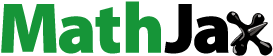
Abstract
The overexpression of polysialic acid (polySia) on neural cell adhesion molecules (NCAM) promotes hypersialylation, and thus benefits cancer cell migration and invasion. It has been proposed that the binding between the polysialyltransferase domain (PSTD) and CMP-Sia needs to be inhibited in order to block the effects of hypersialylation. In this study, CMP was confirmed to be a competitive inhibitor of polysialyltransferases (polySTs) in the presence of CMP-Sia and triSia (oligosialic acid trimer) based on the interactional features between molecules. The further NMR analysis suggested that polysialylation could be partially inhibited when CMP-Sia and polySia co-exist in solution. In addition, an unexpecting finding is that CMP-Sia plays a role in reducing the gathering extent of polySia chains on the PSTD, and may benefit for the inhibition of polysialylation. The findings in this study may provide new insight into the optimal design of the drug and inhibitor for cancer treatment.
Introduction
It has been known that NCAMs could be modulated by Polysialic acid (polySia), which is a distinctive glycan expressed on the surface of normal human neuronal cells. In mammalian cells, it is primarily linked posttranslationally to N-glycans on NCAM proteinsCitation1–9. These glycoproteins are also expressed on the surface of a number of human cancers where they may function as a metastatic factorCitation4,Citation10–12.
Peripheral adult organs express plySia-NCAM proteins less frequently than neural cells. However, some tumours of neural crest-origin, small cell lung cancers (SCLC), pancreatic cancers, neuroblastomas and malignant myelomas can re-express polySia-NCAM glycoproteins on their surface. This expression is often correlated with aggressive and invasive diseases with poor clinical outcomesCitation4,Citation11–13. Frequently overlooked, is that the ‘tumour associated’ polySia-NCAM proteins described above are the identical molecules that are of key importance in neurodevelopment/neurogenesis, neural plasticity, cognition, learning and memoryCitation13–15.
The synthesis of polySia can be catalysed by two polysialyltranseferases (polySTs), ST8Sia IV (PST) and ST8Sia II (STX)Citation7,Citation8,Citation10,Citation15. However, the overexpression of polySia on NCAM promotes hypersialylation, and benefits cancer cell migration and invasion. Several earlier studies in the area of polysialylation focused on identifying inhibitors of the polySTs to develop therapeutics to target metastatic tumours in humansCitation16. The studies by Paulson and colleaguesCitation17 showed that polysialylation of NCAM could be inhibited by cytidine monophosphate (CMP). After that, Falconer, R.A. et al. postulated that the polysialyltransferases were a ‘new target’ for metastatic cancerCitation18. In addition, it has also been known that CMP-sialic acid (CMP-Sia), the activated nucleotide sugar of N-acetylneuraminic acid (Neu5Ac; Sia)Citation19–25, is required by the polySTs for the biosynthesis of polySiaCitation9,Citation26–28. Accordingly, the synthesis of the polySia-NCAM protein may require the interactions of polyST-(CMP-Sia) and polyST-polySia in the lumen of the Golgi apparatusCitation13,Citation29–32.
In 2006, Nakata et al. identified that the polysialyltransferase domain (PSTD) is a polybasic motif of 32 amino acids in the polySTsCitation29. PSTD has been proposed to be a functional domain in polySTs, and is essential for polysialylation of NCAM proteins based on the vitro experimental data and molecular modelling analysisCitation33–37. The experimental results using Chinese human ovary cells suggested that the catalysis function of polySTs could be inhibited by CMP, and thus result in the decline of polySia expression on NCAMCitation38. However, the molecular mechanism of the inhibition is still unclear.
So far, both 3D X-ray and NMR structures of the polySTs have not yet been reported, due to the existence of many hydrophobic residues in the polySTs, which are in the membrane environmentCitation30,Citation33,Citation34. Recently, the 3-D solution structure of the PSTD peptide, an active site in the ST8Sia IV, has been obtained based on our NMR studiesCitation30,Citation36. Thus, a hypothesis about the interaction between the PSTD and the ligands may correspond to the interactions between the polyST and its ligands. This is an efficient research strategy and methodology for studying biological problems using biophysical and NMR structural biology, and the above hypothesis has been successfully tested by the recent NMR studiesCitation30–32.
