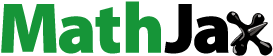
Abstract
This study aims to investigate the phytoconstituents of the chloroform fraction of three Cystoseira spp. namely C. myrica, C. trinodis, and C. tamariscifolia using UPLC/ESI/MS technique. The results revealed the identification of 19, 20 and 11 metabolites in C. myrica, C. trinodis, and C. tamariscifolia, respectively mainly terpenoids, flavonoids, phenolic acids and fatty acids. Also, an in vitro antioxidant study using FRAP and DPPH assays was conducted where the chloroform fraction of C. trinodis displayed the highest antioxidant activity in both assays, which would be attributed to its highest total phenolics and total flavonoids. Besides, the investigation of COX-1, α-glucosidase and α-amylase inhibitory activities were performed. Regarding C. trinodis, it showed the strongest inhibitory activity towards COX-1. Moreover, it showed potent inhibitory activity towards α-glucosidase and α-amylase enzymes. According to the molecular docking studies, the major compounds characterised showed efficient binding to the active sites of the target enzymes.
Introduction
In recent times, marine organisms have emerged as a crucial source of bioactive compounds that possess remarkable biological effects owing to their diverse nature. One particular class of marine organisms, namely macroalgae or seaweeds, has gained significant importance in this regard due to its abundance of bioactive compounds. The broad range of biological activities exhibited by marine macroalgae makes it one of the most significant groups in the marine ecosystemCitation1–4. Marine macroalgae exhibited a plethora of biological effects such as antioxidant, anti-inflammatory, cytotoxic, antimicrobial and antidiabetic effectsCitation2,Citation5–7. In addition, macroalgae produce different secondary metabolites of mixed biogenesis with a vast range of structural diversity to protect themselves and as a defense mechanism against their environment such as terpenoids and phenolicsCitation1,Citation8.
Genus Cystoseira is one of the famous macroalgae that belongs to Phaeophyta (brown macroalgae), family Sargassaceae and the order Fucales that comprises about 50 species worldwide that are distributed across subtropical waters, with the largest diversity and abundance of species found in the Mediterranean SeaCitation9,Citation10. Previous studies on members of the Cystoseira genus have led to the discovery of a diverse range of biological activities, including antioxidant, anti-inflammatory, cytotoxic, antidiabetic, and antimicrobial propertiesCitation1,Citation5,Citation6,Citation11. Besides, Cystoseira brown macroalgae possess different secondary metabolites specifically terpenoids, diterpenes, meroterpenoids and flavonoidsCitation12–16. Recently, the dependence on natural products to manage several diseases is of great importance. For example, natural secondary metabolites have the potential to regulate blood sugar levels and serve as effective antihyperglycemic agents through inhibition of key enzymes α-glucosidase and α-amylaseCitation17,Citation18. Besides, the quest for alternative anti-inflammatory drugs and medications has led to a focused search among marine seaweed. Natural marine products that exhibit both anti-inflammatory and antioxidant activities have garnered significant attention due to their potential for pharmacological useCitation19–21.
The Cystoseira thallus consists of enduring base components, namely the holdfast and the main axis, which are predominantly flattened into 'foliar expansions’ or basal leaves. These leaves can either be spiny or leaf-like. The receptacles, found at the tips of branches above the aerocysts, are the fertile sections of the annual thallus that may contain air vesicles. The number of secondary axes increases exponentially with main axis length, suggesting environmental constraints may limit central axis growth within a 44 cm size at the greatest frequency. These algae possess not only chlorophyll pigments, but also xanthophyll and fucoxanthin pigments. The brown colour of brown seaweeds is attributed to the presence of xanthophyll and fucoxanthin pigmentsCitation22–25. The colour of Cystoseira varies across different species and undergoes some degree of variation over the lifespan of the thallus. Certain species, like C. myrica, exhibit a dark brown colour. Species like C. trinodis and C. tamariscifolia also have a greenish-blue brown colourationCitation22,Citation26. The photos of the three Cystoseira spp. Under study are illustrated in the (Figure S1).
Therefore, we continue our ongoing exploration for secondary compounds derived from Cystoseira spp. In this study, we present the results of our investigation of the chloroform fraction phytochemical composition and variations between three Cystoseira spp.; namely C. myrica, C. trinodis, and C. tamariscifolia along with their antioxidant activity, inhibitory effects against α-glucosidase, α-amylase and cyclooxygenase I enzymes. Furthermore, the study intends to assess the binding affinities between the identified major components and the tested enzymes using molecular docking studies.
Materials and methods
Plant material
Cystoseira myrica, C. trinodis and C. tamariscifolia, three samples of brown algae were collected from the shores of the Gulf of Suez in Ras Sedr city, Egypt 29°50′19.7′N and 32°37′36.8′E in October 2020. A voucher sample was identified according to Ibraheem et al.Citation27 and deposited at the Pharmacognosy Department, Faculty of Pharmacy, Badr University in Cairo under code of BUC-PHG-CM-3, BUC-PHG-CT-4 and BUC-PHG-CT-5, respectively. To prevent evaporation, the samples were transported to the laboratory using sterile plastic bags filled with seawater. Subsequently, the algae were carefully rid of epiphytes and rock debris, and any surface salts were removed through a brief rinsing with freshwater.
Preparation of the plant extract
The grounded air-dried C. myrica, C. trinodis and C. tamariscifolia, (100 g) were macerated in methanol three times at room temperature followed by concentration by evaporation under reduced pressure to yield a dark brown residue. Separately, the crude extract of each species was suspended in distilled water and fractionated using chloroform to yield the chloroform fraction that was concentrated under reduced pressure an low temperature (40 °C) using a rotary evaporator (Hei-VAP Value, Heidolph) to yield a dark brown sticky residue that weight for each sample 1.15, 1.35 and 1.30 g, respectively that was stored at 4 °C for further analysisCitation28–30.
UPLC-ESI-MS analysis
Metabolite profiling of the three species chloroform fraction was carried out using UPLC-ESI-MS analysis at the Centre of Drug Discovery Research and Development, Department of Pharmacognosy, Faculty of Pharmacy, Ain Shams University, Egypt, according to the previously reported methodCitation3,Citation31. The experimental procedure involved utilising a Waters®TQD UPLC-MS instrument equipped with an ESI source and a Waters® acquity UPLC RP-C18 column, (100 × 2 mm, ID), and featuring a particle size of 1.7 µm. Additionally, an integrated precolumn was employed during the analysis. A gradient of acetonitrile and water was applied, ranging from 2% to 100%, in addition to 0.1% formic acid. One run took 35 min, with a flow rate of either 1 or 0.5 ml/min. For ESI-, the MS was run at −10 V, with a source temperature of 240 °C. High purity N2 was used as an auxiliary gas and sheath at 80 and 40 (arbitrary units) flow rates, respectively. The volume of the injection was 5 µL. The spray voltage utilised was 4.48 kilovolts (kV), the tube lens voltage was 10.00 volts (V), and the capillary voltage was 39.6 V. The samples were dissolved in methanol of HPLC grade and subsequently filtered using a PTFE (polytetrafluoroethylene) membrane with a pore size of 0.2 µm. The mass spectrometry analysis was conducted within the m/z range of 100 to 1200. Tentative identification of metabolites was attained by comparing the mass and spectral data of the identified compounds, obtained under negative ionisation mode, with previously reported data from the genus and the family and online public databases. The acquisition and analysis of data were performed using the XcaliburTM 2.0.7 software (Thermo Scientific, Karlsruhe, Germany).
