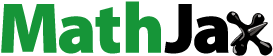
Abstract
Protein tyrosine phosphatase 1B (PTP1B) is a key factor and regulator of glucose, lipid metabolism throughout the body, and a promising target for treatment of type 2 diabetes mellitus (T2DM). Gynostemma pentaphyllum is a famous oriental traditional medicinal herbal plant and functional food, which has shown many beneficial effects on glucose and lipid metabolism. The aim of the present study is to assess the inhibitory activity of five new and four known dammarane triterpenoids isolated from the hydrolysate product of total G. pentaphyllum saponins. The bioassay data showed that all the compounds exhibited significant inhibitory activity against PTP1B. The structure-activity relationship showed that the strength of PTP1B inhibitory activity was mainly related to the electron-donating group on its side chain. Molecular docking analysis suggested that its mechanism may be due to the formation of competitive hydrogen bonding between the electron-donating moiety and the Asp48 amino acid residues on the PTP1B protein.
Introduction
Diabetes mellitus is a chronic metabolic disease caused by insufficient insulin secretion or insulin resistance, with hyperglycaemia as the typical clinical symptom, which can lead to a series of complications such as retinopathy and cardiovascular disease, and even life-threatening in severe casesCitation1. Type 2 diabetes mellitus accounts for the majority of diabetic patients, mainly due to insulin resistance, which reduces the sensitivity of target cells to insulin and the uptake of glucose capacity, which ultimately leads to elevated glucose levels in the bloodCitation1. Although hypoglycaemic agents have been widely used, these agents have a great many limitations, including adverse effects and high rates of secondary failureCitation2. With the continuous research on the physiological function of insulin, a number of new targets for the treatment of diabetes have been discovered. Among them, protein-tyrosine phosphatase 1B (PTP1B) is an important negative regulator of insulin signalling and plays an important role in the process of insulin signallingCitation3. The sensitivity of target cells to insulin can be improved by inhibiting the activity of PTP1BCitation4. Therefore, it has been suggested that inhibition of PTP1B is an effective therapeutic approach to treatment of type-2 diabetes mellitus and obesityCitation5–8.
Gynostemma pentaphyllum (Thunb.) Makino (Cucurbitaceae), usually called “Jiaogulan” in China, is also widely distributed in Asia including Japan, Korea and other southeast Asian countriesCitation9–11. In China, G. pentaphyllum has been prescribed in Chinese medicines to treat various diseases, especially diabetes, referred as “thirsty disease” for a long history from the Ming Dynasty (1368–1644 AD)Citation12,Citation13 and mainly cultivated and became an important income resource for local farmers in Shaanxi, Guangxi, Fujian, Guizhou and Hubei province, todayCitation14,Citation15. Previous phytochemical investigations indicated that the dammarane triterpenoid and flavonoid glycosides were the major constituents in G. pentaphyllumCitation16,Citation17. To date, more than 180 gypenosides, along with flavonoids were isolated from the plant exhibiting various biological activities such as antioxidant, anti-inflammatory, antitumor, antidiabetic and hepatoprotective effectsCitation18–22. The structure-activity relationship studies indicated that as the presence of sugar moieties in the saponins, aglycones were more effective than glycosidesCitation23. Previously, it was reported in the literature that some triterpenoids isolated from the hydrolysis products of G. pentaphyllum total saponins were found to have inhibitory activity against PTP1BCitation24,Citation25.
In the present study, to further search for bioactive aglycones, a hydrolyzate of G. pentaphyllum total saponins was investigated, and led to the isolation of five new triterpene derivative 1–5, together with four known analogues 6–9. Their structures were elucidated using spectroscopic methods including HR-ESI-MS, 1D and 2D NMR or X-ray crystallography. Among them, compound 1 was an unpredictable new skeleton dammarane triterpenoids. In addition, the inhibitory activity of these triterpenoids against PTP1B were evaluated.
