Abstract
A series of pleuromutilin analogs containing substituted benzoxazole were designed, synthesised, and assessed for their antibacterial activity both in vivo and in vitro. The MIC of the synthesised derivatives was initially assessed using the broth dilution method against four strains of Staphylococcus aureus (MRSA ATCC 43300, S. aureus ATCC 29213, clinical isolation of S. aureus AD3 and S. aureus 144). Most of the synthesised derivatives displayed prominent in vitro activity (MIC ≤ 0.5 µg/mL). Compounds 50 and 57 exhibited the most effective antibacterial effect against MRSA (MIC = 0.125 µg/mL). Furthermore, the time-kill curves showed that compounds 50 and 57 had a certain inhibitory effect against MRSA in vitro. The in vivo antibacterial activity of compound 50 was evaluated further using a murine thigh model infected with MRSA (–1.24 log10CFU/mL). Compound 50 exhibited superior antibacterial efficacy to tiamulin. It was also found that compound 50 did not display significant inhibitory effect on the proliferation of RAW 264.7 cells. Molecular docking study revealed that compound 50 can effectively bind to the active site of the 50S ribosome (the binding free energy −7.50 kcal/mol).
Introduction
Staphylococcus aureus (S. aureus) is an important zoonotic bacterium. S. aureus can cause infections in skin and soft tissue and can even lead to a variety of zoonotic diseases such as mastitis, bacteraemia, and infective endocarditisCitation1. Penicillin and methicillin had been used to control S. aureus infections. In 1958, two years after the clinical used of methicillin, the first case of methicillin-resistant S. aureus (MRSA) infection was reportedCitation2. Due to its inherent resistance, MRSA could not be effectively treated with methicillin or other beta-lactam drugsCitation3. With the massive use of antibiotics in clinical practice, MRSA has evolved into a "superbug" that poses a significant risk to human and animal safety and economic lossesCitation4,Citation5. Thus, it is urgent to develop antibiotics with unique antibacterial mechanisms to combat MRSA infections.
Pleuromutilin (1, ) is a tricyclic diterpene natural product, which was first isolated from cultures of two basidiomycetes Pleurotus mutiliz and Pleurotus PasseckerianusCitation6. Pleuromutilin displayed potent antibacterial activity against many Gram-positive bacteria and MycoplasmaCitation7,Citation8. Pleuromutilin derivatives selectively inhibit bacterial protein synthesis by interacting with the V domain of the peptidyl transferase centre (PTC) of the 50S ribosome subunitCitation9. Due to the unique antibacterial mechanism, pleuromutilin and its derivatives rarely exhibit cross-resistance with other clinically used antibioticsCitation10. The structural modifications on the C14 side chain of pleuromutilin has prompted the discovery of tiamulin (2, ) and valnemulin (3, ), which were approved as veterinary medicines in 1979 and 1999, respectivelyCitation11. Retapamulin (4, ) was the first pleuromutilin derivative antibiotic for the treatment of topical skin infection in humans, which was approved by the Food and Drug Administration (FDA), in 2007Citation12. Lefamulin (5, ) is the first approved systemic pleuromutilin antibiotic for the treatment of community-acquired bacterial pneumonia (CABP)Citation13. The success of these antibiotics has encouraged researchers to focus on developing new thymidine antibiotics. Thus, pleuromutilin has attracted considerable attention of researchers as a novel and effective antibacterial agent.
Figure 1. Structures of pleuromutilin (1), tiamulin (2), valnemulin (3), retapamulin (4), lefamulin (5), general structure (45).
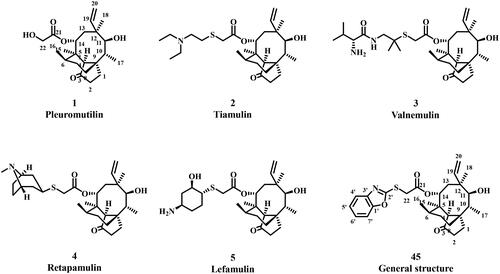
In our previous research, we successfully designed and synthesised a range of pleuromutilin derivatives that demonstrated potent antimicrobial activity against MRSACitation14–Citation16. One promising class of compounds in synthetic chemistry is benzoheterocycles containing mercaptans, which exhibit a wide variety of biological and pharmacological activitiesCitation17–19. Benzoxazole, a key component in the synthesis of drug molecules, has been identified for its desirable pharmacological propertiesCitation20–22. In recent years, significant progress has been made in the development of benzoxazole derivatives into clinical drug candidatesCitation23. The benzoxazole ring, a structural isomer of adenine and guanine bases, is an important heterocyclic group in the structure of nucleic acids. It is believed that derivatives carrying this ring system may exert their antimicrobial activity by inhibiting nucleic acid synthesisCitation24. Therefore, we propose that incorporating a benzoxazole moiety onto the C14 side chain of thymidine may result in the development of new derivatives that exhibit superior antibacterial activity compared to the prototype pleuromutilin.
In this study, we designed and synthesised 19 pleuromutilin derivatives with a benzoxazole-2-thiol structure. We also conducted preliminary evaluations of their antibacterial activity against MRSA, both in vitro and in vivo.
Results and discussion
Chemistry
The general synthetic course was displayed in Scheme 1. All pleuromutilin derivatives were prepared by 22-O-tosylpleuromutilin (compound 6), which was firstly synthesised by the reaction between pleuromutilin and p-toluenesulfonyl according to previous literatureCitation25. Compounds 7–25 were converted into benzoxazole-2-thiol derivatives (compounds 26–44) through a cyclisation reaction with CS2 under basic conditions, respectivelyCitation26. And compounds 26–44 were generated in good to exceptional yields under simple workup and purification conditions. Compound 6 and compounds 26–44 were transformed by nucleophilic substitution reaction to produce the target pleuromutilin derivatives (compounds 45–63).
Scheme 1. Reagent and conditions: (i) acetonitrile, p-toluenesulfonyl chloride, NaOH, rt, 3 h; (ii)EtOH, CS2, KOH, 60 °C, 3 h; (iii) acetonitrile, thiol derivative, K2CO3, 70 °C, 3 h.
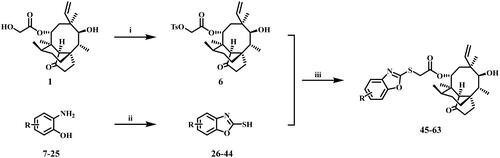
All the crude products were purified using silica column chromatography to get the target pleuromutilin derivatives. The structures of 19 compounds were characterised by 1H NMR, 13C NMR, HR-MS (ESI) analysis, and the results showed that the compounds were synthesised in the expected structure.
In vitro antibacterial activity
The MIC and MBC values of new synthesised pleuromutilin derivatives containing benzoxazole-2-thiol were evaluated against MRSA (ATCC 43300), S. aureus (ATCC 29213), S. aureus (AD3) and S. aureus (144) in accordance with the terms of the Clinical and Laboratory Standards Institute (CLSI)Citation27. The results of the minimum inhibitory concentration (MIC) and the minimum bactericidal concentration (MBC) were summarised in .
Table 1. MIC and MBC (μg/mL) values of compounds 45-63, tiamulin, valnemulin and vancomycin against MRSA ATCC 43300, MSSA ATCC 29213, S. aureus AD3 and S. aureus 144.
As shown in , the majority of the new pleuromutilin derivatives exhibited potent antibacterial activity against MRSA (ATCC 43300) and S. aureus (ATCC 29213), S. aureus (AD3) and S. aureus (144). Moreover, 14 compounds demonstrated superior MIC values to tiamulin, while four compounds exhibited an antibacterial activity of 0.125 μg/mL against MRSA.
In addition, we investigated the preliminary structure-activity relationships (SARs) by exploring 19 pleuromutilin derivatives. These derivatives have different substituent structures of benzoxazole-2-thiol, including both electron-donating groups (such as methyl, alkoxy, etc.) and electron-withdrawing groups (such as fluorine, chlorine, trifluoromethyl, etc.). Compound 45, which has no substituent, serves as a natural baseline for comparison and discussion of the simple functionalization incorporated in other target compounds.
Antibacterial activity data showed that modifications made to benzoxazoles led to compounds with varying degrees of decreased or maintained activity. Based on this, we speculated that the spatial effects of benzoxazole were the main factor influencing the activity of these compounds. Specifically, we found that 6-position and 7-position of the benzoxazole were sensitive to modifications. Compounds containing chlorine atom or methyl formate group resulted in a significant reduction in activity upon introduction of a modification group (compounds 55–56 and 59). However, modifications at the 5-position were tolerated, with the addition of a modification group only slightly reducing activity (compounds 54, 58 and 60). We also found that the electron effect on benzoxazole had a relatively minor impact on its activity. While when electron-absorbing groups were incorporated, a decrease in activity was observed. We observed that the anti-MRSA activity of compounds with 5-COOCH3 and 5-COOH substituents was weaker than that of compound with 5-OCH3 substituents (compounds 58 and 60 vs 57). The compounds 61–63, with tert-butyl or trifluoromethyl substituents, displayed significantly inactive against MRSA (MIC ≥64 μg/mL). The series of compounds bearing fluorine substituents (compounds 50–53) exhibited antibacterial activity comparable to compound 45. In this series of derivatives, 4-F substituted compound 50 and 5-OCH3 substituted compound 57 exhibited better overall activity.
The ratios of MBC to MIC for the target pleuromutilin derivatives ranged from 1 to 4, indicating outstanding bactericidal activity of these compounds. The results showed that most pleuromutilin derivatives displayed excellent bactericidal ability against S. aureus. Among these derivatives, compounds 50 and 57 exhibited a greater bactericidal effect than the other pleuromutilin derivatives. Therefore, time-kill kinetics experiments were conducted to test the in vitro antibacterial activity of the two compounds. The results were presented in .
