Abstract
Isatin, known as 1H-indole-2,3-dione, was originally recognised as a synthetic molecule until its discovery in the fruits of the cannonball tree, Couroupita guianensis. It is naturally occurring in plants of the genus Isatis and serves as a metabolic derivative of adrenaline in humans. Isatin possesses significant pharmacological importance, and its synthetic versatility has prompted extensive interest in its derivative compounds due to their diverse biological and pharmacological properties. These derivatives represent a valuable class of heterocyclic compounds with potential applications as precursors for synthesizing numerous valuable drugs. In the pursuit of advancing our research on isatin hybrids, we investigate the utilisation of readily available hydrazonoindolin-2-one and isatin as starting materials for the synthesis of a wide range of analogues. Characterisation of the synthesized compounds was carried out through various analytical techniques. Furthermore, the obtained compounds were subjected to extensive testing to evaluate their anticancer and antimicrobial activities. Specifically, their efficacy against key proteins, namely Staphylococcus aureus protein (PDB ID: 1JIJ), Escherichia coli protein (PDB ID: 1T9U), Pseudomonas aeruginosa protein (PDB ID: 2UV0), and Acinetobacter baumannii protein (PDB ID: 4HKG), was examined through molecular docking calculations. Several molecules, such as 3, 4, 6, 16, and 19, displayed remarkable activity against the renal cancer cell line UO-31. Additionally, the results of antimicrobial activity testing revealed that compound 16 exhibited significant cytotoxicity against Candida albicans and Cryptococcus neoformans. Subsequently, ADME/T calculations were performed to gain insights into the potential effects and reactions of these molecules within human metabolism. This comprehensive study provides valuable insights into the potential pharmacological applications of isatin derivatives and underscores their significance in drug development.
Introduction
Isatin and its analogues exhibit remarkable versatility as synthetic compounds, serving as valuable precursors for the production of numerous pharmaceuticals currently available in the marketCitation1. Isatin, an indole derivative, occurs naturally in plants and constitutes a component of the secretion produced by the parotid gland of bufo frogs. Natural occurrences of substituted isatin compounds also exist; for instance, methoxyphenyl pentylisatin has been extracted from the tumorigenic plant Melochia tomentosa in the Caribbean. Furthermore, various substituted isatins have been discovered in fungi, with methylbuten-2-yl-isatin being isolated from Streptomyces albus and Chaetomium globosumCitation2–4. Derivatives of isatin constitute an essential faction of heterocyclic chemistry, and their biological potential has been an exciting field for their pharmacological properties. These activities include analgesic, anticancer, anti-inflammatory, antitubercular, antimicrobial, antifungal, and antiviralCitation5–7.
The isatin skeleton attached to heterocyclic rings is a privileged core in numerous molecules available in the market (). Interestingly, oxazolidine, thiazolidine, and other fused ring systems attached to isatin exhibited promising antitumor activities with different modes of actionCitation8–10. Despite the extensive progress on isatin and its derivatives, the development methods with pharmacological profiles and therapeutic safety represent a significant concern for many scientists. Due to the wide range of properties, heterocyclic chemistry has generated intensive interest over the last few years. Several reports on synthesizing heterocyclic molecules with anticancer and anti-microbial potential have been published so farCitation11,Citation12. Drug resistance along with clinical experience confirms that single targets might not always provide the preferred biological outcome in cancer chemotherapy. This is due to the development of resistance by self-modification of the target through mutation. Moreover, among the large number of compounds synthesized and screened for their potential in vitro antiproliferative agents, derivatives possessing the benzothiophene, 9H fluorine, and dihydrobenzodioxane structural description hybridized with isatin moiety have gained significant attention for current medicinal chemistsCitation13. In recent periods, the molecular hybrid approach has resulted in many new and interesting chemical entities with better antitumor activity, selectivity, and bridged side effects. A recent report suggested the use of 4-oxadiazoles and its derivatives as potent anticancer agents against MCF-7 cellsCitation14–16. Moreover, in another report by Gong and co-workers, the design, synthesis, and SAR of diarylthiosemicarbazide derivatives as potent antiproliferative agents against various cancer cell lines were publishedCitation17. Due to the major limitations such as multi-drug resistance and drug-related toxicity, several cutting-edge chemotherapies are unsuccessful. Therefore, the demand for newer and safe anticancer drugs with high efficiency and low toxicity for future cancer chemotherapy is highly desirable.
Theoretical calculations represent a vital computational approach employed to predict molecule activities and expedite the development and enhancement of active sites. Such calculations significantly shorten the process of designing more effective and potent molecules. In this context, diverse levels and base sets of ligand and metal complexes were employed in theoretical calculations to assess the activities of the molecules. Despite substantial progress in the synthesis of 1H-indole-2,3-dione analogues and our ongoing interest in developing an efficient methodology for synthesizing fine chemicals and pharmaceutically relevant heterocyclic compounds, this study focuses on synthesizing isatin scaffolds coupled with various privileged heterocyclic molecules to identify potent nuclei. Within the scope of this paper, we also investigate the interaction of these molecules with Aspergillus niger protein, identifying the most adherent molecules. Additionally, the activities of each studied molecule on the HF/6-31g basis set are probed using Gaussian calculations. These calculations provide valuable insights into their activities through various parameters. Furthermore, molecular docking calculations are employed to evaluate the activities of these molecules against specific proteins, including Staphylococcus aureus protein (PDB ID: 1JIJ), Escherichia coli protein (PDB ID: 1T9U), Pseudomonas aeruginosa protein (PDB ID: 2UV0), and Acinetobacter baumannii protein (PDB ID: 4HKG). Subsequently, ADME/T calculations are performed to assess the effects and reactions of these molecules within the context of human metabolism.
Results and discussion
Chemistry
The versatility of 1H-indole-2,3-dione underlies its wide range of pharmacological and synthetic applications, enabling the creation of diverse intermediates tailored for various chemical purposes. Incorporating pharmacophores into heterocycles has proven to be an effective strategy for generating biologically active analogues. The reaction of free amino groups with various aldehydes produces diverse precursors, with pyridines standing out as compounds possessing a wide range of biological applicationsCitation6,Citation18–20. For instance, when 3-pyridine carboxaldehyde was reacted with isatin hydrazide under mildly acidic conditions in ethanol as the solvent, it resulted in a moderate yield of 3-((pyridin-3-ylmethylene)-hydrazono)indolin-2-one 3 (see ). This molecule was reported earlier and found active against lung, MCF7, and hepatocellular cancer cell linesCitation21. The identification of the proposed molecule was corroborated by the presence of an exocyclic CH proton signal at 7.63 ppm and the disappearance of the aldehyde signal in the proton spectrum. Acknowledging the constraints of the established methods, we endeavoured to develop a novel and efficient approach. Consequently, employing ethanol in combination with a catalytic quantity of acetic acid yielded a satisfactory yield of 3-(((3-bromobenzo[b]thiophen-2-yl)methylene)-hydrazono)indolin-2-one 4. The 1H NMR spectrum of the synthesized molecules displayed the characteristic signal of the exocyclic CH at its designated position, and the aromatic protons exhibited their respective resonance regions.