Materials and methods
General experimental procedures
In the separation process, the column chromatography as performed by silica-gel (100–200 and 300–400 mesh, Qingdao Marine Chemical Co., Qingdao, China), and Sephadex LH-20 (GE Healthcare, Marlborough, MA). The TLC detection was carried by heating a silica gel plate (Qingdao Marine Chemical Co., Qingdao, China) spayed with 10% H2SO4 in ethanol. The 1D and 2D NMR spectra were recorded on a Varian INOVA AS 400 instrument (Agilent, Santa Clara, USA) with TMS as the internal standard. The chemical shift was shown in ppm while coupling constant J in Hz. Deuterated solvent (CD3Cl) was purchased from Sigma Aldrich for NMR analysis. HR-ESI-MS data were collected by a UPLC–Q/TOF-MS system including a 1290 Infinity II UPLC system (Agilent, USA) and an Agilent 6545 Q/TOF-MS system (Agilent, MA, USA). Optical rotations were measured by a JASCO P-1020 polarimeter (Kyoto, Japan). The single crystal X-ray diffraction data were obtained by a SuperNova, Dual, Cu at zero, AtlasS2 diffractometer (Rigaku, Japan). Besides, other analytical grade chemical solvent was obtained from Jinhuada Chemical Co., ltd. Guangzhou, P.R. China. Recombinant human PTP1B protein (ab51277) was purchased from Abcam (Shanghai) Trading Co., Ltd. All chemical reagents used in the enzymatic assays were produced by Laibo Chemicals Industries, Ltd.
Plant material
The total saponins extract (>80%, by UV) of G. pentaphyllum (Thunb.) Makino was purchased from Shaanxi Zhongxin biotech Co. Ltd., in 2019 and authenticated by Associate Professor Daopeng Tan (Pharmacognosy, Zunyi Medical University). A voucher specimen (No. 20190501) was deposited in the Herbarium of Zunyi Medical University.
Acid hydrolysis and isolation
The crude of saponins (500 g) of G. pentaphyllum was dissolved by MeOH (1000 ml) and then hydrolysed by adding 10% HCl (500 ml) at 50 °C for 8 hCitation13. After the evaporation under reduced pressure and filtration, the crude gypenoside aglycones residue (150 g) was obtained. The residue was subjected to silica gel column chromatography and eluted with petroleum ether/EtOAC (50:1–1:1) and CH2Cl2/MeOH (50:1–1:1) gradient to afford ten fractions (Fr. A-J). Fr. B (2.5 g) was fractionated by silica gel CC with n-hexane/EtOAC (50:1) to yield compound 1 (15 mg), 2 (23 mg), 6 (60 mg) and 7 (55 mg). Fr. C (1.8 g) was purified over silica gel CC with petroleum ether/acetone (40:1) to afford compound 3 (12 mg), 8 (45 mg) and 9 (50 mg). Fr. D (2.3 g) was separated by silica gel CC with n-hexane/acetone (30:1) to obtain compound 4 (11 mg) and 5 (17 mg).
Gypensapogenin I (1): White amorphous powder; [α]= −9.8° (c 0.08, MeOH); The 1H NMR (400 MHz, CD3Cl) and 13C NMR (100 MHz, CD3Cl) spectral data was shown in ; HR-ESI-MS: m/z 453.3368 [M + H]+ (calcd. 453.3368, for C30H45O3).
Table 1. 1H and 13C NMR data of compounds 1–5 (in CD3Cl, J in Hz at 400 MHz and 100 MHz).
Gypensapogenin II (2): White amorphous powder; [α]= −38.4° (c 0.1, MeOH); The 1H NMR (400 MHz, CD3Cl) and 13C NMR (100 MHz, CD3Cl) spectral data was shown in ; HR-ESI-MS: m/z 439.3577 [M + H]+ (calcd. 439.3576, for C30H47O2).
Gypensapogenin III (3): White amorphous powder; [α]= −24.8° (c 0.1, MeOH); The 1H NMR (400 MHz, CD3Cl) and 13C NMR (100 MHz, CD3Cl) spectral data was shown in ; HR-ESI-MS: m/z 492.4057 [M + NH4]+ (calcd. 492.4053, for C30H54O4N).
Gypensapogenin IV (4): White amorphous powder; [α]= −37.4° (c 0.1, MeOH); The 1H NMR (400 MHz, CD3Cl) and 13C NMR (100 MHz, CD3Cl) spectral data was shown in ; HR-ESI-MS: m/z 473.3635 [M-H2O + H]+ (calcd. 473.3631, for C30H49O4).
