability in BT-549 cells. Taken together, these findings demonstrate that compound 19f is a novel ATAD2 inhibitor, which may provide a candidate lead for future TNBC drug development.
Results and discussion
Fragment-based design novel ATAD2 inhibitors
To discover potent ATAD2 inhibitors with novel scaffolders, a fragment-based screening strategy is performed (). First, we screened a small molecule fragment library built by limiting molecular weight among 100–250 Da through the Libdocking protocolCitation14. The top 100 hits were retained to access to next processing, and the CDOCKER interaction energy was calculatedCitation15. Subsequently, we detected the affinity of the top 10 hits with ATAD2 by TR-FRET assay. The results showed that only 2 hits (TS-1, TS-2) presented weak affinity () with IC50 values of 411 μM and 324 μM respectively. Structurally, TS-2 (theophylline) is a novel scaffold for ATAD2 inhibitors and may have a non-classical binding mode similar to that of 2, while TS-1 has been reported in the previous studyCitation16. The molecular docking result suggests that theophylline binds to the ZA loop of ATAD2 bromodomain, initiating three hydrogen interactions with residues Arg1007 and Pro1012, sharing a similar conformation of 2, which indicates that theophylline is a promising start point to optimise to be novel ATAD2 inhibitors. Compared to the structure of 2, we induced a chain of two carbon atoms as the linker to expand into the classical active centre (Asn1064). Compound 2 doesn’t form a hydrogen interaction with Asn1064, which may limit its affinity with ATAD2. Hence, we designed a series of benzamide derivatives, expecting that the amide bond could initiate a hydrogen bond with Asn1064 to enhance the Affinity. Additionally, a small size hydrophobic group (usually methyl) occupying a hydrophobic site was observed next to the hydrogen bond receptor, which would heighten the binding of ATAD2 and Ligand. Methyl or methoxyl was incorporated into the 4-site of the benzene ring to mimic the “methyl” of the substrate acetyl group. Collectively, we designed a series of theophylline derivatives as novel ATAD2 inhibitors through fragment-based screening and scaffold growth strategy.
Chemistry
The synthesis of compounds 15a–k and 19a–l derivatives was carried out (Schemes 1–2). Intermediate 9 was prepared by a substitution reaction of 7 with ethyl bromoacetate (2), and intermediate 9 was hydrolysed to give intermediate 10. The reaction of intermediate 11 with secondary amine derivatives (12) yielded intermediate 13 and further reduced to obtain intermediate 14. Compounds 15 were prepared through an amide condensation of intermediate 10 and 14. Additionally, Compounds 19a–l were synthesised by a similar route as compounds 15.
Analysis of the structure-activity relationship
To obtain novel potent ATAD2 inhibitors by optimising from theophylline based on scaffold growth strategy, according to the docking results of theophylline-ATAD2, the thiomorpholine group was incorporated into the middle benzene ring via an amine bond to yield 15a without ATAD2 inhibition activity (). Furthermore, we induced methyl and methoxy to the 4-site of the middle benzene ring (15b, 15c), displaying weak inhibitory activities against ATAD2. We hypothesised that hydrogen bond receptors could enhance the affinity of ATAD2, so the thiomorpholine group was substituted with the thiomorpholine 1,1-dioxide group (15d–15f), resulting in a complete loss of activity against ATAD2. Next, the replacement of the S atom with an oxygen atom yielded 15 g, displaying a weak inhibition potency. Different substituents were induced into the AROMATIC ring to yield 15h–15k, the 4-site methoxyl substituted (15i) presented the most potent activity against ATAD2 with an IC50 value of 11.32 μM. On the whole, the round of structural optimisation is not successful. We rechecked the binding configuration of ligand and ATAD2 and speculated that the secondary amide failed to form the active configuration due to its rigidity. Next, the secondary amide was replaced by the primary amide to improve the flexibility of the ligand. First, the cyclopropylamine substituted (19a–19c) display significant improvement in ATAD2 inhibitory activity, a similar potency (IC50 ≈ 9 μM) was observed between 19b and 19c (). Encouraged by the results, cyclopentyl, a bigger group, was induced to yield 19d with an improved IC50 value of 5.13 μM. The methyl (19e) exhibited about a three-fold increase in activity compared to 19d. The methoxy (19f) presented the most potent activity against ATAD2 with an IC50 value of 0.27 μM. Further increasing the size of the substituent resulted in a significant decrease in activity (19 g–19i) or even complete loss (19j–19l). In vitro anti-proliferation assays, these compounds only presented a low micromole antiproliferatory potency in BT-549 cells. The most potent compound 19f displayed an IC50 value of 5.43 μM against BT-549 cells for 24 h. Although the antiproliferative activity of 19f is about one-fold weaker than that of the positive drug (BAY-850), it is the first compound with the particular binding mode inhibitor to show moderate inhibitory activity, which still has positive implications for the design of novel ATAD2 inhibitors.
Table 1. The ATAD2 inhibitory activity of compounds 15a–k.
Table 2. the ATAD2 inhibitory activity of compounds 19a–l.
Molecular docking and molecular dynamic simulation
To explore the binding mode of compound 19f () and ATAD2, molecular docking was performed (). The results show that compound 19f could be combined with ATAD2 active site with an interaction energy value of −40.44 kcal/mol and the theophylline group initiates two key hydrogen interactions with the conserved residue Arg1007 from the ZA loop of ATAD2. Additional three hydrogen bonds were observed between the amide group of the linker and residues Val1008, Lys1011, and Glu1017, and the benzene ring interacts with residues Phe1009 and Tyr1021 through two Pi-Pi interactions. Furthermore, the methoxy group at the 4-site occupies the hydrophobic pocket near the active centre (Asn1064), mimicking the “methyl” of the acetyl group from substrates. Surprisingly, an important hydrogen interaction is formed between the terminal amide group and residue Asn1064, which is rigidly conservative for the classic ATAD2 inhibitors. The cyclopentyl positions to the solvent-accessible area as the previously reported atypical binding mode of 2 and ATAD2. Furthermore, the root-mean-square deviation (RMSD) of ATAD2 atoms around 5 Å of compound 19f fluctuated between 0.5 and 2.1 in 100 ns MD simulation (), which suggested the bindings of compound 19f and ATAD2 presented fairly stable active profiles. In addition, the binding free energy of the system revealed that compound 19f showed the strongest binding affinity against ATAD2, which further confirmed the strong enzyme inhibition activity (). Subsequently, the residues energy decomposition indicated that residues Lys1011, Nal1008, Phe1009, Val1013, Asn1064, and Ile1074 made a great contribution to the binding of compound 19f and ATAD2 (). In addition, compound 19f showed a weaker ATAD2 inhibitory activity compared with BAY-850, but a stronger ATAD2 inhibitory activity than AM879, which was previously found by us (). These results demonstrated that compound 19f is a potent ATAD2 inhibitor adopting a combination of classic and atypical binding mode. Intriguingly, compound 19f showed anti-proliferative activity comparable to BAY-850 and AM879 in BT-549 cells (). In another TNBC cell line MDA-MB-231 cells, compound 19f also illustrated good anti-proliferative activity, but did not show obvious cytotoxicity to normal breast MCF-10A cells (Figure S1). Taken together, compound 19f might be a promising candidate for TNBC treatment.
Figure 3. The docking of compound 19f and ATAD2 bromodomain. (A) The structure of compound 19f; (B) The hydrogen interactions of compound 19f and ATAD2 bromodomain in the cartoon; (C) The detailed interactions between compound 19f and ATAD2 bromodomain; (D) The RMSD of ATAD2 atoms around 5 Å of compound 19f; (E) the residues energy decomposition of compound 19f and ATAD2; (F) The effect of AM879, BAY-850 and compound 19f on ATAD2 activity detected by TR-FRET technology; (G) The effect of AM879, BAY-850, and compound 19f on the cell viability of BT-549 cells.
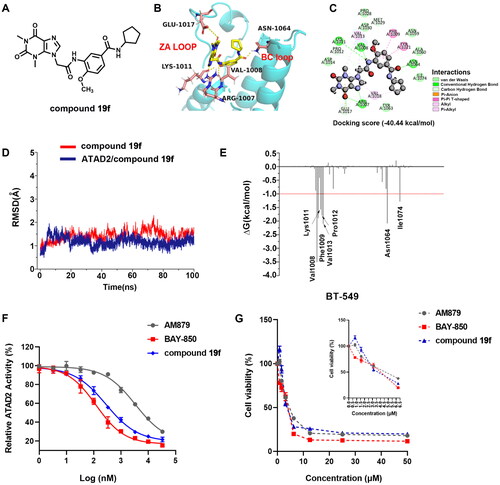
Table 3. The binding free energies were calculated by MM-GBSA method (kcal/mol).
Compound 19f inhibits ATAD2-Myc activation in BT-549 cells
Next, we investigated the effect of 19f on the biological function of ATAD2 in BT-549 cells. Considering that ATAD2 plays a tumour-promoting role mainly through activation of c-Myc (lack of sex hormone in TNBC)Citation3, we first detected the phosphorylation level of c-Myc at Ser62 in BT-549 cells after compound 19f treatment. Of note, MYC is a well-known proto-oncogene involved in the development and progression of many cancersCitation17,Citation18, including breast cancerCitation19. The phosphorylation of c-Myc at ser62 can promote the stabilisation of c-Myc and enhance the MYC-mediated cancer-promoting pathwayCitation18,Citation20. ATAD2 is a cofactor of MYC that binds to MYC and stimulates its transcriptional activityCitation21,Citation22. By examining the expression and phosphorylation of c-Myc, it was possible to determine whether the ATAD2 function was affected by compound 19f. As shown in , the immunofluorescence results showed that compound 19f could reduce the fluorescence intensity of p-c-MycSer62 in a dose-dependent manner (). Compared to the ATAD2 inhibitor BAY-850, AM879 we previously discovered, 19f has a more significant impact on p-c-MycSer62 level than AM879, and a similar result to the BAY-850 (). Subsequently, immunoblotting was used to detect the expression levels of ATAD2, c-Myc, and p-c-Myc in BT-549 cells treated with BAY-850, AM879, and compound 19f, respectively. The results demonstrated that AM879 and compound 19f both have no significant effect on the expression of ATAD2, but inhibited the expression levels of c-Myc and p-c-MycSer62 in a dose-dependent manner, and compound 19f had a more significant effect (). Interestingly, BAY-850 inhibited the expression of ATAD2, which is consistent with previous reports in other literature that BAY-850 reduced ATAD2 expressionCitation23. Similarly, BAY-850 also reduced c-Myc expression and ser62 the phosphorylation at ser62 in a dose-dependent manner (). Taken together, compound 19f impeded ATAD2 activity and c-Myc activation in TNBC cells.
Figure 4. compound 19f inhibits ATAD2-Myc activation in TNBC cells. (A) BT-549 cells were treated with 5, 10, 20 μM compound 19f for 48h, and the expression level of p-c-MycSer62 was detected by immunofluorescence. The nuclei were labelled with DAPI. Scale bar = 100 μm. (B) Relative p-c-MycSer62 intensity was quantified with Image J, and the mean intensity value of the control group was 100%, ****p < 0.0001. (C) BT-549 cells were treated with 10 μM AM879, BAY-850 or compound 19f for 48h, the expression level of p-c-Myc Ser62 was detected by immunofluorescence. The nuclei were labelled with DAPI. Scale bar = 100 μm. (D) Relative p-c-MycSer62 intensity was quantified with Image J, and the mean intensity value of the control group was 100%. ****, p < 0.0001, compared with the control group. ##, p < 0.01, compared with the AM879 treated group. (E,F) BT-549 cells were treated with 5, 10, 20 μM AM879, BAY-850, or compound 19f for 48h, the expression level of ATAD2, c-Myc, and p-c-MycSer62 were detected by western blot. ns, no significance. ***, p < 0.001; ****, p < 0.0001, compared with the control group.