Therefore, ethanol is used along with a catalytic amount of acetic acid afforded 3-(((3-bromobenzo[b]thiophen-2-yl)methylene)-hydrazono)indolin-2-one 4 in good yield. In the 1H NMR spectrum of synthesized molecules, the signal of exocyclic CH appears at the characteristic position; moreover, aromatic protons appeared in their respective regions. To increase the solubility of the synthesized molecule, 4 was treated with acetic anhydride with a catalytic amount of pyridine afforded 1-acetyl-3-(((3-bromobenzo-[b]thiophen-2-yl)methylene)-hydrazono)indolin-2-one 5 in high yield. Disappearance of NH and the presence of methyl group at δ = 2.66 ppm confirms the proposed molecule. The mass and FTIR data are also in accord with the proposed structures of compounds 4 and 5. To increase the efficacy and to find the most potent analogue, already reported 5-fluoro-3-((thiophen-2-ylmethylene)-hydrazono)indolin-2-one was treated with acetic anhydride afforded 1-acetyl-5-fluoro-3-((thiophen-2-yl)-methylene)hydrazono)indolin-2-one 6 in higher yield. It is reported earlier that incorporating a thiol in heterocycles has led to several analogues possessing interesting biological propertiesCitation22. Therefore, 2-(2-oxoindolin-3-ylidene)-hydrazinethiocarbohydrazide 7 was synthesized by a reaction of 1 with thiocarbohydrazide in ethanol under reflux (Scheme 1). The product was obtained in moderate yield and was confirmed by proton and mass spectrometry. The recent increase in chemotherapeutic agents and the advancement in proteomics have generated several new drug targets, which change the action of chemotherapy at the molecular level. Sugar molecules attached to heterocyclic moiety can enhance the bioavailability and solubility, which is vital for the success of the drug.
Scheme 1. Synthesis of diversified isatin analogues. Ethanol, reflux, a = pyridine-3-carboxaldehyde, b = 3-bromobenzothiophene-2-carboxaldehyde, c = 2-thiophenecarboxaldehyde, d = thiosemicarbazide, e = 4-(β-d-allopyranosyloxy)benzaldehyde, f = fluorene-2-carboxaldehyde, g = 1,4-benzodioxan-6-carboxaldehyde, and h = indole-3-carbxaldehyde.
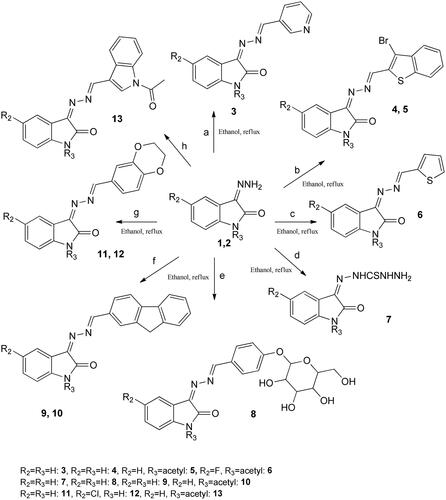
Moreover, fluorene and dihydrobenzo[1,4]-dioxane skeletons are well known for their antimicrobial potential. For synthesizing compounds, 8, 9, 11, and 12, 3-hydrazonoindolin-2-one 3 was treated with 4-(β-d-allopyranosyloxy)-benzaldehyde, fluorene-2-carboxaldehyde, 1,4-benzodioxane-6-carboxaldehyde, and fluorinated analogue of 1,4-benzodioxane-6-carboxaldehyde, respectively in the presence of acetic acid in ethanol (Scheme 1). No aldehyde peak was observed; a new peak corresponds to exocyclic CH proton in the downfield region in all 8, 9, 11, and 12 proton spectrums.
Furthermore, compounds 9 and 3-(((1H-indol-3-yl)methylene)hydrazono)indolin-2-one were treated with an excess of acetic anhydride with few drops of pyridine at room temperature, obtaining 10 and 13. The primary support for the proposed structures was provided by 1H NMR spectra, particularly concerning the protons of the methyl group, which appeared as a singlet at around 2.66 and 2.67 ppm in 10 and 13, respectively. In molecule 13, both NH groups were acetylated rendering the molecule highly soluble in most solvents. Mass and FTIR spectrums are also following the proposed structures. 2-Amino-diphenylethanol bearing a chiral centre represents an essential class of substituted isatin. 3-(2-Hydroxy-1,2-diphenylethylimino)indolin-2-one 14 was obtained when chiral aminoethanol was reacted with isatin under reflux in ethanol. In the proton NMR spectrum, two doublets representing two CH groups and the free NH appear as a broad singlet in the downfield region (11.14 ppm), further confirming the postulated structure.
Moreover, the purine heterocyclic system, the parent ring in various analogues of biological importance, is considered an essential natural ring systemCitation23. In this work, the construction of purine heterocyclic system was carried out by a reaction of isatin 1 with 8-(4-aminophenoxy)-9-ethyl-1,3-dimethyl-1H-purine-2,6(3H,9H)-dione rendered 15 in moderate yield (Scheme 2). The product is confirmed by one- and two-dimensional NMR and mass spectroscopy.
Scheme 2. Synthesis of spiro-isatin and imi. a = 2-amino-1,2-diphenylethanol, b = 8-(4-aminophenoxy)-9-ethyl-1,3-dimethyl-1H-purine-2,6(3H,9H)-dione, c = 2-chloroethylmethyl sulphide, d = bromoethanol, and e = 2-mercaptoethanol.
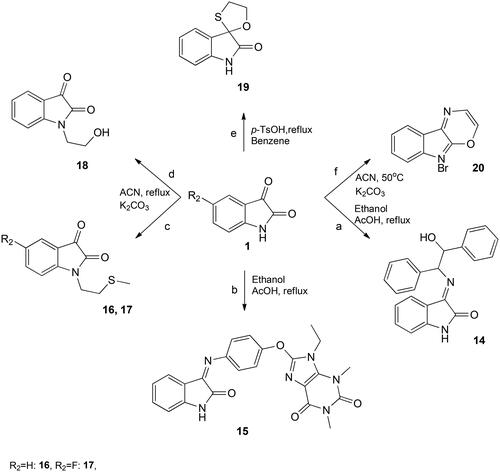
Alkylating the nitrogen atom within isatin leads to the generation of several biologically significant derivatives. These derivatives serve as versatile synthetic intermediates capable of producing a diverse range of promising pharmaceutical compounds. While previous attempts at synthesizing these derivatives have been documented, they have encountered significant challenges due to the demanding reaction conditions and the formation of various unwanted byproducts, rendering the isolation of the desired molecules unfeasibleCitation24. In this study, N-alkylation of isatin was executed by subjecting 2-chloroethylmethyl sulphide and bromoethanol to a reaction in the presence of potassium carbonate, conducted under reflux conditions in acetonitrile. The resulting mixture yielded products 16, 17, and 18 in substantial yields, and these were subsequently precipitated upon addition to ice water (refer to ). Confirmation of the proposed molecules was obtained through the disappearance of the NH proton and the emergence of thiomethyl protons, corresponding to SCH3 in the upfield region (approximately 2.3 ppm) in the proton NMR spectrum. Before undergoing spectroscopic characterization, all synthesized compounds were meticulously washed with water and cold ethanol.