Figure 2. Time-kill curves for MRSA ATCC 43300 with different concentrations of compound 50 (a) and compound 57 (b).
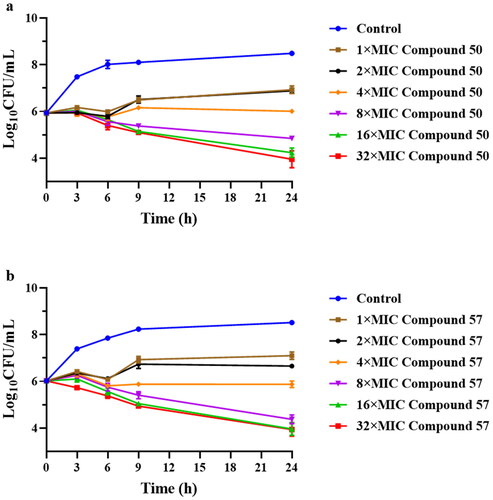
Both compounds 50 and 57 had an inhibition effect on MRSA (ATCC 43300) at the concentration of 4 × MIC. Compound 57 at 4 × MIC induced marked MRSA killing (−1.06 log10CFU/mL reduction) after 3 h of incubation. Remarkably, bacterial growth inhibition was observed at 24 h. In comparison, compound 50 at 4 × MIC could induce obviously MRSA killing (–1.54 log10CFU/mL reduction) at 3 h of incubation and kill 99.9% of MRSA after exposure for 24 h. The bactericidal effects of compounds 50 and 57 on MRSA were found to be dose-dependent and time-dependent, indicating that their efficacy was linked to both the concentration and duration of exposure. Therefore, in clinical practice, multiple or continuous intravenous administration of time-dependent antibacterial drugs may offer better therapeutic outcomesCitation28.
In order to evaluate the potential of the two derivatives for continuous inhibition of bacteria and provide rational dosing regimen, we conducted the post-antibiotic effect (PAE) tests on compounds 50 and 57. As a well-established pharmacodynamic parameter, PAE has important guiding significance for the rational use of antibiotics in clinical practiceCitation29. The results of the PAEs were displayed in and the bacterial growth kinetics curve was shown in . After being exposed to 4 × MIC and 2 × MIC for 2 h, we observed that compound 50 exhibited a PAE of 3.55 h and 1.93 h, respectively (). Similarly, the PAE of compound 57 against MRSA at the same concentrations was 2.71 h and 1.59 h (), respectively. These results indicated that compounds 50 and 57 exhibited a longer PAE than tiamulin (2.04 h) at 4 × MIC concentrationCitation30.
Figure 3. The bacterial growth kinetic curves for MRSA ATCC 43300 exposed to compound 50 (a) and compound 57 (c) for 2 h and compound 50 (b) and compound 57 (d) for 1 h.
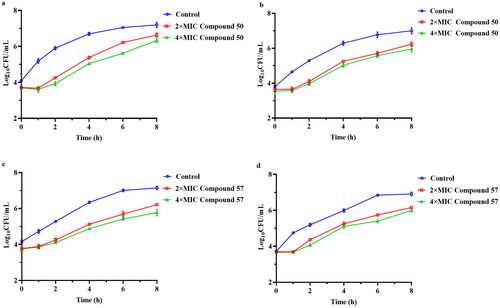
Table 2. The PAEs values of compounds 50 and 57 against MRSA ATCC 43300.
In the clinical use of medication, combining PAE with MIC, MBC and other pharmacodynamic parameters can enable a thorough assessment of the efficacy of antibacterial drugs. In particular, the PAE value can offer valuable insights for designing clinical dosing regimens and provide a theoretical basis for adjusting medication intervals. This is of significant importance in reducing the occurrence of adverse drug reactions. Based on our results, it appears that the administration interval for compounds 50 and 57 may be longer than that of tiamulin. This suggests that these two derivatives possess greater efficacy in inhibiting bacterial growth, and may therefore be administered less frequently without compromising their efficacy.
In vivo antibacterial activity
Compound 50 had been shown to display remarkable in vitro antibacterial activity against MRSA. In order to explore deeper into the in vivo antibacterial efficacy of this compound, we conducted an experiment using a neutropenic murine thigh infection model. To ensure accuracy, we utilised tiamulin as a positive control, while physiological saline served as a negative control. The results were indicated in .
In comparison to the control group, administration of tiamulin (20 mg/kg) resulted in a significant reduction (–0.94 log10CFU/mL) in bacterial load within the thigh muscles of mice (p < 0.0001, n = 6/group). Similarly, administration of compound 50 at the same dosage notably reduced MRSA load (–1.24 log10CFU/mL) in the thighs of the mice in comparison to the control group (p < 0.0001, n = 6/group). These results indicated that compound 50 were more effective in treating MRSA in mice than tiamulin.
Cytotoxicity assay
The cytotoxicity of compounds 45–63 and tiamulin to RAW 264.7 cells were evaluated by MTT assay. The results were displayed in . The results indicated that most of these compounds displayed similar influence compared with the control drugs, which demonstrated no significant inhibitory effect on RAW 264.7 cells at the concentration of 4 μg/mL.
Molecular docking study
Due to the excellent antibacterial activity exhibited by compound 50, the similarity-based molecular docking study was performed to predict the possible binding mode of compound 50 with 50S ribosomal subunit (PDB ID: 1XBP). Through Autodock software, the redocking of tiamulin into 1XBP placed the drug in the same conformation as that in the X-ray structure (RMSD = 0.743). The binding free energy of compound 50 with the 50S ribosome was calculated to be −7.50 kcal/mol. The docking results of compound 50 showed a similar binding mode () to tiamulin. The docking results was displayed in , which presented a superimposition mode of 50S ribosomes and compound 50.
As shown in , compound 50 was successfully localised in the binding pocket of 50S ribosomal subunit and exhibited five hydrogen bonds. Three of them were formed between the ketone (C21 atom) group and three different residues (A-2430, C-2046 and U-2564), with distances of 3.4 Å, 3.4 Å and 3.2 Å, respectively. One hydrogen bond was formed between the hydroxyl (C11 atom) and C-2431 with a distance of 2.7 Å. The fifth hydrogen bond was constituted by the benzoxazole side chain on the compound 50 and the residues of G-2044 (distance: 2.9 Å). According to the docking results, compound 50 possessed more hydrogen bonds with 50S ribosome residues compared with tiamulin, which might be acted as a hydrogen bond acceptor forming hydrogen bonds with 50S ribosome residue to improve antibacterial activity.
Conclusion
A series of novel pleuromutilin derivatives incorporating benzoxazole were designed and synthesised under mild reaction conditions. These newly developed derivatives were evaluated for their antibacterial activity both in vitro and in vivo against MRSA. Results showed that most of the tested compounds exhibited superior antibacterial activity compared to tiamulin. The time-killing curve results demonstrated that compound 50 displayed a time-dependent manner against MRSA. In addition, compound 50 exhibited a longer post-antibiotic effect (PAE) compared to tiamulin. Furthermore, compound 50 was more effective than tiamulin in MRSA infection mice thigh models. Notably, five strong hydrogen bonds were observed between compound 50 and residues of 50S ribosome. Taken together, these findings suggested that compound 50 might serve as a promising agent for treating MRSA infections, thus warranting further optimisation and discovery.
Experimental section
General information
Pleuromutilin (>90% pure) was purchased from Great Enjoyhood Biochemical Co. Ltd., (Daying, China). Carbon disulphide was purchased from TiTan Technology Co. Ltd., (Shanghai, China). 2-aminophenol derivatives were purchased from Bide Technology Co. Ltd., (Shanghai, China). The other analytical grade solvents were purchased from Guangzhou General Reagent Factory (Guangzhou, China). Chromatographic purification was carried out on silica gel columns (200–300 mesh, Branch of Qingdao Haiyang Chemical Co. Ltd., Shandong, China). 1H NMR and 13C NMR spectra were measured on Bruker AV-400, Bruker AV-500 or Bruker AV-600 spectrometer in chloroform-d (CDCl3) or DMSO-d6. Triethylsilane was used as the internal standard. Chemical shift values (δ) were indicated as ppm. High-resolution mass spectrometry was obtained by Waters Xevo G2-XS QTof Quadrupole Mass Spectrometry with an electrospray ionisation (ESI) source. The purity of target compounds was detected with Shimadzu Corporation Shim-pack Sceptre C18-120 (4.60 mm × 250 mm, 5 μm) column by high-performance liquid chromatography (HPLC) (Shimadzu Corporation Essentia LC-16). The solvent was a mixture of acetonitrile/water under 1.0 ml min−1 flow rate and the peak position was determined by UV spectrophotometry at 254 nm. The purities of the synthesised compounds are above 95% pure by HPLC analysis.
Synthesis of 22-O-tosylpleuromutilin (compound 6)
Pleuromutilin (1; 18.9 g; 50.0 mmol; 1.0 equiv) was dissolved in acetonitrile (30 ml) and stirred at 0 °C, then p-toluenesulfonyl chloride (11.8 g; 60 mmol; 1.2 equiv) was added in the mixed solution. Sodium hydroxide (4.0 g, 100.0 mmol) was dissolved in 15 ml of water and dropped slowly into the system. Next, the temperature was increased to room temperature, and stirring was continued for 3 h. After the reaction completion, acetonitrile was removed, and the residue was suspended in dichloromethane, then washed with water, and the organic layer was collected dried over anhydrous sodium sulphate and concentrated in vacuo to give a residue. The residue was suspended in isopropanol (30 ml) and the generated white solid was collected by filtration (24.3 g; 91%). The product was used in the next step without further purification.