More recent studies have shown that the NMR 3D structure of a synthesised 35-amino acid PSTD peptide is very similar to the predicted PSTD structure of the molecular modelCitation30,Citation36. Thus, this NMR-derived 3-D structural model not only verifies that the predicted model is quite accurate but also shows that this synthetic peptide should be appropriate for NMR study of the interactions between the PSTD and the other ligandsCitation36. Using this NMR-derived structural model of the PSTD, we have found that CMP-Sia was preferentially bound to the short helix H1, and the region between H1 and the long helix H2. On the other hand, polySia was mainly bound to the H2 helix of the PSTD. In addition, the peak intensities of 20 residues in H2 helix were significantly decreased after polySia bound to the PSTD. This finding suggests a slow chemical exchange and the formation of the aggregates might appear in the binding region of polySia in the PSTDCitation30,Citation36.
To further investigate the molecular mechanisms of pharmacological inhibition of polyST by CMP and derivatives, the interactions of the PSTD-CMP were carried out to determine the molecular details of CMP inhibiting NCAM polysialylation.
Materials and methods
Material sources
The PSTD (246K-277R) should be a 32 amino acid sequence peptide from ST8Sia IV molecule. However, in order to obtain more accurate 3D structural information by NMR spectroscopy, one amino acid (245 L) and two amino acids (278 P and 279S) from ST8Sia IV sequence were added into the N- and C- terminals of PSTD, respectivelyCitation30. Thus, a 35 amino acid sequence peptide sample containing PSTD was synthesised as follows: 245LKNKLKVRTAYPSLRLIHAVRGYWLTNKVPIKRPS279’. In which, the PSTD sequence is labelled by underline. This intact peptide sample was chemically synthesised by automated solid-phase synthesis using the F-MOC-protection strategy and purified by HPLC (DG Peptide Company, Hangzhou, China). The molecular weight of this peptide sample was 4117.95 and its purity was 99.36%.
CMP and CMP-Sia were purchased from Santa Cruz Biotechnology. The molecular weights of CMP and CMP-Sia, are 323.2, and 614.50 g/mol, respectively.
Circular dichroism (CD) Spectroscopy
The concentrations of the 35 amino acid-PSTD peptide and CMP in 20 mM phosphate buffer (pH 6.7) with 25% tetrafluoroethylene (TFE) were 8.0 uM and 400 uM, respectively. The measured and recorded methods of CD spectra are the same as the previous articlesCitation30,Citation37.
NMR Spectroscopy: Sample preparation
The 35 amino acid peptide containing the PSTD was prepared as described in referenceCitation37. Chemical shifts were referenced with respect to 2-dimethyl-2-silapentane-5-sulfonic acid (DSS) used as the internal standard. The ratio of CMP to the peptide was selected based on CD studies that indicated alterations in the secondary structure of the peptide in 25%TFE (v/v), 10% D2O (v/v), and 65% (v/v) 20 mM phosphate buffer (pH 6.7).
The concentrations of 35AA-PSTD peptide, CMP, CMP-Sia, triSiA (trimer of α-2,8-linked sialic acid DP3) and polySia (DP95) in the buffer were 2 mM, 1 mM, 1 mM, 1 mM, and 0.1 mM, respectivelyCitation30,Citation37 for all NMR experiments.
NMR spectroscopic Methods
The NMR spectroscopic methods are the same as the previous paperCitation37, and the chemical shift perturbation (CSP) of each amino acid in the PSTD was obtained using the formula:
(1)
(1)
where, DN and DNH represent the changes in Citation15N and Citation1H chemical shifts, respectively, upon ligand bindingCitation39.
Results
CD Data
As shown in , our CD spectra display that the main secondary structure of the PSTD was α-helical structure in the absence and presence of CMP ligand. The remaining half of the PSTD structure existed as a random-coil. These results are consistent with PSTD conformation in our 3-D derived ST8Sia IV molecular modelCitation30,Citation36,Citation40, and suggest the stability of the α-helical structure even in the presence of CMP.
NMR data
Using immunoblotting and NMR spectral analyses, several inhibitors of polySia-NCAM expression have been identifiedCitation41–43. For example, in 2013, Al-Saraireh et al. reported the pharmacological inhibition of ST8SiaII-mediated polysialylation of NCAM by CMP, and that this inhibition could modulate tumour cell migrationCitation41,Citation42,Citation44. This study demonstrated that CMP was a potential ‘druggable’ inhibitor of polysialylation, and could possibly interfere with the metastatic spread of selected polySia-NCAM positive cancers.