Furthermore, spiro derivatives of isatin have been found to produce main pharmacological activity, especially in natural product chemistryCitation25. Various studies have been reported involving the C-3 position of isatin in synthesizing spiro analogues like spiroxindole. The major drawback of the written protocol for synthesizing oxathiino[2,3-b]indole 19 is the low yield and formation of side products. In the current paper, we developed a facile route to synthesize 3,4a,5,9b-tetrahydro-2H-[1,4]oxathiino[2,3-b]indole 19 in our search for the synthesis of a diverse range of biologically active heterocyclic compounds. Para toluenesulfonic acid (p-TsOH) is used as an active catalyst in benzene to synthesize spirthioindole in excellent yields. In the reaction of isatin with bromoethyl amine, acetonitrile was used with K2CO3. The product was obtained after prolonged heating at 50 °C while no side products were observed. The synthesized compounds 19 and 20 were well characterised by spectral data. In 1H NMR spectra, the appearance of CH2 at around 3–4 ppm in 19 and two doublets that correspond to CH attached to N and O in the downfield region in 20 are proof for the proposed structures (Scheme 2). The IR spectra of all the synthesized compounds also showed spectral bands in their respective regions.
Biology
Anticancer evaluation
All the synthesized compounds were subjected to an in vitro antiproliferative screening against 60 different cancer cell lines at the National Cancer Institute (NCI)Citation26. The mean percentages of growth inhibition (GI %) of the tested compounds are shown in . Human cell lines that were used in the current paper are derived from leukaemia, lung, colon, CNS, melanoma, ovarian, renal, prostate, and breast cancers. Some of the synthesized compounds showed excellent anti-proliferative activity when treated with a concentration of 10 mM as presented in . The inhibitory activity of compounds in the series indicates some sort of inhibition with the enzyme albeit weak. Moreover, it is imperative that the activity is primarily selective to those compounds featuring privileged groups attached to isatin moietyCitation27. For example, molecules 3 (34.14%), 4 (34.38%), 19 (37.09%), 6 (41.34%), and 16 (43.82%) showed excellent activity against renal cancer cell line UO-31 (). This is assumed to be due to the biological activity asserted by the attached group, which plays a major role in reducing cytotoxicity.
Table 1. Growth inhibition of selected compounds.
Table 2. Activity comparison against various cell lines.
The Schiff bases of isatin 3, 4, 6, and 15 enhance inhibition compared with that of fluorene and benzodioxane. This could be due to the biological activity associated with thiophene and benzothiazole moiety, while the benzothiazole bearing a bromo group also plays a role in increased biological activity. It was anticipated that the presence of a purine nucleus enhances the inhibition considerably, as in the case of 15. Moreover, the impact of the isatin group in the overall activity is also considered. Compound 6 is found to be the most potent molecule showing inhibition against leukaemia (35.92%), central nervous system (35.5%), and renal cancer (41.34%) cell lines. Compound 9 was most active on the SNB-75 CNS cell line with a growth inhibition of 36.56% and the UO-31 renal cancer cell line with a growth inhibition of 37.09%. This activity is due to the spirothio indole nucleus, which is proven to be active against various cell linesCitation28.
Antimicrobial activity
The synthesized compounds underwent testing against five bacterial and two fungal strains, revealing that some of these compounds demonstrated activity against fungi, with the most notable candidates being compounds 3 and 16 (refer to ). Compound 16, in particular, displayed exceptional cytotoxicity against Candida albicans and Cryptococcus neoformans at an initial concentration of 32 μg/ml, as detailed in . Furthermore, compound 3 exhibited moderate activity against Staphylococcus aureus, a gram-positive bacteriumCitation29. For the compounds with relatively weak inhibitory effects identified in this study, it would be imperative to consider further structural modifications, such as extending the structure or incorporating bioactive or related groups, to enhance their inhibitory potency.
Table 3. Antimicrobial data of selected molecule.
Structure–activity relationship
According to the biological activity data and the structural variations between the molecules, the structural modification of indolin to N-acetylindolin along with thiophene moiety led to improvement in cytotoxicity results that may be explained by increasing hydrogen bond formation in 6. Regarding groups present at the benzothiophene ring, 4 exhibited higher activity within the same type of molecules and had more contribution in the cytotoxicity than the other groups. The electron-withdrawing group on benzothiophene exhibited moderate to significant influence on antitumor activity. Meanwhile, in 3, pyridine displayed higher cytotoxicity among other groups like benzylidine and fluorene, suggesting that π-electron delocalisation at the pyridine ring has a higher effect as compared with others.
Regarding groups protecting the indolinedione ring, methylthio in 16 exhibited higher activities as compared to the hydroxyethyl group in 18, indicating that the thiol group has more contribution to the cytotoxicity than the alcohol group. Compound 16 also showed excellent antifungal activity against Candida albicans and Cryptococcus neoformans, which confirms the strong effect exerted by the methylthiol group on biological activity. The same effect has been observed in 19, which exhibited better cytotoxicity as compared to 20, due to oxothiino indole moiety. This study may provide useful information for further design of isatin hybrids having better antitumor activity.
Molecular modelling
In general, molecular docking calculations are carried out to support experimental activities, to design more stable and more active molecules, and to detect active sites of molecules. Molecular modelling is an important method that examines the interactions of molecules with proteins through molecular docking calculations. This method determines the activity of molecules against proteins and the interaction between them, and as this interaction increases, the activity of the molecules increases. In this study, the microorganism used is Sa: Staphylococcus aureus, EC: Escherichia coli, Kp: Klebsiella pneumonia, Pa: Pseudomonas aeruginosa, Ab: Acinetobacter baumannii, Ca: Candida albicans, and Cn: Cryptococcus neoformans var. grubii against synthesised molecules. The theoretical calculation results of the four bacteria that showed the most agreement as a result of the theoretical calculation are given in the study. It is observed that hydrogen bonds, polar and hydrophobic interactions, π–π, and halogen bond interactions occur between molecules and proteins. The interactions that occur are given in and . As a result of the calculations, many parameters have been calculated, and each calculated parameter gives information about the different properties of the molecules. When these parameters are examined, the first parameter that determines the activities of the molecules is the docking score parameter. Moreover, glide bond, glide van der Waals energy, and Glide ecoul parameters give numerical values of interactions between molecules and proteins.
The parameters that give information about the interaction pose between molecules and proteins are glide model, glide energy, glide internal, and glide posenum. In the current paper, the interaction between molecule 12 and the Staphylococcus aureus protein is given in , and it is seen that hydrogen bonding occurs between the oxygen atom attached to the carbonyl carbon in molecule 12 and the LYS 84 protein in this interaction. The interaction between 12 and Escherichia coli protein is given in , and it is evident that hydrogen bonding occurs between the oxygen atom attached to the carbonyl carbon in molecule 12 and the ARG 468 protein in this interaction. It is also observed that one of the oxygen atoms in the 2,3-dihydrobenzo[b][1,4]dioxane ring in molecule 12 forms a hydrogen bond with the THR 37 protein, and the other oxygen atom forms a hydrogen bond with the SER 389 and ASN 391 protein. Furthermore, the interaction between molecule 8 and the Pseudomonas aeruginosa protein is shown in and it is observed that the hydrogen bonding occurs between the hydrogen atom attached to the amine in the indolin-2-one ring in molecule 8 and the ARG 71 protein. The hydroxy groups in the 2-(hydroxymethyl)tetrahydro-2H-pyran-3,4,5-triol ring in molecule 8 appear to form hydrogen bonds with GLN 94, ILE 92, HIE 78, and GLN 81 proteins. It is envisaged that hydrogen bonding occurs between the oxygen atom in the centre of molecule 8 and the GLN 98 protein. Finally, in , it is seen that polar and hydrophobic interactions occur between molecule 6 and the protein of Acinetobacter baumannii.