Synthesis of benzoxazole-2-thiol derivatives (compounds 26–44)
Compounds 26–44 were synthesised by using a similar method. A 100 ml round bottomed flask containing ethanol (40 ml) was charged with 2-aminophenol (2.0 g, 18.3 mmol), potassium hydroxide (1.54 g, 27 mmol, solid) and carbon disulphide (7.0 g, 91.5 mmol) and the mixture was refluxed for 3 h at 60 °C. The solution was then cooled to room temperature to pH 4–5, then poured to water (40.0 ml) and extracted with EtOAc (3 × 20.0 ml). The combined organic layers were dried over Sodium sulphate anhydrous (Na2SO4) and evaporated. The remains were purified by column chromatography (petroleum ether: ethyl acetate= 15: 1)Citation26. Yield: 43%–96%.
Synthesis of 22-[(benzoxazole-2-yl) thio] deoxy pleuromutilin derivatives (compounds 45–63)
A general synthetic route based on the 22-deoxy-22-tosylpleuromutilin (compound 6), and a variety of benzoxazole-2-thiol derivatives were used to prepare target productions (Scheme 1). Synthetic route of target productions was referred from our previous literaturesCitation16. Compound 6 (1.0 g; 1.87 mmol; 1.0 equiv) was dissolved in acetonitrile (20 ml). Then added K2CO3 (0.39 g; 2.81 mmol; 1.5 equiv) and thiol derivative (2.24 mmol; 1.2 equiv) to the solution and stirred for 3 h at 70 °C until the reaction was completed. The acetonitrile was removed, and the residue was washed with dichloromethane (30 ml) and water (50 ml) for 3 times. The organic phase was dried over anhydrous Na2SO4 and evaporated in vacuum. All of the crude production was purified by silica gel column chromatography (petroleum ether: ethyl acetate = 10: 1).
22-[(Benzoxazole-2-yl) thio] deoxy pleuromutilin (compound 45)
Yield: 90%; Purity of compound was 98.1% (determined by RP HPLC at 254 nm, tR = 10.0 min, acetonitrile: water = 80: 20); 1H NMR (500 MHz, CDCl3) δ = 7.47 (dd, J = 7.3, 1.7, 1H), 7.35 (dd, J = 7.5, 1.6, 1H), 7.23 − 7.14 (m, 2H), 6.35 (H19, dd, J = 17.4, 11.0, 1H), 5.72 (H14, d, J = 8.5, 1H), 5.20 (H20, dd, J = 11.0, 1.5, 1H), 5.08 (H20, dd, J = 17.4, 1.5, 1H), 4.01 − 3.89 (H22, m, 2H), 3.26 (H11, d, J = 6.5, 1H), 2.26 − 1.92 (H2, H4, H10, H13, m, 5H), 1.72 − 1.41 (H1, H6, H7, H8, 11-OH, m, 6H), 1.37 (H15, s, 3H), 1.32 − 1.23 (H7, H13, m, 2H), 1.04 (H8, H18, s, 4H), 0.79 (H17, d, J = 7.0, 3H), 0.69 (H16, d, J = 7.1, 3H). 13C NMR (151 MHz, CDCl3) δ 216.9 (C3), 166.55 (C21), 163.12, 152.06, 141.68, 138.74 (C19), 124.42, 124.14, 118.62, 117.31 (C20), 110.00, 74.58 (C11), 70.50(C14), 58.10(C4), 45.43(C9), 44.49 (C13), 43.94 (C12), 41.88 (C5), 36.71 (C6), 36.01 (C10), 34.93, 34.44 (C2), 30.40 (C8), 26.84 (C7), 26.37 (C18), 24.82 (C1), 16.76 (C16), 14.79 (C15), 11.47(C17); HR-MS (ESI): Calcd for C29H37NO5S (M + Na+): 534.2284; Found: 534.2294.
22-[(4-methylbenzoxazole-2-yl) thio] deoxy pleuromutilin (compound 46)
Yield: 82%; Purity of compound was 96.7% (determined by RP HPLC at 254 nm, tR = 10.5 min, acetonitrile: water = 80: 20); 1H NMR (600 MHz, CDCl3) δ = 7.17 (d, J = 8.0, 1H), 7.06 (t, J = 7.8, 1H), 7.00 (dt, J = 7.5, 1.0, 1H), 6.36 (H19, dd, J = 17.4, 11.0, 1H), 5.71 (H14, d, J = 8.5, 1H), 5.21 (H20, dd, J = 11.0, 1.5, 1H), 5.08 (H20, dd, J = 17.4, 1.5, 1H), 4.01 − 3.91 (H22, m, 2H), 3.26 (H11, d, J = 6.5, 1H), 2.45 (s, 3H), 2.25 − 1.93 (H2, H4, H10, H13, m, 5H), 1.72 − 1.52 (H6, H8, 11-OH, m, 3H), 1.51 − 1.36 (H1, H7, m, 3H), 1.35 (H15, s, 3H), 1.32 − 1.22 (H7, H13, m, 2H), 1.04 (H8, H18, s, 4H), 0.79 (H17, d, J = 7.1, 3H), 0.70 (H16, d, J = 7.1, 3H). 13C NMR (151 MHz, CDCl3) δ 216.9 (C3), 166.7 (C21), 161.9, 151.8, 141.0, 138.8 (C19), 125.0, 123.8, 117.4, 117.3 (C20), 107.3, 74.6 (C11), 70.4 (C14), 58.1 (C4), 45.4 (C9), 44.6 (C13), 44.0 (C12), 41.9 (C5), 36.7 (C6), 36.00 (C10), 34.9 (C2), 34.4 (C22), 30.4 (C8), 26.8 (C7), 26.3 (C18), 24.8 (C1), 21.7, 16.8 (C16), 14.8 (C15), 11.5 (C17); HR-MS (ESI): Calcd for C30H39NO5S (M + CHO2–): 570.2531; Found:570.2532.
22-[(5-methylbenzoxazole-2-yl) thio] deoxy pleuromutilin (compound 47)
Yield: 36%; Purity of compound was 95.2% (determined by RP HPLC at 254 nm, tR = 9.7 min, acetonitrile: water = 80: 20); 1H NMR (600 MHz, CDCl3) δ = 7.26 (d, J = 1.6, 1H), 7.22 (d, J = 8.3, 1H), 6.98 (dd, J = 8.3, 1.7, 1H), 6.35 (H19, dd, J = 17.4, 10.9, 1H), 5.71 (H14, d, J = 8.5, 1H), 5.21 (H20, dd, J = 10.9, 1.5, 1H), 5.09 (H20, dd, J = 17.4, 1.6, 1H), 3.98 − 3.89 (H22, m, 2H), 3.26 (H11, d, J = 6.5, 1H), 2.36 (s, 3H), 2.25 − 1.92 (H2, H4, H10, H13, m, 5H), 1.71 − 1.55 (H6, H8, 11-OH, m, 3H), 1.51 − 1.37 (H1, H7, m, 3H), 1.35 (H15, s, 3H), 1.32 − 1.25 (H7, H13, m, 2H), 1.04 (H8, H18, s, 4H), 0.79 (H17, d, J = 7.2, 4H), 0.69 (H16, d, J = 7.1, 3H). 13C NMR (151 MHz, CDCl3) δ 216.9 (C3), 166.6 (C21), 162.9, 150.3, 141.9, 138.8 (C19), 134.2, 125.1, 118.7, 117.3 (C20), 109.3, 74.6 (C11), 70.5 (C14), 58.1 (C4), 45.4 (C9), 44.5 (C13), 44.0 (C12), 41.9 (C5), 36.7 (C6), 36.0 (10), 34.9 (C2), 34.4 (C22), 30.4 (C8), 26.8 (C7), 26.3 (C18), 24.8 (C1), 21.4, 16.8 (C16), 14.8 (C15), 11.5 (C17); HR-MS (ESI): Calcd for C30H39NO5S (M + CHO2–): 570.2531; Found:570.2532.
22-[(6-methylbenzoxazole-2-yl) thio] deoxy pleuromutilin (compound 48)
Yield: 71%; Purity of compound was 96.6% (determined by RP HPLC at 254 nm, tR = 9.8 min, acetonitrile: water = 80: 20); 1H NMR (600 MHz, CDCl3) δ = 7.33 (d, J = 8.1, 1H), 7.16 (s, 1H), 7.01 (dd, J = 8.1, 1.6, 1H), 6.35 (H19, dd, J = 17.4, 11.0, 1H), 5.71 (H14, d, J = 8.5, 1H), 5.21 (H20, dd, J = 11.1, 1.5, 1H), 5.08 (H20, dd, J = 17.4, 1.5, 1H), 3.98 − 3.88 (H22, m, 2H), 3.26 (H11, dd, J = 10.5, 6.5, 1H), 2.38 (s, 3H), 2.25 − 1.92 (H2, H4, H10, H13, m, 5H), 1.71 − 1.54 (H6, H8, 11-OH, m, 3H), 1.51 − 1.36 (H1, H7, m, 3H), 1.35 (H15, s, 3H), 1.32 − 1.25 (H7, H13, m, 2H), 1.04 (H8, H18, s, 4H), 0.79 (H17, d, J = 7.0, 3H), 0.69 (H16, d, J = 7.1, 3H). 13C NMR (151 MHz, CDCl3) δ 217.0 (C3), 166.6 (C21), 162.2, 152.3, 139.5, 138.8 (C19), 134.5, 125.5, 117.9, 117.3 (C20), 110.3, 74.6 (C11), 70.4 (C14), 58.1 (C4), 45.4 (C9), 44.5 (C13), 43.9 (C12), 41.9 (C5), 36.7 (C6), 36.0 (10), 34.9 (C2), 34.4 (C22), 30.4 (C8), 26.8 (C7), 26.4 (C18), 24.8 (C1), 21.6, 16.8 (C16), 14.8 (15), 11.5 (C17); HR-MS (ESI): Calcd for C30H39NO5S (M + CHO2–): 570.2531; Found:570.2532.