The PSTD-CMP interaction
In the present study, the binding of CMP to the PSTD in ST8Sia IV was studied by analysing 1H-15N-HSQC spectra of the PSTD in the absence and presence of CMP (). The binding region of CMP on the PSTD is in the residue range K246-L258, which is also CMP-Sia binding region in the PSTD (. Another CMP binding region in the PSTD is residue range V264-K276 () according to the results shown in , in which the CSP curves for the PSTD-(CMP-Sia) and the PSTD-polySia bindings are also shown in this figure.
Figure 2. The overlaid Citation1H-15N-HSQC NMR spectra of the PSTD of ST8Sia IV in the absence (black) and presence (red) of CMP (A), and CSPs of the PSTD for the 2 mM PSTD - 2 mM CMP-Sia binding (blue), the 2 mM PSTD-100uM polySia binding (red), and the PSTD-1 mM CMP biding (brown), respectively (B). The CSP values ofthe (CMP-Sia) - PSTD, and the polySia - PSTD interactions are taken from the referenceCitation30.

Figure 3. The major binding regions of CMP-Sia, polySia and CMP on the PSTD structural model based on the current and previous NMR dataCitation30,Citation36.

Comparison in the CSPs between the CMP binding region and the CMP-Sia binding region in the PSTD
As shown in , the largest CSPs for both the PSTD-(CMP-Sia) binding and for the PSTD-CMP binding are all focused on the residue range K246-L258, and almost all CSP values for the PSTD-CMP binding are larger than that for the PSTD-(CMP-Sia) binding in this residue range (). In addition, other CMP and CMP-Sia binding regions in the PSTD are residue ranges V264-K276 and V267-K276, respectively. The CSP values of most residues in the residue range V264-K276 for the PSTD-CMP binding are also larger than that for the PSTD-(CMP-Sia) binding. These results suggest that the PSTD-(CMP-Sia) binding could be inhibited by CMP.
On the other hand, there is only a polySia binding region, V260-N271, for the PSTD-polySia binding. The CSPs of all residues of the second CMP binding region (V264-K276) on the PSTD are less than that for the PSTD-polySia binding. For example, the CSPs in the PSTD from I263 to L269 for CMP-PSTD interaction are much less than that for polySia-PSTD interaction (the red curve in ). Therefore, the inhibition of the PSTD-polySia binding could not be inhibited by CMP.
Comparison in the CSPs between the PSTD-CMP interaction and the PSTD-polySia/CMP-Sia mixture interaction
In order to further analyse CMP’s inhibition effect on the polysialylation, the CSPs for the PSTD-CMP interaction were compared with the CSPs for the PSTD-polySia/CMP-Sia mixture interaction.
It has been proposed that the non-competitive binding occurs when CMP-Sia and polySia coexist in the solution systemCitation36. As shown in , CMP-Sia was mainly bound to the residual range from K246-L258, but polySia was bound to the H2 helix, and the significant change in chemical shift was found at four residues V264, Y267, W268, and L269Citation30,Citation36. As shown in , the CSPs of the PSTD for the PSTD-CMP interaction are significantly larger than that for the PSTD-polySia/CMP-Sia mixture interaction in the range K246-L258 (CMP-Sia binding region in the PSTD) (), but smaller than that in the range V260-N271 (polySia binding region in the PSTD) (). These results further suggest that the CMP-Sia binding region in the polySia/CMP-Sia mixture could be occupied by CMP only.
Figure 4. The comparison in CSPs of the PSTD for the PSTD - (CMP-Sia) binding (red), the PSTD- CMP biding (brown), and for the mixture of polySia and CMP-Sia (green) (A); The comparison in CSPs of the PSTD for the PSTD - polySia binding (red), the PSTD - CMP biding (brown), and for the mixture of polySia and CMP - Sia (green) (B). The CSP values of the all interactions are taken from referencesCitation30,Citation36.

Table 1. The major NMR features of the PSTD binding to different ligands or mixture (polySia, CMP-Sia, CMP, triSia, and the mixture of polySia and CMP-Sia) based on analysis of the overlaid 1H-15N-HSQC spectra and chemical shift perturbations of the PSTD residues.