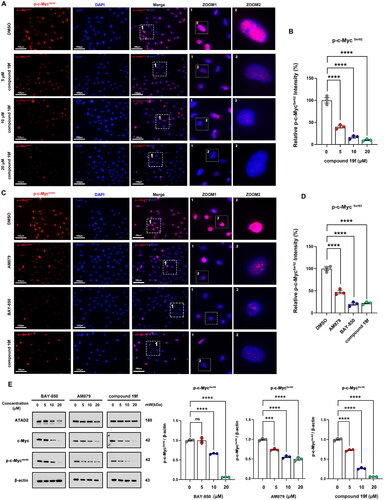
Compound 19f inhibits the proliferation of BT-549 cells
Next, we continue to examine the effect of compound 19f on the proliferation ability of BT-549 cells. As shown in , compound 19f can reduce the fluorescence intensity of EdU-488 in a dose-dependent manner, indicating that compound 19f can inhibit the synthesis of new DNA in BT-549 cells in a dose-dependent way, proving that compound 19f can inhibit TNBC cell proliferation (). Next, we detected the effect of compound 19f on the long-term cell proliferation ability of BT-549 cells. Compared with the control group, compound 19f can inhibit the colony formation ability of BT-549 cells in a concentration-dependent manner (), suggesting that compound 19f can also inhibit the long-term proliferation of BT-549 cells. In addition, we used 3D cell culture to investigate the effect of compound 19f on the formation of cell spheres. It is worth noting that 3D cell culture technology can better simulate the in vivo state of the tumorCitation24. The results indicated that compound 19f dose-dependently reduce the diameter of the 3D tumour cell spheres (). Subsequently, we compared the effects of BAY-850, AM879, and compound 19f on the proliferative ability of BT-549 cells. The results demonstrated that compound 19f showed similar anti-proliferation ability to BAY-850, slightly better than AM879 (). Collectively, compound 19f presents good anti-proliferative activity in BT-549 cells.
Figure 5. compound 19f inhibits the proliferation of TNBC cells. (A) BT-549 cells were treated with 5, 10, and 20 μM compound 19f for 48h, the newly synthesised DNA was detected using EdU-488, the nuclei were labelled with Hoechst 33258. Scale bar = 200 μm. (B) Relative EdU-488 intensity was quantified with Image J, and the mean intensity value of the control group was 100%. ****, p < 0.0001. (C) Colony formation assays were used to detect the Long-term cell proliferation ability after compound 19f treatment. Relative colony formation ability was quantified with Image J, and the mean value of the control group was 100%. ****p < 0.0001, compared with the control group. (D) 3D spherical plates were used to investigate the effect of compounds on the formation of cell spheres. Relative 3D cell spheres diameter was quantified with Image J, and the mean value of the control group was 100%. ****p < 0.0001, compared with the control group.
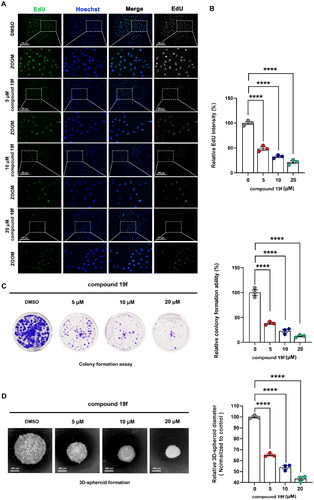
Figure 6. Comparison of the antiproliferative ability of compound 19f, BAY-850 and AM879 on BT-549 cells. (A) BT-549 cells were treated with 10 μM compound 19f, BAY-850 and AM879, the newly synthesised DNA was detected using EdU-488, and the nuclei were labelled with Hoechst 33258. Scale bar = 200 μm. (B) Relative EdU-488 intensity was quantified with Image J, and the mean intensity value of the control group was 100%. ****p < 0.0001, compared with the control group. (C) Colony formation assay were used to detect the long-term cell proliferation ability after compound 19f, BAY-850 and AM879 treatment. Relative colony formation ability was quantified with Image J, and the mean value of the control group was 100%. ****p < 0.0001, compared with the control group. (D) 3D spherical plates were used to investigate the effect of compounds on the formation of cell spheres. Relative 3D cell spheres diameter was quantified with Image J, and the mean value of the control group was 100%. ****p < 0.0001, compared with the control group.
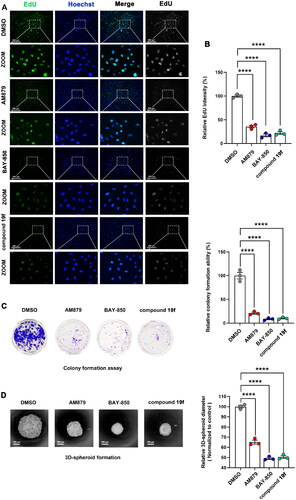
2.7. Compound 19f induces apoptosis in BT-549 cells
Due to the close relationship between ATAD2 and apoptosis, inhibition of ATAD2 can induce apoptosisCitation8. Therefore, we next tested whether compound 19f can induce apoptosis in BT-549 cells. Interestingly, compound 19f could induce obvious apoptosis in a dose-dependent manner, with an increase in early apoptosis and late apoptosis ratio (). Then, we investigated the expression of classic apoptotic marker protein. The results revealed that compound 19f promoted the expression of Bax, the cleavage of caspase-3, caspase-9 and PARP. Meanwhile, the expression of Bcl-2 was significantly decreased after compound 19f treatment. Intriguingly, the cleavage of caspase-8 was not changed significantly, indicating that death receptor-mediated apoptosisCitation25 may be not involved in the regulation of BT-549 cell apoptosis by compound 19f (). Thus, the inhibition of ATAD2 by compound 19f induces apoptosis in BT-549 cells.
Figure 7. Compound 19f induces apoptosis in BT-549 cells. (A) BT-549 cells were treated with 5, 10, 20 μM compound 19f for 48h, the apoptosis ratio was measured by Annexin V-PI staining. (B) Relative apoptosis ratio was quantified with GraphPad Prism 8. *p < 0.05; **p < 0.01; ****, p < 0.0001, ns, no significance, compared with the control group. (C-D) BT-549 cells were treated with 5, 10, 20 μM compound 19f for 48h, the expression level of Bax, Bcl-2, Caspase-3, Caspase-8, Caspase-9, and PARP were detected by western blot. ns, no significance; *p < 0.05; **p < 0.01; ***p < 0.001; ****p < 0.0001, compared with the control group.
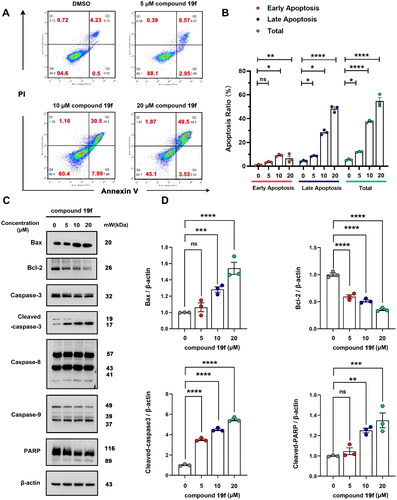
Compound 19f inhibits the migration of BT-549 cells
To further evaluate the potential application of compound 19f in TNBC, we subsequently examined its impact on the migration of TNBC cells. Not surprisingly, the migration ability of BT-549 cells was significantly decreased after compound 19f treatment. The wound healing assay revealed that compound 19f increased the relative wound area in a dose-dependent manner (). Meanwhile, compound 19f also decreased the migrated cell number in a dose-dependent manner (). Next, we checked the expression of MMP-2 and E-cadherin, which are two key migration markers, and found that compound 19f up-regulated the expression of E-cadherin and down-regulated MMP-2 (). Taken together, compound 19f exhibits good anti-migration activity in vitro.
Figure 8. Compound 19f inhibits BT-549 cells migration. (A) Wound healing assay of BT-549 cells treated with 2.5, 5, 10, 20 μM compound 19f for 48h. (B) Relative wound areas were quantified with Image J, and the mean value of the control group was 100%. *p < 0.05; **p < 0.01; ***p < 0.001; ****, p < 0.0001, compared with the control group. (C) Transwell assay of BT-549 cells treated with 5, 10, 20 μM compound 19f for 48h. (D) Relative migrated cell count was quantified with Image J, and the mean value of the control group was 100%. ****p < 0.0001, compared with the control group. (E–F) BT-549 cells were treated with 5, 10, 20 μM compound 19f for 48h, the expression level of MMP-2 and E-cadherin were detected by western blot. ****p < 0.0001, compared with the control group.
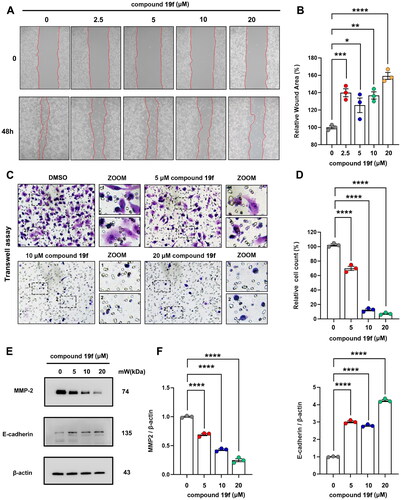
Conclusion
In summary, we have successfully designed and synthesised a series of theophylline derivatives as novel ATAD2 inhibitors through fragment-based screening and scaffold growth strategy. Compound 19f is identified as a potent ATAD2 inhibitor with an IC50 value of 0.27 μM, presenting a significant elevation of 87 folds in affinity for ATAD2 bromodomain compared to the lead theophylline. Additionally, compound 19f may adopt a combination of the classic and atypical binding mode for ATAD2. Compound 19f significantly inhibited BT-549 cells proliferation with an IC50 value of 5.43 μM, although the antiproliferative activity of compound 19f is slightly weaker than that of the positive drug (BAY-850), it is the first compound with the particular binding mode inhibitor to show cellular antiproliferative potency. In addition, compound 19f could impede ATAD2 activity and c-Myc activation, and induce significant apoptosis, and anti-migration in BT-549 cells. Collectively, compound 19f is a promising candidate lead for the development of novel potent ATAD2 inhibitors.
Experimental
Chemistry
All commercially purchased reagents/solvents are used directly without any further processing. The NMR spectra were detected by AVANCE NEO 600 (Bruker Switzerland AG). ESI-MS spectra were collected by TSQ Altis™ Plus. The purities (HPLC) of all compounds were more than 95%. HPLC device: Agilent 1260, Column: Phenomenex Luna 5 μm C18 (2) 100 A, 250 × 4.6 mm S/N: H19-293790. The mobile phase is different concentrations of methanol in water with a flow rate of 1.0 ml/min.
Synthesis
Synthesis of intermediate 9
To a solution of theophylline (7, 10 mmol) in DMF (15 ml), K2CO3 (30 mmol), and the resulting mixture was allowed to warm up to 60 °C for 30 min, and ethyl bromoacetate (8, 12 mmol) were added. Upon completion the reaction, the residue was added 50 ml water, and extracted with ethyl acetate (3 × 50 ml). The combined organic layers were washed with saturated aqueous sodium bicarbonate and brine and then dried over anhydrous sodium sulphate. After removing the solvent under reduced pressure to give the colourless oil. The crude oil was purified by silica sel flash chromatography (dichloromethane/methanol 50:1) as a colourless oil, yield 89%. Ethyl 2–(1,3-dimethyl-2,6-dioxo-1,2,3,6-tetrahydro-9H-purin-9-yl) acetate (9) 1H-NMR (600 MHz, DMSO-d6), δ(ppm): 8.06 (1H, s), 5.17 (2H, s), 4.17 (2H, q, J = 7.1 Hz), 3.44 (3H, s), 3.20 (3H, s), 1.22 (3H, t, J = 7.1 Hz); 13C-NMR (150 MHz, DMSO-d6), δ(ppm): 168.1, 154.8, 151.4, 148.4, 143.6, 106.7, 61.8, 47.6, 29.9, 27.9, 14.4.
Synthesis of intermediate 10
To a solution of 9 (10 mmol) in 75% MeOH (15 ml), LiOH (30 mmol), and the resulting mixture was stirred for 8 h. Upon completion, the residue was evaporated to remove excess MeOH and acidified with 1 M HCl to pH = 3. The resulting precipitate was collected by filtration to yield the intermediate 10 as a white solid and used directly for the next reaction without purification, yield 89%. 2–(1,3-dimethyl-2,6-dioxo-1,2,3,6-tetrahydro-9H-purin-9-yl)acetic acid (9) 1H-NMR (600 MHz, DMSO-d6), δ(ppm): 13.29 (1H, br. s), 8.04 (1H, br. s), 5.08 (2H, s), 3.44 (3H, s), 3.20 (3H, s); 13C-NMR (150 MHz, DMSO-d6), δ(ppm): 169.4, 154.8, 151.4, 148.3, 143.6, 106.8, 47.6, 29.9, 27.9.
General procedure synthesis of intermediates 13
To a solution of intermediate 11 (5 mmol), secondary amine derivatives (12, 5 mmol) and DIEA (10 mmol) in DMF (10 ml), and HATU was added at room temperature for 24 h. The mixture was added water (50 ml), extracted with ethyl acetate (30 ml) three times. The combined organic layers were washed with water, saturated aqueous sodium bicarbonate and brine, and then dried over anhydrous sodium sulphate. After removing the solvent under reduced pressure, the crude product was purified by flash chromatography on silica gel, eluting with dichloromethane/methanol (1–2%), yield 83–95%.