ADME/T analysis (absorption, distribution, metabolism, excretion, and toxicity) was performed to examine the effects and responses of these studied molecules in human metabolism. With this analysis, the absorption of molecules by human metabolism, distribution in human metabolism, excretion from metabolism, and finally toxicity values in metabolism are calculatedCitation30,Citation31. The parameters calculated here are examined for chemical as well as biological properties of molecules. As a result of these ADME/T calculations, the calculated parameters of the molecules are given in . Although the calculated chemical and biological parameters of the molecules found in this study are within the desired values for use in human metabolism as a drug, the numerical values of the rule of five (ROF)Citation32,Citation33 and rule of three (ROT)Citation34 parameters are two important parameters considered for their use as drugs. Although the ROF parameter is generally known as the number of violations of Lipinski’s rule of five, it is known to contain four parameters. On the other hand, the ROT parameter is known as several numbers of violations of Jorgensen’s rule of three.
Table 4. ADME properties of molecule.
Docking with 1A06 protein
In the current paper, the interaction between molecule 6 and the 1A06 protein is given in , and it is seen that hydrogen bonding occurs between the imine moiety in molecule 6 and the PHE 254 protein in this interaction. Moreover, the interaction between molecule 6 and 1A06 protein is given in and it is evident that hydrogen bonding occurs between the exocyclic nitrogen double bond in molecule 6 and the PHE 270 protein in this interaction. It is also observed that the phenyl ring in molecule 6 forms a hydrogen bond with the PHE 228 protein and MET 231 protein as evident in .
Materials and methods
All solvents and reagents were purchased from Aldrich Chemical Co. (St. Louis, MO) and were used as obtained. The reactions were carried out under air atmosphere unless stated otherwise. IR spectra were recorded with a Bruker Alpha spectrometer (Billerica, MA).1H- and 13C NMR spectra were recorded on a Bruker instrument at 500 and 700 MHz for 1H and 176 MHz for 13C at 295 K in DMSO-d6 and CDCl3. The mass spectrometric experiments were carried out on a Jeol JMS-700 mass spectrometer (Akishima, Japan). Thin-layer chromatography (TLC) was performed on a fluorescent aluminium backed silica gel HF254 plates (Merck, Kenilworth, NJ) and was viewed under UV 254 and 265 lights and charring with EtOH/H2SO4. Merck silica gel 60 (230–400 mesh) was used for column chromatography separations.
General procedure for the synthesis of compounds 4, 6, 8, 9, and 11
A mixture containing the respective isatin hydrazide (1.1 mmol) and appropriate aldehyde (1.0 mmol) with catalytic amount of acetic acid was heated under reflux and stirring for 3 h in dry ethanol (20 ml). After completion of the reaction, as indicated by TLC, the reaction mixture was poured onto crushed ice and the solid separated was filtered, washed with ice-cold water several times (50 ml) and subsequently dried to afford pure product.
General procedure for the synthesis of compound 7
A mixture containing the isatin (1 mmol) and thiosemicarbazide (1.1 mmol) with catalytic amount of acetic acid was heated under reflux and stirring for 2 h in dry ethanol (20 ml). After completion of the reaction, the mixture was poured onto crushed ice and the solid separated was filtered, washed with ice-cold water several times (50 ml) and subsequently dried to afford pure product. The obtained product was dissolved in excess of acetic anhydride (25 ml) with few drops of pyridine and stirred overnight at room temperature. The solid obtained after adding ice was filtered and washed with water and dried to afford pure product.
General procedure for the synthesis of compounds 5, 10, 12, and 13
To a vigorously stirred solution of respective imines (1.0 mmol) in acetic anhydride (50 ml) was added few drops of pyridine. The resulting mixture was stirred overnight for completion of the reaction and the progress of the reaction was monitored by TLC. The solvent was evaporated to afford the crude product, which was poured onto crushed ice and the separated solid was filtered, washed several times with cold ethanol (25 ml) to obtain pure product.
General procedure for the synthesis of compounds 16, 17, 18, and 20
A mixture containing the respective isatin hydrazide (1.1 mmol), 2-chloroethylmethyl sulphide, and/or bromoethanol (1.5 mmol) respectively was refluxed in acetonitrile (20 ml) in the presence of K2CO2 (2.0 mmol) for 3 h. The mixture was then stirred at room temperature for completion of the reaction, as indicated by TLC. The product obtained was poured onto crushed ice and the solid separated was filtered, washed with ice-cold water several times (50 ml) and subsequently dried to afford pure product.
General procedure for the synthesis of compound 19
To a vigorously stirred solution of isatin (1.1 mmol) in benzene (50 ml) was added mercaptoethanol (2 mmol) and p-TsOH (0.01 mmol). The resulting mixture was stirred at room temperature for 1 h and then refluxed for 5 h. The progress of the reaction was monitored by TLC. After completion of the reaction, the product was evaporated and purified by column chromatography (1:2 hexane–ethylacetate).
3-(((3-Bromobenzo[b]thiophen-2-yl)methylene)hydrazono)indolin-2-one (4)
Yield 68%, yellowish brown solid, m.p.: 244–247 °C. IR: 1715 and 3100 cm−1: 1H NMR (700.170 MHz, DMSO-d6): δ = 6.93 (d, 1H, J = 7 Hz, Ph), 7.08 (s, 1H, Ph), 7.45 (s, 1H, Ph), 7.62, 7.65 (m, 2H, Ph), 7.93 (d, 2H, J = 7 Hz, Ph), 8.19 (s, 1H, CH), 8.93 (s, 1H, Ph), 10.94 (s, 1H, NH). 13C NMR (DMSO-d6) δ = 111.5, 116.4, 124.1, 124.2, 124.5, 126.8, 127.0, 129.1, 129.5, 130.1, 133.9, 138.2, 145.9, 152.0, 154.9, 164.8, and 185.7.
MS (ESI, 70 eV): m/z 383 (MH)+. Anal. Calcd. for C17H10BrN3OS (382.97).
1-Acetyl-3-(((3-bromobenzo[b]thiophen-2-yl)methylene)hydrazono)indolin-2-one (5)
Yield 88%, light yellow solid, m.p.: 171–174 °C. IR: 1710 and 3070 cm−1: 1H NMR (700.170 MHz, DMSO-d6): 2.67 (s, 3H, CH3), 7.39 (s, 1H, Ph), 7.64–7.68 (m, 3H, Ph), 7.96 (d, 1H, J = 7 Hz, Ph), 8.21 (s, 1H, CH), 8.28 (dd, 2H, J = 4 Hz, Ph), and 8.98 (s, 1H, Ph). 13C NMR (DMSO-d6) δ = 26.9, 116.9, 117.9 124.1, 124.4, 125.9, 126.9, 129, 129.3, 133.8 (2×), 134.5, 138.2, 139.4, 143.4, 149.9, 155.6, 163.7, and 170.6.
MS (ESI, 70 eV): m/z 425 (MH)+. Anal. Calcd. for C19H12BrN3O2S (424.98).