22-[(7-methylbenzoxazole-2-yl) thio] deoxy pleuromutilin (compound 49)
Yield: 85%; Purity of compound was 98.0% (determined by RP HPLC at 254 nm, tR = 9.9 min, acetonitrile: water = 80: 20);1H NMR (600 MHz, CDCl3) δ 7.29 (d, J = 7.9 Hz, 1H), 7.09 (t, J = 7.7 Hz, 1H), 6.97 (dt, J = 7.5, 1.1 Hz, 1H), 6.35 (H19, dd, J = 17.4, 11.0 Hz, 1H), 5.71 (H14, d, J = 8.5 Hz, 1H), 5.20 (H20, dd, J = 11.0, 1.5 Hz, 1H), 5.08 (H20, dd, J = 17.4, 1.6 Hz, 1H), 3.99 − 3.91 (H22, m, 2H), 3.26 (H11, d, J = 6.5 Hz, 1H), 2.40 (s, 3H), 2.25 − 1.92 (H2, H4, H10, H13, m, 5H), 1.71 − 1.55 (H6, H8, 11-OH, m, 3H), 1.51 − 1.36 (H1, H7, m, 3H), 1.36 (H15, s, 3H), 1.31 − 1.26 (H7, H13, m, 2H), 1.08 − 1.02 (H8, H18, m, 4H), 0.79 (H17, d, J = 7.0 Hz, 3H), 0.69 (H16, d, J = 7.1 Hz, 3H). 13C NMR (151 MHz, CDCl3) δ 217.0 (C3), 166.6 (C21), 162.7, 151.3, 141.3, 138.8 (C19), 125.3, 124.3, 120.6, 117.3 (C20), 115.9, 74.6 (C11), 70.5 (C14), 58.1 (C4), 45.5 (C9), 44.5 (C13), 44.0 (C12), 41. 9 (C5), 36.7 (C6), 36.0 (C10), 35.0 (C2), 34.5 (C22), 30.4 (C8), 26.9 (C7), 26.4(C18), 24.8 (C1), 16.8 (C16), 15.1, 14.8 (C15), 11.5 (C17); HR-MS (ESI): Calcd for C30H39NO5S (M + CHO2–): 570.2531; Found:570.2531.
22-[(4-fluorobenzoxazole-2-yl) thio] deoxy pleuromutilin (compound 50)
Yield: 86%; Purity of compound was 97.5% (determined by RP HPLC at 254 nm, tR = 8.0 min, acetonitrile: water = 80: 20); 1H NMR (600 MHz, CDCl3) δ 7.18 (dd, J = 8.2, 1.0 Hz, 1H), 7.13 (td, J = 8.2, 4.7 Hz, 1H), 6.94 (ddd, J = 9.3, 8.0, 1.0 Hz, 1H), 6.34 (H19, dd, J = 17.4, 11.0 Hz, 1H), 5.73 (H14, d, J = 8.5 Hz, 1H), 5.17 (H20, dd, J = 11.0, 1.5 Hz, 1H), 5.07 (H20, dd, J = 17.4, 1.5 Hz, 1H), 4.01 − 3.93 (H22, m, 2H), 3.27 (H11, dd, J = 10.3, 6.5 Hz, 1H), 2.26 − 1.96 (H2, H4, H10, H13, m, 6H), 1.71 − 1.56 (H6, H8, 11-OH, m, 3H), 1.52 − 1.37 (H1, H7, m, 3H), 1.37 (H15, s, 3H), 1.34 − 1.28 (H7, H13, m, 2H), 1.09 − 1.03 (H8, H18, m, 4H), 0.80 (H17, d, J = 7.1 Hz, 3H), 0.70 (H16, d, J = 7.1 Hz, 3H). 13C NMR (151 MHz, CDCl3) δ 217.0 (C3), 166.4 (C21), 163.9, 154.2, 138.7 (C19), 123.0, 128.1, 124.6, 117.3 (C20), 110.9, 106.2, 74.6 (C11), 70.7 (C14), 58.1 (C4), 45.5 (C9), 44.54 (C13), 44.0 (C12), 41.9 (C5), 36.7 (C6), 36.0 (C10), 35.1 (C2), 34.5 (C22), 30.4 (C8), 26.8 (C7), 26.3 (C18), 24.8 (C1), 16.7 (C16), 16.6, 14.8 (C15), 14.8, 11.5 (C17); HR-MS (ESI): Calcd for C29H36FNO5S (M – H+): 528.2225; Found: 528.2225.
22-[(5-fluorobenzoxazole-2-yl) thio] deoxy pleuromutilin (compound 51)
Yield: 48%; Purity of compound was 96.6% (determined by RP HPLC at 254 nm, tR = 8.3 min, acetonitrile: water = 80: 20); 1H NMR (600 MHz, CDCl3) δ 7.28 (dd, J = 8.9, 4.2 Hz, 1H), 7.15 (dd, J = 8.3, 2.6 Hz, 1H), 6.91 (td, J = 9.1, 2.6 Hz, 1H), 6.35 (H19, dd, J = 17.4, 10.9 Hz, 1H), 5.72 (H14, d, J = 8.5 Hz, 1H), 5.21 (H20, dd, J = 10.9, 1.5 Hz, 1H), 5.09 (H20, dd, J = 17.4, 1.5 Hz, 1H), 3.98 − 3.89 (H22, m, 2H), 3.27 (H11, d, J = 6.4 Hz, 1H), 2.25 − 1.94 (H2, H4, H10, H13, m, 5H), 1.71 − 1.55 (H6, H8, 11-OH, m, 3H), 1.51 − 1.37 (H1, H7, m, 3H), 1.36 (H15, s, 3H), 1.32 − 1.25 (H7, H13, m, 2H), 1.06 (H8, H18, s, 4H), 0.80 (H17, d, J = 7.1 Hz, 3H), 0.69 (H16, d, J = 7.1 Hz, 3H). 13C NMR (151 MHz, CDCl3) δ 216.9 (C3), 166.4 (C21), 165.2, 159.2, 148.4, 142.6, 138.8 (C19), 117.3 (C20), 111.6, 111.4, 105.4, 74.6 (C11), 70.6 (C14), 58.1 (C4), 45.4 (C9), 44.5 (C13), 44.0 (C12), 41.9 (C5), 36.7 (C6), 36.0 (10), 34.9 (C2), 34.55 (C22), 30.4 (C8), 26.8 (C7), 26.4 (C18), 24.8 (C1), 16.8 (C16), 14.7 (C15), 11.5 (C17); HR-MS (ESI): Calcd for C29H36FNO5S (M + Na+): 552.2190; Found: 552.2185.
22-[(6-fluorobenzoxazole-2-yl) thio] deoxy pleuromutilin (compound 52)
Yield: 89%; Purity of compound was 96.8% (determined by RP HPLC at 254 nm, tR = 8.8 min, acetonitrile: water = 80: 20); 1H NMR (600 MHz, DMSO-d6) δ 7.66 − 7.61 (m, 1H), 7.57 (dd, J = 8.8, 4.9 Hz, 1H), 7.23 (ddd, J = 9.8, 8.7, 2.5 Hz, 1H), 6.10 − 6.04 (H19, m, 1H), 5.56 (H14. d, J = 8.3 Hz, 1H), 5.06 (H20, ddd, J = 17.9, 7.0, 1.7 Hz, 1H), 4.95 (H20, dd, J = 11.2, 1.7 Hz, 1H), 4.54 − 4.49 (11-OH, m, 1H), 4.19 (H22, d, J = 3.0 Hz, 2H,), 3.39 (H11, t, J = 6.1 Hz, 1H) 2.40 − 2.37 (H2, m, 1H), 2.22 − 2.14 (H2, m, 1H), 2.10 − 1.96 (H4, H10, H13, m, 3H), 1.67 − 1.57 (H6, H8, m, 2H), 1.51 − 1.35 (H1, H7, m, 2H), 1.34 (H15, d, J = 4.0 Hz, 3H), 1.28 − 1.20 (H1, H7, H13, m, 3H,), 1.03 − 0.94 (H8, H18, m, 4H), 0.80 (H17, d, J = 7.0 Hz, 3H), 0.64 (H16, t, J = 7.0 Hz, 3H). 13C NMR (151 MHz, DMSO-d6) δ 217.6 (C3), 166.6 (C21), 164.4, 158.9, 151.7, 141.1, 138.2 (C19), 119.2, 115.7 (C20), 112.8, 99.4, 73.0 (C11), 71.1 (C14), 57.6 (C4), 45.4 (C9), 44.6 (C13), 42.0 (C12), 40.5 (C5), 36.9 (C6), 36.8 (C10), 34.9 (C2), 34.4 (C22), 30.5 (C8), 29.1 (C7), 27.0 (C18), 24.9 (C1), 16.5 (C16), 14.9 (C15), 12.0 (C17); HR-MS (ESI): Calcd for C29H36FNO5S (M + Na+): 552.2190; Found: 552.2185.
