Abstract
Persistent inflammation contributes to various inflammatory conditions. Inflammation-related diseases may be treated by inhibiting pro-inflammatory mediators and cytokines. Curcumin and coumarin derivatives can target signalling pathways and cellular factors to address immune-related and inflammatory ailments. This study involved designing and synthesising three series of coumarin-based analogs that incorporated curcumin and other heterocycles. These analogs were evaluated for their potential as anti-inflammatory agents in LPS-induced macrophages. Among the fourteen synthesised coumarin derivatives, compound 14b, which contained 3,4-dimethoxybenzylidene hydrazinyl, demonstrated the highest anti-inflammatory activity with an EC50 value of 5.32 μM. The anti-inflammatory effects of 14b were achieved by modulating signalling pathways like AKT/mTOR and Nrf2/HO-1, and downregulating NF-kβ, resulting in reduced production of pro-inflammatory cytokines such as IL-6, IL-1β, and TNF-α. The modelling studies revealed that 14b and dexamethasone bind to the same TNF-α pocket, suggesting that 14b has potential as a therapeutic agent superior to dexamethasone for TNF-α.
HIGHLIGHTS
Three series of curcumin-based analogs, incorporating other heterocycles, were synthesised with the intention of exploring their potential as anti-inflammatory agents.
Subsequently, these analogs underwent biological assessment in macrophages induced by LPS to determine their anti-inflammatory efficacy.
Among the fourteen coumarin derivatives synthesised, the most potent anti-inflammatory activity was observed in the coumarin compound 14b, which featured a 3,4-dimethoxybenzylidene hydrazinyl moiety, with an EC50 value of 5.32 μM.
The anti-inflammatory effects of compound 14b were achieved through the modulation of signalling pathways such as AKT/mTOR and Nrf2/HO-1, as well as the downregulation of NF-kβ, resulting in decreased production of pro-inflammatory cytokines including IL-6, IL-1β, and TNF-α.
Molecular modelling studies revealed that both compound 14b and dexamethasone bind to the same binding site on TNF-α, suggesting that 14b has the potential to serve as a therapeutic agent for TNF-α and other pro-inflammatory cytokines that surpasses that of dexamethasone.
1. Introduction
The process of inflammation is a multifaceted immune response to injuries and infections triggered by pathogens. Macrophages, fibroblasts, mast cells, and neutrophils are activated, in addition, macrophages play a crucial roleCitation1. Lipopolysaccharide (LPS), found in the cell wall of gram-negative bacteria, induces the release of different pro-inflammatory mediators and cytokines such as TNF-α, IL-1β, IL-1, IL-6, and IL-8. The purpose of inflammation is to eradicate infectious pathogens and facilitate the mending of injured tissues. If complete removal of the cause of acute inflammation is not possible, it has the potential to progress into chronic inflammation, persisting for extended periods ranging from weeks to yearsCitation2. Chronic inflammation is characterised by the continued and excessive production of inflammatory mediators and cytokines, which play a role in the development of various inflammatory diseases. Inhibiting these pro-inflammatory mediators and cytokines could be a viable therapeutic strategy for the treatment of conditions associated with inflammationCitation3.
Curcumin has the potential for treating inflammatory and immune-related diseases by influencing cellular targets and various signalling pathways. It can regulate transcription factors and protein kinases, which activate different pathways like PI3K/AKT/mTOR, Ras/Raf/MEK/ERK, and GSK-3β. Curcumin reduces cytokine production like IL-1, IL-6, IL-8, IL-1β, and TNF-α, released by pro-inflammatory cells. Additionally, it suppresses the activity of enzymes like LOX5, COX2, PGE2, and HO-1Citation4–6.
Coumarin and its derivatives, including umbelliferone, exhibit anti-inflammatory properties by hindering the activation of NF-κB, which consequently diminishes the discharge of pro-inflammatory mediators such as nitric oxide, prostaglandin E2, iNOS, COX-2, TNF-α, IL-4, and INF-γ, primarily when COX-2 is hinderedCitation7–13. Furthermore, it has been noted that adjusting umbelliferone at the C7 hydroxy location can intensify its anti-inflammatory effectCitation12,Citation13.
The hybrid structures-based technique has been successful in developing new drug candidatesCitation14–16, and in this context, curcumin analogues and umbelliferone derivatives were combined into a single molecule to enhance their anti-inflammatory properties (). In series A, a dimethoxyphenyl fragment was linked to substituted umbelliferone at C7 hydroxy via an acetohydrazide linker. In series B, bioisosteric replacement of the dimethoxyphenyl fragment with heterocyclic rings such as benzoxadiazole, indolone, and pyrazole was explored to study their anti-inflammatory response. Series C incorporated the bioisosteric pyrazole ring linked with an acetyl spacer. The 14 synthesised coumarin derivatives were evaluated for their cytotoxic activity on LPS-macrophages. The most active compounds were investigated for their anti-inflammatory effect against the production of proinflammatory cytokines (TNF-α, IL-6, IL-1β) as well as their effect on NF-kβ, AKT/mTOR and Nrf2/HO-1 signalling pathways in LPS-activated macrophages. Finally, molecular modelling was accomplished to elucidate the biological results obtained.
2. Results and discussion
2.1. Chemistry
The synthesis of the target molecules is depicted in Schemes 2 and 3, while Scheme 1 elicited the routes adopted for the synthesis of starting materialsCitation17–22. The chemical structures of the newly synthesised coumarin molecules were confirmed by elemental microanalyses and spectral data (IR, 1H NMR and 13C NMR). The synthesis of 7-hydroxy coumarin 1 by one pot three component reaction was reported in literatureCitation17. Alkylation has been performed by the reaction of 1 with ethyl chloroacetate in absolute ethanolCitation17. The synthesis of 7-coumarin acetohydrazide 3 was obtained through reacting coumarin ester derivative 2 with hydrazine hydrate in absolute ethanol. Heating of the acetohydrazide derivative 3 at reflux with appropriate chalcone in absolute ethanol in the presence of sodium hydroxide furnished the corresponding pyrazolinyl derivatives 5a–c. Refluxing of 3 with malononitrile in pure ethanol resulted in the formation of 3,5-diiminopyrazolidinyl derivative 6. Cyclocondensation of the hydrazinyl intermediate 3 with ethylcyanoacetate in pure ethanol furnished 5-iminopyrazolidinyl derivative 7. Moreover, the reaction of the key hydrazide intermediate with 3-methyl-1-phenyl-2-pyrazolin-5-one in dioxane gave the corresponding N-phenyl pyrazolyl derivative 9. Referring to Scheme 3, the key hydrazide material was allowed to react with 4-chloro-7-nitrobenzo[c][1,2,5]oxadiazole in absolute ethanol to produce 4-nitrobenzo oxadiazolyl molecule 10. Whereas, condensing the key hydrazinyl molecule with p-tosyl chloride in dioxane furnished the corresponding N,N’-ditosyl derivative 11. In addition, condensing the key hydrazide intermediate with isatin in absolute ethanol in the presence of catalytic amount of glacial acetic acid afforded 2-oxoindolin-3-ylidene derivative 12. Finally, the hydrazide key intermediate 3 was refluxed with appropriate aldehyde or ketone derivative in absolute ethanol in the presence of few drops of glacial acetic acid gave the desired hydrazone derivatives 13a,b and 14a,b.
Scheme 1. Synthetic pathways for preparation of starting materials 3 and 4a–c. Reagents: a) ethyl chloroacetate, anhydrous K2CO3, acetone, reflux 24 h; b) hydrazine hydrate, absolute ethanol, reflux 2 h; c) 30% NaOH, ethanol, stirring r.t., 2 h.
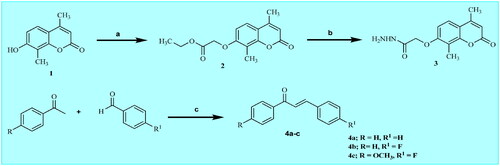
Scheme 2. Synthetic pathways for preparation of target coumarin derivatives 5a-c and 6–9. Reagents: d) chalcone 4a-c, NaOH, absolute ethanol, reflux 72 h; e) malononitrle, absolute, ethanol, reflux 8 h; f) ethylcyanoacetate, absolute ethanol, reflux 9 h; g) acetylacetone, absolute ethanol, reflux 15 h; h) 3-methyl-1-phenyl-2-pyrazoline-5-one, dioxane, reflux 12 h.