1-Acetyl-5-fluoro-3-((thiophen-2-ylmethylene)hydrazono)indolin-2-one (6)
Yield 84%, yellow solid, m.p.: 175–178 °C. IR: 1710, 1725, and 3100 cm−1: 1H NMR (700.170 MHz, DMSO-d6): 2.64 (s, 3H, CH3), 7.35 (t, 1H, J = 14 Hz, thiaz-H), 7.48 (t, 1H, J = 14 Hz, Ph), 7.91–7.97 (m, 1H, thiaz-H), 8.08–8.11 (m, 2H, Ph, thiaz-H), 8.39–8.43 (m, 1H, CH), and 9.05 (s, 1H, Ph). 13C NMR (DMSO-d6) δ = 26.2, 111.9, 115.4, 120.1, 129.2 (2×), 134 (2×), 136.1 (2×), 141.3, 151.1, 156.9, 164.6, and 169.7.
MS (ESI, 70 eV): m/z 316 (MH)+. Anal. Calcd. for C15H10FN3O2S (315.05).
2-(2-Oxoindolin-3-ylidene)hydrazinethiocarbohyzrazide (7)
Yield 73%, brown solid, m.p.: 278–280 °C. IR: 1710, 3100, and 3100 cm−1: 1H NMR (700.170 MHz, DMSO-d6): 6.91–6.99 (m, 1H, Ph), 7.17–7.21 (m, 2H, Ph), 7.59–7.63 (m, 1H, Ph), 7.61–7.64 (m, 1H, Ph), 11.33 (br s, 2H, NH2), 13.01 (br s, 1H, NH), and 14.95 (br s, 1H, NH). 13C NMR (DMSO-d6) δ = 112.6, 119.9, 121.1, 138.8, 151.1, 159.8, 163.1, 175.7, and 184.8.
MS (ESI, 70 eV): m/z 236 (MH)+. Anal. Calcd. for C9H9N5OS (235.05).
3-((4-(3,4,5-Trihydroxy-6-(hydroxymethyl)tetrahydro-2H-pyran-2-yloxy)benzylidene) hydrazono)indolin-2-one (8)
Yield 71%, light yellow solid, m.p.: 146–149 °C. IR: 1715, 3100, and 3500 cm−1: 1H NMR (700.170 MHz, DMSO-d6): 3.35–3.41 (m, 3H, 3 × H), 3.77–3.81 (m, 3H, OH, 2 × CH), 3.99 (s, 1H, OH), 4.55–4.61 (m, 1H, CH), 4.74–4.81 (m, 1H, CH), 5.11–5.15 (m, 1H, CH), 5.27–5.30 (m, 2H, CH2), 6.99 (s, 1H, Ph), 7.16 (s, 1H, Ph), 7.31–7.35 (m, 2H, Ph), 7.57–7.62 (m, 1H, Ph), 8.11–8.15 (m, 3H, Ph), 8.41 (s, 1H, CH), and 10.91 (br s, 1H, NH). 13C NMR (DMSO-d6) δ = 61.3, 67.4, 70.6, 71.9, 75.2, 98.5, 111.2, 117, 117.1, 122.8, 127.4, 129.4, 131.3 (2×), 134 (2×), 145.3, 151.2, 161.2, 162.3, and 165.2.
MS (ESI, 70 eV): m/z 428 (MH)+. Anal. Calcd. for C21H21N3O7 (427.14).
3-(((9H-Fluoren-2-yl)methylene)hydrazono)indolin-2-one (9)
Yield 64%, yellow solid, m.p.: 181–183 °C. IR: 1715, 1725, and 3100 cm−1: 1H NMR (700.170 MHz, DMSO-d6): δ = 4.08 (s, 2H, CH2), 6.93 (d, 1H, Ph, J = 7 Hz), 7.09 (s, 1H, Ph), 7.43 (dd, J = 7 Hz, 3H, Ph), 7.68 (d, 1H, J = 13.8 Hz, Ph), 8.03 (d, 3H, J = 14 Hz, Ph), 8.12 (s, 1H, CH), 8.22 (s, 1H, Ph), 8.76 (s, 1H, Ph), and 10.90 (s, 1H, br s, NH). 13C NMR (DMSO-d6) δ = 36.9, 111.3, 117, 121.1, 121.4, 122.9, 125.8, 125.9, 127.5, 128.4, 128.8, 129.3, 132.4, 134.1, 140.6, 144.3, 144.6, 145.4, 145.6, 151, 162.3, and 165.1.
MS (ESI, 70 eV): m/z 338 (MH)+. Anal. Calcd. for C22H15N3O (337.12).
3-(((9H-Fluoren-2-yl)methylene)hydrazono)-1-acetylindolin-2-one (10)
Yield 82%, light yellow solid, m.p.: 162–165 °C. IR: 1712, 1725, and 3150 cm−1: 1H NMR (700.170 MHz, DMSO-d6): 2.66 (s, 3H, CH3), 4.08 (s, 2H, CH2), 7.40 (dd, 3H, J = 14 Hz, Ph), 7.60 (dd, 2H, J = 14 Hz, Ph), 8.04–8.09 (m, 3H, CH, Ph), 8.08 (d, 2H, J = 7 Hz, Ph), 8.25–8.31 (m, 1H, Ph), and 8.84 (s, 1H, Ph). 13C NMR (DMSO-d6) δ = 27, 36.9, 116.8, 118.3, 121.2, 121.5, 125.8, 125.9, 126.2, 127.5, 128.6, 128.9, 129.2, 132.2, 133.9, 140.5, 142.9, 144.4, 144.7, 145.9, 149.1, 163.7, 164.1, and 170.7.
MS (ESI, 70 eV): m/z 380 (MH)+. Anal. Calcd. for C24H17N3O2 (379.13).
3-(((2,3-Dihydrobenzo[b][1,4]dioxin-6-yl)methylene)hydrazono)indolin-2-one (11)
Yield 64%, yellow solid, m.p.: 177–180 °C. IR: 1715, 3100 cm−1: 1H NMR (700.170 MHz, DMSO-d6): 4.37 (d, 4H, J = 14 Hz, 2 × CH2), 6.91 (t, 1H, J = 14 Hz, Ph), 7.09 (t, 2H, J = 7 Hz, Ph), 7.40–7.53 (m, 3H, Ph), 7.99 (d, 1H, J = 14 Hz, Ph), 8.58 (d, 1H, J = 14 Hz, Ph), and 10.85 (br s, 1H, NH). 13C NMR (DMSO-d6) δ = 64.4, 65, 111.2, 117, 117.7, 118.4, 122.8, 123.4, 127.3, 129.2, 134, 144.2, 145.3, 147.7, 151.1, 161.8, and 165.1.
MS (ESI, 70 eV): m/z 308 (MH)+. Anal. Calcd. for C17H13N3O3 (307.10).
5-Chloro-3-(((2,3-dihydrobenzo[b][1,4]dioxin-6-yl)methylene)hydrazono)indolin-2-one (12)
Yield 84%, yellow solid, m.p.: 183–186 °C. IR: 1710 and 3150 cm−1: 1H NMR (700.170 MHz, DMSO-d6): 4.36 (q, 4H, 2 × CH2), 6.93 (d, 1H, J = 7 Hz, Ph), 7.10 (d, 1H, J = 14 Hz, Ph), 7.48 (dd, 3H, J = 14 Hz, J = 6.8 Hz, Ph), 7.98 (s, 1H, Ph), 8.64 (s, 1H, CH), and 11.01 (br s, 1H, NH). 13C NMR (DMSO-d6) δ = 64.4, 65.1, 112.8, 117.7, 118.2, 118.5, 122.6, 126.2, 127.1, 128.5, 133.4, 144.1, 144.3, 148.1, 150.6, 163.6, and 164.8.