22-[(7-fluorobenzoxazole-2-yl) thio] deoxy pleuromutilin (compound 53)
Yield: 90%; Purity of compound was 99.0% (determined by RP HPLC at 254 nm, tR = 8.7 min, acetonitrile: water = 80: 20); 1H NMR (600 MHz, CDCl3) δ 7.26 (dd, J = 8.0, 0.9 Hz, 1H), 7.15 (td, J = 8.1, 4.6 Hz, 1H), 6.94 (ddd, J = 9.6, 8.2, 0.9 Hz, 1H), 6.35 (H19, dd, J = 17.4, 11.0 Hz, 1H), 5.73 (H14, d, J = 8.5 Hz, 1H), 5.20 (H20, dd, J = 11.0, 1.5 Hz, 1H), 5.08 (H20, dd, J = 17.4, 1.5 Hz, 1H), 3.99 − 3.91 (H22, m, 2H), 3.27 (H11, d, J = 6.5 Hz, 1H), 2.25 − 1.94 (H2, H4, H10, H13, m, 5H), 1.71 − 1.55 (H6, H8, 11-OH, m, 3H), 1.51 − 1.37 (H1, H7, m, 3H), 1.36 (H15, s, 3H), 1.32 − 1.26 (H7, H13, m, 2H), 1.05 (H8, H18, s, 4H), 0.80 (H17, d, J = 7.0 Hz, 3H), 0.70 (H16, d, J = 7.2 Hz, 3H). 13C NMR (151 MHz, CDCl3) δ 216.9 (C3), 166.3 (C21), 164.0, 147.2, 145.6, 144.9, 138.7 (C19), 124.9, 117.3 (C20), 114.4, 111.2, 74.6 (C11), 70.7 (C14), 58.1 (C4), 45.4 (C9), 44.5 (C13), 44.0 (C12), 41.9 (C5), 36.7 (C6), 36.0 (C10), 35.1 (C2), 34.4 (C22), 30.4 (C8), 26.8 (C7), 26.4 (C18), 24.8 (C1), 16.8 (C16), 14.8 (C15), 11.5 (C17); HR-MS (ESI): Calcd for C29H36FNO5S (M + Na+): 552.2190; Found: 552.2185.
22- [(5-chlorobenzoxazole-2-yl) thio] deoxy pleuromutilin (compound 54)
Yield: 65%; Purity of compound was 95.2% (determined by RP HPLC at 254 nm, tR = 10.7 min, acetonitrile: water = 80: 20); 1H NMR (600 MHz, CDCl3) δ 7.44 (d, J = 2.1 Hz, 1H), 7.27 (d, J = 8.6 Hz, 1H), 7.15 (dd, J = 8.6, 2.1 Hz, 1H), 6.35 (H19, dd, J = 17.4, 11.0 Hz, 1H), 5.72 (H14, d, J = 8.5 Hz, 1H), 5.20 (H20, dd, J = 11.0, 1.5 Hz, 1H), 5.09 (H20, dd, J = 17.4, 1.6 Hz, 1H), 3.98 − 3.89 (H22, m, 2H), 3.27 (H11, t, J = 7.4 Hz, 1H), 2.24 − 1.94 (H2, H4, H10, H13, m, 5H), 1.71 − 1.56 (H6, H8, 11-OH, m, 3H), 1.48 − 1.37 (H1, H7, m, 3H), 1.36 (H15, s, 3H), 1.32 − 1.24 (H7, H13, m, 2H), 1.06 (H8, H18, s, 4H), 0.79 (H17, d, J = 7.1 Hz, 3H), 0.69 (H16, d, J = 7.1 Hz, 3H). 13C NMR (151 MHz, CDCl3) δ 216.9 (C3), 166.4 (C21), 165.0, 150.6, 142.8, 138.7 (C19), 130.0, 124.3, 118.7, 117.3 (C20), 110.6, 74.6 (C11), 70.6 (C14), 58.4 (C4), 45.4 (C9), 44.5 (C13), 43.9 (C12), 41.9 (C5), 36.8 (C6), 36.0 (C10), 34.9 (C2), 34.4 (C22), 30.4 (C8), 26.8 (C7), 26.4 (C18), 24.8 (C1), 16.8 (C16), 14.8 (C15), 11.5 (C17); HR-MS (ESI): Calcd for C29H36ClNO5S (M – H+): 544.1930; Found: 544.1931.
22-[(6-chlorobenzoxazole-2-yl) thio] deoxy pleuromutilin (compound 55)
Yield: 72%; Purity of compound was 95.3% (determined by RP HPLC at 254 nm, tR = 11.0 min, acetonitrile: water = 80: 20); 1H NMR (600 MHz, DMSO-d6) δ 7.84 (d, J = 1.9 Hz, 1H), 7.56 (d, J = 8.5 Hz, 1H), 7.40 (dd, J = 8.5, 2.0 Hz, 1H), 6.07 (H19, dd, J = 17.8, 11.2 Hz, 1H), 5.56 (H14, d, J = 8.4 Hz, 1H), 5.05 (H20, dd, J = 17.8, 1.7 Hz, 1H), 4.95 (H20, dd, J = 11.2, 1.7 Hz, 1H), 4.51 (11-OH, d, J = 6.1 Hz, 1H), 4.20 (H22, d, J = 2.4 Hz, 2H), 3.39 (H11, t, J = 6.0 Hz, 1H), 2.40 − 2.37 (H2, m, 1H), 2.21 − 2.13 (H2, m, 1H), 2.10 − 1.97 (H4, H10, H13, m, 3H), 1.67 − 1.57 (H6, H8, m, 2H), 1.50 − 1.35 (H1, H7, m, 2H), 1.33 (H15, s, 3H), 1.29 − 1.21 (H1, H7, H13, m, 3H), 0.97 (H8, H18, s, 4H), 0.80 (H17, d, J = 7.0 Hz, 3H), 0.63 (H16, d, J = 7.2 Hz, 3H). 13C NMR (151 MHz, DMSO-d6) δ 216.5 (C3), 165.5, 151.0, 140.1, 139.6 (C19), 128.1, 124.5, 118.5, 114.6 (C20), 110.3, 71.9 (C11), 70.0 (C14), 56.6 (C4), 44.3 (C9), 43.5 (C13), 42.6 (C12), 41.0 (C12), 39.5 (C5), 35.8 (C6), 35.7, 33.8 (C2), 33.4(C22), 29.5 (C8), 28.0 (C7), 26.0 (C18), 23.8 (C1), 15.5 (C15), 13.8, 10.9 (C17); HR-MS (ESI): Calcd for C29H36ClNO5S (M – H+): 544.1930; Found: 544.1934.
22-[(7-chlorobenzoxazole-2-yl) thio] deoxy pleuromutilin (compound 56)
Yield: 63%; Purity of compound was 96.9% (determined by RP HPLC at 254 nm, tR = 10.9 min, acetonitrile: water = 80: 20); 1H NMR (600 MHz, DMSO-d6) δ 7.54 (dd, J = 7.9, 1.1 Hz, 1H), 7.44 (dd, J = 8.1, 1.0 Hz, 1H), 7.37 (t, J = 8.0 Hz, 1H), 6.07 (H19, dd, J = 17.8, 11.2 Hz, 1H), 5.56 (H14, d, J = 8.5 Hz, 1H), 5.04 (H20, dd, J = 17.8, 1.7 Hz, 1H), 4.94 (H20, dd, J = 11.2, 1.7 Hz, 1H), 4.51 (11-OH, d, J = 6.1 Hz, 1H), 4.22 (H22, d, J = 3.1 Hz, 2H), 3.39 (H11, t, J = 6.1 Hz, 1H), 2.41 − 2.37 (H2, m, 1H), 2.21 − 2.13 (H2, m, 1H), 2.10 − 1.98 (H4, H10, H13, m, 3H), 1.67 − 1.57 (H6, H8, m, 2H), 1.51 − 1.35 (H1, H7, m, 2H), 1.33 (H15, s, 3H), 1.29 − 1.22 (H1, H7, H13, m, 3H), 0.97 (H8, H18, s, 4H), 0.80 (H17, d, J = 7.0 Hz, 3H), 0.63 (H16, d, J = 7.1 Hz, 3H). 13C NMR (151 MHz, DMSO-d6) δ 217.6 (C3), 166.5 (C21), 164.8, 148.1, 143.0, 141.1 (C19), 126.5, 125.1, 117.8 115.7(C20), 114.4, 73.0 (C11), 71.2 (C14), 60.2 (C4), 45.4 (C9), 44.6 (C13), 42.0 (C12), 40.6 (C5), 36.9 (C6), 36.8 (C10), 35.0 (C2), 34.4 (C22), 30.5 (C8), 29.1 (C7), 27.1 (C18), 24.9 (C1), 16.6 (C16), 14.9 (C15), 12.0 (C17); HR-MS (ESI): Calcd for C29H36ClNO5S (M – H+): 544.1930; Found: 544.1934.
22-[(5-methoxybenzoxazole-2-yl) thio] deoxy pleuromutilin (compound 57)
Yield: 84%; Purity of compound was 95.8% (determined by RP HPLC at 254 nm, tR = 7.8 min, acetonitrile: water = 80: 20); 1H NMR (400 MHz, DMSO-d6) δ 7.51 (d, J = 8.9 Hz, 1H), 7.10 (d, J = 2.6 Hz, 1H), 6.90 (dd, J = 8.9, 2.6 Hz, 1H), 6.08 (H19, dd, J = 17.8, 11.2 Hz, 1H), 5.57 (H14, d, J = 8.3 Hz, 1H), 5.07 (H20, dd, J = 17.8, 1.8 Hz, 1H), 4.96 (H20, dd, J = 11.2, 1.7 Hz, 1H), 4.51 (11-OH, d, J = 6.0 Hz, 1H), 4.18 (H22, s, 2H), 3.78 (s, 3H), 3.40 (H11, t, J = 6.0 Hz, 1H), 2.42 − 2.34 (H2, m, 1H), 2.24 − 2.13 (H2, m, 1H), 2.10 − 1.95 (H4, H10, H13, m, 3H), 1.63 (H6, H8, dtd, J = 19.9, 11.1, 9.9, 5.8 Hz, 2H), 1.52 − 1.37 (H1, H7, m, 2H), 1.34 (H15, s, 3H), 1.31 − 1.23 (H1, H7, H13, m, 3H), 1.07 − 0.94 (H8, H18, m, 4H), 0.81 (H17, d, J = 7.0 Hz, 3H), 0.64 (H16, d, J = 7.0 Hz, 3H). 13C NMR (151 MHz, DMSO-d6) δ 217.6 (C3), 167.0 (C21), 166.5, 153.8, 141.9, 141.1 (C19), 126.1, 122.2, 116.1, 115.6 (C20), 111.8, 73.0 (C11), 71.1 (C14), 57.6 (C4), 45.4 (C9), 44.5 (C13), 43.7 (C12), 42.0 (C5), 40.5, 37.3 (C6), 36.9(C10), 34.9 (C2), 34.4 (C22), 30.5 (C8), 28.9 (C7), 27.0 (C18), 24.9 (C1), 16.5 (C16), 14.9 (C15), 12.0 (C17); HR-MS (ESI): Calcd for C30H39NO6S (M + Na+): 564.2390; Found: 564.2374.