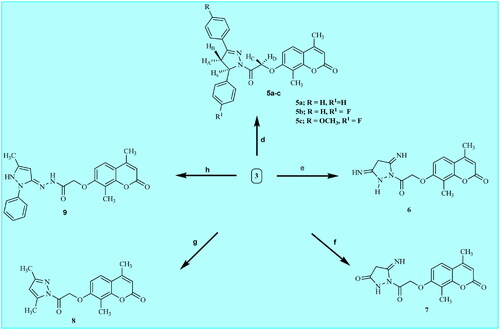
Scheme 3. Synthetic pathways for preparation of target coumarin derivatives 10–12, 13a,b and 14a,b. Reagents: i) 4-chloro-7-nitrobenzo[c][1,2,5]oxadiazole, absolute ethanol, reflux 8 h; j) tosyl chloride, dioxane, reflux 8 h; k) isatin, absolute ethanol, glacial acetic acid reflux 8 h. l) appropriate aryl aldehyde, absolute ethanol, glacial acetic acid, reflux 9–16 h; m) appropriate aryl ketone, absolute ethanol, glacial acetic acid reflux 10–16 h.
![Scheme 3. Synthetic pathways for preparation of target coumarin derivatives 10–12, 13a,b and 14a,b. Reagents: i) 4-chloro-7-nitrobenzo[c][1,2,5]oxadiazole, absolute ethanol, reflux 8 h; j) tosyl chloride, dioxane, reflux 8 h; k) isatin, absolute ethanol, glacial acetic acid reflux 8 h. l) appropriate aryl aldehyde, absolute ethanol, glacial acetic acid, reflux 9–16 h; m) appropriate aryl ketone, absolute ethanol, glacial acetic acid reflux 10–16 h.](/cms/asset/101c75c9-b3e7-4203-8dff-89429ce4653b/ienz_a_2243551_sch0003_c.jpg)
2.2. In vitro biological activity studies
2.2.1. Cytotoxicity of the synthesised coumarin derivatives on LPS-macrophages
In vitro cytotoxicity testing was conducted on all fourteen newly synthesised compounds. First, the compounds were tested on non-treated normal human macrophages, and it was observed that all compounds had low toxicity in normal cells, with IC50 values ranging from 16.37–130 µM. Next, the MTT assay was performed on LPS-Macrophage cells to identify the promising candidates for anti-inflammatory activity. shows that the coumarin derivative 14b had the most significant anti-inflammatory effect, with an EC50 value of 5.32 µM. Therefore, compound 14b was selected for further in vitro studies at its EC50 value to investigate the underlying mechanisms responsible for its impressive anti-inflammatory activity.
Table 1. The calculated EC50 and IC50 of the tested compounds on normal and LPS-treated Macrophages.
2.2.2. Effect of coumarin derivative 14b on the expression of pro-inflammatory cytokines and NF-kβ
Numerous research studies have showcased the remarkable in vivo and in vitro anti-inflammatory effects of umbelliferon. It has proven effective in treating acute lung injuryCitation23 and diabetic miceCitation24 by suppressing NF-κB, a key player in inflammation. These findings revealed that not only umbelliferon but also other coumarins can alleviate the expression of inflammatory markers like TNF-α, IL-1β, iNOS, IFN-γ, and IL-6 induced by LPS, thanks to their reliance on the NF-κB pathwayCitation25,Citation26. In parallel, curcumin and its derivatives have emerged as captivating anti-inflammatory agent due to their diverse range of cellular actions, including the regulation of transcription factors (NF-κB) and cytokine levelsCitation4,Citation6,Citation27,Citation28. Notably, administering curcumin at a dose of 100 mg/kg significantly reduced the infiltration of macrophages in the kidneys and the expression of pro-inflammatory cytokines like TNF-α and IL-1β in diabetic animals, concurrently inhibiting NF-κBCitation4,Citation29. Motivated by these findings, our objective was to explore the molecular mechanism underlying the inhibitory effects of a newly designed hybrid, a combination of coumarin and curcumin analogue 14b, on the expression of inflammatory cytokines and the activity of the NF-κB pathway. To achieve this, we employed the ELISA immuno-technique and dexamethasone used as reference compound.
The results in this regard are presented in , showcasing the remarkable impact of compound 14b, a coumarin molecule bearing 3,4-dimethoxybenzylidene hydrazinyl, on key inflammatory cytokines. Notably, 14b exhibited a significant reduction in IL-6 levels, reaching 1.41-fold less than negative control cells. Impressively, it surpassed the performance of the reference compound, Dexa, by a 1.2-fold reduction in IL-6 levels (). Furthermore, compound 14b demonstrated remarkable efficacy in decreasing IL-1β levels, surpassing both untreated macrophage cells and Dexa-treated cells, with reductions of 1.65-fold and 1.26-fold, respectively (). Additionally, the data revealed a noteworthy decrease in TNF-α levels, with 14b achieving reductions of 1.19-fold compared to untreated control cells and 1.14-fold compared to Dexa-treated macrophage cells ().
Figure 2. Represents the impact of coumarin molecule bearing 3,4-dimethoxybenzylidene hydrazinyl 14b compared to negative control and macrophage cells treated with Dexa on the levels of pro-inflammatory cytokines. (A) Effect on IL-6 level. (B) Effect on IL-1β level. (C) Effect on TNF-α level. (D) Effect on NF-kβ level.
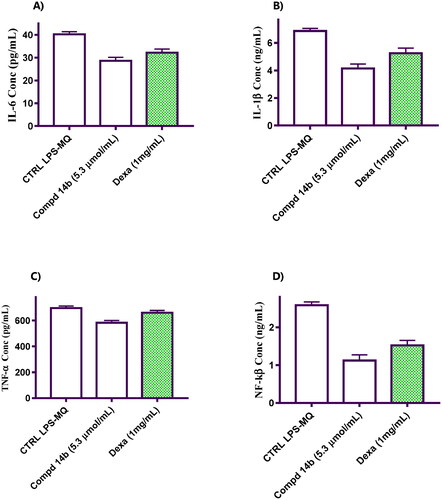
Moreover, the results highlighted the potency of compound 14b in modulating NF-kβ levels, exhibiting a significant reduction of 2.31-fold relative to untreated control cells. Notably, 14b displayed comparable efficacy to Dexa, a well-established reference compound ().
These findings underscore the impressive anti-inflammatory effects of compound 14b, as evidenced by its ability to effectively decrease IL-6, IL-1β, and TNF-α levels, while efficiently modulating NF-kβ levels. The superior performance of 14b compared to Dexa suggests its potential as a promising therapeutic candidate for addressing inflammatory conditions.
2.2.3. Measurement of protein expression “mTOR and Nrf2” in LPS-Human macrophages cells (inflammatory model) treated with EC50 (5.3 μmol/mL) of coumarin derivative 14b for 48 h
The intricate interplay between Nrf2 and NF-κB has been the subject of extensive investigation, yielding valuable insights into the realm of inflammation. Notably, multiple studies have elucidated the impact of Nrf2 on NF-κB activityCitation30,Citation31. Remarkably, the absence of Nrf2 has been observed to exacerbate NF-κB activity, culminating in heightened release of inflammatory cytokinesCitation30,Citation31. Conversely, upregulation of Nrf2 has exhibited the potential to mitigate inflammatory responses in rodent models of liver and kidney injuryCitation32,Citation33. These findings emphasise the pivotal role of Nrf2 as a crucial regulator of the inflammatory milieu, safeguarding against excessive inflammation and its detrimental consequences. Turning our attention to another fascinating aspect of cellular signalling—Akt (protein kinase B) and its effector, mTOR (mammalian target of rapamycin). Studies have established mTOR as a downstream target of Akt, playing a pivotal role in the regulation of NF-κB activityCitation34. This remarkable partnership between Akt and mTOR unveils a complex network of signalling cascades, contributing to the finely tuned orchestration of inflammatory processes.