Gypensapogenin V (5): White amorphous powder; [α]= −19.4° (c 0.1, MeOH); The 1H NMR (400 MHz, CD3Cl) and 13C NMR (100 MHz, CD3Cl) spectral data was shown in ; HR-ESI-MS: m/z 473.3650 [M + H]+ (calcd. 473.3631, for C30H49O4).
Protein tyrosine phosphatase 1B inhibition assay
The enzymatic assays of protein tyrosine phosphatase 1B (PTP1B) were referred Citation26. The PTP1B inhibitory activities of test compounds were examined in 96-well plates using the colorimetric method. Each test compounds and NaVO4 (positive control) were dissolved separately in DMSO and then diluted into gradient concentrations with MOPS solution (25 mM MOPS, 2 mM dl-dithiothreitol (DTT), 1 mM EDTA, 0.1 M NaCl, pH = 7.0). The reaction system was 100 μL: MOPS (25 mM, pH = 7.0), DTT (2 mM), EDTA (1 mM, pH = 7.0), NaCl (0.1 M), PTP 1B (25 nM), and disodium-4-nitrophenyl phosphate (pNPP, 2 mM), and the enzyme-catalysed reaction was terminated by the addition of 1.0 M NaOH after incubation at 37 °C for 30 min. The inhibition efficiency of the compounds on the enzymatic activity of PTP1B was assayed by detecting the amount of the hydrolysed product, p-nitrophenol (p-NP) at 405 nm using a microplate reader. The PTP1B inhibition activity was expressed as inhibition (%) and was calculated as EquationEquation (1)(1)
(1) :
(1)
(1)
Protein tyrosine phosphatase 1B inhibition kinetic assay
The kinetic behaviour of the active compounds against PTP1B and the corresponding inhibition constants (Ki values) were investigated using Lineweaver-Burk double reciprocal plots and Dixon plots. Different concentrations of p-NPP substrates (1, 2, 5 and 10 mM) were subjected to enzymatic reactions with various concentrations of active compounds (0, 0.5, 1.0 and 5.0 μM for 18) and 100 nM PTP1B in 96-well plates. The absorbance of the reaction mixture was recorded every 3 min by a plate reader. The enzymatic velocity of the enzyme reaction was calculated based on the time-Δabs plot. All assays were performed in triplicate.
Molecular docking study
Dammarane triterpenoids with optimal PTP1B inhibitory activity was selected for molecular docking with PTP1B protein. The PTP1B protein crystal structure was downloaded from the AlphaFold Protein Structure Database (https://alphafold.ebi.ac.uk) and modified by the Autodock tools 1.5.6 softwareCitation27,Citation28. The PTP1B protein molecule was optimised for dehydrogenation, hydrogenation, etc., and defined its active site including Tyr46, Asp48, Phe182, Cys215, Ser216, Ile219, Arg221, Met258, Gln262, Gln266Citation29. The 3D structure of preferred dammarane triterpenoids were built by ChemBioDraw Ultra14.0 and then converted to PDBQT coordinateds using AutoDockTools. The rotatable bonds of the ligand were assigned through AutoDock Tools, and the ligand docking was performed by the AutoDock Vina. Construct 2D maps of protein-ligand interactions using LigPlot software to analyse the interaction forces between protein and ligand binding. Construct 3D maps of protein-ligand interactions using PyMOL software to show the binding sites between proteins and ligands.
ADME analysis
SwissADME which is a web-based tool was applied for ADME analysis. SwissADME helped us to predict the ADME parameters, pharmacokinetic qualities, drug-like properties and medicinal chemistry friendliness of dammarane triterpenoids of G. pentaphyllum by utilising its ability to compute physicochemical descriptorsCitation30.
Results and discussion
Structure elucidation
Five unreported dammarane triterpenoids 1–5 together with four known analogues 6–9 were isolated from the hydrolyzate of total G. pentaphyllum saponins. Structures of undescribed triterpenoids 1–5 were elucidated on the basis of spectral data or single crystal X-ray diffraction data interpretation, whereas the structures of four known compounds 6–9 were confirmed by comparing their spectral data in literatureCitation23.