22-[(Methyl benzoxazole-5-carboxylate-2-yl) thio] deoxy pleuromutilin (compound 58)
Yield: 90%; Purity of compound was 95.8% (determined by RP HPLC at 254 nm, tR = 8.0 min, acetonitrile: water = 80: 20); 1H NMR (400 MHz, DMSO-d6) δ 7.88 − 7.77 (m, 2H), 7.47 (t, J = 7.8 Hz, 1H), 6.07 (H19, dd, J = 17.8, 11.2 Hz, 1H), 5.57 (H14, d, J = 8.3 Hz, 1H), 5.03 (H20, dd, J = 17.8, 1.8 Hz, 1H), 4.92 (H20, dd, J = 11.2, 1.7 Hz, 1H), 4.48 (11-OH, d, J = 5.8 Hz, 1H), 4.25 (H22, d, J = 1.5 Hz, 2H), 3.39 (H11, t, J = 5.1 Hz, 1H), 2.72 (s, 3H), 2.38 (H2, d, J = 2.5 Hz, 1H), 2.23 − 2.12 (H2, m, 1H), 2.07 − 1.95 (H4, H10, H13, m, 3H), 1.62 (H6, H8, dtd, J = 20.0, 11.1, 10.0, 6.0 Hz, 2H), 1.53 − 1.36 (H1, H7, m, 2H), 1.34 (H15, s, 3H), 1.27 (H1, H7, H13, dd, J = 14.5, 11.4 Hz, 3H), 0.95 (H8, H18, s, 4H), 0.80 (H17, d, J = 6.9 Hz, 3H), 0.63 (H16, d, J = 6.9 Hz, 3H). 13C NMR (151 MHz, DMSO-d6) δ 217.6 (C3), 166.6 (C21), 166.2, 165.98, 154.7, 141.8, 141.1 (C19), 127.0, 126.4, 119.7, 115.7 (C20), 111.0, 73.0 (C11), 71.1 (C14), 57.6 (C4), 52.9, 45.4 (C9), 44.6 (C13), 43.7 (C12), 42.0 (C5), 36.9 (C6), 36.8 (C10), 34.9 (C2), 34.4 (C22), 30.5 (C8), 29.0 (C7), 27.1 (C18), 24.9 (C1), 16.5 (C16), 14.9 (C15), 12.0 (C17); HR-MS (ESI): Calcd for C31H39NO7S (M + Na+): 592.2339; Found: 592.2335.
22-[(Methyl benzoxazole-6-carboxylate-2-yl) thio] deoxy pleuromutilin (compound 59)
Yield: 92%; Purity of compound was 95.1% (determined by RP HPLC at 254 nm, tR = 8.1 min, acetonitrile: water = 80: 20); 1H NMR (600 MHz, DMSO-d6) δ 8.15 (d, J = 1.6 Hz, 1H), 7.98 (dd, J = 8.3, 1.6 Hz, 1H), 7.66 (d, J = 8.3 Hz, 1H), 6.08 (H19, dd, J = 17.8, 11.3 Hz, 1H), 5.57 (H14, d, J = 8.5 Hz, 1H), 5.06 (H20, dd, J = 17.8, 1.7 Hz, 1H), 4.95 (H20, dd, J = 11.1, 1.7 Hz, 1H), 4.51 (11-OH, d, J = 5.9 Hz, 1H), 4.24 (H22, d, J = 2.1 Hz, 2H), 3.88 (s, 3H), 3.39 (H11, t, J = 5.5 Hz, 1H), 2.41 − 2.38 (H2, m, 1H), 2.21 − 2.14 (H2, m, 1H), 2.10 − 1.99 (H4, H10, H13, m, 3H), 1.67 − 1.57 (H6, H8, m, 2H), 1.52 − 1.35 (H1, H7, m, 2H), 1.34 (H15, s, 3H), 1.31 − 1.24 (H1, H7, H13, m, 3H), 1.03 − 0.94 (H8, H18, m, 4H), 0.80 (H17, d, J = 7.0 Hz, 3H), 0.65 (H16, d, J = 7.1 Hz, 3H). 13C NMR (151 MHz, DMSO-d6) δ 217.5 (C3), 166.7 (C21), 165.2, 164.6, 151.5, 145.8, 137.9 (C19), 128.2, 127.5, 116.8, 115.8 (C20), 110.9, 71.1(C11), 70.9 (C14), 66.3, 57.6 (C4), 45.4(C9), 44.6 (C13), 43.6 (C12), 42.0 (C5), 36.9 (C6), 36.8 (C10), 34.8 (C2), 34.5 (C22), 30.5 (C8), 29.1 (C7), 27.1(C18), 24.9 (C1), 16.5 (C16), 14.9 (C15), 12.0 (C17); HR-MS (ESI): Calcd for C31H39NO7S (M + Na+): 592.2339; Found: 592.2335.
22-[(Benzoxazole-5-carboxylic acid-2-yl) thio] deoxy pleuromutilin (compound 60)
Yield: 54%; Purity of compound was 96.3% (determined by RP HPLC at 254 nm, tR = 3.7 min, acetonitrile: water = 80: 20); 1H NMR (600 MHz, DMSO-d6) δ 13.13 (s, 1H), 8.07 (d, J = 1.7 Hz, 1H), 7.95 (dd, J = 8.5, 1.7 Hz, 1H), 7.71 (d, J = 8.5 Hz, 1H), 6.07 (H19, dd, J = 17.8, 11.2 Hz, 1H), 5.57 (H14, d, J = 8.4 Hz, 1H), 5.06 (H20, dd, J = 17.8, 1.7 Hz, 1H), 4.95 (H20, dd, J = 11.1, 1.7 Hz, 1H), 4.51 (11-OH, d, J = 5.8 Hz, 1H), 4.23 (H22, d, J = 1.6 Hz, 2H), 3.39 (H11, t, J = 4.8 Hz, 1H), 2.41 − 2.35 (H2, m, 1H), 2.18 (H2, ddd, J = 19.5, 10.5, 2.3 Hz, 1H), 2.10 − 1.97 (H4, H10, H13, m, 3H), 1.68 − 1.55 (H6, H8, m, 2H), 1.51 − 1.35 (H1, H7, m, 2H), 1.34 (H15, s, 3H), 1.32 − 1.24 (H1, H7, H13, m, 3H), 1.03 − 0.94 (H8, H18, m, 4H), 0.80 (H17, d, J = 7.0 Hz, 3H), 0.65 (H16, d, J = 7.1 Hz, 3H). 13C NMR (151 MHz, DMSO-d6) δ 217.6 (C3), 166.6 (C21), 166.2, 166.0, 154.7, 141.8, 141.1 (C19), 127.0, 126.4, 119.7, 115.7 (C20), 111.0, 73.0 (C11), 71.1 (C14), 57.6 (C4), 45.4 (C9), 44.6 (C13), 43.7 (C12), 42.0 (C5), 36.9 (C6), 36.8 (C10), 34.9 (C2), 34.4 (C22), 30.5 (C8), 29.0 (C7), 27.0 (C18), 24.9 (C1), 16.5 (C16), 14.9 (C15), 12.0 (C17); HR-MS (ESI): Calcd for C30H37NO7S (M – H+): 554.2218; Found: 554.2205.
22-[(5-tert-butybenzoxazole -2-yl) thio] deoxy pleuromutilin (compound 61)
Yield: 93%; Purity of compound was 96.1% (determined by RP HPLC at 254 nm, tR = 6.1 min, acetonitrile: water = 80: 20);1H NMR (600 MHz, CDCl3) δ 7.49 (d, J = 1.8 Hz, 1H), 7.26 (d, J = 8.6 Hz, 1H), 7.22 (dd, J = 8.6, 1.8 Hz, 1H), 6.35 (H19, dd, J = 17.4, 11.0 Hz, 1H), 5.72 (H14, d, J = 8.5 Hz, 1H), 5.20 (H20, dd, J = 10.9, 1.5 Hz, 1H), 5.07 (H20, dd, J = 17.3, 1.7 Hz, 1H), 3.98 − 3.87 (H22, m, 2H), 3.26 (H11, d, J = 6.5 Hz, 1H), 2.25 − 1.90 (H2, H4, H10, H13, m, 5H), 1.71 − 1.55 (H6, H8, 11-OH, m, 3H), 1.51 − 1.38 (H1, H7, m, 3H), 1.37 (H15, s, 3H), 1.28 (H7, H13, s, 11H), 1.08 − 1.01 (H8, H18, m, 4H), 0.79 (H17, d, J = 7.0 Hz, 3H), 0.70 (H16, d, J = 7.1 Hz, 3H). 13C NMR (151 MHz, CDCl3) δ 216.9 (C3), 166.4 (C21), 163.5, 160.7, 159.1, 151.9, 138.8 (C19), 138.7, 138.0, 118.7, 117.3 (C20), 112.1, 98.3, 74.6 (C11), 70.6, 70.5 (C14), 58.1 (C4), 45.4 (C9), 44.5 (C13), 43.9 (C12), 41.9(C5), 36.7 (C6), 36.0 (C10), 35.0 (C2), 34.4 (C22), 30.4 (C8), 26.8 (C7), 26.4 (C18), 24.8 (C1), 16.8 (C16), 14.8 (C15), 11.5 (C17); HR-MS (ESI): Calcd for C33H45NO5S (M – H+): 566.2945; Found: 566.2946.