In light of these remarkable revelations, our research investigates the expression levels of mTOR and Nrf2 in LPS-stimulated human macrophage cells. Through this meticulous exploration, our aim is to unravel the intricate molecular mechanisms that govern the dynamic interplay between these factors. In this study, it was observed that LPS macrophages exhibited a high expression level of mTOR protein in the untreated cells. However, upon treatment with 14b and dexamethasone, a noticeable decrease in the cytoplasmic and nuclear localisation of mTOR was observed (indicated by the pink colour and marked by white arrows in and ). Conversely, there was an increase in the expression of the Nrf2 protein in the treated cells as compared to the untreated cells (as depicted in and ).
2.2.4. Measurement of gene expression “HMOX1 and Akt” in LPS-Human macrophages cells (inflammatory model) treated with EC50 (5.3 µmol/mL) of coumarin derivative 14b for 48 h
Stunningly, the Nrf2 target gene HO-1 (heme oxygenase-1) has emerged as a compelling player in modulating NF-κB-mediated transcription of adhesion molecules. Notably, HO-1 achieves this regulatory feat by orchestrating a reduction in free intracellular iron levels within endothelial cellsCitation35,Citation36. This captivating mechanism sheds light on the intricate control mechanisms employed by HO-1, potentially offering novel therapeutic avenues for modulating adhesion molecule-dependent inflammatory processes. Excitingly, our investigation uncovered intriguing results regarding the effects of coumarin derivative 14b on LPS-human macrophage cells. Pre-treatment of these cells with an EC50 concentration of 14b (5.3 µmol/mL) demonstrated a remarkable anti-inflammatory activity. This was achieved through the induction of Nrf2 translocation into the nucleus, leading to a substantial upregulation of HO-1 expression by 6.5-fold compared to untreated macrophage cells (). Additionally, the pre-treatment with 14b resulted in a significant upregulation of Akt gene expression, exhibiting a 5.1-fold increase relative to the untreated macrophage cells ().
Figure 5. Represents the impact of coumarin molecule bearing 3,4-dimethoxybenzylidene hydrazinyl 14b compared to negative control and macrophage cells treated with Dexa on the gene expression levels of HO-1 and Akt in the tested groups.
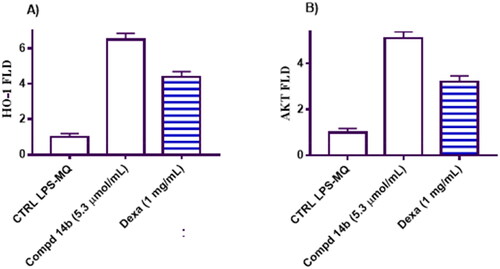
In comparison, Dexa, a known anti-inflammatory agent, also exhibited notable effects on the expression of HO-1 and Akt genes. Treatment with Dexa led to a 4.38-fold increase in HO-1 gene expression and a 3.2-fold increase in Akt gene expression.
These findings provide compelling evidence for the potential of coumarin derivative 14b as a potent anti-inflammatory compound, highlighting its ability to induce Nrf2-mediated HO-1 upregulation and activate the Akt pathway. The observed effects demonstrate the promising therapeutic prospects of 14b in modulating inflammatory responses, shedding light on new avenues for the development of effective anti-inflammatory interventions.
In summary, the extraordinary anti-inflammatory properties of compound 14b stem from its ability to orchestrate a series of intricate molecular events. By upregulating the Nrf2/HO-1 signalling pathway and concurrently downregulating the AKT/mTOR signalling pathway, 14b effectively modulates the activity of NF-κB, a key mediator of inflammation. This multifaceted approach culminates in a remarkable reduction in the production of pro-inflammatory cytokines, specifically IL-1β, IL-6, and TNF-α, as visually depicted in . These exciting outcomes highlight the promising future of 14b as a potential game-changer in the development of innovative anti-inflammatory interventions. Further exploration and validation of 14b’s anti-inflammatory properties may open doors to new treatment strategies for a wide range of inflammatory disorders.
2.3. Molecular modelling study
The objective of the modelling study was to assess the inhibitory effects of a coumarin-based compound, referred to as 14b, on TNF-α compared to Dexa (a reference compound), utilising in vitro inhibitory activity tests. To verify the accuracy of the docking process, the original co-crystallised ligand, 307, was redocked into the active binding site of TNF-α (PDB code: 2AZ5)Citation37. The docking outcomes demonstrated that the ligand fit snugly within the active pocket of TNF-α through interactions between the indole moiety and the crucial amino acid Tyr119. Moreover, the hydrophobic regions consisting of Leu57, Tyr59, Ser60, Tyr119, Leu120, Gly121, Gly122, and Tyr151 closely surrounded and shielded the trifluoromethylphenyl indole scaffold, while the trifluoromethyl group engaged in halogen (fluorine) interactions with Gly121.
presents the results of docking Compound 14b () in comparison to Dexa () and the native ligand 307. The study found that compound 14b had more potent inhibitory activity against TNF-α compared to Dexa, the reference drug. This increased potency could be attributed to the additional hydrophobic contacts that the coumarin scaffold formed with Tyr119 and Tyr151, in addition to the H- bond formed with Gly121 via the p-methoxy group and the H-bond with ser60. The findings of this study suggest that compound 14b has potential as a therapeutic agent for TNF-α related diseases.
Table 2. Binding score, H-bonding, and Hydrophobic interactions of the target compound 14b, Dexa and co-lig. 307 in the active site of TNF-α.
In summary, it was found that coumarin derivative 14b and Dexa bind to the same binding pocket on TNF-α and form similar μ-μ interactions with the amino acid Tyr119, which is also the binding mode of the native ligand 307. Furthermore, the inhibitory activity against TNF-α was improved for 14b by creating additional hydrogen bonds with the crucial amino acids Ser60 and Gly121 and increasing hydrophobic contacts with Tyr119 and Tyr151.
3. Conclusion
In this study, our designed hybrid compound, 14b, which combines coumarin and curcumin analogues, exhibited a highly desirable safety profile, demonstrating low toxicity in normal cells. Notably, 14b showcased remarkable anti-inflammatory activity, surpassing the effectiveness of dexamethasone, a well-known anti-inflammatory agent, with an impressive EC50 value of 5.32 µM. The anti-inflammatory effects of 14b were achieved through the modulation of crucial signalling pathways, including AKT/mTOR and Nrf2/HO-1, while downregulating NF-kβ activity. This multi-pronged approach resulted in a significant reduction in the production of pro-inflammatory cytokines such as IL-1β, IL-6, and TNF-α. Furthermore, molecular docking studies revealed a fascinating insight: both compound 14b and dexamethasone bind to the same pocket on TNF-α, suggesting that 14b holds immense potential as a therapeutic agent for targeting TNF-α, along with other pro-inflammatory cytokines like IL-1β and IL-6. These findings unveil the comprehensive mechanism through which compound 14b exerts its anti-inflammatory effects, offering valuable insights into the intricate interplay between key signalling pathways involved in inflammatory responses. The ability of 14b to precisely regulate the production of pro-inflammatory cytokines positions it as a promising candidate for the development of novel therapeutics aimed at mitigating inflammatory diseases and their associated complications.
4. Material and methods
4.1. Chemistry
4.1.1. General
Unless otherwise stated, solvents and reagents were obtained from Aldrich and used without further purification. Melting points were determined using the open capillary tube method on a Stuart SMP10 melting point apparatus and were reported without correction. Elemental analysis was performed using the Thermo Scientific TM FLASH 2000 CHNS/O analyser at the Regional Centre for Mycology and Biotechnology, Al-Azhar University, Egypt. Infra-red spectra were recorded as potassium bromide discs on a Bruker FT-IR spectrophotometer at MUST University and presented as wave numbers (υmax) in cm-1. 1H NMR spectra were obtained using Bruker 400 MHz & 300 MHz spectrophotometers with TMS as the internal standard, and chemical shifts (δ) were recorded in ppm on the δ scale at Ain Shams University and the Microanalytical Centre, Cairo University, Egypt. 13 C NMR spectra were acquired using a Bruker 100 MHz spectrophotometer with TMS as the internal standard, and chemical shifts (δ) were reported in ppm on the δ scale at Ain Shams University and the National Research Centre, Egypt. Mass spectra were generated using a Hewlett Packard 5988 spectrometer or Shimadzu QP-2010 plus at the Regional Centre for Mycology & Biotechnology, Al-Azhar University, Egypt. The progress of reactions was monitored by TLC using precoated aluminium sheets with silica gel (Merck 60 F 254) and a chloroform:methanol (9:1) eluting system. Visualisation was achieved using a UV lamp.