Gypensapogenin I (1) was isolated as a white amorphous powder with a molecular formula of C30H44O3 as determined by the HR-ESI-MS at m/z 453.3368 [M + H]+, (calcd. for 453.3368) and NMR data, requiring ten degrees of unsaturation (). The 1H NMR spectrum showed signals due to an olefinic proton (δ: 5.87), two oxygen-bearing protons (δ: 4.38, 3.83) and six methyl groups (δ: 1.25, 1.23, 1.12, 0.86, 0.83, 0.82). The 13C NMR spectrum showed 30 carbon resonances and demonstrated the presence of an unsaturated ketone by the signals at δ: 201.0, 149.9, 132.8, 130.0, 106.8. So, compound 1 was determined as a sapogenin from the hydrolized saponins of G. pentaphyllum. Furthermore, the NMR spectrum of compound 1 closely resembled that of Gypensapogenin A and B in the high fieldCitation23. Those suggested that the structure of compound 1 was similar to Gypensapogenin A and B with a 7-membered cycle, an oxygen bridge and a double bond. In the HMBC spectrum, the long-range correlations from δ: 5.88 (H-22) to δ: 201.0 (C-20), 149.4 (C-21), 19.0 (C-23) and 31.9 (C-24), δ:2.15 (H-23) to δ: 149.4 (C-21), 106.8 (C-22), 31.9 (C-24) and 74.3 (C-25), δ:1.25, 1.23 (H-26, 27) to δ: 74.3 (C-25), 31.9 (C-24), respectively, indicated the existence of a dihyro-pyran cycle with two methyl groups in the side chain. With the help of HSQC, COSY and NOESY data, compound 1 was elucidated as and and named Gypensapogenin I. The chemical structure was finally confirmed by the single crystal X-ray diffraction analysis (flack parameter −0.7 (5), ). Additionally, the structure of compound 1 was demonstrated as an unprecedented dammarane triterpene skeleton characterised as C-21 was inserted into the side chain. The possible formation of Gypensapogenin I was deduced as shown in .
Crystal Data for Gypensapogenin I, C30H44O3 (M = 452.65 g/mol), orthorhombic, space group P212121 (no. 19), a = 6.1257(6) Å, b = 9.1748(10) Å, c = 45.065(5) Å, V = 2532.7(5) Å3, Z = 4, T = 150.00(10) K, μ(Cu Kα) = 0.575 mm−1, Dcalc = 1.187 g/cm3, 7830 reflections measured (3.922° ≤ 2Θ ≤ 148.812°), 4370 unique (Rint = 0.0546, Rsigma = 0.0659) which were used in all calculations. The final R1 was 0.1277 (I > 2σ(I)) and wR2 was 0.3306 (all data).
Gypensapogenin II (2) was isolated as a white amorphous powder with a molecular formula of C30H46O2 as determined by the HR-ESI-MS at m/z 439.3577 [M + H]+, (calcd. for 439.3576) and NMR data, requiring eight degrees of unsaturation (). The 1H NMR spectrum showed signals due to an olefinic proton (δ: 6.02), one oxygen-bearing protons (δ: 3.17) and seven methyl groups (δ: 2.26, 1.85, 0.95, 0.95, 0.89, 0.82, 0.75). The 13C NMR spectrum showed 30 carbon resonances and demonstrated the presence of a ketone carbonyl at the signals of δ: 197.4 and a pair of alkenyl carbon signals at δ: 129.4 and 144.6. So, compound 2 was elucidated to be a triterpene, which was very similar with Gypensapogenin C in the 1H and 13C NMR spectrum dataCitation23. Analysis of HMBC, HMQC, COSY and NOESY spectrum led to the elucidation of the structure of compound 2 named Gypensapogenin II ( and ).