22-[(5-trifluoromethylbenzoxazole-2-yl) thio] deoxy pleuromutilin (compound 62)
Yield: 89%; Purity of compound was 97.9% (determined by RP HPLC at 254 nm, tR = 10.5 min, acetonitrile: water = 80: 20); 1H NMR (600 MHz, DMSO-d6) δ 7.92 (d, J = 1.8 Hz, 1H), 7.87 (d, J = 8.5 Hz, 1H), 7.71 (dd, J = 8.7, 1.9 Hz, 1H), 6.07 (H19, dd, J = 17.8, 11.2 Hz, 1H), 5.58 (H14, d, J = 8.4 Hz, 1H), 5.03 (H20, dd, J = 17.8, 1.7 Hz, 1H), 4.94 (H20, dd, J = 11.2, 1.7 Hz, 1H), 4.50 (11-OH, d, J = 6.2 Hz, 1H), 4.25 (H22, s, 2H), 3.39 (H11, t, J = 6.1 Hz, 1H), 2.41 − 2.36 (H2, m, 1H), 2.18 (H2, ddd, J = 18.2, 11.0, 2.0 Hz, 1H), 2.11 − 1.96 (H4, H10, H13, m, 3H), 1.67 − 1.57 (H6, H8, m, 2H), 1.52 − 1.35 (H1, H7, m, 2H), 1.34 (H15, s, 3H), 1.28 − 1.21 (H1, H7, H13, m, 3H), 1.03 − 0.91 (H8, H18, m, 4H), 0.80 (H17, d, J = 7.0 Hz, 3H), 0.64 (H16, d, J = 7.1 Hz, 3H). 13C NMR (151 MHz, DMSO-d6) δ 217.6 (C3), 166.7 (C21), 166.5, 153.8, 141.9, 141.1 (C19), 123.8, 122.2, 116.1, 115.6 (C20), 111.8, 73.0 (C11), 71.1 (C14), 57.6 (C4), 45.4 (C9), 44.5 (C13), 43.7(C12), 42.0 (C5), 36.9 (C6), 36.8 (C10), 34.9 (C2), 34.4 (C22), 30.5 (C8), 28.9 (C7), 27.0 (C18), 24.9 (C1), 16.5 (C16), 14.9 (C15), 12.0 (C17); HR-MS (ESI): Calcd for C29H36F3NO5S (M – H+): 578.2193; Found: 578.2195.
22-[(6-trifluoromethyl benzoxazole-2-yl) thio] deoxy pleuromutilin (compound 63)
Yield: 88%; Purity of compound was 97.2% (determined by RP HPLC at 254 nm, tR = 10.7 min, acetonitrile: water = 80: 20); 1H NMR (600 MHz, CDCl3) δ 7.63 (d, J = 1.6 Hz, 1H), 7.55 (d, J = 8.3 Hz, 1H), 7.50 (dd, J = 8.3, 1.6 Hz, 1H), 6.35 (H19, dd, J = 17.4, 11.0 Hz, 1H), 5.73 (H14, d, J = 8.5 Hz, 1H), 5.20 (H20, dd, J = 11.0, 1.5 Hz, 1H), 5.09 (H20, dd, J = 17.4, 1.5 Hz, 1H), 4.01 − 3.92 (H22, m, 2H), 3.27 (H11, d, J = 6.2 Hz, 1H), 2.24 − 1.96 (H2, H4, H10, H13, m, 5H), 1.71 − 1.56 (H6, H8, 11-OH, m, 3H), 1.51 − 1.38 (H1, H7, m, 3H), 1.37 (H15, s, 3H), 1.33 − 1.25 (H7, H13, m, 2H), 1.05 (H8, H18, s, 4H), 0.80 (H17, d, J = 7.0 Hz, 3H), 0.70 (H16, d, J = 7.1 Hz, 3H). 13C NMR (151 MHz, CDCl3) δ 217.4 (C3), 166.7 (C21), 163.1, 150.1, 148.0, 141.5, 138.8 (C19), 121.8, 117.3, 115.3 (C20), 109.1, 74.5 (C11), 70.5 (C14), 58.2 (C4), 45.5 (C9), 44.4 (C13), 43.9 (C12), 41.9 (C5), 36.8 (C6), 36.0 (C10), 34.9 (C2), 34.5 (C22), 31.7 (C8), 30.4 (C7), 26.8 (C18), 24.8 (C1), 16.7 (C16), 14.8 (C15), 11.5 (C17); HR-MS (ESI): Calcd for C29H36F3NO5S (M – H+): 578.2193; Found: 578.2194.
In vitro efficacy of pleuromutilin derivatives
Minimal inhibitory concentration (MIC) testing and minimum bactericidal concentration (MBC) testing
The minimal inhibitory concentration (MIC) and minimum bactericidal concentration (MBC) values of the new pleuromutilin derivatives that were produced against certain bacterial strains were determined. The strains used are listed in . Tiamulin, valnemulin and vancomycin were used as positive control drugs. According to the Clinical and Laboratory Standards Institute (CLSI), the MIC and MBC values were manipulated using broth dilution. Each compound to be measured was dissolved in a solution of 95% deionised water, 2.5% dimethyl sulfoxide (DMSO), and 2.5% Tween-80 with a concentration of 320 µg/mL. All wells of the 96-well plate were inoculated with the working bacterial solution, resulting in a final inoculum density of 5 × 105 CFU/mL. Three parallel experiments were carried out for each concentration of these compounds and positive controls. After 24 h of incubation at 37 °C, the lowest inhibitory concentration of the sample on the observable growth of the tested bacteria was recorded as the MIC value.
After the MIC results was obtained, the 96-well plates were incubated at 37 °C for 24 h. 25 µL of the bacterial solution from the wells with no obvious bacterial growth was inoculated on MH agar plates. Then, the inoculated MH agar plates were further cultured at 37 °C for 24 h. The MBC was identified as the lowest concentration of these tested compounds that was able to cause a 99.9% reduction in viable bacterial cells on agar platesCitation30.
Constant concentration time-kill curves
To assess the antibacterial activity of compound 50 and compound 57 against MRSA at a constant drug concentration, time-kill curve experiments were performed according to the MIC value. The concentrations of compound 50 and compound 57 were equal to 1 × MIC, 2 × MIC, 4 × MIC, 8 × MIC, 16 × MIC and 32 × MIC as described. MRSA (ATCC 43300) was incubated in MH broth at 37 °C for 4.5 h and diluted to 1 × 106 CFU/mL in MH broth. All the samples were incubated in an oscillating thermostatic at 37 °C. The mixture (0.1 ml) was extracted to 0.9 ml of sterile saline (0.9%) at 0, 3, 6, 9 and 24 h respectively. The samples were serially diluted 10 times in sterile saline (0.9%) and inoculated onto agar plates. The results were counted after incubation at 37 °C for 24 h. Three independent experiments were performed. Time-kill curves were generated by graphing the log10 CFU/mL versus time and determining the change in bacterial concentration.
Determination of the post-antibiotic effect (PAE)
The PAE of compound 50 and compound 57 on MRSA (ATCC 43300) was determined using MH broth dilution method. The final concentration of MRSA (ATCC 43300) was 1 × 106 CFU/mL by dilution with MH broth. Compounds 50 and 57 were mixed into the solution separately at a final concentration of 2 × MIC and 4 × MIC, respectively. The test groups were incubated at a 37 °C constant temperature vibration incubator for 1 h and 2 h. The test drugs were removed by diluting 1000 times with the preheated MH broth. After dilution, both samples were collected and incubated in a 37 °C constant temperature incubator a 95% humidified atmosphere and 5% CO2. 0.1 ml of sample from each culture was 10 times diluted in sterile saline, and inoculated on MH agar plates at 0 h, 2 h, 4 h, 6 h, and 8 h. The amounts of the colonies were recorded after incubation at 37 °C for 24 h. Three independent experiments were performed. Bacterial growth curves were constructed by plotting the log10 CFU of bacteria counts versus time. The PAE value was calculated by the following equation (PAE = TA – TC) and presented after one hour (TA is the time required for the increase of 1 log10 CFU/mL from the removal of the antimicrobial, and TC represents the time required for bacterial cultures not treated with an antimicrobial to increase 1 log10 CFU/mL)Citation31.
Neutropenic murine thigh infection model
A thigh infection model experiment was performed using female ICR/Swiss mice that were six weeks old, specific pathogen-free (SPF), and weighed 22 to 28 g. Cyclophosphamide was injected intraperitoneally at 4 days (150 mg/kg) and 1 day (100 mg/kg) before infection to induce neutropenia (<0.1 × 109/L). MH broth was used to dilute a logarithmic-phase culture solution of MRSA to a final concentration of approximately 1 × 107 CFU/mL. After dilution, each mouse thigh was injected with 0.1 ml MH broth 2 h before dosing to generate a thigh infection model caused by MRSA. Then these mice were randomly divided into 3 groups (6 per group) and intravenously injected compound 50 (20 mg/kg), tiamulin (20 mg/kg) and sterile normal saline as two experimental groups and one control group respectively. Mice given intravenous injections were euthanized after 24 h, and thigh tissue was aseptically extracted, placed in pre-weighed tubes (containing 1.5 ml of cooled saline), weighed, and homogenised. Thigh tissue homogenates were serially plated onto MH agar plates after being diluted ten times. After 24 h of incubation at 37 °C, the resulting bacterial colonies on each plate was manually counted.