Total phenolics and flavonoids content
The quantification of both total phenolic (TPC) and flavonoid contents (TFC) in the chloroform fractions was carried out using spectrophotometric techniques, specifically the Folin-CiocalteuCitation32,Citation33 and AlCl3 methodsCitation34, respectively. Briefly, to determine the TPC, the Folin-Ciocalteau method was employed, involving the mixing of 5 µL of plant extract with a volume of 3 ml of Folin-Ciocalteau reagent (10%) and 0.8 ml of sodium bicarbonate (7.5%).
Following the mixing of the reaction solution, it was incubated at room temperature for 30 min. The absorbance of the resulting mixture was then measured at 765 nm using a Milton Roy (Spectronic 1201) spectrophotometer. The TPC was expressed as mg gallic acid equivalents (GAE)/g extract. For the TFC analysis, 0.1 ml of the extract was mixed with 3.90 ml of distilled water, followed by the addition of 0.3 ml of a 5% sodium nitrite solution. After allowing the mixture to react for 5 min, 0.3 ml of a 10% aluminium chloride solution was added. The resulting mixture was allowed to react for an additional 6 min. Subsequently, 2 ml of 1 mM−1 sodium hydroxide solution was added to the mixture, followed by the addition of 2.4 ml of distilled water to all samples. The absorbance of the samples was measured at 510 nm using a Milton Roy (Spectronic 1201) spectrophotometer, with a sample blank without any reaction serving as the reference. The TFC of the extracts are expressed as mg quercetin equivalents (QE)/g extract.
Biological evaluation
In vitro assessment of antioxidant activity using DPPH and FRAP assays
Two methods were employed in this study to evaluate the antioxidant potential of the chloroform fractions of the three species under study. These included the use of 1,1-diphenyl-2-picrylhydrazyl (DPPH) radical scavenging assayCitation35,Citation36 and the ferric ion reducing antioxidant power (FRAP) assayCitation37.
DPPH radical scavenging assay
In DPPH Radical Scavenging Activity, A freshly prepared methanol solution of 2,2-diphenyl-1-picrylhydrazyl (DPPH) radical with a concentration of 0.004% w/v was prepared and kept in the dark at a temperature of 10 °C. The tested fraction was dissolved in methanol to prepare a sample solution. Followed by, a 40 µL volume of this sample was added to 3 ml of the DPPH solution. The absorbance readings were taken immediately using a UV-visible spectrophotometer (Milton Roy, Spectronic 1201). The decrease in absorbance at 515 nm was monitored continuously, with data being recorded at 1-min intervals until the absorbance reached a stable level. The absorbance of the DPPH radical solution without the presence of any antioxidant (control) and the reference compound ascorbic acid were also investigated. All measurements were conducted in triplicate, and the obtained values were averaged. The percentage inhibition (PI) of the DPPH radical was calculated according to the formula:
Where AC = Absorbance of the control at t = 0 min and AT = absorbance of the sample + DPPH at t = 16 min. The 50% inhibitory concentration (IC50), the concentration required to inhibit DPPH radical by 50%, was estimated from graphic plots of the dose response curve.
FRAP scavenging assay
In Ferric reducing antioxidant power (FRAP), the conversion of ferric ions to ferrous ions by the extract indicates its potential antioxidant activity. This method involves the use of different concentrations of the extract sample to reduce ferricyanide. Samples in 1 ml of methanol were mixed with 2.5 ml of 0.2 M sodium phosphate buffer (pH 6.6) and 2.5 ml of potassium ferricyanide [K3Fe (CN)6] (1%, w/v). Following a 20 min incubation at 50 °C, the reaction mixture was treated with 2.5 ml of trichloroacetic acid (10%, w/v). The reaction mixture was centrifuged, and the supernatant solution (2.5 ml) was mixed with 2.5 ml of deionised water and 0.5 ml of freshly prepared ferric chloride (0.1%, w/v). The resulting solution’s absorbance was measured at 700 nm, with a blank serving as the reference, using a spectrophotometer (Milton Roy, Spectronic 1201). Ascorbic acid was used as reference standard. The reducing capability percentage (%) was calculated as follows, according to Canabady-Rochelle et al. 2015Citation38.
A0: absorbance of the control solution and As: sample absorbance.
In vitro assessment of anti-hyperglycaemic activity
α-amylase inhibition assay
The method used to evaluate the a-amylase inhibitory activity of the tested chloroform fractions was based on a previously reported procedure and involved the use of BioVision’s α-amylase inhibitor screening kit (K482-100)Citation39–41. In this experiment, 500 µL of both test samples and acarbose (1000–7.81 μg/mL) were combined with a 0.20 mM phosphate buffer solution (pH 6.9) containing a 0.5 mg/mL concentration of α-amylase. After mixing, the resulting solution was incubated at 25 °C for 10 min. Subsequently, 500 µL of a 1% starch solution in 0.02 M sodium phosphate buffer (pH 6.9) was introduced into each tube. The reaction mixtures were left to incubate at 25 °C for 10 min, after which the reaction was stopped by adding 1.0 ml of the 3,5-dinitro-salicylic acid colour reagent. Afterward, the test tubes were incubated in a boiling water bath for 5 min, then allowed to cool to room temperature. Following this, the reaction mixture was diluted by adding 10 ml of distilled water, and the absorbance was measured at 540 nm. For the control group, water was used in place of the extracts to represent 100% enzyme activity. All measurements were conducted in triplicate, and the resulting values were expressed as the mean ± standard deviation (SD). Using Acarbose as a standard drug and the concentration of the α-amylase and α-glucosidase inhibitor required to inhibit 50% of its activity under the conditions of the assay was determined as the IC50 value.
α-glucosidase inhibition assay
The method used to evaluate the a-glucosidase inhibitory activity was based on a previously reported procedure and involved the use of BioVision’s α-glucosidase inhibitor screening kit (K938-100)Citation42. To begin the experiment, approximately 10 µL of the extract being tested was combined with glutathione (10 µL), α-glucosidase solution (10 µL) in a phosphate buffer solution (pH 6.8), and PNPG (4-nitrophenyl-α-D-glucopyranoside) (10 µL) in a 96-well microplate. The resulting mixture was then incubated for 15–20 min at room temperature.
In a similar manner, a blank was prepared by adding the sample solution to all the reaction reagents except for the α-glucosidase solution. The reaction relied on the activity of an active α-glucosidase enzyme to break down a synthetic substrate (PNPG), which results in the release of a chromophore (p-nitrophenol; OD = 410 nm). The reaction was halted by adding 50 µL of 0.2 M sodium carbonate. The absorbance of both the tested oil and the blank was measured at 410 nm. The absorbance value of the blank was subtracted from that of the tested oil, and the resulting value was used to determine the IC50 value.