(3-nitrophenyl)(thiomorpholino)methanone (13a) light yellow solid, yield 83%
1H-NMR (600 MHz, CDCl3), δ (ppm): 8.30 (1H, d, J = 8.1 Hz), 8.25 (1H, m), 7.73 (1H, dt, J = 7.6, 1.3 Hz), 7.64 (1H, t, J = 7.6 Hz), 4.05 (2H, br. s), 3.68 (2H, br. s), 2.76 (2H, br. s), 2.61 (2H, br. s); 13C-NMR (100 MHz, CDCl3), δ (ppm): 168.0, 148.1, 137.3, 132.8, 129.9, 124.5, 122.0, 50.1, 44.8, 27.8.
(4-methyl-3-nitrophenyl)(thiomorpholino)methanone (13b) light yellow solid, yield 89%
1H-NMR (600 MHz, CDCl3), δ (ppm): 8.01 (1H, d, J = 1.5 Hz), 7.54 (1H, dd, J = 7.8, 1.5 Hz), 7.42 (1H, d, J = 7.8 Hz), 4.02 (2H, br. s), 3.71 (2H, br. s), 2.69 (4H, br. s), 2.64 (3H, s); 13C-NMR (150 MHz, CDCl3), δ (ppm): 168.1, 149.0, 135.3, 134.7, 133.3, 131.2, 123.3, 50.2, 44.7, 27.7, 20.3.
(4-methoxy-3-nitrophenyl)(thiomorpholino)methanone (13c) light yellow solid, yield 92%
1H-NMR (600 MHz, CDCl3), δ (ppm): 7.93 (1H, d, J = 2.1 Hz), 7.65 (1H, dd, J = 8.6, 2.1 Hz), 7.16 (1H, d, J = 8.6 Hz), 4.01 (3H, s), 3.91 (4H, br. s), 2.68 (4H, br. s); 13C-NMR (150 MHz, CDCl3), δ (ppm): 168.1, 154.0,139.1, 133.2, 127.6, 124.9, 113.7, 56.8, 50.3, 45.1, 27.6.
(1,1-dioxidothiomorpholino)(3-nitrophenyl)methanone (13d) light yellow solid, yield 87%
1H-NMR (600 MHz, CDCl3), δ (ppm): 8.41 (1H, br. s), 8.32 (1H, dd, J = 8.2, 1.4 Hz), 7.93 (1H, d, J = 7.7 Hz), 7.77 (1H, d, J = 8.0 Hz), 4.03 (4H, br. s), 3.67 (4H, br. s), 3.26 (4H, br. s); 13C-NMR (150 MHz, CDCl3), δ (ppm): 167.7, 148.2, 137.2, 133.6, 130.7, 124.9, 122.4, 51.0, 46.0.
(1,1-dioxidothiomorpholino)(4-methyl-3-nitrophenyl)methanone (13e) light yellow solid, yield 94%
1H-NMR (600 MHz, CDCl3), δ (ppm): 8.01 (1H, d, J = 1.5 Hz), 7.54 (1H, dd, J = 7.8, 1.5 Hz), 7.42 (1H, d, J = 7.8 Hz), 3.86 (4H, br. s), 3.26 (4H, br. s), 2.30 (3H, s); 13C-NMR (150 MHz, CDCl3), δ (ppm): 167.8, 153.3, 139.2, 133.6, 127.5, 124.6, 114.9, 57.4, 51.1, 20.3.
(1,1-dioxidothiomorpholino)(4-methoxy-3-nitrophenyl)methanone (13f) light yellow solid, yield 86%
1H-NMR (600 MHz, CDCl3), δ (ppm): 8.07 (1H, d, J = 2.1 Hz), 7.79 (1H, dd, J = 8.6, 2.1 Hz), 7.45 (1H, d, J = 8.6 Hz), 3.97 (3H, s), 3.86 (4H, br. s), 3.26 (4H, br. s); 13C-NMR (150 MHz, CDCl3), δ (ppm): 167.8, 153.3, 139.2, 133.6, 127.5, 124.6, 114.9, 57.4, 51.1.
Morpholino(3-nitrophenyl)methanone (13 g) light yellow solid, yield 89%
1H-NMR (600 MHz, CDCl3), δ (ppm): 7.90 (1H, d, J = 1.9 Hz), 7.82 (1H, d, J = 8.2 Hz), 7.49 (1H, dd, J = 8.2, 1.9 Hz), 3.74 (6H, br. s), 3.48 (2H, br. s); 13C-NMR (150 MHz, CDCl3), δ (ppm): 166.7, 149.7, 135.7, 135.5, 131.6, 124.5, 116.2, 66.6, 48.2, 42.7.
(4-methyl-3-nitrophenyl)(morpholino)methanone (13h) light yellow solid, yield 86%
1H-NMR (600 MHz, CDCl3), δ (ppm): 8.04 (1H, d, J = 1.5 Hz), 7.58 (1H, dd, J = 7.8, 1.5 Hz), 7.43 (1H, d, J = 7.8 Hz), 3.74 (6H, br. s), 3.50 (2H, br. s), 2.65 (3H, s); 13C-NMR (150 MHz, CDCl3), δ (ppm): 167.8, 149.0, 135.4, 134.3, 133.2, 131.5, 123.6, 66.7, 48.2, 42.7, 20.3.
(4-methoxy-3-nitrophenyl)(morpholino)methanone (13i) light yellow solid, yield 86%
1H-NMR (600 MHz, CDCl3), δ (ppm): 7.96 (1H, d, J = 1.9 Hz), 7.68 (1H, dd, J = 8.6, 1.9 Hz), 7.16 (1H, d, J = 8.6 Hz), 4.02 (3H, s), 3.73 (6H, br. s), 3.66 (2H, br. s); 13C-NMR (150 MHz, CDCl3), δ (ppm): 167.7, 154.0, 139.1, 133.5, 127.2, 125.1, 113.6, 66.7, 56.8, 48.2, 42.7.
(4-chloro-3-nitrophenyl)(morpholino)methanone (13j) light yellow solid, yield 78%
1H-NMR (600 MHz, CDCl3), δ (ppm): 7.95 (1H, s), 7.64 (1H, d, J = 8.3 Hz), 7.58 (1H, d, J = 8.3 Hz), 3.77 (2H, br. s), 3.69 (2H, br. s), 3.46 (2H, br. s); 13C-NMR (150 MHz, CDCl3), δ (ppm): 166.7, 147.8, 135.0, 132.3, 131.8, 128.7, 124.6, 66.7, 48.2, 42.7.
(2-fluoro-3-nitrophenyl)(morpholino)methanone (13k) light yellow solid, yield 90%
1H-NMR (600 MHz, CDCl3), δ (ppm): 8.12 (1H, t, J = 7.6 Hz), 7.70 (1H, t, J = 6.7 Hz), 7.40 (1H, t, J = 7.9 Hz), 3.83 (2H, br. s), 3.80 (2H, br. s), 3.68 (2H, br. s), 3.35 (2H, br. s); 13C-NMR (150 MHz, CDCl3), δ (ppm): 162.7, 137.5, 134.6, 134.6, 127.2, 127.2, 126.9, 126.7, 125.2, 125.1, 66.6, 47.4, 42.6.
Synthesis of compounds 14
To a solution of intermediate 13 (3 mmol), iron powder (12 mmol) and ammonium chloride (NH4Cl, 15 mmol) in 95% ethanol (20 ml), and a catalytic amount of glacial acetic acid were added under strenuous stirring, and then the mixture was allowed to warm up to reflux for 4 h. The additional iron powder was removed by filtration, and the filtrate was concentrated to dryness to give the crude product and used directly for the next reaction without purification, yield 87–97%.
(3-aminophenyl)(thiomorpholino)methanone (14a) light brown solid, yield 88%
1H-NMR (600 MHz, CDCl3), δ(ppm): 7.17 (1H, t, J = 7.7 Hz), 6.71 (1H, t, J = 7.2 Hz), 6.67 (2H, s), 3.99 (2H, brs), 3.84 (2H, brs), 3.67 (2H, brs), 2.72 (2H, brs), 2.55 (2H, brs); 13C-NMR (150 MHz, CDCl3), δ(ppm): 170.9, 146.7, 136.9, 129.5, 116.4, 116.2, 113.1, 50.0, 44.4, 28.1, 27.4.
(3-amino-4-methylphenyl)(thiomorpholino)methanone (14b) light brown solid, yield 94%
1H-NMR (600 MHz, CDCl3), δ(ppm): 7.05 (1H, d, J = 7.5 Hz), 6.68 (1H, brs Hz), 6.65 (1H, d, J = 7.5 Hz), 3.96 (2H, brs), 3.84 (2H, brs), 3.70 (2H, brs), 2.66 (4H, brs); 13C-NMR (150 MHz, CDCl3), δ(ppm): 171.0, 144.8, 134.5, 130.4, 123.9, 116.6, 113.1, 50.1, 44.5, 29.6, 27.7, 17.2.
(3-amino-4-methoxyphenyl)(thiomorpholino)methanone (14c) light brown solid, yield 91%
1H-NMR (600 MHz, CDCl3), δ(ppm): 6.77 − 6.73 (3H, m), 3.89 (2H, br. s), 3.88 (4H, br. s), 3.87 (3H, s), 2.64 (4H, br. s); 13C-NMR (150 MHz, CDCl3), δ(ppm): 171.1, 148.3, 136.3, 128.3, 117.3, 113.5, 109.8, 55.5, 49.8, 44.9, 27.8, 27.8.
(3-aminophenyl)(1,1-dioxidothiomorpholino)methanone (14d) light brown solid, yield 96%
1H-NMR (600 MHz, CDCl3), δ(ppm): 7.22 (1H, t, J = 7.8 Hz), 6.73 (1H, s), 6.78 (1H, d, J = 7.8 Hz), 6.75 (1H, d, J = 7.5 Hz), 6.72 (1H, br. s), 4.10 (4H, br. s), 3.06 (4H, br. s); 13C-NMR (150 MHz, CDCl3), δ(ppm): 171.2, 147.0, 135.0, 129.8, 117.0, 116.3, 113.0, 52.1, 45.8, 29.7.
(3-amino-4-methylphenyl)(1,1-dioxidothiomorpholino)methanone (14e) light brown solid, yield 85%
1H-NMR (600 MHz, CDCl3), δ(ppm): 7.10 (1H, d, J = 7.6 Hz), 6.73 (1H, s), 6.71 (1H, d, J = 7.6 Hz), 4.9 (4H, br. s), 3.06 (4H, br. s), 2.19 (3H, s); 13C-NMR (150 MHz, CDCl3), δ(ppm): 171.4, 145.2, 132.6, 130.7, 124.9, 116.5, 113.0, 52.0, 45.9, 41.1, 17.3.
(3-amino-4-methoxyphenyl)(1,1-dioxidothiomorpholino)methanone (14f) light brown solid, yield 81%
1H-NMR (600 MHz, CDCl3), δ(ppm): 6.80 (3H, s), 4.10 (4H, br. s), 3.90 (3H, s), 3.06 (4H, br. s); 13C-NMR (150 MHz, CDCl3), δ(ppm): 171.5, 149.0, 136.6, 126.3, 117.5, 113.4, 109.8, 55.6, 52.0.
(3-amino-4-methoxyphenyl)(morpholino)methanone (14 g) light brown solid, yield 80%
1H-NMR (400 MHz, CDCl3), δ(ppm): 7.15 (1H, t, J = 7.7 Hz), 6.72 − 6.70 (3H, m), 3.75 (4H, br. s), 3.60 (2H, br. s), 3.47 (4H, m); 13C-NMR (100 MHz, CDCl3), δ(ppm): 170.6, 146.6, 136.3, 129.4, 116.7, 116.4, 113.5, 66.9, 48.1, 42.4.
(3-amino-4-methylphenyl)(morpholino)methanone (14h) light brown solid, yield 96%
1H-NMR (600 MHz, CDCl3), δ(ppm): 7.05 (1H, d, J = 7.5 Hz), 6.71 (1H, d, J = 1.4 Hz), 6.68 (1H, dd, J = 7.5, 1.4 Hz), 3.71 − 3.50 (10H, m), 2.17 (3H, s); 13C-NMR (150 MHz, CDCl3), δ (ppm): 1170.7, 144.8, 134.1, 130.4, 124.1, 116.9, 113.4, 66.9, 48.2, 42.5, 17.2.
(3-amino-4-methoxyphenyl)(morpholino)methanone (14i) light brown solid, yield 94%
1H-NMR (600 MHz, CDCl3), δ (ppm): 6.78 (1H, d, J = 8.1 Hz), 6.77 (2H, br. s), 3.89 (2H, br. s), 3.87 (3H, s), 3.67 (8H, m); 13C-NMR (150 MHz, CDCl3), δ (ppm): 170.7, 148.4, 136.3, 127.9, 117.6, 113.8, 109.7, 66.9, 55.5.