The protocol for this study was reviewed and approved by the Institutional Animal Care and Use Committee of the South China Agricultural University (Approval number: 2022C084).
Cytotoxicity assay
MTT assay was used to assess the cell viability of tiamulin and the target compounds on the viability of RAW 264.7 macrophages. RAW 264.7 cells were purchased from the National Collection of Authenticated Cell Cultures (Shanghai, China). DMEM supplemented with 10% FBS containing RAW 264.7 cells at a density of 1.0 × 105 cells per well were added into 96-well plates and incubated at 5% CO2 and 37 °C for 24 h. Finally, the absorbance at 490 nm was recorded using a microplate spectrophotometer (BIO-TEK Instrument Inc, USA). The method was performed as described in referenceCitation32.
Molecular modelling
To reveal the binding mechanisms of synthesised pleuromutilin analogues, docking was conducted on the S. aureus 50S ribosomal complex with tiamulin (PDB ID code: 1XBP). The peptidyl transferase centre (PTC), which consists mostly of all residues within 40 Å surrounding the tiamulin in 1XBP. The binding site of tiamulin in 1XBP was set to the docking position. All compounds were prepared with Avogadro 1.1.1. The crystal structure was acquired from RCSB Protein Data Bank (http://www.rcsb.org). Docking was carried out by Pymol and AutoDockCitation33.
Supplemental Material
Download PDF (7.4 MB)Disclosure statement
The authors declare that they have no known competing financial interests or personal relationships that could have appeared to influence the work reported in this paper.
Additional information
Funding
References
- David MZ, Daum RS. Community-associated methicillin-resistant Staphylococcus aureus: epidemiology and clinical consequences of an emerging epidemic. Clin Microbiol Rev. 2010;23(3):616–687.
- Tenover FC, McDougal LK, Goering RV, Killgore G, Projan SJ, Patel JB, Dunman PM. Characterization of a strain of community-associated methicillin-resistant Staphylococcus aureus widely disseminated in the United States. J Clin Microbiol. 2006;44(1):108–118.
- Qin HL, Liu J, Fang WY, Ravindar L, Rakesh KP. Indole-based derivatives as potential antibacterial activity against methicillin-resistance Staphylococcus aureus (MRSA). Eur J Med Chem. 2020;194:112245.
- Washer P, Joffe H. The "hospital superbug": social representations of MRSA. Soc Sci Med. 2006;63(8):2141–2152.
- Ippolito G, Leone S, Lauria FN, Nicastri E, Wenzel RP. Methicillin-resistant Staphylococcus aureus: the superbug. Int J Infect Dis. 2010;14(Suppl 4):S7–S11.
- Huffman BJ, Shenvi RA. Natural products in the "Marketplace": interfacing synthesis and biology. J Am Chem Soc. 2019;141(8):3332–3346.
- Kavanagh F, Hervey A, Robbins WJ. Antibiotic substances from basidiomycetes: VIII. Pleurotus Multilus (Fr.) Sacc. and pleurotus passeckerianus pilat. Proc Natl Acad Sci USA. 1951;37(9):570–574.
- Kavanagh F, Hervey A, Robbins WJ. Antibiotic substances from basidiomycetes: IX. Drosophila Subtarata. (Batsch Ex Fr.) Quel. Proc Natl Acad Sci USA. 1952;38(7):555–560.
- Hogenauer G. The mode of action of pleuromutilin derivatives. Location and properties of the pleuromutilin binding site on Escherichia coli ribosomes. Eur J Biochem. 1975;52(1):93–98.
- Woodford N, Afzal-Shah M, Warner M, Livermore DM. In vitro activity of retapamulin against Staphylococcus aureus isolates resistant to fusidic acid and mupirocin. J Antimicrob Chemother. 2008;62(4):766–768.
- van Duijkeren E, Greko C, Pringle M, Baptiste KE, Catry B, Jukes H, Moreno MA, Pomba MCMF, Pyörälä S, Rantala M, et al. Pleuromutilins: use in food-producing animals in the European Union, development of resistance and impact on human and animal health. J Antimicrob Chemother. 2014;69(8):2022–2031.
- Daum RS, Kar S, Kirkpatrick P. Retapamulin. Nat Rev Drug Discov. 2007;6(11):865–866.
- Lee YR, Jacobs KL. Leave it to Lefamulin: a pleuromutilin treatment option in community-acquired bacterial pneumonia. Drugs. 2019;79(17):1867–1876.
- Luo J, Yang QE, Yang YY, Tang YZ, Liu YH. Design, synthesis, and structure-activity relationship studies of novel pleuromutilin derivatives having a piperazine ring. Chem Biol Drug Des. 2016;88(5):699–709.
- Jin Z, Wang L, Gao H, Zhou YH, Liu YH, Tang YZ. Design, synthesis and biological evaluation of novel pleuromutilin derivatives possessing acetamine phenyl linker. Eur J Med Chem. 2019;181:111594.
- Wang Q, Liu J, Zhou Z-D, Zhou K-X, Li F, Zhang Q-W, Wang S-K, Wang W, Jin Z, Tang Y-Z, et al. Design, synthesis, biological evaluation and molecular docking studies of novel pleuromutilin derivatives containing nitrogen heterocycle and alkylamine groups. J Enzyme Inhib Med Chem. 2022;37(1):2078–2091.
- Boschelli DH, Connor DT, Bornemeier DA, Dyer RD, Kennedy JA, Kuipers PJ, Okonkwo GC, Schrier DJ, Wright CD. 1,3,4-Oxadiazole, 1,3,4-thiadiazole, and 1,2,4-triazole analogs of the fenamates: in vitro inhibition of cyclooxygenase and 5-lipoxygenase activities. J Med Chem. 1993;36(13):1802–1810.
- Kohli P, Srivastava SD, Srivastava SK. Synthesis and biological activity of mercaptobenzoxazole based thiazolidinones and their arylidenes. Jnl Chinese Chemical Soc. 2007;54(4):1003–1010.
- Refaey SAM, Taha F, Abd El-Malak AM. Inhibition of stainless steel pitting corrosion in acidic medium by 2-mercaptobenzoxazole. Appl Surf Sci. 2004;236(1–4):175–185.
- Kakkar S, Tahlan S, Lim SM, et al. Benzoxazole derivatives: design, synthesis and biological evaluation. Chem Cent J. 2018;12(1):92.
- Sattar R, Mukhtar R, Atif M, Hasnain M, Irfan A. Synthetic transformations and biological screening of benzoxazole derivatives: a review. J Heterocycl Chem. 2020;57(5):2079–2107.
- Dorababu A. Synthesis, pharmacological evaluation and structure-activity relationship of recently discovered enzyme antagonist azoles. Heliyon. 2020;6(4):e03656.
- Muntoni F, Tejura B, Spinty S, Roper H, Hughes I, Layton G, Davies KE, Harriman S, Tinsley J. A Phase 1b trial to assess the pharmacokinetics of ezutromid in pediatric duchenne muscular dystrophy patients on a balanced diet. Clin Pharmacol Drug Dev. 2019;8(7):922–933.
- Oehlers L, Mazzitelli CL, Brodbelt JS, Rodriguez M, Kerwin S. Evaluation of complexes of DNA duplexes and novel benzoxazoles or benzimidazoles by electrospray ionization mass spectrometry. J Am Soc Mass Spectrom. 2004;15(11):1593–1603.
- Li B, Zhang Z, Zhang J-F, Liu J, Zuo X-Y, Chen F, Zhang G-Y, Fang H-Q, Jin Z, Tang Y-Z, et al. Design, synthesis and biological evaluation of pleuromutilin-Schiff base hybrids as potent anti-MRSA agents in vitro and in vivo. Eur J Med Chem. 2021;223:113624.
- Azizian H, Esmailnejad A, Vavsari VF, Mahernia S, Amanlou M, Balalaie S. Pantoprazole derivatives: synthesis, urease inhibition assay and in silico molecular modeling studies. Chemistry. 2020;5(15):4580–4587.
- Kavaliauskas P, Grybaite B, Mickevicius V, Petraitiene R, Grigaleviciute R, Planciuniene R, Gialanella P, Pockevicius A, Petraitis V. Synthesis, ADMET properties, and in vitro antimicrobial and antibiofilm activity of 5-Nitro-2-thiophenecarbaldehyde N-((E)-(5-Nitrothienyl)methylidene)hydrazone (KTU-286) against staphylococcus aureus with defined resistance mechanisms. Antibiotics. 2020;9(9):612.
- Ahmad I, Huang L, Hao H, Sanders P, Yuan Z. Application of PK/PD modeling in veterinary field: dose optimization and drug resistance prediction. Biomed Res Int. 2016;2016:5465678–12.
- Zhanel GG, Craig WA. Pharmacokinetic contributions to postantibiotic effects. Focus on aminoglycosides. Clin Pharmacokinet. 1994;27(5):377–392.
- Liu J, Zhang G-Y, Zhang Z, Li B, Chai F, Wang Q, Zhou Z-D, Xu L-L, Wang S-K, Jin Z, et al. Design, synthesis, in vitro and in vivo evaluation against MRSA and molecular docking studies of novel pleuromutilin derivatives bearing 1, 3, 4-oxadiazole linker. Bioorg Chem. 2021;112:104956.
- MacKenzie FM, Gould IM. The post-antibiotic effect. J Antimicrob Chemother. 1993;32(4):519–537.
- Jin Z, Yang YZ, Chen JX, Tang YZ. Inhibition of pro-inflammatory mediators in RAW264.7 cells by 7-hydroxyflavone and 7,8-dihydroxyflavone. J Pharm Pharmacol. 2017;69(7):865–874.
- Trott O, Olson AJ. Software news and update AutoDock Vina: improving the speed and accuracy of docking with a new scoring function, efficient optimization, and multithreading. J Comput Chem. 2010;31(2):455–461.