Assessment of anti-inflammatory activity (cyclooxygenase inhibition assay)
The cyclooxygenase inhibition assay was conducted using COX-1 Inhibitor Screening Kit (Catalog number K548-100, Milpitas, CA 95035 USA). The assay was carried using different concentrations of the tested sample (from 0.5 to 1000 μg/mL, in a geometric sequence) were preincubated with the cyclooxygenase enzyme (COX-1) at room temperature (25 °C) for 5 min in the presence of haematin. Phenol (500 μM), 1-leuco-dichlorofluorescein (20 μM), arachidonic acid (50 μM) and haematin (1 μM) in 1 ml of 0.1 M Tris-buffer (pH 8) were pre-mixed and added to the enzyme mixture. The spectrophotometric measurement was performed using a Milton Roy (Spectronic 1201) instrument at 502 nm, and the absorbance was recorded within 15 s of the sample addition. A reference standard of Celecoxib was used, and a control sample was prepared without the addition of enzymes. The IC50 value (μg/mL), which is the concentration of the extract that inhibited 50% of cyclooxygenase -I that was calculated from the curveCitation43.
2.6. Molecular Modelling
In the present work, Vina, Autodock and MGL tools were implemented for conducting the molecular docking. Sixteen major compounds of the studied Cystoseira spp. were sketched and converted into 3D structures using Discovery Studio. The X-ray crystallographic structures of α-amylase, α-glucosidase, COX-1 & tyrosinase enzymes were downloaded from protein data bank (PDB) (www.pdb.org) accessed on 15 July 2023 using the following IDs: 7TAA, 7KB8, 5WBE and 5M8QCitation44–47, respectively. Docking investigations were performed using MOE 2019Citation48,Citation49, which was also employed to generate 2D interaction diagrams illustrating the docking of ligands with potential targets. Each protein file’s co-crystallized ligand was used to identify the binding site of the enzymes where the docking was conducted. Finally, 16 major compounds of the extract were docked using triangle matcher as a placement method and London dG as a scoring algorithm.
Results and discussion
UPLC-ESI-MS analysis for characterization of Cystoseira myrica, C. trinodis and C. tamariscifolia chloroform fractions
The metabolic profiling of the three species of Cystoseira is illustrated in () and resulted in identification of 19, 20 and 11 compounds, respectively that belongs to different classes of secondary plant metabolites. The total ion chromatogram (TIC) in negative ionisation mode is represented in (Figure S2). The compounds were identified by analysing their mass data and comparing them with previously described compounds in existing literature.
Table 1. Metabolite profiling of C. myrica, C. trinodis and C. tamariscifolia Chloroform fraction using UPLC-ESI-MS in the negative ion mode.
Generally, the tentatively identified compounds belong to terpenoids, flavonoids, phenolic acids and fatty acids. The terpenoids represent the major class of metabolites in the chloroform fraction of the three species. Two acyclic diterpenes were characterised as (2E, l0E)-1-hydroxy-6,13-diketo-7-methylene-3,11,15-trimethylhexadeca-2, l0, l4-triene (3) and (2E,10E)-1,6-dihydroxy-7-methy1ene-13-keto-3,11,15-trimethylhexadeca-2,10,14-triene (24) with molecular formula of C20H30O3 and C20H32O3 and mass ion peaks at 317.10 and 319.30, respectively which were characterised in C. myrica and C. trinodis and formerly identified from C. crinitaCitation50. Moreover, the mass ion peak at m/z 295.30, corresponding to the predicted molecular formula C18H32O3, was identified as phytol (22), earlier characterised from C. tamariscifoliaCitation51.
Two meroditerpenoids were characterised with ion mass peaks at m/z 251.10 and 557.35 corresponding to the predicted molecular formula C16H28O2 (12) and C32H45O8 (14), respectively, they were identified previously from C. baccata and C. usneoidesCitation15,Citation52 Whereas that at m/z 357.20 for the suggested molecular formula C22H30O4 was dereplicated as nor sesquiterpenoid; cystomexicone A or B (8) that was formerly characterised from C. abies marinaCitation53. Diterpene pachydictyol A (21) was dereplicated as major component in C. tamariscifolia with ion mass peak at m/z 287.30 corresponding to the molecular formula C20H32O. This compound was formerly isolated from C. myricaCitation12. Phloroglucinol − meroterpenoid hybrids were characterised as cystophloroketal A and B (31 & 33) with same mass ion peak at m/z 563.45 corresponding to the predicted molecular formula C34H44O7, were isolated previously from C. tamariscifoliaCitation54. Regarding polyphenolic compounds as phenolic acids and flavonoids, the mass ion peaks at m/z 197.85, 325.30 and 559.40 in accordance with the molecular formula C9H10O5, C15H17O8 and C27H28O13, were dereplicated as syringic acid (5), p-coumaric acid glucoside (15) and 1-O-caffeoyl-3-O-sinapoylquinic acid (19), respectively where phenolic acids were previously characterised from brown algae different species as Thalassiophyllum, Fucus and CystoseiraCitation55,Citation56. Moreover, the identified flavonoids were more abundant in C. myrica and C. trinodis characterised as quercetin and its gallic acid derivative (20 & 25), naringin (11), neoeriotrin (13) while 4′,7-dimethoxyluteolin (6) was dereplicated in C. tamariscifolia as shown in (). Another ion mass peak at m/z 535.40 was dereplicated as 3[A]chlorobifuhalol hexacetate (23) which belongs to phlorotannins and in agreement with the molecular formula of C24H21ClO12 that was previously identified from the brown algae Carpophyllum angustifolium (Sargassaceae)Citation57.
Furthermore, six fatty acids were characterised in the three species namely, eicosaenoic acid (10), docosanedioic acid (16) and hydroxy-linolenic acid (18). Where, octadecanoic acid, ethyl ester (7), dihydroxy hexadecanoic acid (26) and octadecatrienoic acid (33) identified specifically in C. tamariscifolia. Fatty acids especially the polyunsaturated fatty acids representes as main metabolites as formerly reported in six different Cystoseira spp.Citation58. The chemical structures of the major characterised metabolites in three Cystoseira spp. are illustrated in ().