Gypensapogenin III (3) was isolated as a white amorphous powder with a molecular formula of C30H50O4 as determined by the HR-ESI-MS at m/z 492.4057 [M + NH4]+, (calcd for 492.4053) and NMR data, requiring six degrees of unsaturation (). In the 1H NMR spectrum, seven methyl group signals at δ:1.65, 1.59, 1.23, 1.03, 0.98, 0.93, 0.79, one olefinic proton at δ:5.30, and one oxygen bearing proton at δ:3.43 were observed. And in the 13C NMR spectrum, 30 carbon resonances signals were observed. Among them, one ester carbonyl, a group of allylic and two oxygen bearing carbons were presented at the signals of δ: 181.0, 132.6, 126.4, 79.2 × 2, respectively. On the basis of literatureCitation25,Citation31, the 1H and 13C NMR spectrum data of compound 3 were very similar to that of (3β,20S,23R)-3,20,23-trihydroxydammar-24-en-21-oic acid-21,23-lactone except for the changes in the signals of H-24 and C-23. Interpretation of HMBC, HMQC, COSY and NOESY spectrum, the structure of compound 3 was identified as and and named Gypensapogenin III.
Gypensapogenin IV (4) was isolated as a white amorphous powder with a molecular formula of C30H50O5 as determined by the HRESIMS at m/z 473.3635 [M-H2O + H]+, (calcd for 473.3631) and NMR data, requiring six degrees of unsaturation (). Compound 4 exhibited NMR spectrum features similar to those of compound 3 except for the inexistence of allylic carbons replaced by more one ketone carbonyl in the 1H and 13C NMR spectrum. On the basis of HMBC, HMQC, COSY and NOESY spectrum, the structure of compound 4 was elucidated as and and named Gypensapogenin IV.
Gypensapogenin V (5) was isolated as a white amorphous powder with a molecular formula of C30H48O4 as determined by the HRESIMS at m/z 473.3650 [M + H]+, (calcd for 473.3631) and NMR data, requiring seven degrees of unsaturation (). Compound 5 exhibited the NMR spectrum features similar to those of compound 3 except that there was one less oxycarbon atom and one more carbonyl carbon atom in the 1H and 13C NMR spectrum. On the basis of HMBC, HMQC, COSY and NOESY spectrum, the structure of compound 5 was elucidated as and and named Gypensapogenin V.
Biological evaluation
The pNPP method was used to examine their PTP1B inhibitory activities of these nine dammarane derivatives isolated from G. pentaphyllum. The results showed that all these nine compounds exhibited some PTP1B inhibitory activity and compounds 2, 3, 4, 9 showed more inhibitory activity against PTP1B than the positive control (NaVO4) ().
Comparing the structures of these compounds with their inhibitory activities against PTP1B, we can find such conformational relationships. firstly, the presence of -OH in C-3 is favourable for the inhibitory activity of PTP1B, however, in compounds 1, 6, 7, the spatial site-blocking formed by the seven-membered ring A reduces their activities. Secondly, in the side chain, the presence of electron-donating (–OH, –COOH) is favourable for their inhibitory activity, whereas the presence of electron-withdrawing groups such as carbonyl group, double bond, etc. is unfavourable for its inhibitory activity.
Kinetic characterisation
Lineweaver − Burk double reciprocal plot and Dixon plot were further applied to study the kinetic behaviour of compounds 3, 4 and 9 against PTP1B. As shown in , the maximum rate of the PTP1B catalysed reaction was not changed, and the Km was increased with the increase of concentrations. Therefore, compounds 3, 4 and 9 are competitive inhibitors against PTP1B. In the Dixon plot method (), the Ki values of 3, 4 and 9 were determined to be 1.16 ± 0.47 μM, 2.44 ± 0.09 μM and 5.58 ± 0.70 μM, respectively. The relatively lower Ki value of 3 makes it to be a promising competitive PTP1B inhibitor.
Molecular docking analysis
To further predict the PTP1B inhibitory activity of optimal dammarane triterpenoid, molecular docking was used to simulate the binding modes between PTP1B protein and the 9 dammarane-type triterpenoids from G. pentaphyllum, respectively. After docking, the conformational cluster with the lowest binding free energy is shown in the complex of the docked compounds with PTP1B protein (, ). The molecular docking results showed that all the triterpenoids had good affinity with the PTP1B protein (affinity ≤ −7.1 kcal/mol, ). Moreover, the ligand-protein residue interactions were analysed, where hydrogen attached to the hydroxyl or the oxygen on the heterocyclic ring in all ligands except compounds Gypensapogenin A-C, showed hydrogen-bonding interaction with Arg79, Asp48, Ile281, Ser80, His208, or Ser205 active sites of residues. As shown in , Gypensapogenin III was found to have the strongest PTP1B inhibitory activity (2.34 ± 0.24 μM). In , hydrogen-binding between the hydroxyl group on the side chain of Gypensapogenin III and Asp48 protein residues were observed with a bond distance of 3.06 Å, in addition to several hydrophobic interactions between Gypensapogenin III and other protein residues. PTP1B protein contains 435 amino acid residues, of which residues 30–278 are its catalytic domains. The active site, Asp48, can form hydrogen bonds with the phosphorylated tyrosine backbone during the catalytic process and play an important role in substrate binding and recognitionCitation32,Citation33. Hence, the competitive hydrogen bonding of Gypensapogenin III with Asp48 may be a key mechanism for its PTP1B inhibitory activity.