MS (ESI, 70 eV): m/z 343 (MH)+. Anal. Calcd. for C17H12ClN3O3 (341.06).
1-Acetyl-3-(((1-acetyl-1H-indol-3-yl)methylene)hydrazono)indolin-2-one (13)
Yield 78%, yellow solid, m.p.: 163–166 °C. IR: 1715, 1730, and 3050 cm−1: 1H NMR (700.170 MHz, DMSO-d6): 2.67 (s, 3H, CH3), 3.35 (s, 3H, CH3), 7.37 (d, 1H, J = 7 Hz, Ph), 7.53–7.59 (m, 2H, Ph), 7.61 (t, 1H, J = 14 Hz, J = 14 Hz, Ph), 8.30 (d, 1H, J = 7 Hz, Ph), 8.43–8.50 (m, 3H, CH, Ph), 8.74 (s, 1H, Ph), and 8.90 (s, 1H, Ph). 13C NMR (DMSO-d6) δ = 24.3, 27.1, 116.8, 116.9, 118.3, 122.1, 125.4, 125.7, 126.7 (2×), 127.8, 133.8 (2×), 136.6, 136.8, 142.7, 148.3, 158.5, 164.1, 170.3, and 170.7.
MS (ESI, 70 eV): m/z 373 (MH)+. Anal. Calcd. for C21H16N4O3 (372.12).
3-(2-Hydroxy-1,2-diphenylethylimino)indolin-2-one (14)
Yield 78%, red solid, m.p.: 159–162 °C. IR: 1710, 3300, and 3400 cm−1: 1H NMR (700.170 MHz, DMSO-d6): 6.03 (d, 1H, J = 4 Hz, CH), 6.09 (d, 1H, J = 4 Hz, CH), 6.94 (d, 1H, J = 7 Hz, Ph), 7.26–7.31 (m, 1H, Ph), 7.32–7.37 (m, 2H, Ph), 7.38–7.41 (m, 2H, Ph), 7.42–7.48 (m, 3H, Ph), 7.58 (d, 2H, J = 14 Hz, Ph), 7.63–7.70 (m, 2H, Ph), 8.01–8.06 (m, 2H, Ph), and 11.14 (br s, 1H, NH). 13C NMR (DMSO-d6) δ = 71.1, 76.1, 114.3, 124.6, 127.2 (2×), 127.7, 128.1 (2×), 128.9, 129.1 (3×), 129.3 (2×), 133.7, 135.1, 137.7, 149.6, 159.6, 183.8, and 199.6.
MS (ESI, 70 eV): m/z 343 (MH)+. Anal. Calcd. for C22H18N2O2 (342.14).
9-Ethyl-1,3-dimethyl-8-(4-(2-oxoindolin-3-ylideneamino)phenoxy)-1H-purine-2,6(3H,9H)-dione (15)
Yield 71%, brown solid, m.p.: 167–169 °C. IR: 1715, 1735, and 3100 cm−1: 1H NMR (700.170 MHz, DMSO-d6): 1.41 (t, 3H, J = 14 Hz, J = 14 Hz, CH3), 3.25 (s, 6H, N-CH3), 4.27 (d, 2H, J = 7 Hz, CH2), 6.92 (d, 2H, J = 14 Hz, Ph), 7.03 (d, 2H, J = 14 Hz, J = 14 Hz, Ph), 7.43 (d, 2H, J = 14 Hz, J = 14 Hz, Ph), 7.51–7.56 (m, 1H, Ph), 7.57–7.61 (m, 1H, Ph), and 11.01 (br s, 1H, NH). 13C NMR (DMSO-d6) δ = 15.8, 18.1, 39.5 (2×), 111.5 (2×), 111.6 (2×), 116.2, 116.6, 123 (2×), 128.6 (2×), 134.8 (2×), 139.5, 145.2 (2×), 145.6 (2×), 163.8, and 184.8.
MS (ESI, 70 eV): m/z 445 (MH)+. Anal. Calcd. for C23H20N6O4 (444.15).
1-(2-(Methylthio)ethyl)indoline-2,3-dione (16)
Yield 66%, yellow solid, m.p.: 248–250 °C. IR: 1715 and 3100 cm−1: 1H NMR (500.133 MHz, CDCl3): δ = 2.19 (s, 3H, SCH3), 2.81 (t, 2H, J = 14 Hz, CH2), 3.95 (t, 2H, J = 14 Hz, CH2), 6.95 (d, 1H, J = 14 Hz, J = 7 Hz, Ph), 7.13 (d, 1H, J = 7 Hz, Ph), and 7.60 (t, 2H, J = 15 Hz, Ph). 13C NMR (DMSO-d6) δ = 18.1, 19.7, 62.6, 100.6, 111.3, 120.7, 121.5, 137.1, 142.4, 162.2, and 179.3.
MS (ESI, 70 eV): m/z 222 (MH)+. Anal. Calcd. for C11H11NO2S (221.05).
5-Fluoro-1-(2-(methylthio)ethyl)indoline-2,3-dione (17)
Yield 55%, red solid, m.p.: 182–184 °C. IR: 1715 and 3040 cm−1: 1H NMR (700.170 MHz, DMSO-d6): 2.36 (s, 3H, SCH3), 3.25–3.38 (m, 2H, CH2), 4.25 (d, 2H, J = 7 Hz, CH2), 6.95 (d, 1H, J = 7 Hz, Ph), 7.25 (d, 1H, J = 7 Hz, Ph), and 7.53–7.59 (m, 1H, Ph). 13C NMR (DMSO-d6) δ = 15.8, 28.3, 30.1, 111.3, 120.3, 122.9, 132.3, 133.9, 148.5, 151.3, and 165.9.
MS (ESI, 70 eV): m/z 240 (MH)+. Anal. Calcd. for C11H10FNO2S (239.04).
1-(2-Hydroxyethyl)indoline-2,3-dione (18)
Yield 62%, yellow solid, m.p.: 151–154 °C. IR: 1720 and 3300 cm−1: 1H NMR (700.170 MHz, DMSO-d6): 4.36 (t, 2H, J = 3 Hz, CH2), 4.59 (t, 2H, J = 3.1 Hz, CH2), 6.86 (d, 1H, J = 7 Hz, Ph), 7.09 (t, 1H, J = 7 Hz, Ph), 7.32 (d, 1H, J = 7 Hz, Ph), 7.37 (t, 1H, J = 14 Hz, Ph), and 8.14 (br s, 1H, OH). 13C NMR (DMSO-d6) δ = 65.8 (2×), 110.7, 123.3 (2×), 124.3, 125.2, 131.6, 141.7, and 174.4.
MS (ESI, 70 eV): m/z 192 (MH)+. Anal. Calcd. for C10H9NO3 (191.06).