Total phenolics and flavonoids content
The total phenolic and flavonoid content of the chloroform fraction of C. myrica, C. trinodis and C. tamariscifolia were calculated as mg/g gallic acid equivalents (GAE) and mg/g quercetin equivalents (QE), respectively and the results are represented in (). The chloroform fraction of C. trinodis displayed the highest total phenolic content (TPC) 17.51 ± 0.85 mg/g GAE, followed by C. myrica (16.75 ± 1.03 mg/g GAE) and C. tamariscifolia (13.34 ± 0.62 mg/g GAE). The total flavonoid content values exhibited comparable patterns to the total phenolic content values. Where, the chloroform fraction of C. trinodis exhibited the highest total flavonoid content (TFC) 35.48 ± 3.14 mg/g QE, followed by C. myrica (25.41 ± 0.84 mg/g QE) and the lowest TFC was that of C. tamariscifolia (9.76 ± 1.25 mg/g QE). A previous study by Mansur et al. 2020 on C. tamariscifolia collected from shallow subtidal at Hannafore Point, Cornwall, UK revealed that the chloroform fraction TFC ranged from 16.69 ± 0.52 to 49.21 ± 4.83 in the four seasons and TPC ranged from 7.81 ± 0.31 to 68.75 ± 2.79 in the four seasons, where the highest content in the SpringCitation16.
Table 2. Total phenolic and flavonoid content of C. myrica, C. trinodis and C. tamariscifolia chloroform fraction.
In vitro biological activities
Antioxidant activity using DPPH and FRAP assay
Plants are believed to hold potential as a valuable resource for uncovering new and unique compounds. By exploring natural plants and isolating their compounds, we can come across numerous biologically active substances that serve as promising starting points for developing medications. That would be achieved through investigation of the secondary metabolites and plant extracts potential in the various cytotoxic, antioxidant, anti-inflammatory, antimicrobial and antiviral assaysCitation4,Citation59–61. The antioxidant potential of the three chloroform fractions under study revealed a potent efficacy on DPPH and FRAP radical scavenging as illustrated in (). The IC50 value of the chloroform fraction of C. trinodis (13.79 ± 1.09 µg/mL) for DPPH assay was comparable to that of the standard drug ascorbic acid (10.22 ± 0.64 µg/mL). Also, C. trinodis showed significantly stronger FRAP scavenging potency (IC50 = 26.32 ± 1.97 µg/mL) than the C. tamariscifolia. On the other hand, C. myrica exhibited the lowest antioxidant potential in DPPH assay and no activity on FRAP assay. Resveratrol is widely known as an antioxidant, anti-inflammatory moleculeCitation62 that is present in high percentage in C. trinodis. Resveratrol showed the most powerful inhibition with an EC50 value of 85 ± 2.7 µM in DPPH scavenging radical assayCitation63. Also, syringic acid and 1-O-caffeoyl-3-O-sinapoylquinic acid are phenolic acids well known for their antioxidant propertiesCitation64. In addition to the contribution of phenolics to the anti-oxidant properties of C. trinodis, the presence of mertoterpenoids, specifically cystophloroketal B, enhances its antioxidant activityCitation65.
Table 3. Antioxidant activity (IC50) of C. myrica, C. trinodis and C. tamariscifolia chloroform fraction using DPPH and FRAP assays.
Previous studies investigated the antioxidant activity of different Cystoseira spp.Citation5,Citation6,Citation66,Citation67. Abu-Khudir et al. 2021 found that the 80% methanol extract of C. crinita exhibited antioxidant activity, as demonstrated by its performance in DPPH and ABTS assays. The extract displayed IC50 values of 125.6 µg/mL and 254.8 µg/mL for DPPH and ABTS, respectivelyCitation6. Čagalj et al. 2022 reported the antioxidant activity of C. compressa across different seasons using different assays and the results revealed that all samples exhibited strong free radical scavenging activity and DPPH inhibition, with values exceeding 80%. Notably, the August sample demonstrated the highest activity, as evidenced by its ORAC value of 72.1 ± 1.2 µM TECitation66. Also, another study by Mhadhebia et al. reported the antioxidant activity of three Cystoseira spp. namely, C. crinita, C. sedoides and C. compressa by using DPPH and FRAP assaysCitation7. In DPPH assay, the aqueous extract of C. compressa showed highest potency followed by C. crinita and C. sedoides with IC50 values of 12.0 ± 0.7, 20.0 ± 0.5 and 75.0 ± 0.8 µg/mL, respectively as compared to Trolox with IC50 of 90.0 ± 0.2 µg/mL. In the FRAP assay, C. compressa exhibits a significantly higher capacity to eradicate Fe3+ compared to C. crinita, with values of 2.6 and 0.9 mg GAE/g dried sample, respectively.
Anti-hyperglycaemic activity through α-amylase and α-glucosidase inhibition
The efficacy of the three tested chloroform fractions towards the inhibition of α-glucosidase and α-amylase are recognised as one of the most important enzymes involved in diabetes management. Many reports revealed the dependence on natural products to control the hyperglycaemia associated with type 2 diabetes mellitus especially through inhibition of α-glucosidase and α-amylase enzymesCitation40,Citation68–70. As shown in (), In terms of our findings, the chloroform fraction of C. trinodis showed remarkable inhibition of α-glucosidase and α-amylase with an IC50 value of 20.29 ± 1.87 and 24.81 ± 2.34 µg/mL, respectively. Followed by C. tamariscifolia with an IC50 value of 28.51 ± 1.87 and 46.29 ± 2.91 µg/mL, respectively. While the lowest activity was recognised for C. myrica with an IC50 value of 46.91 ± 3.68 and 68.71 ± 4.09 µg/mL. the results were compared to the standard drug acarbose (IC50 = 3.12 ± 0.28 and 12.29 ± 0.73 µg/mL) towards α-glucosidase and α-amylase, respectively (Figure S3). A previous study revealed that resveratrol, a key component of C. trinodis, had an IC50 values of 376.22 and 99.18 nm against α-amylase and α-glucosidase, respectivelyCitation65. These values indicate the highest activity of C. trinodis compared to other species examined in the study. Previous study by Çelenk and Sukatar (2020) showed that three Cystoseira spp. namely, C. barbata, C. compressa and C. crinita have a potent α-glucosidase inhibition rates 90.7 ± 0.01, 89.8 ± 0.02 and 91.9 ± 0.00, respectivelyCitation8.
Table 4. Anti-hyperglycaemic activity and Anti-inflammatory activity % (IC50) of C. myrica, C. trinodis and C. tamariscifolia chloroform fraction.
Anti-inflammatory activity
The anti-inflammatory potential of the three chloroform factions was investigated as the inflammation process is linked to many chronic ailments as ageing, gastritis, diabetes, hypertension, cardiovascular disease, rheumatoid and cancerCitation71,Citation72. As presented in (), the findings were evaluated using the percentage of inhibition and given as mean ± standard deviation and compared to the standard drug clelcoxibe with an IC50 value equals to 9.07 ± 0.67 µg/mL. The results revealed that the three chloroform fractions showed a promising anti-inflammatory activity through inhibition of COX-1 expression. It was notable that the chloroform fraction of C. trinodis showed the highest activity with IC50 =16.13 ± 0.89, followed by C. myrica IC50 =19.76 ± 1.46 and C. tamariscifolia had the lowest anti-inflammatory efficacy IC50 =52.19 ± 3.12 µg/mL (Figure S4). Our results were in accordance with the previous reports concerning the anti-inflammatory effects of different Cystoseira spp. 7,Citation8,Citation71. The extracts obtained from Cystoseira amentacea var. stricta using ethanol and DMSO exhibited potent inhibition of NO overproduction, with EC50 values ranging from 546 to 1293 µg/mL. Moreover, these extracts strongly suppressed the production of inflammatory mediators such as IL-1, IL-6, cyclooxygenase-2 and inducible NO synthase gene expression in RAW 264.7 macrophages that were induced by LPSCitation71. Another study by Mhadhebia et al. reported the anti-inflammatory potency of three Cystoseira spp. namely, C. crinita, C. sedoides and C. compressa. The three species revealed anti-inflammatory activity in-vivo, using carrageenan induced rat paw oedema assay at doses of 25 or 50 mg/Kg i.p.Citation7. The findings of the current study are consistent with earlier reports on various species of Cystoseira that have demonstrated antioxidant, antidiabetic, and anti-inflammatory effects.