In addition, two unexpecting findings are that the CSPs of the range I263-270 for the polySia/CMP-Sia mixture are larger than that for the PSTD-polySia binding, particularly at residues V264, R265, Y267, W268, and L269 (), and the peak intensities of only nine residues, S257, L258, R259, I261, R265, Y267, W268, L269, and N271 are decreased in the polySia/CMP-Sia mixture in . In contrast, 20 residues were found to be significantly decreased in peak intensity for the PSTD-polySia interactionCitation36 (). These results suggest that the existence of CMP-Sia may play a role in reducing the gathering of polySia chains on the PSTD.
Discussion
The importance of these NMR and CD studies is that they reveal for the first time the molecular mechanism underlying the basis for CMP inhibition of the polyST activity by its binding to the PSTD motif in ST8Sia IV thereby preventing polysialylation of NCAM proteins. The previous biophysical studies also establish that both CMP-Sia and polySia interact with the PSTD region within the polySTs, a motif that is an obligatory requirement for polysialylation of NCAM proteinsCitation30,Citation36.
According to the previous NMR studies, there are two binding regions in the PSTD for CMP-Sia interactionCitation36. One is in the residue range K246-L258, and another one is in the range V267-K276. In this study, there are also two CMP binding regions for the PSTD-CMP interaction. As shown in , the residue range of the first CMP binding region is also K246-L258, and the residue range of the second CMP binding region is the range V264-K276, which covers the second CMP-Sia binding region (V267-K276) (.
Our NMR studies show that amino acid residues within PSTD with the largest CSP values were in the range from V251 to T253, for the (CMP-Sia)-PSTD interaction. Obviously, the CSP values in the range K246-L258 and in H2 helix region for CMP-PSTD interaction are larger than that for (CMP-Sia)-PSTD interaction (. These suggest that (CMP-Sia)-PSTD binding could be inhibited by CMP.
On the other hand, the largest CSP values for polySia-PSTD binding were in H2 helix (R259-T270), particularly in residues V264, Y267, W268, and L269, which are consistent with the recent wenxiang diagram analysisCitation45. Because the CSP values are larger for polySia-PSTD interaction than that for the PSTD-CMP interaction, and suggest that the PSTD-polySia binding could not be inhibited by CMP.
It has been proposed that CMP-Sia in the Golgi apparatus must first interact with PSTD to initiate polysialylation of NCAM proteins. This step is required before the extended elongation of the polySia chains can take placeCitation3,Citation4,Citation7–11. Thus, the inhibitions of the PSTD-(CMP-Sia) interaction and the polySia chain formation in the early stage of the polysialylation could have occurred through CMP’s addition.
The previous NMR studies also showed that 20 residues in the PSTD displayed significant decrease in peak intensity after polySia was bound to the PSTD, suggested polySia long chain molecules have been aggregated on the 20 residues (), which are mostly located on the residual ranges K246-L258 and V260-N271Citation30. However, when CMP-Sia, polySia and the PSTD are co-existed in the solution systemCitation36, there are only 9 residues decreased in the peak intensity. These 9 residues are located in the residue range S257-N271 ( and . This implies that the aggregation is only occurred in the H2 helix region for the mixture of the PSTD, polySia, and CMP-Sia. Although CMP-Sia may play a role in reducing the gathering of polySia chain on the PSTD, the CSPs in the polySia binding region is still larger for the PSTD-polySia/CMP-Sia mixture interaction than that for the PSTD-polySia interaction only. Thus, our results again verified that the PSTD-(CMP-Sia) could be inhibited by CMP, which could not inhibit the PSTD-polySia binding even in mixture status of CMP-Sia, polySia, and the PSTD.
Using HPLC trace extract in vitro experiment, Al-Saraireh et al. showed that the polysialylation of the trimer of α-2,8-linked sialic acid (triSia) was inhibited by CMP in the presence of ST8SiaII and CMP-Neu5Ac (CMP-Sia)Citation42. This result is consistent with our data based on the previous NMR spectroscopyCitation36. As shown in , the CSP values of the PSTD for the PSTD-triSia interaction are much less than that for the PSTD-CMP interaction whether in the CMP-Sia binding sites or the polySia binding sites on the PSTD, and thus further confirmed that CMP should be a competitive inhibitor of ST8SiaII or ST8Sia IV. However, it should be noted that triSia (DP3, where DP refers to degree of polymerisation) belongs to oligosialic acid, and polySia used in our NMR studies is DP95. It has been designated that the DP3 ∼ 7 for oligoSia, and DP ≥8 for polySiaCitation46. In addition, α-2,8- and α-2,6-linked polySia exist as helical structures, which have large conformational differences compared with di- and oligoSia structuresCitation47. Thus, it’s possible that more residues in the H2 helix of the PSTD should be bound to polySia with the increase of polySia chain extension, and the aggregation tendency of polySia on the polyST will increase with degree of polymerisation. This is why CMP’s inhibition of polysialylation should be more efficient before formation of long chain polySia.