(3-amino-4-chlorophenyl)(morpholino)methanone (14j) light brown solid, yield 92%
1H-NMR (600 MHz, CDCl3), δ(ppm): 7.26 (1H, d, J = 8.0 Hz), 6.80 (1H, d, J = 1.8 Hz), 6.67 (1H, dd, J = 8.0, 1.8 Hz), 4.23 (2H, br. s), 3.72 − 3.45 (8H, m); 13C-NMR (150 MHz, CDCl3), δ (ppm): 169.7, 143.3, 134.8, 129.4, 120.5, 117.0, 114.3, 66.8, 48.1, 42.5.
(3-amino-2-fluorophenyl)(morpholino)methanone (14k) light brown solid, yield 97%
1H-NMR (600 MHz, CDCl3), δ(ppm): 6.97 (1H, t, J = 7.8 Hz), 6.81 (1H, t, J = 8.3 Hz), 6.68 (1H, t, J = 6.9 Hz), 3.83 (2H, br. s), 3.81 (2H, br. s), 3.77 (2H, d, J = 4.2 Hz), 3.64 (2H, br. s), 3.36 (2H, br. s); 13C-NMR (150 MHz, CDCl3), δ (ppm): 165.5, 147.7, 146.1, 134.8, 134.8, 125.0, 125.0, 123.7, 123.6, 117.8, 117.8, 117.3, 66.9, 66.8, 47.4, 42.3.
Synthesis of intermediate 15a–k
To a solution of intermediate 10 (1 mmol), intermediate 14 (1 mmol), and DIEA (2 mmol) in DMF (10 ml), and HATU (1 mmol) was added at room temperature for 24 h. The mixture was added water (50 ml), and extracted with ethyl acetate (30 ml) three times. The combined organic layers were washed with water, saturated aqueous sodium bicarbonate and brine, and then dried over anhydrous sodium sulphate. After removing the solvent under reduced pressure, the crude product was purified by flash chromatography on silica gel, eluting with dichloromethane/methanol (2–4%), yield 78–93%.
2–(1,3-dimethyl-2,6-dioxo-1,2,3,6-tetrahydro-9H-purin-9-yl)-N-(3-(thiomorpholine-4-carbonyl)phenyl)acetamide (15a)
White solid, m.p. 246–248 °C, yield 79%. 1H-NMR (600 MHz, DMSO-d6), δ (ppm): 10.58 (1H, s), 8.07 (1H, s), 7.67 (1H, s), 7.58 (1H, d, J = 8.1 Hz), 7.40 (1H, t, J = 7.8 Hz), 7.09 (1H, d, J = 7.8 Hz), 5.22 (2H, s), 3.85 (2H, br. s), 3.54 (2H, br. s), 3.46 (3H, s), 3.19 (3H, s), 2.66 (2H, br. s), 2.58 (2H, br. s); 13C-NMR (150 MHz, DMSO-d6), δ (ppm): 169.4, 165.6, 154.9, 151.4, 148.4, 144.2, 139.1, 137.1, 129.7, 122.1, 120.3, 117.6, 106.9, 50.0, 49.2, 44.3, 29.9, 27.9, 27.4, 27.0; HRMS (ESI)+ calculated for C20H22N6O4S, [M + H]+: m/z 443.1496, found 443.1504.
2–(1,3-dimethyl-2,6-dioxo-1,2,3,6-tetrahydro-9H-purin-9-yl)-N-(2-methyl-5-(thiomorpholine-4-carbonyl)phenyl)acetamide (15b)
White solid, m.p. 251–252 °C, yield 86%. 1H-NMR (600 MHz, DMSO-d6), δ (ppm): 9.82 (1H, s), 8.09 (1H, s), 8.08 (1H, s), 7.48 (1H, s), 7.30 (1H, d, J = 7.8 Hz), 7.12 (1H, d, J = 6.9 Hz), 5.25 (2H, s), 3.82 (2H, br. s), 3.56 (2H, br. s), 3.46 (3H, s), 3.22 (3H, s), 2.63 (2H, br. s), 2.56 (2H, br. s), 2.29 (3H, s); 13C-NMR (150 MHz, DMSO-d6), δ (ppm): 169.4, 165.9, 154.9, 151.5, 148.4, 144.1, 136.0, 134.1, 133.2, 131.0, 124.0, 123.3, 107.0, 49.1, 29.9, 27.9, 27.0, 18.1; HRMS (ESI)+ calculated for C21H24N6O4S, [2M + H]+: m/z 913.3244, found [2M + H]+ 913.3248.
2–(1,3-dimethyl-2,6-dioxo-1,2,3,6-tetrahydro-9H-purin-9-yl)-N-(2-methoxy-5-(thiomorpholine-4-carbonyl)phenyl)acetamide (15c)
White solid, m.p. 257–258 °C, yield 90%. 1H-NMR (600 MHz, DMSO-d6), δ (ppm): 9.88 (1H, s), 8.07 (1H, s), 8.08 (1H, s), 7.17 − 7.12 (2H, m), 5.29 (2H, s), 3.92 (3H, s), 3.69 (4H, br. s), 3.46 (3H, s), 3.20 (3H, s), 2.59 (4H, br. s); 13C-NMR (150 MHz, DMSO-d6), δ (ppm): 169.6, 166.0, 162.7, 154.9, 151.4, 150.5, 148.4, 144.2, 128.1, 127.0, 123.9, 120.5, 111.5, 106.9, 56.4, 55.3, 49.4, 29.9, 27.9; HRMS (ESI)+ calculated for C21H24N6O5S, [M + H]+: m/z 473.1602, found 473.1627.
2–(1,3-dimethyl-2,6-dioxo-1,2,3,6-tetrahydro-9H-purin-9-yl)-N-(3–(1,1-dioxidothiomorpholine-4-carbonyl)phenyl)acetamide (15d)
White solid, m.p. 224–226 °C, yield 95%. 1H-NMR (600 MHz, DMSO-d6), δ (ppm):10.59 (1H, s), 8.07 (1H, s), 7.75 (1H, s), 7.60 (1H, d, J = 7.6 Hz), 7.42 (1H, t, J = 7.9 Hz), 7.21 (1H, d, J = 7.5 Hz), 5.75 (1H, s), 5.22 (2H, s), 4.00 (2H, br. s), 3.70 (2H, br. s), 3.45 (3H, s), 3.25 (4H, br. s), 3.22 (4H, br. s), 3.20 (3H, s); 13C-NMR (150 MHz, DMSO-d6), δ (ppm): 169.4, 165.6, 154.9, 151.4, 148.4, 144.2, 139.1, 136.1, 129.6, 122.3, 120.7, 118.0, 106.9, 55.3, 51.2, 49.2, 29.9, 27.9; HRMS (ESI)+ calculated for C20H22N6O6S, [M + Na]+: m/z 497.1214, found 497.1202.
2–(1,3-dimethyl-2,6-dioxo-1,2,3,6-tetrahydro-9H-purin-9-yl)-N-(5–(1,1-dioxidothiomorpholine-4-carbonyl)-2-methylphenyl)acetamide (15e)
White solid, m.p. 231–233 °C, yield 92%. 1H-NMR (600 MHz, DMSO-d6), δ (ppm): 9.93 (1H, s), 8.09 (1H, s), 7.58 (1H, s), 7.32 (1H, d, J = 7.6 Hz), 7.22 (1H, d, J = 7.6 Hz), 5.26 (2H, s), 3.96 (2H, s), 3.70 (2H, br. s), 3.45 (3H, s), 3.22 (4H, br. s), 3.21 (3H, s), 2.30 (3H, s); 13C-NMR (150 MHz, DMSO-d6), δ (ppm): 169.5, 165.8, 154.9, 151.5, 148.4, 144.1, 136.1, 133.7, 133.1, 131.0, 124.2, 123.6, 107.0, 51.2, 49.0, 29.9, 27.9, 18.2; HRMS (ESI)+ calculated for C21H24N6O6S, [M-H]-: m/z 487.1405, found 487.1404.
2–(1,3-dimethyl-2,6-dioxo-1,2,3,6-tetrahydro-9H-purin-9-yl)-N-(5–(1,1-dioxidothiomorpholine-4-carbonyl)-2-methoxyphenyl)acetamide (15f)
White solid, 247–248 °C, yield 95%. 1H-NMR (600 MHz, DMSO-d6), δ (ppm): 9.89 (1H, s), 8.12 (1H, s), 8.07 (1H, s), 7.26 (1H, dd, J = 8.3, 1.5 Hz), 7.15 (1H, d, J = 8.3 Hz), 5.29 (2H, s), 3.94 (3H, s), 3.85 (4H, br. s), 3.46 (3H, s), 3.22 (4H, br. s), 3.20 (3H, s); 13C-NMR (150 MHz, DMSO-d6), δ (ppm): 169.6, 166.0, 154.9, 151.4, 150.8, 148.4, 144.2, 127.0, 124.2, 120.9, 111.4, 106.9, 56.4, 51.2, 49.4, 40.4, 29.9, 27.9; HRMS (ESI)- calculated for C21H24N6O7S, [M-H]-: m/z 503.1354, found 503.1355.
2–(1,3-dimethyl-2,6-dioxo-1,2,3,6-tetrahydro-9H-purin-9-yl)-N-(3-(morpholine-4-carbonyl)phenyl)acetamide (15 g)
White solid, m.p. 266–268 °C, yield 96%. 1H-NMR (600 MHz, DMSO-d6), δ (ppm): 10.58 (1H, s), 8.08 (1H, s), 7.67 (1H, s), 7.59 (1H, d, J = 8.2 Hz), 7.40 (1H, t, J = 7.8 Hz), 7.11 (1H, d, J = 7.6 Hz), 5.22 (2H, s), 3.61 (6H, br. s), 3.54 (2H, s), 3.45 (3H, s), 3.19 (3H, s); 13C-NMR (150 MHz, DMSO-d6), δ (ppm): 169.1, 165.6, 154.9, 151.4, 148.4, 144.2, 139.1, 136.6, 129.6, 122.4, 120.4, 118.0, 106.9, 66.5, 55.3, 49.2, 48.1, 42.5, 29.9, 27.9; HRMS (ESI)+ calculated for C20H22N6O5, [M + H]+: m/z 427.1724, found 427.1729.
2–(1,3-dimethyl-2,6-dioxo-1,2,3,6-tetrahydro-9H-purin-9-yl)-N-(2-methyl-5-(morpholine-4-carbonyl)phenyl)acetamide (15h)
White solid, m.p. 270–271 °C, yield 88%. 1H-NMR (600 MHz, DMSO-d6), δ (ppm): 9.83 (1H, s), 8.09 (1H, s), 8.08 (1H, s), 7.49 (1H, s), 7.30 (1H, d, J = 7.8 Hz), 7.14 (1H, d, J = 7.6 Hz), 5.25 (2H, s), 3.57 (8H, br. s), 3.45 (3H, s), 3.22 (3H, s), 2.29 (3H, s); 13C-NMR (100 MHz, CDCl3), δ (ppm): 169.0, 165.8, 154.9, 151.5, 148.4, 144.1, 136.0, 133.6, 133.4, 130.9, 124.4, 123.8, 107.0, 66.5, 49.0, 29.9, 27.9, 18.1; HRMS (ESI)+ calculated for C21H24N6O5, [M + H]+: m/z 441.1881, found 441.11885.
2–(1,3-dimethyl-2,6-dioxo-1,2,3,6-tetrahydro-9H-purin-9-yl)-N-(2-methoxy-5-(morpholine-4-carbonyl)phenyl)acetamide (15i)
White solid, m.p. 278–279 °C, yield 94%. 1H-NMR (600 MHz, DMSO-d6), δ (ppm): 9.87 (1H, s), 8.07 (1H, d, J = 8.8 Hz), 7.19 (1H, d, J = 8.7 Hz), 7.13 (1H, d, J = 8.7 Hz), 5.29 (2H, s), 3.93 (3H, s), 3.55 (4H, br. s), 3.46 (4H, br. s), 3.45 (3H, s), 3.21 (3H, s); 13C-NMR (100 MHz, CDCl3), δ (ppm): 169.2, 166.0, 154.9, 151.5, 150.6, 148.4, 144.2, 127.6, 127.0, 124.3, 120.9, 111.4, 106.9, 66.5, 56.4, 49.4, 29.9, 27.9; HRMS (ESI)- calculated for C21H24N6O6, [M-H]-: m/z 455.1685, found 455.1674.