Table 2. Inhibitory activity of dammarane-type triterpenoids from G. pentaphyllum against PTP1B.
Table 3. The molecular docking results of dammarane-type triterpenoids from G. pentaphyllum against PTP1B.
ADME analysis
Drug-likeness was analysed to check whether the 9 dammarane-type triterpenoids from G. pentaphyllum possess favourable ADME (absorption, distribution, metabolism and excretion) properties. Drug-likeness compounds should have a good aqueous solubility, which can be predicted by three methods, including ESOL, (ALI) logS and (SILICOS-IT) logSCitation34. Orally drugs should obey Lipinski’s five rules: molecular weight (MW) not more than 500 g/mol, not more than 10 hydrogen bond acceptors, not more than 5 hydrogen bond donors, LogP value less than 5, and not less than 10 rotatable bondsCitation35, and compounds violating two or more of these rules are not orally active. The water solubility and intestinal membrane permeability of pharmaceuticals are their inherent qualities that affect oral absorption, according to the Biopharmaceutics Classifcation System (BCS) of the US Food and Drug Administration. Due to its function in drug dissolving within the gastric lumina, solubility is one of the important parameters that determine the oral bioavailability of drug moleculesCitation36. Drug-likeness analysis of 9 dammarane-type triterpenoids from G. pentaphyllum was listed in . All the compounds were specific in nature (zero alerts of PAINS). Among them, Gypensapogenin III showed good binding affinity against PTP1B with high drug-likeness parameters such as moderate solubility, and no excretion problems as there is no pharmacokinetic P-gp (permeable glycoprotein) interference and non-inhibitor of CYP enzymes.
Table 4. ADME profile of dammarane-type triterpenoids from G. pentaphyllum as predicted by SwissADME.
Conclusion
In the present study, five new and four reported dammarane-type triterpenoids were isolated and identified from the hydrolysed products of total G. pentaphyllum saponins. Among them, compound 1 (Gypensapogenin I) is a new skeleton compound characterised as C-21 was inserted into side chain. Similar to previous reports in the literatureCitation24,Citation25, all of these dammarane triterpenoid compounds showed good PTP1B inhibitory activity and the strength of PTP1B inhibitory activity was mainly related to the electron-donating group on its side chain. ADME analysis results suggested that Gypensapogenin III have a good binding affinity against PTP1B with high drug-likeness parameters. Its mechanism might be due to the formation of competitive hydrogen bonding between the electron-donating group and the Asp48 amino acid residues on the PTP1B protein.
Disclosure statement
The authors report no conflicts of interest.
Additional information
Funding
References
- Cloete L. Diabetes mellitus: an overview of the types, symptoms, complications and management. Nurs Stand. 2022;37(1):61–66.
- Sun Y, Tao Q, Wu X, Zhang L, Liu Q, Wang L. The utility of exosomes in diagnosis and therapy of diabetes mellitus and associated complications. Front Endocrinol. 2021;12:756581.
- Liu R, Mathieu C, Berthelet J, Zhang W, Dupret JM, Rodrigues Lima F. Human protein tyrosine phosphatase 1B (PTP1B): from structure to clinical inhibitor perspectives. Int J Mol Sci. 2022;23 (13):7027.
- Tamrakar AK, Maurya CK, Rai AK. PTP1B inhibitors for type 2 diabetes treatment: a patent review (2011–2014). Expert Opin Ther Pat. 2014;24(10):1101–1115.