Molecular modelling
This part was carried out to explore the potential mechanism by which the identified key compounds exert their biological effects. Accordingly, the 3D structures of α-amylase, α-glucosidase, COX-1 & tyrosinase enzymes were obtained from the Protein Data Bank (PDB) with the following IDs: 7TAA, 7KB8, 5WBE and 5M8Q, respectively. Subsequently, the sixteen major compounds were subjected to docking within the vicinity of the active sites of the four enzymes. Remarkably, all the compounds exhibited satisfactory binding scores during the docking process with the four proteins ().
Table 5. The docking scores achieved by the major identified compounds towards various enzymes.
Starting with the docking of the major compounds with the α-amylase, 2″,3″-dihydro-3′,3′″-biapigenin methyl ether, naringin, and 4′,7-dimethoxyluteolin were the best compounds achieving docking scores of −14.9, −13.9 and −13.6 Kcal/Mol, respectively. Inspecting the interaction with α-amylase (), 2″,3″-dihydro-3′,3′″-biapigenin methyl ether formed six hydrogen bond interactions with Ile152, Tyr155, Gly167, His296, Asp297, and Arg344, in addition to a hydrophobic interaction with Asp340. Naringin formed two hydrogen bond interactions with Gln35 and Arg204, in addition to group of hydrophobic interactions with His80, Tyr82, Trp83, Glu230, Leu232, His296, Asp297 and Asp340, while 4′,7-dimethoxyluteolin formed four hydrogen bond interactions with Gln35, Asp206 and Arg344, in addition to hydrophobic interactions with Gly584, Asp586 and Trp801.
Figure 2. (I) 2D binding modes of (A) 4′,7-Dimethoxyluteolin, (B) 2″,3″-Dihydro-3',3'″-biapigenin methyl ether, (C) Naringin to the active binding sites of α-amylase enzyme. (II) 2D binding modes of (A) 4′,7-dimethoxyluteolin, (B) 2″,3″-dihydro-3',3'″-biapigenin methyl ether, (C) Naringin to the active binding sites of α-glucosidase enzyme.

Regarding the docking of the major compounds with the α-glucosidase, naringin, 2′',3″-Dihydro-3′,3′″-biapigenin methyl ether, and 4′,7-dimethoxyluteolin were the best compounds achieving docking scores of −17.24, −14.88 and −13.38 Kcal/Mol, respectively. Inspecting (), it was found that, naringin formed five hydrogen bond interactions with Asp304, Tyr318, Asp451 and Asp640, besides it formed several hydrophobic interactions with Gln308, Trp562, Asp564, Trp637, His698 and His700, 2″,3″-Dihydro-3′,3′″-biapigenin methyl ether formed many hydrophobic interaction with Trp525, Trp562, Phe571, Phe673, His698 and His700, while 4′,7-dimethoxyluteolin formed two hydrogen bond interactions with Asp451 and Asp640, moreover it formed several hydrophobic interactions with Asp305, Phe307, Trp423, Asp564, Asp669 and Phe673.
Sahnoun et al. reported that the α-amylase’s binding site for naringin exhibited a higher polar contact count, including 47 more than the count observed with acarbose. The binding site of naringin with α-glucosidase exhibited a higher number of polar contacts (45 interactions) compared to acarbose (39 interactions). Acarbose has less residues compared to other substances.The docking investigations indicate that naringin has a greater inhibitory action against α-glucosidase compared to acarbose. The naringin-α-glucosidase enzyme maintains a relatively constant amount of Rg, resulting in the formation of a more stable structureCitation73.
Moreover, the docking investigation of the major compounds with COX-1, naringin, 2″,3″-dihydro-3′,3′″-biapigenin methyl ether, and 4′,7-dimethoxyluteolin were the best compounds achieving docking scores of −20.29, −18.07 and −17.05 Kcal/Mol, respectively. Inspecting (), it was found that, naringin formed one hydrogen bond interaction with Arg120, and it formed several hydrophobic interactions with Ile89, His90, Val349, Leu352, Ser353, Tyr355, Ile523, and Ala527, 2″,3″-Dihydro-3′,3′″-biapigenin methyl ether formed three hydrogen bond interactions with Tyr355, Tyr385 and Ser530, additionally, it formed several hydrophobic interactions with Ile89, Arg120, Val349, Trp387, Met522, Ile523, Gly526 and Ala527, while 4′,7-dimethoxyluteolin formed four hydrogen bond interactions with Arg120, Tyr385 and Ser530, moreover it formed several hydrophobic interactions with Val349, Leu352, Gly526 and Ala527.
Figure 3. (I) 2D binding modes of (A) 4',7-Dimethoxyluteolin, (B) 2'',3''-Dihydro-3',3'''-biapigenin methyl ether, (C) Naringin to the active binding sites of COX-1 enzyme. (II) 2D binding modes of (A) Cystophloroketal B, (B) 2'',3''-Dihydro-3',3'''-biapigenin methyl ether, (C) Quercetin to the active binding sites of Tyrosinase enzyme.

Furthermore, in the docking of the major compounds with tyrosinase, 2′',3′'-dihydro-3′,3′″-biapigenin methyl ether, quercetin and cystophloroketal B were the best compounds achieving docking scores of −14.81, −13.01 and −11.81 Kcal/Mol, respectively. Inspecting (), it was found that, 2′',3″-dihydro-3′,3′″-biapigenin methyl ether was engaged in many hydrogen bond interaction with Arg321, Tyr369, Asp370, Ser374, Asn378 and Gly389, besides it formed several hydrophobic interactions with Val196, Asp212, His215, Glu216, Phe362, Leu382, and Val391. Quercetin formed one hydrogen bond interaction with His215 many hydrophobic interactions with Glu216, His381, Leu382, and Val391, while cystophloroketal B was able to engage with various hydrophobic interactions with Lys198, His215, Phe362, His381, and Leu382. According to a previous investigation, it was determined that quercetin had a maximum binding affinity of −7.9 kcal/mol towards tyrosinase. This interaction occurred within the hydrophobic pocket of the enzyme, which was encompassed by specific amino acid residues including Val-248, Met-257, Phe-264, Met-280, Val-283, and Ala-286. Consequently, a strong hydrophobic binding was established between quercetin and the residues. Furthermore, the presence of chelation processes was detected between the quercetin and the two copper atoms, representing the primary interaction between quercetin and tyrosinaseCitation62.