N-(2-chloro-5-(morpholine-4-carbonyl)phenyl)-2–(1,3-dimethyl-2,6-dioxo-1,2,3,6-tetrahydro-9H-purin-9-yl)acetamide (15j)
White solid, m.p. 262–264 °C, yield 91%. 1H-NMR (600 MHz, DMSO-d6), δ (ppm): 10.16 (1H, s), 8.09 (1H, s), 7.81 (1H, d, J = 1.3 Hz), 7.60 (1H, d, J = 8.2 Hz), 7.24 (1H, dd, J = 8.2, 1.3 Hz), 5.31 (2H, s), 3.59 (6H, br. s), 3.52 (2H, s), 3.45 (3H, s), 3.21 (3H, s); 13C-NMR (100 MHz, CDCl3), δ (ppm): 169.8, 166.3, 154.9, 151.4, 148.3, 144.2, 138.8, 138.6, 131.1, 131.0, 128.7, 128.5, 128.0, 127.5, 106.9, 57.0, 48.5, 40.4, 34.3, 29.9, 27.9; HRMS (ESI)- calculated for C20H21ClN6O5, [M-H]-: m/z 459.1189, found 459.1181.
2–(1,3-dimethyl-2,6-dioxo-1,2,3,6-tetrahydro-9H-purin-9-yl)-N-(2-fluoro-3-(morpholine-4-carbonyl)phenyl)acetamide (15k)
White solid, m.p. 265–267 °C, yield 93%. 1H-NMR (600 MHz, DMSO-d6), δ (ppm): 10.39 (1H, s), 8.08 (1H, s), 7.98 (1H, t, J = 7.8 Hz), 7.28 (1H, d, J = 6.5 Hz), 7.24 (1H, t, J = 7.9 Hz), 7.15 (1H, t, J = 6.2 Hz), 5.29 (2H, s), 3.66 (6H, br. s), 3.53 (2H, s), 3.46 (3H, s), 3.20 (3H, s); 13C-NMR (100 MHz, CDCl3), δ (ppm): 169.0, 166.3, 154.8, 151.4, 148.3, 144.1, 136.9, 129.2, 128.7, 128.4, 106.8, 53.8, 51.5, 48.3, 44.0, 41.2, 29.9, 27.9; HRMS (ESI)+ calculated for C20H21FN6O5, [M + H]+: m/z 445.1630, found 445.1626.
General procedure synthesis of intermediates 17
To a solution of intermediate 11 (5 mmol), amine derivatives (16, 5 mmol) and DIEA (10 mmol) in DMF (10 ml), and HATU was added at room temperature for 24 h. The mixture was added water (50 ml), extracted with ethyl acetate (30 ml) three times. The combined organic layers were washed with water, saturated aqueous sodium bicarbonate and brine, and then dried over anhydrous sodium sulphate. After removing the solvent under reduced pressure, the crude product was purified by flash chromatography on silica gel, eluting with dichloromethane/methanol (1–2%), yield 79–96%.
N-cyclopropyl-3-nitrobenzamide (17a) light yellow solid, yield 79%
1H-NMR (600 MHz, CDCl3), δ (ppm): 8.56 (1H, s), 8.33 (1H, d, J = 8.1 Hz), 8.16 (1H, d, J = 7.7 Hz), 7.63 (1H, d, J = 7.7 Hz), 6.72 (1H, br. s), 2.94 (1H, m), 0.92 − 0.88 (2H, m), 0.70 − 0.68 (2H, m); 13C-NMR (150 MHz, CDCl3), δ (ppm): 166.5, 148.1, 136.0, 133.3, 129.8, 126.0, 121.6, 23.4, 6.7.
N-cyclopropyl-4-methyl-3-nitrobenzamide (17b) light yellow solid, yield 90%
1H-NMR (600 MHz, CDCl3), δ (ppm): 8.32 (1H, s), 7.96 (1H, dd, J = 8.0, 1.5 Hz), 7.43 (1H, d, J = 8.0 Hz), 6.56 (1H, br. s), 2.94 (1H, m), 2.65 (3H, s), 0.93 − 0.88 (2H, m), 0.71 − 0.66 (2H, m); 13C-NMR (150 MHz, CDCl3), δ (ppm): 166.5, 148.9, 137.0, 133.4, 133.2, 131.4, 122.8, 23.3, 20.4, 6.7.
N-cyclopropyl-4-methoxy-3-nitrobenzamide (17c) light yellow solid, yield 76%
1H-NMR (600 MHz, CDCl3), δ (ppm): 8.22 (1H, d, J = 2.1 Hz), 8.05 (1H, dd, J = 8.7, 2.1 Hz), 7.14 (1H, d, J = 8.7 Hz), 6.57 (1H, br. s), 4.02 (3H, s), 2.91 (1H, m), 2.65 (3H, s), 0.91 − 0.85 (2H, m), 0.69 − 0.66 (2H, m); 13C-NMR (150 MHz, CDCl3), δ (ppm): 166.3, 155.1, 138.8, 133.4, 126.5, 124.2, 113.4, 56.8, 23.3, 23.1, 6.6.
N-cyclopentyl-3-nitrobenzamide (17d) light yellow solid, yield 89%
1H-NMR (600 MHz, CDCl3), δ (ppm): 8.56 (1H, br. s), 8.34 (1H, ddd, J = 8.1, 2.1, 0.9 Hz), 8.17 (1H, d, J = 8.1 Hz), 7.65 (1H, t, J = 8.0 Hz), 6.36 (1H, d, J = 5.3 Hz), 4.44 (1H, m), 2.18 − 2.10 (2H, m), 1.80 − 1.74 (2H, m), 1.71 − 1.66 (2H, m), 1.60 − 1.52 (2H, m); 13C-NMR (150 MHz, CDCl3), δ (ppm): 164.8, 148.1, 136.4, 133.3, 129.8, 125.8, 121.6, 52.1, 52.0, 33.1, 33.1, 23.8.
N-cyclopentyl-4-methyl-3-nitrobenzamide (17e) light yellow solid, yield 84%
1H-NMR (600 MHz, CDCl3), δ (ppm): 8.30 (1H, d, J = 1.5 Hz), 7.94 (1H, dd, J = 8.0, 1.5 Hz), 7.41 (1H, d, J = 8.0 Hz), 6.27 (1H, d, J = 4.6 Hz), 4.40 (1H, m), 2.64 (3H, s), 2.13 − 2.07 (2H, m), 1.77 − 1.70 (2H, m), 1.70 − 1.64 (2H, m), 1.56 − 1.49 (2H, m); 13C-NMR (150 MHz, CDCl3), δ (ppm): 164.7, 148.9, 136.7, 134.0, 133.1, 131.4, 122.8, 52.0, 33.1, 23.8, 20.4.
N-cyclopentyl-4-methoxy-3-nitrobenzamide (17f) light yellow solid, yield 76%
1H-NMR (600 MHz, CDCl3), δ (ppm): 8.13 (1H, d, J = 2.1 Hz), 7.96 (1H, dd, J = 8.8, 2.1 Hz), 7.05 (1H, d, J = 8.8 Hz), 6.17 (1H, d, J = 6.4 Hz), 4.31 (1H, m), 3.93 (3H, s), 2.04 − 1.98 (2H, m), 1.70 − 1.63 (2H, m), 1.62 − 1.55 (2H, m), 1.48 − 1.42 (2H, m); 13C-NMR (150 MHz, CDCl3), δ (ppm): 164.4, 154.9, 138.9, 133.4, 127.0, 124.1, 113.4, 56.8, 51.8, 33.1, 33.1, 29.6, 23.8.
N-cyclohexyl-3-nitrobenzamide (17 g) light yellow solid, yield 88%
1H-NMR (600 MHz, CDCl3), δ (ppm): 8.19 (1H, s), 7.84 (1H, d, J = 8.5 Hz), 7.81 (1H, d, J = 8.5 Hz), 6.12 (1H, d, J = 6.6 Hz), 3.96 (1H, m), 2.04 − 2.02 (2H, br. s), 1.79 − 1.77 (2H, br. s), 1.68 − 1.66 (1H, m), 1.45 − 1.38 (2H, m), 1.29 − 1.22 (2H, m); 13C-NMR (150 MHz, CDCl3), δ (ppm): 163.3, 149.6, 135.5, 135.4, 131.4, 123.9, 117.5, 49.4, 33.1, 25.4, 24.9.
N-cyclohexyl-4-methyl-3-nitrobenzamide (17h) light yellow solid, yield 86%
1H-NMR (400 MHz, CDCl3), δ (ppm): 8.30 (1H, s), 7.94 (1H, d, J = 7.9 Hz), 7.42 (1H, d, J = 7.9 Hz), 6.12 (1H, d, J = 6.7 Hz), 3.97 (1H, m), 2.04 − 2.02 (2H, br. s), 1.81 − 1.76 (2H, br. s), 1.69 − 1.66 (1H, m), 1.45 − 1.38 (2H, m), 1.29 − 1.22 (2H, m); 13C-NMR (150 MHz, CDCl3), δ (ppm): 164.1, 148.9, 136.7, 134.1, 133.2, 131.5, 122.8, 49.1, 33.1, 25.5, 24.9, 20.5.
N-cyclohexyl-4-methoxy-3-nitrobenzamide (17i) light yellow solid, yield 92%
1H-NMR (600 MHz, CDCl3), δ (ppm): 8.22 (1H, d, J = 2.1 Hz), 8.05 (1H, dd, J = 8.7, 2.1 Hz), 7.14 (1H, d, J = 8.7 Hz), 6.08 (1H, d, J = 7.3 Hz), 4.02 (3H, s), 3.96 (1H, m), 2.04 − 2.02 (2H, br. s), 1.81 − 1.76 (2H, br. s), 1.69 − 1.66 (1H, m), 1.45 − 1.38 (2H, m), 1.29 − 1.22 (2H, m); 13C-NMR (150 MHz, CDCl3), δ (ppm): 163.8, 163.8, 155.0, 138.9, 133.3, 127.2, 124.0, 113.4, 56.8, 49.1, 49.0, 33.1, 25.4, 24.9.
N-cycloheptyl-3-nitrobenzamide (17j) light yellow solid, yield 92%
1H-NMR (600 MHz, CDCl3), δ (ppm): 8.56 (1H, t, J = 2.1 Hz), 8.35 (1H, ddd, J = 8.2, 2.1, 0.9 Hz), 8.16 (1H, d, J = 8.2 Hz), 7.65 (1H, t, J = 8.2 Hz), 6.28 (1H, d, J = 6.7 Hz), 4.18 (1H, m), 2.10 − 2.04 (2H, m), 1.70 − 1.68 (4H, m), 1.57 − 1.56 (6H, m); 13C-NMR (150 MHz, CDCl3), δ (ppm): 163.9, 148.1, 136.7, 133.2, 129.7, 125.8, 121.5, 51.5, 35.1, 27.9, 24.1.
N-cycloheptyl-4-methyl-3-nitrobenzamide (17k) light yellow solid, yield 82%
1H-NMR (600 MHz, CDCl3), δ (ppm): 8.30 (1H, s), 7.93 (1H, d, J = 7.9 Hz), 7.41 (1H, d, J = 7.9 Hz), 6.23 (1H, d, J = 6.7 Hz), 4.15 (1H, m), 2.64 (3H, s), 2.06 − 2.02 (2H, m), 1.70 − 1.67 (4H, m), 1.57 − 1.55 (6H, m); 13C-NMR (150 MHz, CDCl3), δ (ppm): 163.8, 148.9, 136.7, 134.2, 133.2, 131.4, 122.8, 51.3, 35.1, 28.0, 24.1, 20.5.
N-cycloheptyl-4-methoxy-3-nitrobenzamide (17 l) light yellow solid, yield 89%
1H-NMR (600 MHz, CDCl3), δ (ppm): 8.21 (1H, d, J = 2.2 Hz), 8.05 (1H, dd, J = 8.7, 2.2 Hz), 7.14 (1H, d, J = 8.7 Hz), 6.17 (1H, d, J = 7.40 Hz), 4.14 (1H, m), 4.02 (3H, s), 2.06 − 2.02 (2H, m), 1.70 − 1.67 (4H, m), 1.57 − 1.55 (6H, m); 13C-NMR (150 MHz, CDCl3), δ (ppm): 163.5, 154.9, 138.9, 133.3, 127.2, 124.0, 113.4, 56.8, 51.3, 51.2, 35.1, 35.1, 28.0, 24.1.
Synthesis of compounds 18
To a solution of intermediate 17 (3 mmol), iron powder (12 mmol) and ammonium chloride (NH4Cl, 15 mmol) in 95% ethanol (20 ml), and a catalytic amount of glacial acetic acid was added under strenuous stirring, and then the mixture was allowed to warm up to reflux for 4 h. The additional iron powder was removed by filtration, and the filtrate was concentrated to dryness to give the crude product and used directly for the next reaction without purification, yield 80–96%.