Conclusion
As reported herein, our NMR analysis suggested that the polysialylation could be inhibited in the presence of CMP-Sia or triSia, and confirmed that CMP is a competitive inhibitor of polySTs. But the NCAM polysialylation could be partially inhibited by CMP when polySia and CMP-Sia co-exist in solution. As a potential inhibitor, CMP may be useful in maintaining a proper balance between the neural system health and control of hypersalivation for the life process. The current studies would benefit from a stronger focus on CAMM (Computer Assisted Molecular Modelling) methodsCitation48,Citation49 for the novel drug design.
Disclosure statement
The authors report no conflicts of interest.
Additional information
Funding
References
- Li M, Wenig BM. Adrenal oncocytic pheochromocytoma. Am J Surg Pathol. 2000;24(11):1552–1557.
- Al Jishi AA, Lach B, Elgheriani A, Kachur E, Cenic A. Primary supratentorial intracerebral malignant paraganglioma. Neuroimmunol Neuroinflammation. 2015;2(2):121–126.
- Varki A, Cummings R, Esko JD, Freeze HH, Stanley P, Bertozzi CR, et al. 2008 Essentials of glycobiology. New York(NY):Cold Spring Harbor.
- Schnaar RL, Gerardy-Schahn R, Hildebrandt H. Sialic acids in the brain: gangliosides and polysialic acid in nervous system development, stability, disease, and regeneration. Physiol Rev. 2014;94(2):461–518.
- Finne J. Occurrence of unique polysialosyl carbohydrate units in glycoproteins of developing brain. J Biol Chem. 1982;257(20):11966–11970.
- Chen X, Varki A. ‘Advances in the biology and chemistry of sialic acids. ACS Chem Biol. 2010;5(2):163–176.
- Colley KJ, Kitajima K, Sato C. Polysialic acid: biosynthesis, novel functions and applications. Crit Rev Biochem Mol Biol. 2014;49(6):498–532.
- Harduin-Lepers A. Comprehensive analysis of sialyltransferases in vertebrate genomes. Glycobiol Insights. 2010;2:29–61.
- Troy FA. II, Polysialylation: from bacteria to brains. Glycobiology. 1992;2(1):5–23.
- Troy FA. II, Sialobiology and the polysialic acid glycotope: occurrence, structure, function, synthesis and glycopathology. In: Rosenberg. A, editor. Biology of the Sialic Acids. New York NY:Plennum Press; 1995. p. 95–144.
- Livingston BD, Jacobs JL, Glick MC, Troy IIFA. Extended polysialic acid chains (n greater than 55) in glycoproteins from human neuroblastoma cells. J.Biol. Chem. 1988;263(19):9443–9448. PMID 3288635.
- Lipinski M, Hirsch MR, Deagostini-Bazin H, Yamada O, Tursz T, Goridis C. Characterization of neural cell adhesion molecules (NCAM) expressed by Ewing and neuroblastoma cell lines. Int J Cancer. 1987;40(1):81–86.
- Troy FA. II, Polysialic acid in molecular medicine. J Biol Chem. 2004;3:407–414.
- Sevigny MB, Ye J, Kitazume-Kawaguchi S, Troy FA. II, Developmental expression and characterization of the α2,8-polysialyltransferase activity in embryonic chick brain. Glycobiology. 1998;8(9):857–867.
- Zhu X, Chen Y, Zhang N, Zheng Z, Zhao F, Liu N, Lv C, Troy FA, Wang B. Molecular characterization and expression analyses of ST8Sia II and IV in piglets during postnatal development: lack of correlation between transcription and posttranslational levels. Glycoconj J. 2015;32(9):715–728.