3,4a,5,9b-Tetrahydro-2H-[1,4]oxathiino[2,3-b]indole (19)
Yield 68%, orange solid, m.p.: 128–130 °C. IR: 1720 and 2900 cm−1: 1H NMR (700.170 MHz, DMSO-d6): 3.5 (dd, 2H, 2 × CH), 4.47 (q, 1H, CH), 4.58 (q, 1H, CH), 6.85 (d, 1H, J = 7 Hz, Ph), 7.03 (t, 1H, J = 14 Hz, Ph), 7.29 (t, 1H, J = 14 Hz, Ph), 7.38 (d, 1H, J = 7 Hz, Ph), and 10.51 (br s, 1H, NH). 13C NMR (DMSO-d6) δ = 34.1, 72.7, 88.5, 110.7, 122.8, 125.8, 127.1, 131.3, 142.1, and 176.9.
MS (ESI, 70 eV): m/z 208 (MH)+. Anal. Calcd. for C10H9NO2S (207.04).
5-Bromo-4a,5-dihydro-[1,4]oxazino[2,3-b]indole (20)
Yield 66%, light brown solid, m.p.: 115–118 °C. IR: 3100 cm−1: 1H NMR (700.170 MHz, DMSO-d6): 7.50 (t, 1H, J = 3.5 Hz, CH), 7.76–7.81 (m, 1H, Ph), 7.88–7.91 (m, 2H, CH, Ph), and 7.96 (d, 2H, J = 14 Hz, Ph). 13C NMR (DMSO-d6) δ = 117.5, 125.2, 127.4, 130.3 (2×), 135.1, 136.2, 145.5, 158.2, and 182.9.
MS (ESI, 70 eV): m/z 249 (MH)+. Anal. Calcd. for C10H6BrN2O (248.97).
Anticancer activity study
The anticancer activity of the synthesized compound was tested against 60 different cell lines by the NCI. Briefly, the compound is prepared in DMSO:glycerol, 9:1 at 4 mmol and kept frozen prior to use. Generally, for inoculation, a 96-well microtiter is used for the study of different cell lines. Based on the doubling time for each cell line, the well density varies. Then, the plates are incubated at 37 °C, 5% CO2, 95% air, and 100% relative humidity for one day. Later, at 5-log M concentration range the tested compound and the control are added to the plates. After two more days, the plates are fixed and stained to identify growth inhibition relative to cells without drug treatment and determination of cell kill as well as net growth inhibition based on time zero control.
Antibacterial studies
Antimicrobial activity of selected compounds was done by Co-Add Australia against following strains: Staphylococcus aureus, Escherichia coli, Klebsiella pneumoniae, Pseudomonas aeruginosa, Acinetobacter baumannii, Candida albicans, and Cryptococcus neoformans var. grubii. Samples were stored frozen at −20 °C, prepared in DMSO and water to a final testing concentration of 32 μg/ml or 20 μM (unless otherwise indicated in the data sheet), in 384-well, non-binding surface plate (NBS) for each bacterial/fungal strain, and in duplicate (n = 2), and keeping the final DMSO concentration to a maximum of 1% DMSO. All the sample preparation was done using liquid handling robots.
Molecular docking calculation
An important method used to determine the molecules with the highest activity against biological materials is docking. Molecular docking calculations are made by Schrödinger’s Maestro Molecular modelling platform (version 12.8)Citation35–37. In the calculations made with this method, it is possible to comment on the active sites of the molecules. Calculations are made up of several steps. It first uses the protein preparation moduleCitation38 to prepare the protein, then the LigPrep moduleCitation39 to prepare the molecules. Prepared proteins and molecules are interacted with each other with the Glide ligand docking toolCitation40. Finally, the Qik-prop module of the Schrödinger softwareCitation41 was used while performing ADME/T analysis in order to examine the effects and effects of the studied molecules on human metabolism.
Conclusions
These results encourage the potential implementation of isatin derived analogs as application in cancer treatment by inhibition of cancer cell lines. Some of the molecules (3, 4, and 19) showed good to excellent antiproliferative activity against renal cancer cell lines and their docking scores are higher as compared to others. Moreover, compound 15 exhibited excellent activity against CNS cancer cell line selectively. The synthesized molecules could further be derivatized and modified for maximum potency and less toxicity. Moreover, the potential efficacy and safety of high doses of synthesized compounds should also be critically assessed by further larger in vivo/human studies.
Author contributions
M.T. contributed to overall structural elucidation, writing and overall management of the project; S.S. obtained and interpreted mass spectra; B.T. contributed in molecular modelling of molecules; M.R.A. involved in obtaining NMR spectra; O.A. isolated the compounds; A.B. synthesized molecules, contributed to interpreting spectra, writing the manuscript.
Supplemental Material
Download PDF (404.1 KB)Acknowledgements
The authors extend their appreciation to the Deputyship for Research & Innovation, Ministry of Education in Saudi Arabia for funding this research (IFKSURC-1-0804).
Disclosure statement
The authors declare no competing interests.
Additional information
Funding
References
- Sartori A, Portioli E, Battistini L, Calorini L, Pupi A, Vacondio F, Arosio D, Bianchini F, Zanardi F. Synthesis of novel c(Amp-RGD)-sunitinib dual conjugates as molecular tools targeting the αβ integrin/VEGFR2 couple and impairing tumor-associated angiogenesis. J Med Chem. 2017;60(1):248–262.
- Chiyanzu I, Hansell E, Gut J, Rosenthal PJ, McKerrow JH, Chibale K. Synthesis and evaluation of isatins and thiosemicarbazone derivatives against cruzain, falcipain-2 and rhodesain. Bioorg Med Chem Lett. 2003;13(20):3527–3530.
- Vine KL, Matesic L, Locke JM, Ranson M, Skropeta D. Cytotoxic and anticancer activities of isatin and its derivatives: a comprehensive review from 2000–2008. Anticancer Agents Med Chem. 2009;9(4):397–414.
- Raj AA, Raghunathan R, SrideviKumari MR, Raman N. Synthesis, antimicrobial and antifungal activity of a new class of spiro pyrrolidines. Bioorg Med Chem. 2003;11(3):407–419.
- Mathur G, Nain S. Recent advancement in synthesis of isatin as anticonvulsant agents: a review. Med Chem. 2014;4(4):417–427.
- Rakesh KP, Ramesh S, Kumar HM, Chandan S, Gowda DC. Quinazolinones linked amino acids derivatives as a new class of promising antimicrobial, antioxidant and anti-inflammatory agents. Eur J Chem. 2015;6(3):254–260.
- Zhang X, Marichannegowda MH, Rakesh KP, Qin H-L. Master mechanisms of Staphylococcus aureus: consider its excellent protective mechanisms hindering vaccine development! Microbiol Res. 2018;212–213:59–66.
- Verma R, Verma SK, Rakesh KP, Girish YR, Ashrafizadeh M, Sharath Kumar KS, Rangappa KS. Pyrazole-based analogs as potential antibacterial agents against methicillin-resistance Staphylococcus aureus (MRSA) and its SAR elucidation. Eur J Med Chem. 2021;212:113134.
- Moku B, Ravindar L, Rakesh KP, Qin H-L. The significance of N-methylpicolinamides in the development of anticancer therapeutics: synthesis and structure–activity relationship (SAR) studies. Bioorg Chem. 2019;86:513–537.
- Ravindar L, Bukhari SNA, Rakesh KP, Manukumar HM, Vivek HK, Mallesha N, Xie Z-Z, Qin H-L. Aryl fluorosulfate analogues as potent antimicrobial agents: SAR, cytotoxicity and docking studies. Bioorg Chem. 2018;81:107–118.