- Nandi S, Saxena M. Potential inhibitors of protein tyrosine phosphatase (PTP1B) enzyme: promising target for type-II diabetes mellitus. Curr Top Med Chem. 2020;20(29):2692–2707.
- Hussain H, Green IR, Abbas G, Adekenov SM, Hussain W, Ali I. Protein tyrosine phosphatase 1B (PTP1B) inhibitors as potential anti-diabetes agents: patent review (2015–2018). Expert Opin Ther Pat. 2019;29(9):689–702.
- Teimouri M, Hosseini H, ArabSadeghabadi Z, Babaei-Khorzoughi R, Gorgani-Firuzjaee S, Meshkani R. The role of protein tyrosine phosphatase 1B (PTP1B) in the pathogenesis of type 2 diabetes mellitus and its complications. J Physiol Biochem. 2022;78(2):307–322.
- Tan D, Wang J, Cao L, Yang D, Lu Y, Wu D, Zhao Y, Wu X, Fan Q, Yang Z, et al. UDP-glycosyltransferases play a crucial role in the accumulation of alkaloids and sesquiterpene glycosides in Dendrobium nobile. Arabian J Chem . 2023;16(5):104673.
- Yin F, Hu L, Lou F, Pan R. Dammarane-type glycosides from Gynostemma pentaphyllum. J Nat Prod. 2004;67(6):942–952.
- Yang F, Shi H, Zhang X, Yu LL. Two novel anti-inflammatory 21-nordammarane saponins from tetraploid Jiaogulan (Gynostemma pentaphyllum). J Agric Food Chem. 2013;61(51):12646–12652.
- Zhou T, Cao L, Du Y, Qin L, Lu Y, Zhang Q, He Y, Tan D. Gypenosides ameliorate high-fat diet-induced nonalcoholic fatty liver disease in mice by regulating lipid metabolism. PeerJ. 2023;11:e15225.
- Razmovski-Naumovski V, Huang HW, Tran VH, Li GQ, Duke CC, Roufogalis BD. Chemistry and Pharmacology of Gynostemma pentaphyllum. Phytochem Rev. 2005;4(2–3):197–219.
- Zhang X, Shi G, Sun Y, Wu X, Zhao Y. Triterpenes derived from hydrolyzate of total Gynostemma pentaphyllum saponins with anti-hepatic fibrosis and protective activity against H(2)O(2)-induced injury. Phytochemistry. 2017;144:226–232.
- Wang J, Ha TKQ, Shi YP, Oh WK, Yang JL. Hypoglycemic triterpenes from Gynostemma pentaphyllum. Phytochemistry. 2018;155:171–181.
- Cui WY, Jin Y, Liu H, Zu ML, Zhai XF, Yang C, Gu YL, Cheng Y, Piao XL. Dammarane-type saponins from Gynostemma pentaphyllum and their cytotoxicities. Nat Prod Res. 2021;35(22):4433–4441.
- Lee C, Lee JW, Jin Q, Jang H, Jang HJ, Rho MC, Lee MK, Lee CK, Lee MK, Hwang BY. Isolation and characterization of dammarane-type saponins from Gynostemma pentaphyllum and their inhibitory effects on IL-6-induced STAT3 activation. J Nat Prod. 2015;78(5):971–976.
- Wang J, Yang JL, Zhou PP, Meng XH, Shi YP. Further new gypenosides from Jiaogulan (Gynostemma pentaphyllum). J Agric Food Chem. 2017;65(29):5926–5934.
- Hung TM, Thu CV, Cuong TD, Hung NP, Kwack SJ, Huh JI, Min BS, Choi JS, Lee HK, Bae K. Dammarane-type glycosides from Gynostemma pentaphyllum and their effects on IL-4-induced eotaxin expression in human bronchial epithelial cells. J Nat Prod. 2010;73(2):192–196.
- Huang TH, Razmovski-Naumovski V, Salam NK, Duke RK, Tran VH, Duke CC, Roufogalis BD. A novel LXR-alpha activator identified from the natural product Gynostemma pentaphyllum. Biochem Pharmacol. 2005;70(9):1298–1308.
- Circosta C, De Pasquale R, Occhiuto F. Cardiovascular effects of the aqueous extract of Gynostemma pentaphyllum Makino. Phytomedicine. 2005;12(9):638–643.