4.1.2. Procedure for synthesis of 7-hydroxy-4,8-dimethyl-2H-chromen-2-one (1) as reportedCitation17
A mixture of 2-methyl resorcinol (1.24 g, 10 mmol) and ethyl acetoacetate (1.30 mL, 10 mmol) was added dropwise to ice cooled sulphuric acid (15 mL), after complete, the mixture was stirred overnight at ambient temperature then poured over crushed ice, filtered, dried and recrystallized from ethanol to afford compound 1.
4.1.3. Procedure for synthesis of ethyl 2-((4,8-dimethyl-2-oxo-2H-chromen-7-yl)oxy)acetate (2) as reportedCitation18
An equimolar amounts of compound 1 and ethyl chloroacetate (10 mmol) was refluxed for 48 h in dry acetone (30 mL) using K2CO3 (5.51 g, 40 mmol), after completion of the reaction, it was poured over ice, the formed precipitate was filtered, dried to give the ester derivative 2.
4.1.4. Procedure for synthesis of 2-((4,8-dimethyl-2-oxo-2H-chromen-7-yl)oxy)acetohydrazide (3) as reportedCitation19
An equimolar amount of 2 and hydrazine hydrate (10 mmol) was refluxed in absolute ethanol (30 mL) for 4 h. The formed precipitate was filtered to give compound 3 to be used in the next step without purification.
4.1.5. Genaral procedure for synthesis of 1,3-diaryl-2-propen-1-ones (4a-c) as reportedCitation20–22
Equimolar amount of acetophenone and benzaldehyde derivatives (10 mmol) were dissolved in ethanol (10 mL) then 20% aqueous NaOH (10 mL) was added. The mixture was stirred for 12–18 h. The formed residue was filtered dried and recrystallized from ethanol.
4.1.6. General procedure for synthesis of compounds (5a–c)
A mixture of compound 3 (1.048 g, 4 mmol), appropriate chalcone derivative 4a–c (4 mmol) and sodium hydroxide (0.2 g, 5 mmol) in absolute ethanol (30 ml) was heated under reflux for 72 h. The reaction mixture was poured onto ice cooled water (15 ml) and neutralised with 2 N HCl then, the precipitate was filtered off, and recrystallized from DMF to give compounds 5a–c.
4.1.6.1. 7–(2-(3,5-Diphenyl-4,5-dihydro-1H-pyrazol-1-yl)-2-oxoethoxy)-4,8-dimethyl-2H-chromen-2-one (5a)
White powder, Yield 53%. mp 240 °C. IR υmax/cm−1: 3059 (CH aromatic), 2980, 2960 (CH aliphatic), 1732, 1720 (2 C = O), 1600 (C = N), 1558, 1498 (C = C). 1H NMR (400 MHz, DMSO-d6), δ ppm: 2.21 (s, 3H, CH3), 2.37 (s, 3H, CH3), 3.19 (dd, 1H, pyrazolyl-HA, JAX = 4.7 Hz, JAB = 18.3 Hz), 3.90 (dd, 1H, pyrazolyl-HB, JBX = 11.8 Hz, JAB =18.3 Hz) 5.33 (d, 1HC, OCH2 J = 16 Hz) 5.46 (d, 1HD, OCH2 J = 16 Hz), 5.59 (dd, 1H, pyrazolyl-HX, JAX = 4.7 Hz, JBX = 11.8 Hz), 6.23 (s, 1H, H-Ar), 6.98 (d, 1H, H-Ar, J = 9 Hz,), 7.24–7.27 (m, 3H, H-Ar), 7.32–7.36 (t, 2H, H-Ar), 7.48–7.53 (m, 4H, H-Ar), 7.84 (d, 2H, H-Ar, J = 7.4 Hz).13C NMR (100 MHz, DMSO-d6), δ ppm: 8.61, 18.63, 42.31, 60.44, 66.75, 108.97, 111.60, 113.25, 113.92, 123.66, 126.03 (2 C), 127.38, 127.87 (2 C), 129.18 (2 C), 129.27 (2 C), 131.14, 131.18, 142.25, 152.47, 153.96, 156.07, 159.34, 160.66, 165.03. MS m/z (%): 452.06 (M+, 18.34), 44.21 (100). Anal. Calcd. for C28H24N2O4 (452.51): C, 74.32; H, 5.35; N 6.19; Found: C, 74.20; H, 5.61; N, 6.38.
4.1.6.2. 7–(2-(5–(4-Fluorophenyl)-3-phenyl-4,5-dihydro-1H-pyrazol-1-yl)-2-oxoethoxy)-4,8-dimethyl-2H-chromen-2-one (5b)
Brown powder, Yield 50%. mp 208–210 °C. IR υmax/cm−1: 3099, 3076 (CH aromatic), 2964, 2927 (CH aliphatic), 1732, 1720 (2 C = O), 1604 (C = N), 1498, 1429 (C = C). 1H NMR (400 MHz, DMSO-d6), δ ppm: 2.21 (s, 3H, CH3), 2.38 (s, 3H, CH3), 3.22 (dd, 1H, pyrazolyl-HA, JAX = 4.7 Hz, JAB = 18.3 Hz), 3.90 (dd, 1H, pyrazolyl-HB, JBX = 11.8 Hz, JAB =18.3 Hz) 5.33 (d, 1HC, OCH2 J = 16 Hz) 5.45 (d, 1HD, OCH2 J = 16 Hz), 5.60 (dd, 1H, pyrazolyl-HX, JAX = 4.7 Hz, JBX = 11.8 Hz), 6.15 (s, 1H, H-Ar), 6.79 (d, 2H, H-Ar, J = 8.9 Hz), 6.98 (d, 1H, H-Ar, J = 8.9 Hz), 7.14–7.19 (t, 1H, H-Ar), 7.29–7.32 (m, 2H, H-Ar), 7.49–7.55 (m, 4H, H-Ar), 7.85 (d, 1H, H-Ar, J = 5.3 Hz). 13C NMR (100 MHz, DMSO-d6), δ ppm: 8.66, 18.63, 47.21, 57.40, 68.84, 109.05 (2 C), 110.75 (2 C), 112.61, 112.96, 113.28, 115.75, 116.07, 123.40 (2 C), 127.41 (2 C), 128.23, 129.29, 131.16, 144.44, 147.21, 152.44, 154.21, 156.15, 161.04, 170.59. MS m/z (%): 470.34 (M+, 6.34), 83.25 (100). Anal. Calcd. for C28H23FN2O4 (470.49): C, 71.48; H, 4.93; N 5.95; Found: C, 71.69; H, 5.09; N, 6.13.