In conclusion, all the major compounds induced a favourable binding with the four targets, especially, 4′,7-dimethoxyluteolin, 2″,3″-dihydro-3′,3′″-biapigenin methyl ether, naringin, quercetin and cystophloroketal B.
Conclusion
The results of this study indicate that the chloroform fraction of the three investigated Cystoseira spp. contain a various secondary metabolites belonging to terpenoids, flavonoids, phenolic acids, and fatty acids, that correlates with their antioxidant and inhibitory activities towards the tested enzymes. The chloroform fraction of C. trinodis showed the highest reducing property in DPPH and FRAP assays, also remarkable inhibition of α-glucosidase, α-amylase, and COX-1 expression. Moreover, through molecular docking studies, the identified major compounds offered a plausible mechanism prediction for the biological effects exerted by the chloroform fractions. Among the major compounds, 4′,7-dimethoxyluteolin, 2′',3″-dihydro-3′,3′″-biapigenin methyl ether, naringin, quercetin and cystophloroketal B induced a favourable binding score with the four enzymes. Eventually, the present study suggest that the Cystoseira spp. show promise as potential candidates for further exploration and the development of potential treatments for chronic conditions such as Alzheimer’s disease, diabetes, and oxidative stress-related disorders. These expected modes of action still need to be confirmed by further mechanistic investigation in the future.
Supplemental Material
Download PDF (584.6 KB)Acknowledgement
The authors extend their appreciation to the Deanship of Scientific Research at King Khalid University for funding this work through Large Groups Project under grant number (RGP.2/311/44).
Disclosure statement
No potential conflict of interest was reported by the author(s).
Additional information
Funding
References
- Aly SH, Elhawary EA, Elissawy AM, Mostafa NM, Eldahshan OA, Singab ANB. 2023. Brown Algae (Phaeophyta) source of different phytochemical and their medicinal application. In: Bachheti A, Bachheti RK, Husen A, editors. Aquatic Medicinal Plants. 1st Edition. England, UK: CRC Press; p. 24.
- Kosanić M, Ranković B, Stanojković T. Biological potential of marine macroalgae of the genus Cystoseira. Acta Biol Hung. 2015;66(4):374–384.
- Aly SH, Elissawy AM, Salah D, Alfuhaid NA, Zyaan OH, Mohamed HI, Singab ANB, Farag SM. Phytochemical investigation of three Cystoseira species and their larvicidal activity supported with in silico studies. Mar Drugs. 2023;21(2):117.
- Elnaggar MS, Elissawy AM, Youssef FS, Kicsák M, Kurtán T, Singab ANB, Kalscheuer R. Austalide derivative from marine-derived Aspergillus sp. and evaluation of its cytotoxic and ADME/TOPKAT properties. RSC Adv. 2023;13(24):16480–16487.
- Sellimi S, Maalej H, Rekik DM, Benslima A, Ksouda G, Hamdi M, Sahnoun Z, Li S, Nasri M, Hajji M. Antioxidant, antibacterial and in vivo wound healing properties of laminaran purified from Cystoseira barbata seaweed. Int J Biol Macromol. 2018;119:633–644.
- Abu-Khudir R, Ismail GA, Diab T. Antimicrobial, antioxidant, and anti-tumor activities of Sargassum linearifolium and Cystoseira crinita from Egyptian Mediterranean Coast. Nutr Cancer. 2021;73(5):829–844.
- Mhadhebi L, Mhadhebi A, Robert J, Bouraoui A. Antioxidant, anti-inflammatory and antiproliferative effects of aqueous extracts of three mediterranean brown seaweeds of the Genus Cystoseira. Iran J Pharm Res. 2014;13(1):207–220.
- De Los Reyes C, Zbakh H, Motilva V, Zubía E. Antioxidant and anti-inflammatory meroterpenoids from the brown alga Cystoseira usneoides. J Nat Prod. 2013;76(4):621–629.
- Valls R, Piovetti L. The chemistry of the Cystoseiraceae (Fucales: Pheophyceae): chemotaxonomic relationships. Biochem Syst Ecol. 1995;23(7-8):723–745.
- Amico V. Marine brown algae of family Cystoseiraceae: chemistry and chemotaxonomy. Phytochemistry. 1995;39(6):1257–1279.
- Çelenk FG, Sukatar A. Macroalgae of izmir gulf: Cystoseira barbata, Cystoseira compressa and Cystoseira crinita species have high α-glucosidase and moderate pancreatic lipase inhibition activities. Iran J Pharm Res. 2020;19(2):391–402.
- Ayyad SEN, Abdel-Halim OB, Shier WT, Hoye TR. Cytotoxic hydroazulene diterpenes from the brown alga Cystoseira myrica. Z Naturforsch C J Biosci. 2003;58(1-2):33–38.
- Fisch KM, Böhm V, Wright AD, König GM. Antioxidative meroterpenoids from the brown alga Cystoseira crinita. J Nat Prod. 2003;66(7):968–975.
- Fattorusso E, Magno S, Mayol L, Santacroce C, Sica D, Amico V, Oriente G, Piattelli M, Tringali C. Oxocrinol and crinitol, novel linear terpenoids from the brown alga Cystoseira crinita. Tetrahedron Lett. 1976;17(12):937–940.
- De Los Reyes C, Ortega MJ, Zbakh H, Motilva V, Zubía E. Cystoseira usneoides: A Brown Alga Rich in Antioxidant and Anti-inflammatory Meroditerpenoids. J Nat Prod. 2016;79(2):395–405.
- Mansur AA, Brown MT, Billington RA. The cytotoxic activity of extracts of the brown alga Cystoseira tamariscifolia (Hudson) Papenfuss, against cancer cell lines changes seasonally. J Appl Phycol. 2020;32(4):2419–2429.
- Malik JA, Aly SH, Hajam YA, Quazi S. 2022. How Metabolomics Shapes the Future of Drugs in Antidiabetic Plants. In: Antidiabetic Potential Plants Era Omi. United States: Apple Academic Press; p. 353–372.
- Etxeberria U, de la Garza AL, Campión J, Martínez JA, Milagro FI. Antidiabetic effects of natural plant extracts via inhibition of carbohydrate hydrolysis enzymes with emphasis on pancreatic alpha amylase. Expert Opin Ther Targets. 2012;16(3):269–297.
- Sakthivel R, Devi KP. Antioxidant, anti-inflammatory and anticancer potential of natural bioactive compounds from seaweeds. Stud Nat Prod Chem. 2019;63:113–160.
- Lim CS, Jin DQ, Sung JY, Lee JH, Choi HG, Ha I, Han JS. Antioxidant and anti-inflammatory activities of the methanolic extract of Neorhodomela aculeate in hippocampal and microglial cells. Biol Pharm Bull. 2006;29(6):1212–1216.