- Rodriguez L. In vitro synthesis of colominic acid by membrane-bound sialyltransferase of Escherichia coli K-235. Kinetic properties of this enzyme and inhibition by CMP and other cytidine nucleotides. Eur J Biochem. 1989;178:741–749.
- Datta AK, Paulson JC. Carbohydrates, lipids, and other natural products. The sialyltransferase ‘Sialylmotif’ participates in binding the donor substrate CMP-NeuAc. J Biol Chem. 1995;270(4):1497–1500.
- Falconer RA, Errington RJ, Snyder SD, Smith PJ, Patterson LH. Polysialyltransferase: a new target in metastatic cancer. Curr Cancer Drug Targets. 2012;12:925–939.
- Kauskot A, Pascreau T, Adam F, Bruneel A, Reperant C, Lourenco-Rodrigues M-D, Rosa J-P, Petermann R, Maurey H, Auditeau C, et al. A mutation in the gene coding for the sialic acid transporter SLC35A1 is required for platelet life span but not proplatelet formation. Haematologica. 2018;103(12):e613–e617.
- Parker JL, Newstead S. Gateway to the golgi:molecular mechanisms of nucleotide sugar transporters. Curr Opin Struct Biol. 2019;57:127–134.
- Parker JL, Newstead S. Structural basis of nucleotide sugar transport across the golgi membrane. Nature. 2017;551(7681):521–524.
- Eckhardt M, Gotza B, Gerardy-Schahn R. Mutants of the CMP-sialic acid transporter causing the Lec2 phenotype. J Biol Chem. 1998;273(32):20189–20195.
- Aoki K, Ishida N, Kawakita M. Substrate recognition by nucleotide sugar transporters: further characterization of substrate recognition regions by analyses of UDP-galactose/CMP-sialic acid transporter chimeras and biochemical analysis of the substrate specificity of parental and chimeric transporters. J Biol Chem. 2003;278(25):22887–22893.
- Aoki K, Ishida N, Kawakita M. Substrate recognition by UDP- galactose and CMP-sialic acid transporters. Different sets of transmembrane helices are utilized for the specific recognition of UDP-galactose and CMP-sialic acid. J Biol Chem. 2001;276(24):21555–21561.
- Angata K, Suzuki M, McAuliffe J, Ding Y, Hindsgaul O, Fukuda M. Differential biosynthesis of polysialic acid on neural cell adhesion molecule (NCAM) and oligosaccharide acceptors by three distinct alpha 2,8-sialyltransferases, ST8Sia IV (PST), ST8Sia II (STX), and ST8Sia III. J Biol Chem. 2000;275(24):18594–18601.
- Rosenberg A. The Beginnings of Sialic Acid. Biology of the Sialic Acids; Berlin, Germany:Springer; 1995; p. 1–5.
- Varki A, Cummings RD, Esko JD, Freeze HH, Stanley P, Bertozzi CR, Hart GW, Etzler ME. Essentials of Glycobiology, 2nd ed. NY, USA: Cold Spring Harbor Laboratory Press: Cold Spring Harbor, 2009. p. 199–216.
- Drake P, Nathan J, Stock C, Chang P, Muench M, Nakata D, Reader R, Gip P, Golden K, Weinhold B, et al. The highly restricted glycan, polysialic acid, is differentially expressed on human and murine leukocytes and modulated immune response. J Immunol. 2008;181(10):6850–6858.
- Nakata D, Zhang L, Troy FA. II, Molecular basis for polysialylation: a nove polybasic polysialyltransferase domain (PSTD) of 32 amino acids unique to the α2,8-polysialyltransferases is essential for polysialylation. Glycoconj J. 2006;23(5-6):423–436.
- Liao SM, Lu B, Liu XH, et al. Molecular interactions of the polysialyltransferase domain (PSTD) in ST8Sia IV with CMP-Sialic acid and polysialic acid required for polysialylation of the neural cell adhesion molecule proteins: an NMR study. Int J Mol Sci. 2020;21(5):1590.
- Zhou GP, Troy FA. II. NMR study of the preferred membrane orientation of polyisoprenols (dolichol) and the impact of their complex with polyisoprenyl recognition sequence peptides on membrane structure. Glycobiology. 2005;15(4):347–359.
- Zhou GP, Troy FA. II. NMR studies on how the binding complex of polyisoprenol recognition sequence peptides and polyisoprenols can modulate membrane structure. Curr Protein Pept Sci. 2005;6(5):399–411.