4.1.6.3. 7–(2-(5–(4-Fluorophenyl)-3–(4-methoxyphenyl)-4,5-dihydro-1H-pyrazol-1-yl)-2-oxoethoxy)-4,8-dimethyl-2H-chromen-2-one (5c)
Brown powder, Yield 49%. mp 241–243 °C. IR υmax/cm−1: 3061, 3028 (CH aromatic), 2964, 2927 (CH aliphatic), 1730, 1720 (2 C = O), 1602 (C = N), 1558, 1498 (C = C). 1H NMR (400 MHz, DMSO-d6), δ ppm: 2.21 (s, 3H, CH3), 2.38 (s, 3H, CH3), 3.16 (dd, 1H, pyrazolyl-HA, JAX = 4.7 Hz, JAB = 18.3 Hz), 3.81 (s, 3H, OCH3), 3.87 (dd, 1H, pyrazolyl-HB, JBX = 11.8 Hz, JAB =18.3 Hz) 5.30 (d, 1HC, OCH2 J = 16 Hz) 5.42 (d, 1HD, OCH2 J = 16 Hz), 5.56 (dd, 1H, pyrazolyl-HX, JAX = 4.7 Hz, JBX = 11.8 Hz), 6.20 (s, 1H, H-Ar), 6.95 (d, 1H, H-Ar, J = 8.9 Hz), 7.03 (d, 2H, H-Ar, J = 8.8 Hz), 7.13–7.17 (t, 2H, H-Ar), 7.28 (dd, 2H, H-Ar, J = 8.5 Hz, 5.5 Hz), 7.53 (d, 1H, H-Ar, J = 8.9 Hz), 7.77 (d, 2H, H-Ar, J = 8.8 Hz). 13C NMR (100 MHz, DMSO-d6), δ ppm: 8.54, 18.59, 42.28, 55.81, 59.60, 66.69, 108.97, 111.45, 113.31, 114.71, 115.78 (2 C), 115.99, 123.49, 123.71, 128.06, 128.14, 129.17 (2 C), 138.38, 152.37, 154.35, 156.07, 158.38, 159.30, 160.59, 161.04, 164.89. MS m/z (%): 500.22 (M+, 12.81), 70.25 (100). Anal. Calcd. for C29H25FN2O5 (500.52): C, 69.59; H, 5.03; N 5.60; Found: C, 69.81; H, 5.20; N, 5.76.
4.1.7. Procedure for synthesis of 7–(2-(3,5-diiminopyrazolidin-1-yl)-2-oxoethoxy)-4,8-dimethyl-2H-chromen-2-one (6)
A mixture of compound 3 (1.048 g, 4 mmol), malononitrile (0.264 g, 4 mmol) in absolute ethanol (30 ml) was heated under reflux for 8 h. the reaction mixture was concentrated, and the residue was filtered off and recrystallized from isopropanol to afford compound (6).
Red powder, Yield 81%. mp 260–261 °C. IR υmax/cm−1: 3406, 3342, 3305 (3 NH), 3099, 3072, 3030 (CH aromatic), 2960 (CH aliphatic), 1708, 1674 (2 C = O), 1606 (C = N). 1H NMR (400 MHz, DMSO-d6), δ ppm: 2.21 (s, 3H, CH3), 2.38 (s, 3H, CH3), 2.73 (s, 1H, NH, D2O exchangeable), 2.88 (s, 1H, NH, D2O exchangeable), 4.36 (s, 2H, CH2), 4.64 (s, 2H, OCH2), 6.20 (s, 1H, H-Ar), 6.94 (d, 1H, J = 8.8 Hz, H-Ar), 7.56 (d, 1H, J = 8.8 Hz, H-Ar), 9.31 (s, 1H, NH, D2O exchangeable). 13C NMR (100 MHz, DMSO-d6), δ ppm: 8.67, 18.63, 36.25, 67.33, 108.67, 111.76, 113.53, 114.15, 123.83, 152.41, 153.95, 158.98, 160.61, 162.80, 166.76 (2 C). MS m/z (%): 328.96 (M+, 20.42), 95.40 (100). Anal. Calcd. for C16H16N4O4 (328.32): C, 58.53; H, 4.91; N 17.06; Found: C, 58.80; H, 5.13; N, 17.33.
4.1.8. Procedure for synthesis of 1–(2-(4,8-dimethyl-2-oxo-2H-chromen-7-yl)oxy)acetyl)-5-iminopyrazolidin-3-one (7)
A mixture of compound 3 (1.048 g, 4 mmol), ethyl cyanoacetate (0.452 g, 4 mmol) in absolute ethanol (30 ml) was refluxed for 9 h. The reaction mixture was concentrated, and the residue was filtered off and crystallised from isopropyl alcohol to afford compound 7.
White powder, Yield 45%. mp >300 °C. IR υmax/cm−1: 3429, 3184 (2 NH), 3057 (CH aromatic), 2958, 2935 (CH aliphatic), 1716, 1622, 1612 (3 C = O), 1575 (C = N). 1H NMR (400 MHz, DMSO-d6), δ ppm: 2.26 (s, 3H, CH3), 2.40 (s, 3H, CH3), 3.44 (s, 2H, CH2 pyrazolidinone), 4.36 (s, 1H, NH, D2O exchangeable), 4.77 (s, 2H, OCH2), 6.24 (s, 1H, H-Ar), 6.99 (d, 1H, J = 12 Hz, H-Ar), 7.59 (d, 1H, J = 12 Hz, H-Ar), 9.88 (s, 1H, NH, D2O exchangeable). 13C NMR (100 MHz, DMSO-d6), δ ppm: 18.66, 20.92, 67.05, 69.70, 108.94, 111.86, 113.45, 114.27, 123.84, 127.64, 152.45, 153.98, 158.95, 160.59, 166.65, 168.48. MS m/z (%): 329.75 (M+, 10.83), 162.24 (100). Anal. Calcd. for C16H15N3O5 (329.31): C, 58.36; H, 4.59; N 12.76; Found: C, 58.58; H, 4.77; N, 12.95.
4.1.9. Procedure for synthesis of 7–(2-(3,5-dimethyl-1H-pyrazol-1-yl)-2-oxoethoxy)-4,8-dimethyl-2H-chromen-2-one (8)
Acid hydrazide derivative 3 (2.62 g, 10 mmol) and acetyl acetone (10 mmol, 0.1 mL) in absolute ethanol (10 mL) was heated under reflux for 9 h. The formed precipitate was filtered, dried and crystallized from ethanol to give compound 8.
4.1.10. Procedure for synthesis of 2-((4,8-dimethyl-2-oxo-2H-chromen-7-yl)oxy)-N'-(5-methyl-2-phenyl-1,2-dihydro-3H-pyrazol-3-ylidene)acetohydrazide (9)
An equimolar amount of compound 3 (1.048 g, 4 mmol) and 3-methyl-1-phenyl-2-pyrazoline-5-one (0.696 g, 4 mmol) was heated under reflux for 12 h in dioxane (15 ml). The reaction mixture was concentrated, and the residue was filtered off and crystallised from ethanol to afford compound 9.
Red powder, Yield 67%. mp 244–246 °C. IR υmax/cm−1: 3444, 3342 (2 NH), 3057, 3030 (CH aromatic), 2983, 2924 (CH aliphatic), 1703, 1691 (2 C = O), 1666 (C = N). 1H NMR (400 MHz, DMSO-d6), δ ppm: 1.84 (s, 3H, CH3), 2.12 (s, 3H, CH3), 2.38 (s, 3H, CH3) 4.72 (s, 2H, OCH2), 5.37 (s, 1H, CH pyrazole), 6.96 (s, 1H, H-Ar), 7.02 (d, 1H, J = 12 Hz, 1H, H-Ar), 7.16–7.20 (m, 5H, H-Ar), 7.41 (d, 1H, J = 12 Hz, H-Ar), 9.69 (s, 1H, NH, D2O exchangeable), 9.86 (s, 1H, NH, D2O exchangeable). 13C NMR (100 MHz, DMSO-d6), δ ppm: 13.27, 14.19, 20.82, 66.58, 101.76, 112.81, 114.65, 118.71, 120.74, 125.48 (2 C), 126.52, 129.28 (2 C), 138.92, 147.24, 149.06, 153.15, 159.94, 161.75, 166.78, 168.96, 169.04. MS m/z (%): 418.14 (M+, 13.70), 57.60 (100). Anal. Calcd. for C23H22N4O4 (418.45): C, 66.02; H, 5.30; N 13.39; Found: C, 66.23; H, 5.36; N, 13.58.
4.1.11. Procedure for synthesis of 2–(4,8-dimethyl-2-oxo-2H-chromen-7-yloxy)-N'-(4-nitrobenzo[c][1,2,5]oxadiazol-7-yl)acetohydrazide (10)
An equimolar quantities of acid hydrazide 3 (1.048 g, 4 mmol) and 4-chloro-7-nitrobenzo[c][1,2,5]oxadiazole (0.79 g, 4 mmol) was heated under reflux in absolute ethanol (30 ml) for 8 h. then concentrated. The solid settled was filtered off and crystallised from isopropyl alcohol to obtain the desired product 10.