- Maneesh A, Chakraborty K, Makkar F. Pharmacological activities of brown seaweed Sargassum wightii (Family Sargassaceae) using different in vitro models. Int J Food Prop. 2017;20(4):931–945.
- Hagaggi NSA, Abdul-Raouf UM. Macroalga-associated bacterial endophyte bioactive secondary metabolites twinning: Cystoseira myrica and its associated Catenococcus thiocycli QCm as a model. World J Microbiol Biotechnol. 2022;38(11):205.
- Gómez Garreta A, Gallardo T, Ribera MA, Cormaci M, Furnari G, Giaccone GB. Checklist of Mediterranean Seaweeds. Bot Mar. 2001;44(2001):425–460.
- Ramus J, Beale SI, Mauzerall D, Howard KL. Changes in photosynthetic pigment concentration in seaweeds as a function of water depth. Mar Biol. 1976;37(3):223–229.
- Prasanna R, Sood A, Suresh A, Nayak S, Kaushik BD. Potentials and applications of algal pigments in biology and industry. Acta Bot Hung. 2007;49(1-2):131–156.
- Bouafif C, Verlaque M, Langar H. Cystoseira taxa new for the marine flora of Tunisia. Cryptogam Algol. 2014;35(3):269–283.
- Ibraheem IBM, Alharbi RM, Abdel-Raouf N, Al-Enazi NM. Contributions to the study of the marine algae inhabiting Umluj Seashore, Red Sea. Beni-Suef Univ J Basic Appl Sci. 2014;3(4):278–285.
- El-Nashar HAS, Sayed AM, El-Sherief HAM, Rateb ME, Akil L, Khadra I, Majrashi TA, Al-Rashood ST, Binjubair FA, El Hassab MA, et al. Metabolomic profile, anti-trypanosomal potential and molecular docking studies of Thunbergia grandifolia. J Enzyme Inhib Med Chem. 2023;38(1):2199950.,.
- Chougale AD, Ghadyale VA, Panaskar SN, Arvindekar AU. Alpha glucosidase inhibition by stem extract of Tinospora cordifolia. J Enzyme Inhib Med Chem. 2009;24(4):998–1001.
- Djeridane A, Yousfi M, Nadjemi B, Boutassouna D, Stocker P, Vidal N. Antioxidant activity of some algerian medicinal plants extracts containing phenolic compounds. Food Chem. 2006;97(4):654–660.
- Aly SH, Elissawy AM, Mahmoud AMA, El-Tokhy FS, Mageed SSA, Almahli H, Al-Rashood ST, Binjubair FA, Hassab MAE, Eldehna WM, et al. Synergistic Effect of Sophora japonica and Glycyrrhiza glabra Flavonoid-Rich Fractions on Wound Healing: In Vivo and Molecular Docking Studies. Molecules. 2023;28(7):2994.
- Singleton VL, Rossi JA. Colorimetry of total phenolics with phosphomolybdic-phosphotungstic acid reagents. Am J Enol Vitic. 1965;16(3):144–158.
- Singleton VL, Orthofer R, Lamuela-Raventós RM. 1999. Analysis of total phenols and other oxidation substrates and antioxidants by means of folin-ciocalteu reagent. In: Methods Enzymol. Vol. 299. Elsevier; p. 152–178.
- Chang C-C, Yang M-H, Wen H-M, Chern J-C. Estimation of total flavonoid content in propolis by two complementary colorimetric methods. J Food Drug Anal. 2002;10(3):178–182.
- Yen GC, Duh PD. Scavenging effect of methanolic extracts of peanut hulls on free-radical and active-oxygen species. J Agric Food Chem. 1994;42(3):629–632.
- Al Zahrani NA, El-Shishtawy RM, Elaasser MM, Asiri AM. Synthesis of novel chalcone-based phenothiazine derivatives as antioxidant and anticancer agents. Molecules. 2020;25(19):4566.
- Ferreira ICFR, Baptista P, Vilas-Boas M, Barros L. Free-radical scavenging capacity and reducing power of wild edible mushrooms from northeast Portugal: Individual cap and stipe activity. Food Chem. 2007;100(4):1511–1516.
- Canabady-Rochelle LLS, Harscoat-Schiavo C, Kessler V, Aymes A, Fournier F, Girardet J-M. Determination of reducing power and metal chelating ability of antioxidant peptides: revisited methods. Food Chem. 2015;183:129–135.
- Mohammed HS, Abdel-Aziz MM, Abu-Baker MS, Saad AM, Mohamed MA, Ghareeb MA. Antibacterial and potential antidiabetic activities of flavone C-glycosides isolated from Beta vulgaris subspecies cicla L. var. flavescens (Amaranthaceae) cultivated in Egypt. Curr Pharm Biotechnol. 2019;20(7):595–604.
- El-Nashar HAS, Eldehna WM, Al-Rashood ST, Alharbi A, Eskandrani RO, Aly SH. GC/MS Analysis of Essential Oil and Enzyme Inhibitory Activities of Syzygium cumini (Pamposia) Grown in Docking Studies. Molecules. 2021;26(22):6984.
- Narkhede MB, Ajimire PV, Wagh AE, Mohan M, Shivashanmugam AT. In vitro antidiabetic activity of Caesalpina digyna (R.) methanol root extract. Asian J Plant Sci Res. 2011;1(2):101–106.
- Zengin G, Uysal A, Gunes E, Aktumsek A. Survey of phytochemical composition and biological effects of three extracts from a wild plant (Cotoneaster nummularia Fisch. et Mey.): a potential source for functional food ingredients and drug formulations. PLoS One. 2014;9(11):e113527.
- Buzzi M, Henderson RJ, Sargent JR. The desaturation and elongation of linolenic acid and eicosapentaenoic acid by hepatocytes and liver microsomes from rainbow trout (Oncorhynchus mykiss) fed diets containing fish oil or olive oil. Biochim Biophys Acta. 1996;1299(2):235–244.
- Brzozowski AM, Davies GJ. Structure of the Aspergillus oryzae alpha-amylase complexed with the inhibitor acarbose at 2.0 A resolution. Biochemistry. 1997;36(36):10837–10845.
- Karade SS, Hill ML, Kiappes JL, Manne R, Aakula B, Zitzmann N, Warfield KL, Treston AM, Mariuzza RA. N-Substituted Valiolamine Derivatives as Potent Inhibitors of Endoplasmic Reticulum α-Glucosidases I and II with Antiviral Activity. J Med Chem. 2021;64(24):18010–18024.
- Cingolani G, Panella A, Perrone MG, Vitale P, Di Mauro G, Fortuna CG, Armen RS, Ferorelli S, Smith WL, Scilimati A. Structural basis for selective inhibition of Cyclooxygenase-1 (COX-1) by diarylisoxazoles mofezolac and 3-(5-chlorofuran-2-yl)-5-methyl-4-phenylisoxazole (P6). Eur J Med Chem. 2017;138:661–668.
- Lai X, Wichers HJ, Soler-Lopez M, Dijkstra BW. Structure of Human Tyrosinase Related Protein 1 Reveals a Binuclear Zinc Active Site Important for Melanogenesis. Angew Chem Int Ed Engl. 2017;56(33):9812–9815.