3-amino-N-cyclopropylbenzamide (18a) light brown solid, yield 86%
1H-NMR (600 MHz, CDCl3), δ(ppm): 7.16 (1H, t, J = 7.8 Hz), 7.11 (1H, s), 7.00 (1H, d, J = 7.8 Hz), 6.77 (1H, dd, J = 7.8, 1.5 Hz), 6.31 (1H, s), 3.80 (2H, br. s), 2.87 (1H, m), 0.84 (2H, m), 0.59 (2H, m); 13C-NMR (150 MHz, CDCl3), δ(ppm): 169.1, 146.8, 135.6, 129.3, 117.8, 116.2, 113.7, 23.1, 6.7.
3-amino-N-cyclopropyl-4-methylbenzamide (18b) light brown solid, yield 89%
1H-NMR (600 MHz, CDCl3), δ(ppm): 7.12 (1H, d, J = 1.5 Hz), 7.04 (1H, d, J = 7.7 Hz), 6.95 (1H, dd, J = 7.7, 1.5 Hz), 6.27 (1H, br. s), 3.72 (2H, br. s), 2.87 (1H, m), 2.17 (3H, s), 0.84 (2H, m), 0.59 (2H, m); 13C-NMR (150 MHz, CDCl3), δ(ppm): 169.0, 144.8, 133.3, 130.3, 125.8, 116.2, 113.6, 23.0, 17.3, 6.7.
3-amino-N-cyclopropyl-4-methoxybenzamide (18c) light brown solid, yield 81%
1H-NMR (600 MHz, CDCl3), δ(ppm): 7.16 (1H, d, J = 2.1 Hz), 7.07 (1H, dd, J = 8.3, 2.1 Hz), 6.74 (1H, d, J = 8.3 Hz), 6.23 (1H, br. s), 3.87 (5H, br. s), 2.86 (1H, m), 0.84 (2H, m), 0.59 (2H, m); 13C-NMR (150 MHz, CDCl3), δ(ppm): 168.8, 149.7, 136.2, 127.2, 117.1, 113.5, 109.4, 55.5, 23.0, 6.7.
3-amino-N-cyclopentylbenzamide (18d) light brown solid, yield 84%
1H-NMR (600 MHz, CDCl3), δ(ppm): 7.18 (1H, t, J = 7.8 Hz), 7.12 (1H, s), 7.04 (1H, d, J = 7.6 Hz), 6.78 (1H, dd, J = 7.6, 1.5 Hz), 6.07 (1H, s), 4.38 (1H, m), 2.11 − 2.05 (2H, m), 1.74 − 1.71 (2H, m), 1.68 − 1.62 (2H, m), 1.51 − 1.45 (2H, m); 13C-NMR (150 MHz, CDCl3), δ(ppm): 167.4, 146.7, 136.1, 129.3, 117.7, 116.2, 113.7, 51.6, 33.2, 23.8.
3-amino-N-cyclopentyl-4-methylbenzamide (18e) light brown solid, yield 83%
1H-NMR (600 MHz, CDCl3), δ(ppm): 7.14 (1H, s), 7.07 (1H, d, J = 7.7 Hz), 6.99 (1H, d, J = 7.7 Hz), 6.05 (1H, br. s), 4.38 (1H, m), 2.19 (3H, s), 2.11 − 2.05 (2H, m), 1.74 − 1.71 (2H, m), 1.68 − 1.62 (2H, m), 1.51 − 1.45 (2H, m); 13C-NMR (150 MHz, CDCl3), δ(ppm): 167.3, 144.8, 133.8, 130.3, 125.6, 116.2, 113.6, 51.5, 33.2, 23.8, 17.3.
3-amino-N-cyclopentyl-4-methoxybenzamide (18f) light brown solid, yield 79%
1H-NMR (600 MHz, CDCl3), δ(ppm): 7.15 (1H, d, J = 1.2 Hz), 7.09 (1H, dd, J = 8.2, 1.2 Hz), 6.75 (1H, d, J = 8.2 Hz), 5.98 (1H, d, J = 5.4 Hz), 4.39 − 4.34 (1H, m), 3.88 (2H, s), 3.87 (3H, s), 2.09 − 2.04 (2H, m), 1.74 − 1.68 (2H, m), 1.67 − 1.62 (2H, m), 1.49 − 1.43 (2H, m); 13C-NMR (150 MHz, CDCl3), δ(ppm): 167.1, 149.5, 136.1, 127.8, 117.1, 113.5, 109.5, 55.6, 51.5, 33.2, 33.2, 23.8, 23.8.
3-amino-N-cyclohexylbenzamide (18 g) light brown solid, yield 86%
1H-NMR (600 MHz, CDCl3), δ(ppm): 7.18 (1H, t, J = 7.7 Hz), 7.12 (1H, s), 7.04 (1H, d, J = 7.5 Hz), 6.78 (1H, d, J = 7.1 Hz), 5.99 (1H, br. s), 3.96 (1H, m), 2.02 (2H, m), 1.75 (2H, m), 1.65 (1H, m), 1.43 (2H, m), 1.22 (3H, m); 13C-NMR (150 MHz, CDCl3), δ(ppm): 166.9, 146.7, 136.3, 129.3, 117.6, 116.2, 113.7, 48.5, 33.2, 25.5, 24.9.
3-amino-N-cyclohexyl-4-methylbenzamide (18h) light brown solid, yield 80%
1H-NMR (600 MHz, CDCl3), δ(ppm): 7.14 (1H, s), 7.07 (1H, d, J = 7.7 Hz), 7.00 (1H, dd, J = 7.7, 1.5 Hz), 5.97 (1H, br. s), 3.96 (1H, m), 2.19 (3H, s), 2.02 (2H, m), 1.75 (2H, m), 1.65 (1H, m), 1.43 (2H, m), 1.22 (3H, m); 13C-NMR (150 MHz, CDCl3), δ(ppm): 166.8, 144.8, 133.9, 130.3, 125.6, 116.2, 113.6, 48.4, 33.2, 25.6, 24.9, 17.3.
3-amino-N-cyclohexyl-4-methoxybenzamide (18i) light brown solid, yield 82%
1H-NMR (600 MHz, CDCl3), δ(ppm): 7.17 (1H, d, J = 1.9 Hz), 7.11 (1H, dd, J = 8.3, 1.9 Hz), 6.77 (1H, d, J = 8.3 Hz), 5.92 (1H, br. s), 3.95 (1H, m), 3.89 (3H, s), 2.02 (2H, m), 1.75 (2H, m), 1.65 (1H, m), 1.43 (2H, m), 1.22 (3H, m); 13C-NMR (150 MHz, CDCl3), δ(ppm): 166.6, 149.5, 136.1, 127.9, 117.1, 113.5, 109.4, 55.5, 48.4, 33.3, 25.6, 24.9.
3-amino-N-cycloheptylbenzamide (18j) light brown solid, yield 87%
1H-NMR (600 MHz, CDCl3), δ(ppm): 7.19 (1H, t, J = 7.8 Hz), 7.12 (2H, s), 7.04 (1H, d, J = 7.5 Hz), 6.79 (1H, d, J = 7.3 Hz), 6.05 (1H, d, J = 5.3 Hz), 4.15 (1H, m), 2.02 (2H, m), 1.66 (4H, m), 1.55 (6H, m); 13C-NMR (150 MHz, CDCl3), δ(ppm): 166.6, 146.7, 136.3, 129.3, 117.6, 116.2, 113.7, 50.7, 35.1, 28.0, 24.1.
3-amino-N-cycloheptyl-4-methylbenzamide (18k) light brown solid, yield 88%
1H-NMR (600 MHz, CDCl3), δ(ppm): 7.11 (1H, d, J = 1.0 Hz), 7.05 (1H, d, J = 7.7 Hz), 6.97 (1H, dd, J = 7.7, 1.0 Hz), 6.02 (1H, d, J = 6.6 Hz), 4.13 (1H, m), 3.72 (2H, br. s), 2.17 (3H, s), 2.01 − 1.97 (2H, m), 1.64 − 1.49 (10H, m); 13C-NMR (150 MHz, CDCl3), δ(ppm): 166.5, 144.8, 134.1, 130.3, 125.5, 116.2, 113.6, 50.7, 35.2, 28.1, 24.1, 17.3.
3-amino-N-cycloheptyl-4-methoxybenzamide (18 l) White solid, yield 91%
1H-NMR (600 MHz, CDCl3), δ(ppm): 7.15 (1H, d, J = 1.0 Hz), 7.08 (1H, d, J = 8.0 Hz), 6.75 (1H, dd, J = 8.0, 1.0 Hz), 5.96 (2H, d, J = 5.7 Hz), 4.12 (1H, s), 3.88 (5H, br. s), 2.01 − 2.00 (2H, m), 1.64 (4H, br. s), 1.53 (6H, m); 13C-NMR (150 MHz, CDCl3), δ(ppm): 166.3, 149.5, 136.1, 128.0, 117.0, 113.5, 109.5, 55.5, 50.6, 35.2, 28.1, 24.1.
Synthesis of compounds 19a–l
To a solution of intermediate 10 (1 mmol), intermediate 18 (1 mmol) and DIEA (2 mmol) in DMF (10 ml), and HATU (1 mmol) was added at room temperature for 24 h. The mixture was added water (50 ml), extracted with ethyl acetate (30 ml) three times. The combined organic layers were washed with water, saturated aqueous sodium bicarbonate and brine, and then dried over anhydrous sodium sulphate. After removing the solvent under reduced pressure, the crude product was purified by flash chromatography on silica gel, eluting with dichloromethane/methanol (2–4%), yield 75–90%.
N-cyclopropyl-3–(2-(1,3-dimethyl-2,6-dioxo-1,2,3,6-tetrahydro-9H-purin-9-yl)acetamido)benzamide (19a)
White solid, m.p. 222–224 °C, yield 76%. 1H-NMR (600 MHz, DMSO-d6), δ (ppm): 10.56 (1H, s), 8.44 (1H, d, J = 4.1 Hz), 8.08 (1H, s), 7.98 (1H, s), 7.73 (1H, d, J = 8.0 Hz), 7.49 (1H, d, J = 7.7 Hz), 7.38 (1H, t, J = 7.9 Hz), 5.21 (2H, s), 3.46 (3H, s), 3.19 (3H, s), 2.83 (1H, m), 0.68 (2H, br. s), 0.56 (2H, br. s); 13C-NMR (150 MHz, CDCl3), δ (ppm): 167.8, 165.5, 154.9, 151.4, 148.4, 144.2, 139.0, 135.7, 129.2, 122.4, 122.0, 118.7, 106.9, 49.1, 29.9, 27.9, 23.5, 6.1; HRMS (ESI)- calculated for C19H20N6O4, [M-H]-: m/z 395.1473, found 395.1463.
N-cyclopropyl-3–(2-(1,3-dimethyl-2,6-dioxo-1,2,3,6-tetrahydro-9H-purin-9-yl)acetamido)-4-methylbenzamide (19b)
White solid, m.p. 218–219 °C, yield 80%. 1H-NMR (600 MHz, DMSO-d6), δ (ppm): 9.85 (1H, s), 8.39 (1H, d, J = 4.0 Hz), 8.09 (1H, s), 7.84 (1H, s), 7.56 (1H, d, J = 8.0 Hz), 7.29 (1H, d, J = 8.0 Hz), 5.25 (2H, s), 3.45 (3H, s), 3.22 (3H, s), 2.82 (1H, m), 2.27 (3H, s), 0.67 (2H, br. s), 0.54 (2H, br. s); 13C-NMR (150 MHz, DMSO-d6), δ (ppm): 167.4, 165.7, 154.9, 151.5, 148.4, 144.1, 136.0, 135.8, 132.9, 130.6, 124.7, 124.5, 106.9, 48.9, 29.9, 27.9, 23.4, 18.2, 6.1; HRMS (ESI)+ calculated for C20H22N6O4, [M + H]+: m/z 411.1775, found 411.1776.