White powder, Yield 89%. mp 220–222 °C. IR υmax/cm−1: 3446, 3317 (2 NH), 3050, 3030 (CH aromatic), 2903 (CH aliphatic), 1705, 1680 (2 C = O), 1620, 1614 (C = N). 1H NMR (400 MHz, DMSO-d6), δ ppm: 2.10 (s, 3H, CH3), 2.36 (s, 3H, CH3), 4.91 (s, 2H, OCH2), 6.51 (d, 1H, J = 8.4 Hz, H-Ar), 7.05–7.08 (m, 2H, H-Ar), 7.78 (d, 1H, J = 10 Hz, H-Ar), 8.52 (d, 1H, J = 8.4 Hz, H-Ar), 10.28 (s, 1H, NH), 11.01 (s, 1H, NH). 13C NMR (100 MHz, DMSO-d6), δ ppm: 13.51, 15.25, 66.85, 101.82, 106.19, 112.66, 114.70, 118.77, 119.83, 126.61, 131.72, 137.44, 143.54, 144.97, 147.31, 153.10, 159.87, 161.91, 167.71. MS m/z (%): 425.40 (M+, 3.59), 161.18 (100). Anal. Calcd. for C19H15N5O7 (425.35): C, 53.65; H, 3.55; N 16.46; Found: C, 53.87; H, 3.69; N, 16.67.
4.1.12. Procedure for synthesis of 2–(4,8-dimethyl-2-oxo-2H-chromen-7-yloxy)-N,N'-ditosylacetohydrazide (11)
A mixture of compound 3 (1.048 g, 4 mmol), tosyl chloride (0.76 g, 4 mmol) in dioxane (15 ml) was heated under reflux for 8 h. The reaction mixture was concentrated, and the residue was filtered off and crystallised from DMF to afford compound 11.
White powder, Yield 80%. mp 140–142 °C. IR υmax/cm−1: 3444 (NH), 3120, 3078 (CH aromatic), 2983, 2926 (CH aliphatic), 1741, 1714 (2 C = O), 1606 (C = N), 1386, 1371 (SO2). 1H NMR (400 MHz, DMSO-d6), δ ppm: 2.24 (s, 3H, CH3), 2.26 (s, 3H, CH3), 2.39 (s, 3H, CH3), 2.54 (s, 3H, CH3), 4.96 (s, 2H, OCH2), 6.22 (s, 1H, H-Ar), 6.97 (d, 1H, J = 8 Hz, H-Ar), 7.13 (d, 4H, J = 8 Hz, H-Ar), 7.50 (d, 4H, J = 8 Hz, H-Ar), 7.57 (d, 1H, J = 8 Hz, H-Ar), 8.41 (s, 1H, NH, D2O exchangeable). 13C NMR (100 MHz, DMSO-d6), δ ppm: 8.47, 14.35, 15.93, 18.34, 61.18, 111.56, 113.13, 114.11, 123.75, 123.98, 124.20, 126.09 (4 C), 128.52 (4 C), 137.91 (2 C), 152.44, 152.65, 153.85, 158.60, 160.49, 168.71. MS m/z (%): 570.88 (M+, 15.89), 410.30 (100). Anal. Calcd. for C27H26N2O8S2 (570.11): C, 56.83; H, 4.59; N 4.91; Found: C, 56.83; H, 4.59; N, 4.59.
4.1.13. Procedure for synthesis of 2–(4,8-dimethyl-2-oxo-2H-chromen-7-yloxy)-N'-(2-oxoindolin-3-ylidene)acetohydrazide (12)
A mixture of compound 3 (1.048 g, 4 mmol) and isatin (0.59 g, 4 mmol) in absolute ethanol (30 ml) containing a few drops of glacial acetic acid was heated under reflux for 8 h. The reaction mixture was concentrated, and the residue was filtered off and crystallised from DMF to afford compound 12.
Red powder, Yield 79%. mp >300 °C. IR υmax/cm−1: 3400, 3350 (2 NH), 3084, 3066 (CH aromatic), 2980, 2933 (CH aliphatic), 1732, 1710, 1693 (3 C = O), 1608 (C = N). 1H NMR (400 MHz, DMSO-d6), δ ppm: 2.02 (s, 3H, CH3), 2.30 (s, 3H, CH3), 4.89 (s, 2H, OCH2), 6.89–7.08 (m, 4H, H-Ar), 7.36 (t, 1H, H-Ar), 7.52 (s, 1H, H-Ar), 7.65 (d, 1H, J = 8 Hz, H-Ar), 10.81 (s, 1H, NH, D2O exchangeable), 11.22 (s, 1H, NH, D2O exchangeable). 13C NMR (100 MHz, DMSO-d6), δ ppm: 13.46, 15.32, 67.65, 101.91, 111.24, 111.56, 112.62, 112.91, 121.20, 122.09, 123.07, 123.29, 126.62, 132.27, 143.03, 147.02, 152.98, 153.43, 161.40, 163.05, 168.93. MS m/z (%): 391.36 (M+, 12.21), 74.16 (100). Anal. Calcd. for C21H17N3O5 (391.12): C, 64.45; H, 4.38; N 10.74; Found: C, 64.78; H, 4.60; N, 11.02.
4.1.14. General procedure for synthesis of 2–(4,8-dimethyl-2-oxo-2H-chromen-7-yloxy)-N'-(4-substituted benzylidene)acetohydrazide 13a,b
An equimolar amount of compound 3 (1.048 g, 4 mmol) and appropriate aldehyde derivative (4 mmol), namely (N, N-dimethylamino benzaldehyde and 3,4-dimethoxy benzaldehyde) in absolute ethanol (30 ml) containing few drops of glacial acetic acid was heated under reflux for 9–16 h. The formed precipitate was filtered off and crystallised from DMF to afford compounds 13a,b.
4.1.14.1. 2–(4,8-Dimethyl-2-oxo-2H-chromen-7-yloxy)-N'-(4-(dimethylamino)benzylidene)acetohydrazide (13a)
Orange powder, Yield 63%. mp 262–263 °C. IR υmax/cm−1: 3444 (NH), 3099, 3080 (CH aromatic), 2981, 2924 (CH aliphatic), 1714, 1680 (2 C = O), 1602 (C = N). 1H NMR (400 MHz, DMSO-d6), δ ppm: 2.24 (s, 3H, CH3), 2.36 (s, 3H, CH3), 2.95 (s, 6H, 2-CH3), 4.78, (s, 2H, OCH2), 6.18 (s, 1H, H-Ar), 6.54–6.96 (m, 3H, H-Ar), 7.50–7.82 (m, 3H, H-Ar), 8.13 (s, 1H, HC = N), 11.35 (s, 1H, NH, D2O exchangeable). 13C NMR (100 MHz, DMSO-d6), δ ppm: 8.69, 18.41, 21.26 (2 C), 65.78, 108.74, 110.95, 111.64, 111.93 (2 C), 113.51, 121.64, 123.53, 128.66 (2 C), 145.21, 149.28, 151.76, 153.64, 159.59, 168.70, 172.47. MS m/z (%): 394.39 (M + 1, 11.33), 393.42 (M+, 24.71), 146.25 (100). Anal. Calcd. for C22H23N3O4 (393.44): C, 67.16; H, 5.89; N 10.68; Found: C, 67.42; H, 5.97; N, 10.91.
4.1.14.2. N'-(3,4-Dimethoxybenzylidene)-2–(4,8-dimethyl-2-oxo-2H-chromen-7-yloxy)acetohydrazide (13b)
White powder, Yield 45%. mp 253–255 °C. IR υmax/cm−1: 3444 (NH), 3088, 3010 (CH aromatic), 2958, 2929 (CH aliphatic), 1712, 1608 (2 C = O), 1583 (C = N). 1H NMR (400 MHz, DMSO-d6), δ ppm: 2.25 (s, 3H, CH3), 2.37 (s, 3H, CH3), 3.34 (s, 6H, 2-OCH3), 4.90 (s, 2H, OCH2), 6.25 (s, 1H, H-Ar), 6.98 (d, 1H, J = 8 Hz, H-Ar), 7.50–7.62 (m, 4H, H-Ar), 8.03 (s, 1H, HC = N), 10.30 (s, 1H, NH, D2O exchangeable). 13C NMR (100 MHz, DMSO-d6), δ ppm: 8.71, 18.41, 38.52 (2 C), 68.64, 108.79, 111.32, 113.50, 114.40, 116.00, 121.94 (2 C), 122.32, 131.95, 132.34 (2 C), 138.28, 152.73, 153.96, 159.00, 160.88, 166.81. MS m/z (%): 410.28 (M+, 12.76), 215.08 (100). Anal. Calcd. for C22H22N2O6 (410.42): C, 64.38; H, 5.40; N 6.83; Found: C, 64.95; H, 5.53; N, 6.98.