- Zhou GP, Huang RB, Troy FA. II. 3D structural conformation and functional domains of polysialyltransferase ST8Sia IV required for polysialylation of neural cell adhesion molecules. Protein Pept Lett. 2015;22(2):137–148.
- Huang RB, Chen D, Liao SM, Lu B, Wang QY, Xie NZ, Troy FA, Ii; Zhou GP. The intrinsic relationship between structure and function of the sialyltransferase ST8Sia family members. Curr. Top. Med. Chem. 2017;17:2465–2475.
- Zhou GP, Liao SM, Chen D, Huang RB. The cooperative effect between polybasic region (PBR) and polysialyltransferase domain (PSTD) within tumor-target polysialyltransferase ST8SiaII. Curr Top Med Chem. 2019;19(31):2831–2841.
- Lu B, Liu X-H, Liao S-M, Lu Z-L, Chen D, Troy Ii FA, Huang R-B, Zhou G-P. A possible modulation mechanism of intramolecular and intermolecular interactions for NCAM polysialylation and cell migration. Curr Top Med Chem. 2019;19(25):2271–2282.
- Peng LX, Liu XH, Lu B, Liao SM, Huang JM, Zhou F, Chen D, Troy FA, II, Huang RB, Zhou GP. The inhibition of polysialyltransferase ST8SiaIV through heparin binding to polysialyltransferase domain (PSTD). MC. 2019;15(5):486–495.
- Miyazaki T, Kiyohiko Angata K, Seeberger PH, Hindsgaul O, Fukuda M. CMP substitutions preferentially inhibit polysialic acid synthesis. Glycobiology. 2008;18(2):187–194.
- Vaynberg J, Qin J. Weak protein–protein interactions as probed by NMR spectroscopy. Trends Biotechnol. 2006;24(1):22–27.
- Bhide GP, Prehna G, Ramirez BE, Colley KJ. The polybasic region of the polysialyltransferase ST8Sia-IV binds directly to the neural cell adhesion molecule, NCAM. Biochemistry. 2017;56(10):1504–1517.
- Liu XH, Lu B, Peng LX, Liao SM, Zhou F, Chen D, Lu ZL, Zhou GP, Huang RB. The NMR structure of the polysialyltranseferase domain (PSTD) in polysialyltransferase. https://doi.org/10.2210/pdb6AHZ/pdb
- Al-Saraireh YMJ, Sutherland M, Springett BR, Freiberger F, Ribeiro Morais G, Loadman PM, Errington RJ, Smith PJ, Fukuda M, Gerardy-Schahn R, et al. Pharmacological inhibition of polysialyltransferase ST8SiaII modulates tumour cell migration. PloS One. 2013;8(8):8e73366.
- Wang L, Liu Y, Wu L, Sun XL. Sialyltransferase inhibition and recent advances. Biochim Biophys Acta. 2016;1864(1):143–153.
- Pietanza MC, Byers LA, Minna JD, Rudin CM. Small cell lung cancer: will recent progress lead to improved outcomes? Clin Cancer Res. 2015;21(10):2244-he ex–2255.
- Zhou GP, Huang RB. The graphical studies of the major molecular interactions for neural cell adhesion molecule (NCAM) polysialylation by incorporating Wenxiang Diagram into NMR spectroscopy. Int J Mol Sci. 2022;23(23):15128.
- Sato C, Kitajima K. Disialic, oligosialic and polysialic acids: distribution, functions and related disease. J Biochem. 2013;154(2):115–136.
- Mühlenhoff M, Eckhardt M, Gerardy-Schahn R. Polysialic acid: three-dimensional structure, biosynthesis and function. Curr Opin Struct Biol. 1998;8(5):558–564.
- Schauer R, Kamerling JP. Exploration of the sialic acid world. In: Baker DC, editor. Advances in carbohydrate chemistry and biochemistry. Philadelphia (PA): Elsevier; 2018, p. 1–213.
- Zhang R, Loers G, Schachner M, Boelens R, Wienk H, Siebert S, Eckert T, Kraan S, Rojas-Macias MA, LüTteke T, et al. Molecular basis of the receptor interactions of polysialic acid (polySia), polySia mimetics and sulphated polysaccharides. ChemMedChem. 2016;11(9):990–1002.