- Rakesh KP, Darshini N, Manukumar HM, Vivek HK, Eissa MYH, Prasanna DS, Mallesha N. Xanthone conjugated amino acids as potential anticancer and DNA binding agents: molecular docking, cytotoxicity and SAR studies. Anticancer Agents Med Chem. 2018;18(15):2169–2177.
- Ullas BJ, Rakesh KP, Shivakumar J, Gowda CD, Chandrashekar PG. Multi-targeted quinazolinone-Schiff’s bases as potent bio-therapeutics. Results Chem. 2020;2:100067.
- Wang S, Zhao Y, Zhang G, Lv Y, Zhang N, Gong P. Design, synthesis and biological evaluation of novel 4-thiazolidinones containing indolin-2-one moiety as potential antitumor agent. Eur J Med Chem. 2011;46(8):3509–3518.
- Singh A, Kaur H, Arora S, Singh Bedi PM. Design, synthesis, and biological evaluation of novel morpholinated isatin–quinoline hybrids as potent anti-breast cancer agents. Arch Pharm. 2021;355(2):e2100368.
- Heer S, Kaur K, Gulati HK, Kumar N, Sharma A, Singh J, Bhagat K, Kaur G, Kaur K, Singh H, et al. Design, synthesis, and biological evaluation of isatin-indole-3-carboxaldehyde hybrids as a new class of xanthine oxidase inhibitors. Arch Pharm. 2022;355(6):e2200033.
- Singh A, Kaur K, Kaur H, Mohana P, Arora S, Bedi N, Chadha R, Bedi PMS. Design, synthesis and biological evaluation of isatin–benzotriazole hybrids as new class of anti-Candida agents. J Mol Struct. 1274;1274:134456.
- Zhai X, He Y, Yang Z, Gong P. Syntheses and antiproliferative activities of novel diarylthiosemicarbazide derivatives. Chem Res Chin Univ. 2013;29(1):62–66.
- Majumder A, Gupta R, Mandal M, Babu M, Chakraborty D. Air-stable palladium(0) phosphine sulfide catalysts for Ullmann-type C–N and C–O coupling reactions. J Organomet Chem. 2015;781:23–34.
- Jain R, Bansal M. A facile synthesis and central nervous system activities of fluorine-containing spiro-[3H-indole-3,4′(4H)-pyran]-2(1H)ones. Pharmazie. 1995;50:224–225.
- Friedel M, Marko ND, Tang W. Inhibition of cyclin-dependent kinase 1 (CDK1) by indirubin derivatives in human tumour cells. Nat Cell Biol. 1999;1:60.
- Eldehna WM, Altoukhy A, Mahrous H, Abdel-Aziz HA. Design, synthesis and QSAR study of certain isatin–pyridine hybrids as potential anti-proliferative agents. Eur J Med Chem. 2015;90:684–694.
- Vintonyak VV, Warburg K, Kruse H, Grimme S, Hübel K, Rauh D, Waldmann H. Identification of thiazolidinones spiro-fused to indolin-2-ones as potent and selective inhibitors of the Mycobacterium tuberculosis protein tyrosine phosphatase B. Angew Chem Int Ed Engl. 2010;49(34):5902–5905.
- Bari A, Grenier D, Azelmat J, Syed SA, Al-Obaid AM, Hosten EC. Synthesis and anti-inflammatory activity of diversified heterocyclic systems. Chem Biol Drug Des. 2019;94(4):1750–1759.
- Bari A, Ghani U, Syed SA, Riazullah . Thiosemicarbazide binds with the dicopper center in the competitive inhibition of mushroom tyrosinase enzyme: synthesis and molecular modeling of theophylline analogues. Bioorg Med Chem Lett. 2021;36:127826.
- Ragavendran JV, Sriram D, Patel SK, Reddy IV, Bharathwajan N, Stables J, Yogeeswari P. Design and synthesis of anticonvulsants from a combined phthalimide–GABA–anilide and hydrazone pharmacophore. Eur J Med Chem. 2007;42(2):146–151.
- Mologni L, Rostagno R, Brussolo S, Knowles PP, Kjaer S, Murray-Rust J, Rosso E, Zambon A, Scapozza L, McDonald NQ, et al. Synthesis, structure–activity relationship and crystallographic studies of 3-substituted indolin-2-one RET inhibitors. Bioorg Med Chem. 2010;18(4):1482–1496.
- Liang Z, Zhang D, Ai J, Chen L, Wang H, Kong X, Zheng M, Liu H, Luo C, Geng M, et al. Identification and synthesis of N′-(2-oxoindolin-3-ylidene)hydrazide derivatives against c-Met kinase. Bioorg Med Chem Lett. 2011;21(12):3749–3754.
- Refer to supplementary data for complete details.
- Refer to supplementary data for antimicrobial procedures and sample preparations.
- Poustforoosh A, Hashemipour H, Tüzün B, Azadpour M, Faramarz S, Pardakhty A, Mehrabani M, Nematollahi MH. The impact of D614G mutation of SARS-COV-2 on the efficacy of anti-viral drugs: a comparative molecular docking and molecular dynamics study. Curr Microbiol. 2022;79(8):241.
- Kekeçmuhammed H, Tapera M, Tüzün B, Akkoç S, Zorlu Y, Sarıpınar E. Synthesis, molecular docking and antiproliferative activity studies of a thiazole-based compound linked to hydrazone moiety. ChemistrySelect. 2022;7(26):e202201502.
- Lipinski CA. Lead-and drug-like compounds: the rule-of-five revolution. Drug Discov Today Technol. 2004;1(4):337–341.
- Lipinski CA, Lombardo F, Dominy BW, Feeney PJ. Experimental and computational approaches to estimate solubility and permeability in drug discovery and development settings. Adv Drug Delivery Rev. 1997;23(1–3):3–25.
- Jorgensen WJ, Duffy EM. Prediction of drug solubility from structure. Adv Drug Deliv Rev. 2002;54(3):355–366.
- Schrödinger Release 2021-3: Maestro. New York (NY): Schrödinger, LLC; 2021.
- Singh A, Sharma S, Arora S, Attri S, Kaur P, Kaur Gulati H, Bhagat K, Kumar N, Singh H, Vir Singh J, et al. New coumarin–benzotriazole based hybrid molecules as inhibitors of acetylcholinesterase and amyloid aggregation. Bioorg Med Chem Lett. 2020;30(20):127477.
- Singh A, Kaur H, Singh H, Singh B, Bedi PMS, Kaur S. Ameliorative effects of Grewia asiatica leaves in animal models of pain and inflammation. J Herbs Spices Med Plants. 2022;29(1):63–72.
- Schrödinger Release 2021-3: protein preparation wizard. New York (NY)/New York (NY)/New York (NY): Epik, Schrödinger, LLC/Impact, Schrödinger, LLC/Prime, Schrödinger, LLC; 2021.
- Schrödinger Release 2021-3: LigPrep. New York (NY): Schrödinger, LLC; 2021.
- Shahzadi I, Zahoor AF, Tüzün B, Mansha A, Anjum MN, Rasul A, Irfan A, Kotwica-Mojzych K, Mojzych M. Repositioning of acefylline as anti-cancer drug: synthesis, anticancer and computational studies of azomethines derived from acefylline tethered 4-amino-3-mercapto-1,2,4-triazole. PLOS One. 2022;17(12):e0278027.
- Schrödinger Release 2021-3: QikProp. New York (NY): Schrödinger, LLC; 2021.