4.1.15. Gereral procedure for synthesis of 2–(4,8-dimethyl-2-oxo-2H-chromen-7-yloxy)-N'-(1-(substitutedphenyl)ethylidene)acetohydrazide 14a,b
A mixture of compound 3 (1.048 g, 4 mmol) and appropriate acetophenone derivative (4 mmol), namely p-amino acetophenone and 3,4-dimethoxy acetophenone in absolute ethanol containing few drops of glacial acetic acid (30 ml) was heated under reflux for 10–16 h. The formed precipitate was filtered off and crystallised from ethanol to afford 14a,b.
4.1.15.1. 2–(4,8-Dimethyl-2-oxo-2H-chromen-7-yloxy)-N'-(1–(4-aminophenyl)ethylidene)acetohydrazide (14a)
Yellow powder, Yield 45%. mp 290–292 °C. IR υmax/cm−1: 3460, 3358 (NH2), 3213 (NH), 3043 (CH aromatic), 2939 (CH aliphatic), 1701, 1672 (2 C = O), 1653 (C = N). 1H NMR (300 MHz, DMSO-d6), δ ppm: 2.17 (s, 3H, CH3), 2.26 (s, 3H, CH3), 2.37 (s, 3H, CH3), 4.87 (s, 1H, OCH2), 5.30 (s, 1H, OCH2), 5.44 (s, 2H, NH2), 6.19 (s, 1H, H-Ar), 6.57 (d, J = 8 Hz, 2H, H-Ar), 6.89–7.01 (dd, J = 8 Hz, 1H, H-Ar), 7.54–7.67 (m, 3H, H-Ar), 10.63 (s, 1H, NH). 13C NMR (100 MHz, DMSO-d6), δ ppm: 8.47, 18.41, 21.26, 65.78, 108.70, 110.95, 111.64 (2 C), 111.93, 113.51, 121.64, 123.53, 128.66 (2 C), 145.21, 149.28, 151.76, 153.64, 159.59, 168.70, 172.47. MS m/z (%): 379.73 (M+, 7.40), 76.46 (100). Anal. Calcd. for C21H21N3O4 (379.42): C, 66.48; H, 5.58; N, 11.08; Found: C, 55.60; H, 5.69; N, 11.26.
4.1.15.2. 2–(4,8-Dimethyl-2-oxo-2H-chromen-7-yloxy)-N'-(1–(3,4-dimethoxyphenyl)ethylidene)acetohydrazide (14b)
White powder, Yield 36%. mp 266–270 °C. IR υmax/cm−1: 3427 (NH), 3059 (CH aromatic), 2978 (CH aliphatic), 1712, 1680 (2 C = O), 1604 (C = N). 1H NMR (300 MHz, DMSO-d6), δ ppm: 2.24 (s, 3H, CH3), 2.26 (s, 3H, CH3), 2.31 (s, 3H, CH3), 3.28 (s, 3H, OCH3), 3.71 (s, 3H, OCH3), 5.36 (s, 2H, CH2), 6.21 (s, 1H, H-Ar), 6.82–6.87 (m, 2H, H-Ar), 7.36–7.42 (m, 2H, H-Ar), 7.56 (d, 1H, J = 8.7 Hz, H-Ar), 10.80 (s, 1H, NH, D2O exchangeable). MS m/z (%): 424.71 (M+, 19.84), 78.19 (100). Anal. Calcd. for C23H24N2O6 (424.45): C, 65.08; H, 5.70; N 6.60; Found: C, 65.21; H, 5.78; N, 6.81.
4.2. In vitro biological studies
Assessment of cell viability by cell proliferation assay (MTT), measurement of inflammatory cytokines “IL6, IL-1β, TNF-α, and Nrf2” levels, measurement of gene expression “Akt and HMOX1, and measurement of protein expression “Nrf2 and mTOR” in LPS-Human Macrophages cells (inflammatory model) were carried out as described in the supplementary dataCitation38–41.
4.3. Molecular modeling job
The 3D structure of the TNF-α complex (with PDB code: 2AZ5) was retrieved from the Protein Data Bank, and the heterodimer chains A and B containing the co-crystalized ligand 307 were chosen for modelling. To prepare the protein structure for docking simulations, the “Prepare Protein” tool in DS was used to add any missing atoms/chains and remove water molecules. Additionally, the "Prepare Protein" algorithm was utilised to add hydrogen ions to amino acid residues based on physiological conditions, ensuring the most accurate representation of the protein’s native state.To determine the binding site, a binding sphere was selected to cover the co-crystalized ligand. The active compounds were then docked into the TNF-α binding site using CDOCKERCitation42, a grid-based docking program, with default parameters. Finally, the most favourable pose of the docked compounds was identified based on their CDOCKER energy (-CDE) value. The figures were generated using PymolCitation43.
Supplemental Material
Download PDF (2 MB)Disclosure statement
The authors report no conflicts of interest.
Correction Statement
This article has been republished with minor changes. These changes do not impact the academic content of the article.
Additional information
Funding
References
- Newton K, Dixit VM. Signaling innate immunity and inflammation. Cold Spring Harb Perspect Biol. 2012;4:1–20.
- Sherwood ER, Toliver-Kinsky T. Mechanisms of the inflammatory response. Best Pract Res Clin Anaesthesiol. 2004;18(3):385–405.
- Prasad S, Aggarwal BB. Chronic diseases caused by chronic inflammation require chronic treatment: the anti-inflammatory role of dietary spices. J Clin Cell Immunol. 2014;05(04):1–11.
- Hassan F-U, Rehman MS-U, Khan MS, Ali MA, Javed A, Nawaz A, Yang C. Curcumin as an alternative epigenetic modulator: mechanism of action and potential effects. Front Genet. 2019;10:514.
- Rahban M, Habibi-Rezaei M, Mazaheri M, Saso L, Moosavi-Movahedi AA. Anti-viral potential and modulation of Nrf2 by curcumin: pharmacological implications. Antioxidants. 2020;9(12):1228.
- Wang H, Zhang K, Liu J, Yang J, Tian Y, Yang C, Li Y, Shao M, Su W, Song N. Curcumin regulates cancer progression: focus on ncRNAs and molecular signaling pathways. Front Oncol. 2021;11:660712.
- Hadjipavlou-Litina DJ, Litinas KE, Kontogiorgis C. The anti-inflammatory effect of coumarin and its derivatives. AIAAMC. 2007;6(4):293–306.
- Grover J, Jachak SM. Coumarins as privileged scaffold for anti-inflammatory drug development. RSC Adv. 2015;5(49):38892–38905.
- Rabe SZT, Iranshahi M, Mahmoudi M. In vitro anti-inflammatory and immunomodulatory properties of umbelliprenin and methyl galbanate. J Immunotoxicol. 2016;13(2):209–216.
- Yildirim M, Unal ZN, Ersatir M, Yetkin D, Degirmenci U, Giray ES. Anti-inflammatory effects of coumarin–selenophene derivatives on LPS-stimulated RAW 264.7 macrophage cells. Russ J Bioorg Chem. 2022;48(6):1209–1214.
- Revankar HM, Bukhari SNA, Kumar GB, Qin H-L. Coumarins scaffolds as COX inhibitors. Bioorg Chem. 2017;71:146–159.
- Park T, Park J-S, Sim JH, Kim S-Y. Curcumin ameliorates macrophage infiltration cytokine synthesis by IκBα degradation and MAPK activation in macrophage cells. Molecules. 2020;25:3124.