N-cyclopropyl-3–(2-(1,3-dimethyl-2,6-dioxo-1,2,3,6-tetrahydro-9H-purin-9-yl)acetamido)-4-methoxybenzamide (19c)
White solid, m.p. 231–233 °C, yield 86%. 1H-NMR (600 MHz, DMSO-d6), δ (ppm): 9.81 (1H, s), 8.39 (1H, s), 8.28 (1H, d, J = 3.8 Hz), 8.08 (1H, s), 7.58 (1H, dd, J = 8.5, 1.7 Hz), 7.10 (1H, d, J = 8.6 Hz), 5.29 (2H, s), 3.92 (3H, s), 3.45 (3H, s), 3.21 (3H, s), 2.79 (1H, m), 0.65 (2H, br. s), 0.53 (2H, br. s); 13C-NMR (150 MHz, DMSO-d6), δ (ppm): 167.5, 165.7, 154.9, 152.1, 151.5, 148.3, 144.2, 127.0, 126.8, 124.3, 121.7, 110.8, 106.9, 56.4, 49.3, 29.9, 27.9, 23.4, 6.1; HRMS (ESI)- calculated for C20H22N6O5, [M-H]−: m/z 425.1579, found 425.1570.
N-cyclopentyl-3–(2-(1,3-dimethyl-2,6-dioxo-1,2,3,6-tetrahydro-9H-purin-9-yl)acetamido)benzamide (19d)
White solid, m.p. 207–208 °C, yield 87%. 1H-NMR (600 MHz, DMSO-d6), δ (ppm): 10.06 (1H, s), 8.40 (1H, d, J = 7.2 Hz), 8.11 (1H, s), 8.03 (1H, d, J = 1.7 Hz), 7.77 (1H, d, J = 8.3 Hz), 7.61 (1H, d, J = 7.2 Hz), 5.30 (2H, s), 4.19 (1H, m), 3.45 (3H, s), 3.22 (3H, s), 1.86 (2H, br. s), 1.67 (2H, br. s), 1.51 (4H, m); 13C-NMR (150 MHz, DMSO-d6), δ (ppm): 166.2, 165.5, 162.7, 154.9, 151.4, 148.9, 148.4, 144.2, 138.9, 136.2, 129.1, 128.9, 122.6, 121.8, 118.8, 116.5, 114.9, 113.3, 106.9, 51.3, 51.2, 49.1, 36.2, 32.5, 31.2, 29.9, 27.9, 24.0; HRMS (ESI)+ calculated for C21H24N6O4, [M + H]+: m/z 425.1932, found 425.1935.
N-cyclopentyl-3–(2-(1,3-dimethyl-2,6-dioxo-1,2,3,6-tetrahydro-9H-purin-9-yl)acetamido)-4-methylbenzamide (19e)
White solid, m.p. 211–213 °C, yield 89%. 1H-NMR (600 MHz, DMSO-d6), δ (ppm): 9.84 (1H, s), 8.23 (1H, d, J = 7.3 Hz), 8.09 (1H, s), 7.84 (1H, d, J = 1.5 Hz), 7.60 (1H, dd, J = 7.9, 1.5 Hz), 7.29 (1H, d, J = 7.9 Hz), 5.26 (2H, s), 4.19 (1H, m), 3.45 (3H, s), 3.22 (3H, s), 2.27 (3H, s), 1.86 (2H, br. s), 1.67 (2H, br. s), 1.50 (2H, br. s); 13C-NMR (150 MHz, DMSO-d6), δ (ppm): 165.8, 165.7, 154.9, 151.5, 148.4, 144.1, 135.9, 135.6, 133.3, 130.5, 124.8, 124.7, 106.9, 51.3, 48.9, 32.5, 29.9, 27.9, 24.0, 18.2; HRMS (ESI)- calculated for C22H26N6O4, [M-H]-: m/z 437.1943, found 437.1932.
N-cyclopentyl-3–(2-(1,3-dimethyl-2,6-dioxo-1,2,3,6-tetrahydro-9H-purin-9-yl)acetamido)-4-methoxybenzamide (19f)
White solid, m.p. 219–220 °C, yield 81%. 1H-NMR (600 MHz, DMSO-d6), δ (ppm): 9.80 (1H, s), 8.38 (1H, s), 8.12 (1H, d, J = 7.3 Hz), 8.08 (1H, s), 7.62 (1H, dd, J = 8.0, 2.0 Hz), 7.11 (1H, d, J = 8.6 Hz), 5.29 (2H, s), 4.17 (1H, m), 3.93 (3H, s), 3.46 (3H, s), 3.21 (3H, s), 1.84 (2H, br. s), 1.66 (2H, br. s), 1.49 (4H, m); 13C-NMR (150 MHz, DMSO-d6), δ (ppm): 165.9, 165.7, 154.9, 152.0, 151.5, 148.4, 144.2, 127.5, 126.7, 124.4, 121.9, 110.7, 106.9, 56.4, 51.3, 49.3, 32.5, 29.9, 27.9, 24.0; HRMS (ESI)+ calculated for C22H26N6O5, [M + H]+: m/z 455.2037, found 455.2041.
N-cyclohexyl-3–(2-(1,3-dimethyl-2,6-dioxo-1,2,3,6-tetrahydro-9H-purin-9-yl)acetamido)benzamide (19g)
White solid, m.p. 195–197 °C, yield 88%. 1H-NMR (600 MHz, DMSO-d6), δ (ppm): 10.56 (1H, s), 8.26 (1H, d, J = 7.9 Hz), 8.08 (1H, s), 7.95 (1H, s), 7.74 (1H, d, J = 8.0 Hz), 7.52 (1H, d, J = 7.7 Hz), 7.38 (1H, t, J = 7.9 Hz), 5.21 (2H, s), 3.93 (1H, m), 3.46 (3H, s), 3.20 (3H, s), 1.82 (2H, br. s), 1.64 (2H, br. s), 1.57 (4H, m), 1.42 (2H, m); 13C-NMR (150 MHz, DMSO-d6), δ (ppm): 165.5, 165.4, 154.9, 151.5, 148.4, 144.2, 138.9, 136.4, 129.1, 122.6, 121.8, 118.8, 106.9, 50.9, 49.1, 40.4, 34.7, 29.9, 28.2, 27.9, 24.3; HRMS (ESI)+ calculated for C22H26N6O4, [M + H]+: m/z 439.2088, found 439.2108.
N-cyclohexyl-3–(2-(1,3-dimethyl-2,6-dioxo-1,2,3,6-tetrahydro-9H-purin-9-yl)acetamido)-4-methylbenzamide (19h)
White solid, m.p. 199–201 °C, yield 85%. 1H-NMR (600 MHz, DMSO-d6), δ (ppm): 9.84 (1H, s), 8.15 (1H, d, J = 8.0 Hz), 8.09 (1H, s), 7.85 (1H, s), 7.60 (1H, d, J = 8.0 Hz), 7.29 (1H, d, J = 7.9 Hz), 5.26 (2H, s), 3.73 (1H, m), 3.45 (3H, s), 3.20 (3H, s), 2.28 (3H, s), 1.78 (2H, br. s), 1.72 (2H, br. s), 1.59 (1H, d, J = 12.4 Hz), 1.29 (4H, m), 1.10 (1H, m); 13C-NMR (150 MHz, DMSO-d6), δ (ppm): 165.7, 165.2, 154.9, 151.5, 148.4, 144.1, 135.9, 135.6, 133.4, 130.5, 124.9, 124.7, 106.9, 48.9, 48.7, 32.8, 29.9, 27.9, 25.7, 25.4, 18.2; HRMS (ESI)+ calculated for C23H28N6O4, [M + H]+: m/z 453.2245, found 453.2251.
N-cyclohexyl-3–(2-(1,3-dimethyl-2,6-dioxo-1,2,3,6-tetrahydro-9H-purin-9-yl)acetamido)-4-methoxybenzamide (19i)
White solid, m.p. 185–187 °C, yield 86%. 1H-NMR (600 MHz, DMSO-d6), δ (ppm): 9.80 (1H, s), 8.39 (1H, s), 8.08 (1H, s), 8.02 (1H, d, J = 7.9 Hz), 7.62 (1H, dd, J = 8.6, 1.7 Hz), 7.11 (1H, d, J = 8.6 Hz), 5.29 (2H, s), 3.92 (3H, s), 3.71 (1H, m), 3.45 (3H, s), 3.21 (3H, s), 1.77 (2H, br. s), 1.71 (2H, br. s), 1.59 (1H, d, J = 12.4 Hz), 1.31 − 1.23 (4H, m), 1.10 − 1.08 (1H, m); 13C-NMR (150 MHz, DMSO-d6), δ (ppm): 165.7, 165.3, 154.9, 152.0, 151.5, 148.4, 144.2, 127.5, 126.7, 124.4, 121.9, 110.7, 106.9, 56.4, 49.3, 48.7, 32.9, 29.9, 27.9, 25.7, 25.4; HRMS (ESI)+ calculated for C23H28N6O5, [M + H]+: m/z 469.2194, found 469.2201.
N-cycloheptyl-3–(2-(1,3-dimethyl-2,6-dioxo-1,2,3,6-tetrahydro-9H-purin-9-yl)acetamido)benzamide (19j)
White solid, m.p. 189–190 °C, yield 85%. 1H-NMR (600 MHz, DMSO-d6), δ (ppm): 10.55 (1H, s), 8.25 (1H, d, J = 7.9 Hz), 8.08 (1H, s), 7.95 (1H, s), 7.74 (1H, d, J = 8.1 Hz), 7.52 (1H, d, J = 7.7 Hz), 7.38 (1H, t, J = 7.9 Hz), 5.21 (2H, s), 3.94 (1H, m), 3.46 (3H, s), 3.22 (3H, s), 1.82 (2H, br. s), 1.64 (2H, br. s), 1.56 (4H, m), 1.49 (2H, m), 1.40 (2H, m); 13C-NMR (150 MHz, DMSO-d6), δ (ppm): 165.5, 165.4, 154.9, 151.4, 148.4, 144.2, 138.9, 136.4, 129.1, 122.6, 121.8, 118.8, 106.9, 50.9, 49.1, 34.7, 29.9, 28.2, 27.9, 24.3; HRMS (ESI)+ calculated for C23H28N6O4, [M + H]+: m/z 453.2245, found 453.2246.
N-cycloheptyl-3–(2-(1,3-dimethyl-2,6-dioxo-1,2,3,6-tetrahydro-9H-purin-9-yl)acetamido)-4-methylbenzamide (19k)
White solid, m.p. 194–196 °C, yield 87%. 1H-NMR (600 MHz, DMSO-d6), δ (ppm): 9.84 (1H, s), 8.19 (1H, d, J = 7.9 Hz), 8.09 (1H, s), 7.83 (1H, s), 7.59 (1H, dd, J = 7.9, 1.3 Hz), 7.29 (1H, d, J = 7.9 Hz), 5.26 (2H, s), 3.93 (1H, m), 3.45 (3H, s), 3.22 (3H, s), 2.27 (3H, s), 1.82 (2H, br. s), 1.64 (2H, br. s), 1.56 (4H, m), 1.49 (2H, m), 1.40 (2H, m); 13C-NMR (150 MHz, DMSO-d6), δ (ppm): 165.7, 165.1, 154.9, 151.5, 148.4, 144.1, 135.9, 135.7, 133.4, 130.5, 124.8, 124.7, 106.9, 50.8, 48.9, 40.4, 34.7, 29.9, 28.2, 27.9, 24.3, 18.2; HRMS (ESI)+ calculated for C24H30N6O4, [M + H]+: m/z 467.2401, found 467.2409.
N-cycloheptyl-3–(2-(1,3-dimethyl-2,6-dioxo-1,2,3,6-tetrahydro-9H-purin-9-yl)acetamido)-4-methoxybenzamide (19l)
White solid, m.p. 197–198 °C, yield 94%. 1H-NMR (600 MHz, DMSO-d6), δ (ppm): 9.79 (1H, s), 8.38 (1H, d, J = 1.5 Hz), 8.08 (1H, s), 8.07 (1H, d, J = 8.0 Hz), 7.62 (1H, dd, J = 8.0, 1.8 Hz), 7.10 (1H, d, J = 8.6 Hz), 5.29 (2H, s), 3.92 (3H, s), 3.91 (1H, m), 3.45 (3H, s), 3.21 (3H, s), 1.79 (2H, br. s), 1.63 (2H, br. s), 1.54 (4H, m), 1.48 (2H, m), 1.39 (2H, m); 13C-NMR (150 MHz, DMSO-d6), δ (ppm): 165.7, 165.1, 154.9, 152.0, 151.5, 148.3, 144.2, 127.6, 126.7, 124.4, 121.8, 110.7, 106.9, 56.4, 50.8, 49.3, 34.8, 29.9, 28.2, 27.9, 24.4; HRMS (ESI)+ calculated for C24H30N6O4, [M + H]+: m/z 483.2350, found 483.2344.
Molecular docking
The discovery Studio 3.5 was used to perform molecular docking, and the structure of ATAD2 (PDB code 6YB4)Citation10 was downloaded from https://www.rcsb.org. First, the potential active site was defined according to the reference ligand by a radius of 8.5 Å. The structure of ATAD2 bromodomain is prepared by removing water molecules, adding hydrogen atoms, and charging the Charmm forcefield. The ligands were prepared by adding hydrogen atoms and energy minimisation.