- Xie Z, Liu W, Huang H, Slavin M, Zhao Y, Whent M, Blackford J, Lutterodt H, Zhou H, Chen P, et al. Chemical composition of five commercial Gynostemma pentaphyllum samples and their radical scavenging, antiproliferative, and anti-inflammatory properties. J Agric Food Chem. 2010;58(21):11243–11249.
- Lin CC, Huang PC, Lin JM. Antioxidant and hepatoprotective effects of Anoectochilus formosanus and Gynostemma pentaphyllum. Am J Chin Med. 2000;28(1):87–96.
- Li N, Wu CF, Xu XY, Liu ZY, Li X, Zhao YQ. Triterpenes possessing an unprecedented skeleton isolated from hydrolyzate of total saponins from Gynostemma pentaphyllum. Eur J Med Chem. 2012;50:173–178.
- Zhang XS, Bi XL, Wan X, Cao JQ, Xia XC, Diao YP, Zhao YQ. Protein tyrosine phosphatase 1B inhibitory effect by dammarane-type triterpenes from hydrolyzate of total Gynostemma pentaphyllum saponins. Bioorg Med Chem Lett. 2013;23(1):297–300.
- Xu JQ, Shen Q, Li J, Hu LH. Dammaranes from Gynostemma pentaphyllum and synthesis of their derivatives as inhibitors of protein tyrosine phosphatase 1B. Bioorg Med Chem. 2010;18(11):3934–3939.
- Zhou X, Wang LL, Tang WJ, Tang B. Astragaloside IV inhibits protein tyrosine phosphatase 1B and improves insulin resistance in insulin-resistant HepG2 cells and triglyceride accumulation in oleic acid (OA)-treated HepG2 cells. J Ethnopharmacol. 2021;268:113556.
- Varadi M, Anyango S, Deshpande M, Nair S, Natassia C, Yordanova G, Yuan D, Stroe O, Wood G, Laydon A, et al. AlphaFold protein structure database: massively expanding the structural coverage of protein-sequence space with high-accuracy models. Nucleic Acids Res. 2022;50(D1):D439–D444.
- Jumper J, Evans R, Pritzel A, Green T, Figurnov M, Ronneberger O, Tunyasuvunakool K, Bates R, Žídek A, Potapenko A, et al. Highly accurate protein structure prediction with AlphaFold. Nature. 2021;596(7873):583–589.
- Montalibet J, Skorey K, McKay D, Scapin G, Asante-Appiah E, Kennedy BP. Residues distant from the active site influence protein-tyrosine phosphatase 1B inhibitor binding. J Biol Chem. 2006;281(8):5258–5266.
- Daina A, Michielin O, Zoete V. SwissADME: a free web tool to evaluate pharmacokinetics, drug-likeness and medicinal chemistry friendliness of small molecules. Sci Rep. 2017;7(1):42717.
- Gan M, Liu M, Gan L, Lin S, Liu B, Zhang Y, Zi J, Song W, Shi J. Dammarane glycosides from the root of Machilus yaoshansis. J Nat Prod. 2012;75(7):1373–1382.
- Barford D, Flint AJ, Tonks NK. Crystal structure of human protein tyrosine phosphatase 1B. Science. 1994;263(5152):1397–1404.
- Tautz L, Critton DA, Grotegut S. Protein tyrosine phosphatases: structure, function, and implication in human disease. Methods Mol Biol. 2013;1053:179–221.
- Sympli HD. Estimation of drug-likeness properties of GC-MS separated bioactive compounds in rare medicinal Pleione maculata using molecular docking technique and SwissADME in silico tools. Netw Model Anal Health Inform Bioinform. 2021;10(1):14.
- Lipinski CA, Lombardo F, Dominy BW, Feeney PJ. Experimental and computational approaches to estimate solubility and permeability in drug discovery and development settings. Adv Drug Deliv Rev. 2001;46(1–3):3–26.
- Azman M, Sabri AH, Anjani QK, Mustaffa MF, Hamid KA. Intestinal absorption study: challenges and absorption enhancement strategies in improving oral drug delivery. Pharmaceuticals. 2022;15(8):975.