- Bansal Y, Sethi P, Bansal G. Coumarin: a potential nucleus for anti-inflammatory molecules. Med Chem Res. 2013;22(7):3049–3060.
- Wyman KA, Girgis AS, Surapaneni PS, Moore JM, Abo Shama NM, Mahmoud SH, Mostafa A, Barghash RF, Juan Z, Dobaria RD, et al. Synthesis of potential antiviral agents for SARS-CoV-2 using molecular hybridization approach. Molecules. 2022;27:5923.
- Morgan T, Andreas B, Chris d-G. Integrating structure-based approaches in generative molecular design. Curr Opin Struct Biol. 2023;79:102559.
- Alkhzem AH, Woodman TJ, Blagbrough IS. Design and synthesis of hybrid compounds as novel drugs and medicines. RSC Adv. 2022;12(30):19470–19484.
- Ihmaid SK, Aljuhani A, Alsehli M, Rezki N, Alawi A, Aldhafiri AJ, Salama SA, Ahmed HEA, Aouad MR. Discovery of triaromatic flexible agents bearing 1, 2, 3-triazole with selective and potent anti-breast cancer activity and CDK9 inhibition supported by molecular dynamics. J Mol Struct. 2022;1249:131568.
- Kawaguchi M, Sekimoto E, Ohta Y, Ieda N, Murakami T, Nakagawa H. Synthesis of fluorescent probes targeting tumor-suppressor protein FHIT and identification of apoptosis-inducing FHIT inhibitors. J Med Chem. 2021;64(13):9567–9576.
- Beshay BY, Abdellatef AA, Loksha YM, Fahmy SM, Habib NS, Bekhit AE-D, Georghiou PE, Hayakawa Y, Bekhit AA. Design and synthesis of 2-Substituted-4-benzyl-5-methylimidazoles as new potential anti-breast cancer agents to inhibit oncogenic STAT3 functions. Bioorg Chem. 2021;113:105033.
- Mellado M, González C, Mella J, Aguilar LF, Viña D, Uriarte E, Cuellar M, Matos MJ. Combined 3D-QSAR and docking analysis for the design and synthesis of chalcones as potent and selective monoamine oxidase B inhibitors. Bioorg Chem. 2021;108:104689.
- Tilekar K, Upadhyay N, Meyer-Almes F-J, Loiodice F, Anisimova NY, Spirina TS, Sokolova DV, Smirnova GB, Choe J-Y, Pokrovsky VS, et al. Synthesis and biological evaluation of pyrazoline and pyrrolidine‐2, 5‐dione hybrids as potential antitumor agents. Chem Med Chem. 2020;15(19):1813–1825.,.
- Kumar R, Sharma P, Shard A, Tewary DK, Nadda G, Sinha AK. Chalcones as promising pesticidal agents against diamondback moth (Plutella xylostella): microwave-assisted synthesis and structure–activity relationship. Med Chem Res. 2012;21(6):922–931.
- Wang D, Wang X, Tong W, Cui Y, Li X, Sun H. Umbelliferone alleviates lipopolysaccharide-induced inflammatory responses in acute lung injury by down regulating TLR4/MyD88/NF-κB signaling. Inflamm. 2019;42(2):440–448.
- Yin J, Wang H, Lu G. Umbelliferone alleviates hepatic injury in diabetic db/db mice via inhibiting inflammatory response and activating Nrf2-mediated antioxidant. Biosci Rep. 2018;38:BSR20180444.
- Kang J-K, Chung Y-C, Hyun C-G. Anti-inflammatory effects of 6-methylcoumarin in LPS-stimulated RAW 264.7 macrophages via regulation of MAPK and NF-κB signaling pathways. Mol. 2021;26(17):5351.
- Hassanein EHM, Sayed AM, Hussein OE, Mahmoud AM. Coumarins as modulators of the Keap1/Nrf2/ARE signaling pathway. Oxid Med Cell Longev. 2020;2020:1675957.
- Jamil SNH, Ali AH, Feroz SR, Lam SD, Agustar HK, Mohd Abd Razak MR, Latip J. Curcumin and its derivatives as potential antimalarial and anti-inflammatory agents: a review on structure–activity relationship and mechanism of action. Pharmaceuticals. 2023;16(4):609.
- Ni H, Jin W, Yuan B, Zhu T, Wang J, Jiang J, Liang W, Ma Z. Curcumin inhibits the increase of labile zinc and the expression of inflammatory cytokines after traumatic spinal cord injury in rats. J Surg Res. 2014;187(2):646–652.
- Soetikno V, Sari VR, Veeraveedu PT, Thandavarayan RA, Harima H, Sukumaran V. Curcumin ameliorates macrophage infiltration by inhibiting NF-kappa B activation and pro-inflammatory cytokines in streptozotocin induced-diabetic nephropathy. Nutr Metab. 2011; 8:35.
- Wardyn D, Ponsford AH, Sanderson CM. Dissecting molecular crosstalk between Nrf2 and NF-κB response pathways. Biochem Soc Trans. 2015;43(4):621–626.
- Gao W, Guo L, Yang Y, Wang Y, Xia S, Gong H, Zhang B-K, Yan M. Dissecting the crosstalk between Nrf2 and NF-κB response pathways in drug-induced toxicity. Front Cell Dev Biol. 2021;9:809952.
- ALHaithloul HAS, Alotaibi MF, Bin-Jumah M, Elgebaly H, Mahmoud AM. Olea europaea leaf extract up-regulates Nrf2/ARE/HO-1 signaling and attenuates cyclophosphamide-induced oxidative stress, inflammation and apoptosis in rat kidney. Biomed Pharmacother. 2019;111:676–685.
- Mahmoud AM, Hozayen WG, Ramadan SM. Berberine ameliorates methotrexate-induced liver injury by activating Nrf2/HO-1 pathway and PPARγ, and suppressing oxidative stress and apoptosis in rats. Biomed Pharmacother. 2017;94:280–291.
- Dan HC, Cooper MJ, Cogswell PC, Duncan JA, Ting JP, Baldwin AS. Akt-dependent regulation of NF-κB is controlled by mTOR and raptor in association with IKK. Genes Dev. 2008;22(11):1490–1500.
- Soares MP, Seldon MP, Gregoire IP, Vassilevskaia T, Berberat PO, Yu J, Tsui T-Y, Bach FH. Hemeoxygenase-1 modulates the expression of adhesion molecules associated with endothelial cell activation. J Immunol. 2004;172(6):3553–3563.
- Narasimhan KKS, Jayakumar D, Velusamy P, Srinivasan A, Mohan T, Ravi DB, Uthamaraman S, Sathyamoorthy YK, Rajasekaran NS, Periandavan K, et al. Morinda citrifolia and its active principle scopoletin mitigate protein aggregation and neuronal apoptosis through augmenting the DJ-1/Nrf2/ARE signaling pathway. Oxid Med Cell Longev. 2019;2019:2761041.
- Molly MH, et al. Small-Molecule Inhibition of TNF-α. Science. 2005;310:1022–1025.
- Human Interleukin 6 (IL-6) ELISA Kit Cat No. MBS261259, My BioSource, San Diego, USA. https://cdn.mybiosource.com/tds/protocol_manuals/000000-799999/MBS261259.pdf.
- Human Nuclear Factor Kappa B (NFkB) ELISA Kit Cat No. MBS2023291, My BioSource, San Diego, USA. https://cdn.mybiosource.com/tds/protocol_manuals/000000-799999/MBS450580.pdf.
- Human IL-1β (Interleukin 1 Beta) ELISA Kit Cat No.EH0185, Fine Biotech, Wuhan, Hubei, China. https://www.fn-test.com/content/uploads/product/manuals/elisa/EH0185.pdf.
- Human TNF-α (Tumor Necrosis Factor Alpha) ELISA Kit, Catalog No: E-EL-H0109, Elabscience Biotechnology, USA.
- BIOVIA, Dassault Systèmes, [Molecular modeling and simulation], [4.5], San Diego: Dassault Systèmes. 2019.
- Schrodinger L. The PyMOL molecular graphics system. Version 1.3r1. 2010.