Molecular dynamics simulations and binding free energy calculation
The MD simulations were conducted by Amber 10 packageCitation26. The steepest descent method was adopted, and energy minimisation was restrained with 0.1 kcal/mol•Å2 for 5000 steps. Next, the restraints of the ligand were removed, and the last energy minimisation was executed without any restraints. For the equilibration and production, the integration time step was set to 2 fs. The temperature was set from 0 to 310 K for 50 ps for the annealed program. Last, the equilibration of the complex system was processed for 500 ps without any restraints. The production was carried out for 200 ns under removing all restrictions. Additionally, the binding free energy of the complexs was calculated by MM-GBSA using AMBER10Citation27.
The enzymatic assay
The assay was performed by TR-FRET technology using recombinant bromodomain and BET LigandCitation8. The compounds were diluted with indicated folds in 5% DMSO in reaction buffer and 2 µl of the dilution was added to a 20 µl reaction so that the final concentration of DMSO is 0.5% in all of the reactions. The 20 µl reaction mixture consists of the ATAD2 recombinant bromodomain protein, the indicated amount of the inhibitor, ligand, and the reactive dyes. The reaction mixture was incubated for 3 h before reading the TR-FRET signal. Fluorescence signals for both the donor and acceptor dyes were measured using a Tecan Infinite M1000 plate reader. Binding experiments were performed in duplicate at each concentration and the data were analysed using GraphPad Prism.
Cell culture, antibodies, and reagents
BT-549 cells, MDA-MB-231 cells, and MCF-10A cells were obtained from American Type Culture Collection (ATCC, Manassas, VA, USA) and were cultured in RPIM-1640 supplemented with 10% foetal bovine serum under a 5% CO2 atmosphere. The following antibodies were used in this study: ATAD2 (1:1000; 50,563, CST), c-Myc (1:1000; 18,583, CST), p-c-MycSer62 (1:1000; 13,748, CST), caspase-9 (1:1000; 9508, CST), Bax(1:2000; 5023,CST), Bcl-2(1:2000; 15,071,CST), caspase8(1:1000; 9746, CST), MMP-2(1:1000; 40,994,CST), E-cadherin (1:1000; 14,472,CST), PARP (1:2000; 9532, CST), caspase-3 (1:2000; 9665, CST), and β-actin (1:2000; 3700, CST). MTT (M2128, St. Louis, MO, USA). BAY-850 were purchased from MCE (MedChemExpress LLC, HY-119254, 98%).
Cell viability assay
BT-549 cells were seeded at a density of 5 × 104 cells/ml in a 96-well plateCitation15. Following a 24-h incubation period, the cells were exposed to various concentrations of different compounds for the specified durations. Cell viability was evaluated using an MTT assay.
3D Cell culture
For 3D cell culture, 10,000 cells were placed in 96-well spherical plates for 24h and treated with different concentrations of compound 19f, BAY-859 or AM879. The diameter of the cell balls was measured by photographing.
Apoptosis assays
To assess apoptosis, BT-549 cells were exposed to varying concentrations of compound 19f, and apoptosis ratios were quantified via Annexin-V/PI double staining.
Immunofluorescence analysis
To prevent non-specific antibody binding, BT-549 cells were treated with a blocking buffer (PBS +1.5% goat serum) prior to immunofluorescence staining. The cells were subsequently incubated with a p-c-MycSer62 antibody (1:200) diluted in blocking buffer and allowed to incubate overnight at 4 °C. Afterward, the cells were further incubated with fluorescent-labelled secondary antibodies for 1 h at room temperature.
Western blot
Cells were exposed to AM879, BAY-850 or compound 19f for the specified time intervalsCitation15. Both adherent and floating cells were harvested and subsequently resuspended in a lysis buffer, followed by incubation at 4 °C for 1 h. Following centrifugation at 12,000 rpm for 20 min, the protein content of the supernatant was quantified using the BCA protein assay. Then protein were separated by 8–15% SDS-PAGE and transferred onto PVDF membranes. The membranes were blocked with blocking buffer and subjected to primary antibody incubation, followed by incubation with HRP-conjugated secondary antibody. Protein detection was achieved using ECL as the HRP substrate.
Transwell assay
BT-549 cells were seeded into the upper chamber of a Transwell insert at a density of 15,000 cells per well. The cells were treated with either DMSO or compound 19f for 48 h, after which non-migrated cells were removed from the upper surface, while the migrated cells were stained with crystal violet. Then, the cells were washed with PBS and imaged using a phase-contrast microscope.
Statistical analysis
The data are presented as means ± standard error of the mean (SEM) and were confirmed by at least three independent experiments. Statistical analysis was performed using one-way analysis of variance (ANOVA) followed by Student–Newman–Keuls test for post hoc comparisons between multiple groups. A value of p < 0.05 was considered to be statistically significant.
Author contributions
Xiaojun Yao and Jin Zhang conceived and designed the experiments, Dahong Yao, Jieshu You and Xuetao Yang performed the experiments, and Dahong Yao wrote the manuscript.
Supplemental Material
Download PDF (8.7 MB)Disclosure statement
The authors declare no conflict of interest.
Additional information
Funding
References
- Clapier CR, Iwasa J, Cairns BR, Peterson CL. Mechanisms of action and regulation of ATP-dependent chromatin-remodelling complexes. Nat Rev Mol Cell Biol. 2017;18(7):407–422.
- Revenko AS, Kalashnikova EV, Gemo AT, Zou JX, Chen HW. Chromatin loading of E2f-Mll complex by cancer-associated coregulator ANCCA via reading a specific histone mark. Mol Cell Biol. 2010;30(22):5260–5272.
- Fu J, Zhang J, Chen X, Liu Z, Yang X, He Z, Hao Y, Liu B, Yao D. ATPase family AAA domain-containing protein 2 (ATAD2): from an epigenetic modulator to cancer therapeutic target. Theranostics. 2023;13(2):787–809.
- Duan Z, Andrews NP, Chen CZ, Fan M, Wang J, Shen J, Li JJ, Chen HW. Targeting bromodomain protein ANCCA/ATAD2 enhances the efficacy of DNA‑damaging chemotherapy agents and radiation. Oncol Rep. 2020;43(1):318–327.
- Boussouar F, Jamshidikia M, Morozumi Y, Rousseaux S, Khochbin S. Malignant genome reprogramming by ATAD2. Biochim Biophys Acta. 2013;1829(10):1010–1014.
- Baggiolini A, Callahan SJ, Montal E, Weiss JM, Trieu T, Tagore MM, Tischfield SE, Walsh RM, Suresh S, Fan Y, et al. Developmental chromatin programs determine oncogenic competence in melanoma. Science. 2021;373(6559):eabc1048.
- Liu Q, Liu H, Huang X, Fan X, Xiao Z, Yan R, Yao J, An G, Ge Y, Miao J, et al. A targetable MYBL2-ATAD2 axis governs cell proliferation in ovarian cancer. Cancer Gene Ther. 2023;30(1):192–208.
- Yao D, Zhang J, Wang J, Pan D, He Z. Discovery of novel ATAD2 bromodomain inhibitors that trigger apoptosis and autophagy in breast cells by structure-based virtual screening. J Enzyme Inhib Med Chem. 2020;35(1):713–725.
- Bamborough P, Chung CW, Demont EH, Bridges AM, Craggs PD, Dixon DP, Francis P, Furze RC, Grandi P, Jones EJ, et al. A qualified success: discovery of a new series of ATAD2 bromodomain inhibitors with a novel binding mode using high-throughput screening and hit qualification. J Med Chem. 2019;62(16):7506–7525.
- Lucas SCC, Atkinson SJ, Bamborough P, Barnett H, Chung CW, Gordon L, Mitchell DJ, Phillipou A, Prinjha RK, Sheppard RJ, et al. Optimization of potent ATAD2 and CECR2 bromodomain inhibitors with an atypical binding mode. J Med Chem. 2020;63(10):5212–5241.
- Bamborough P, Chung CW, Demont EH, Furze RC, Bannister AJ, Che KH, Diallo H, Douault C, Grandi P, Kouzarides T, et al. A chemical probe for the ATAD2 bromodomain. Angew Chem Int Ed Engl. 2016;55(38):11382–11386.
- Winter-Holt JJ, Bardelle C, Chiarparin E, Dale IL, Davey PRJ, Davies NL, Denz C, Fillery SM, Guerot CM, Han F, et al. Discovery of a potent and selective ATAD2 bromodomain inhibitor with antiproliferative activity in breast cancer models. J Med Chem. 2022;65(4):3306–3331.
- Fernandez-Montalvan AE, Berger M, Kuropka B, Koo SJ, Badock V, Weiske J, Puetter V, Holton SJ, Stockigt D, Ter Laak A, et al. Isoform-selective ATAD2 chemical probe with novel chemical structure and unusual mode of action. ACS Chem Biol. 2017;12(11):2730–2736.
- Murray BW, Guo C, Piraino J, Westwick JK, Zhang C, Lamerdin J, Dagostino E, Knighton D, Loi CM, Zager M, et al. Small-molecule p21-activated kinase inhibitor PF-3758309 is a potent inhibitor of oncogenic signaling and tumor growth. Proc Natl Acad Sci U S A. 2010;107(20):9446–9451.
- Zhang J, Zou L, Tang P, Pan D, He Z, Yao D. Design, synthesis and biological evaluation of 1H-pyrazolo [3,4-d]pyrimidine derivatives as PAK1 inhibitors that trigger apoptosis, er stress and anti-migration effect in MDA-MB-231 cells. Eur J Med Chem. 2020;194(:112220.
- Harner MJ, Chauder BA, Phan J, Fesik SW. Fragment-based screening of the bromodomain of ATAD2. J Med Chem. 2014;57(22):9687–9692.
- Dang CV. MYC on the path to cancer. Cell. 2012;149(1):22–35.
- Dhanasekaran R, Deutzmann A, Mahauad-Fernandez WD, Hansen AS, Gouw AM, Felsher DW. The Myc oncogene – the grand orchestrator of cancer growth and immune evasion. Nat Rev Clin Oncol. 2022;19(1):23–36.
- Zimmerli D, Brambillasca CS, Talens F, Bhin J, Linstra R, Romanens L, Bhattacharya A, Joosten SEP, Da Silva AM, Padrao N, et al. Myc promotes immune-suppression in triple-negative breast cancer via inhibition of interferon signaling. Nat Commun. 2022;13(1):6579.
- Wang W, Liao P, Shen M, Chen T, Chen Y, Li Y, Lin X, Ge X, Wang P. SCP1 regulates c-Myc stability and functions through dephosphorylating c-Myc Ser62. Oncogene. 2016;35(4):491–500.
- Ciro M, Prosperini E, Quarto M, Grazini U, Walfridsson J, McBlane F, Nucifero P, Pacchiana G, Capra M, Christensen J, et al. ATAD2 is a novel cofactor for Myc, overexpressed and amplified in aggressive tumors. Cancer Res. 2009;69(21):8491–8498.
- Fouret R, Laffaire J, Hofman P, Beau-Faller M, Mazieres J, Validire P, Girard P, Camilleri-Broet S, Vaylet F, Leroy-Ladurie F, et al. A comparative and integrative approach identifies ATPase family, AAA domain containing 2 as a likely driver of cell proliferation in lung adenocarcinoma. Clin Cancer Res. 2012;18(20):5606–5616.
- Wang AQ, Lv M, Xu YH, Xie PM, Dong YY. MiR-200b-5p inhibits proliferation of ovarian cancer cells by targeting ATAD2 and regulating PI3K/AKT signaling pathway. Eur Rev Med Pharmacol Sci. 2020; 24(19):9860–9868.
- Yi SA, Zhang Y, Rathnam C, Pongkulapa T, Lee KB. Bioengineering approaches for the advanced organoid research. Adv Mater. 2021;33(45):e2007949.
- Tummers B, Green DR. Caspase-8: regulating life and death. Immunol Rev. 2017;277(1):76–89.
- Case DA, Cheatham TE III, Darden T, Gohlke H, Luo R, Merz KM Jr, Onufriev A, Simmerling C, Wang B, Woods RJ. The amber biomolecular simulation programs. J Comput Chem. 2005;26(16):1668–1688.
- Yao D, Pan D, Zhen Y, Huang J, Wang J, Zhang J, He Z. Ferulin c triggers potent PAK1 and p21-mediated anti-tumor effects in breast cancer by inhibiting tubulin polymerization in vitro and in vivo. Pharmacol Res. 2020;152:104605.