- Vilar S, Cozza G, Moro S. Medicinal Chemistry and the Molecular Operating Environment (MOE): Application of QSAR and Molecular Docking to Drug Discovery. Curr Top Med Chem. 2008;8(18):1555–1572.
- Hassab ME, Fares M, Amin MKA, Al-Rashood ST, Alharbi A, Eskandrani RO, Alkahtani HM, Eldehna WM. Toward the Identification of Potential α-Ketoamide Covalent Inhibitors for SARS-CoV-2 Main Protease: Fragment-Based Drug Design and MM-PBSA Calculations. Processes. 2021;9(6):1004.
- Amico V, Oriente G, Piattelli M, Ruberto G, Tringali C. Novel acyclic diterpenes from the brown alga Cystoseira crinita. Phytochemistry. 1981;20(5):1085–1088.
- El Amrani Zerrifi S, El Khalloufi F, Mugani R, El Mahdi R, Kasrati A, Soulaimani B, Barros L, Ferreira ICFR, Amaral JS, Finimundy TC, et al. Seaweed essential oils as a new source of bioactive compounds for cyanobacteria growth control: Innovative ecological biocontrol approach. Toxins (Basel). 2020;12(8):527.
- Mokrini R, Mesaoud M, Ben Daoudi M, Hellio C, Maréchal JP, El Hattab M, Ortalo-Magné A, Piovetti L, Culioli G. Meroditerpenoids and derivatives from the brown alga Cystoseira baccata and their antifouling properties. J Nat Prod. 2008;71(11):1806–1811.
- Fernández JJ, Navarro G, Norte M. Novel metabolites from the brown alga Cystoseira abies marina. Nat Prod Lett. 1998;12(4):285–291.
- El Hattab M, Genta-Jouve G, Bouzidi N, Ortalo-Magné A, Hellio C, Maréchal JP, Piovetti L, Thomas OP, Culioli G. Cystophloroketals A-E, Unusual Phloroglucinol-Meroterpenoid Hybrids from the Brown Alga Cystoseira tamariscifolia. J Nat Prod. 2015;78(7):1663–1670.
- Joung EJ, Cao L, Lee B, Gwon WG, Park SH, Kim HR. Sargahydroquinoic acid, a cyclooxygenase-2 inhibitor, attenuates inflammatory responses by regulating NF-κB inactivation and Nrf2 activation in lipopolysaccharide-stimulated cells. Inflammation. 2021;44(5):2120–2131.
- Custódio L, Silvestre L, Rocha MI, Rodrigues MJ, Vizetto-Duarte C, Pereira H, Barreira L, Varela J. Methanol extracts from Cystoseira tamariscifolia and Cystoseira nodicaulis are able to inhibit cholinesterases and protect a human dopaminergic cell line from hydrogen peroxide-induced cytotoxicity. Pharm Biol. 2016;54(9):1687–1696.
- Glombitza K, Schmidt A. Nonhalogenated and halogenated phlorotannins from the brown alga Carpophyllum angustifolium. J Nat Prod. 1999;62(9):1238–1240.
- Vizetto-Duarte C, Pereira H, De Sousa CB, Rauter AP, Albericio F, Custódio L, Barreira L, Varela J. Fatty acid profile of different species of algae of the Cystoseira genus: A nutraceutical perspective. Nat Prod Res. 2015;29(13):1264–1270.
- Aly SH, Kandil NH, Hemdan RM, Kotb SS, Zaki SS, Abdelaziz OM, AbdelRazek MMM, Almahli H, El Hassab MA, Al-Rashood ST, et al. GC/MS profiling of the essential oil and lipophilic extract of Moricandia sinaica Boiss. and evaluation of their cytotoxic and antioxidant activities. Molecules. 2023;28(5):2193.
- Elebeedy D, Ghanem A, Aly SH, Ali MA, Faraag AHI, El-Ashrey MK, Salem AM, Hassab ME, Maksoud AE. Synergistic antiviral activity of Lactobacillus acidophilus and Glycyrrhiza glabra against Herpes Simplex-1 Virus (HSV-1) and Vesicular Stomatitis Virus (VSV): experimental and In Silico insights. BMC Microbiol. 2023;23(1):173.
- Aly SH, El-Hassab MA, Elhady SS, Gad HA. Comparative metabolic study of Tamarindus indica L.’s various organs based on GC/MS analysis, In Silico and In Vitro anti-inflammatory and wound healing activities. Plants (Basel). 2023;12(1):87.
- Yu Q, Fan L, Duan Z. Five individual polyphenols as tyrosinase inhibitors: Inhibitory activity, synergistic effect, action mechanism, and molecular docking. Food Chem. 2019;297:124910.
- Vlachogianni IC, Fragopoulou E, Kostakis IK. 2014. Accepted Manuscript.
- Cos P, Rajan P, Vedernikova I, Calomme M, Pieters L, Vlietinck AJ, Augustyns K, Haemers A, Berghe D. Vanden In Vitro Antioxidant Profile of Phenolic Acid Derivatives. Free Radic Res. 2002;36(6):711–716.
- Fuloria NK, Raheja RK, Shah KH, Oza MJ, Kulkarni YA, Subramaniyan V, Sekar M, Fuloria S. Biological activities of meroterpenoids isolated from different sources. Front Pharmacol. 2022;13:830103.
- Čagalj M, Skroza D, Razola-Díaz MDC, Verardo V, Bassi D, Frleta R, Mekinić IG, Tabanelli G, Šimat V. Variations in the Composition, Antioxidant and Antimicrobial Activities of Cystoseira compressa during Seasonal Growth. Mar Drugs. 2022;20(1):64.
- Benslima A, Sellimi S, Hamdi M, Nasri R, Jridi M, Cot D, Li S, Nasri M, Zouari N. Brown seaweed Cystoseira schiffneri as a promising source of sulfated fucans: Seasonal variability of structural, chemical, and antioxidant properties. Food Sci Nutr. 2021;9(3):1551–1563.
- Saber FR, Aly SH, Khallaf MA, El-Nashar HAS, Fahmy NM, El-Shazly M, Radha R, Prakash S, Kumar M, Taha D, et al. Hyphaene thebaica (Areceaeae) as a Promising Functional Food: Extraction, Analytical Techniques, Bioactivity, Food, and Industrial Applications. Food Anal Methods. 2022;16(9-10):1447–1467.
- Saber FR, Munekata PES, Rizwan K, El-Nashar HAS, Fahmy NM, Aly SH, El-Shazly M, Bouyahya A, Lorenzo JM. Family Myrtaceae: The treasure hidden in the complex/diverse composition. Crit Rev Food Sci Nutr. 2023;7:1–19.
- Aly SH, Eldahshan OA, Al-Rashood ST, Binjubair FA, Hassab ME, Eldehna WM, Acqua SD, Zengin G. Chemical constituents, antioxidant, and enzyme inhibitory activities supported by in-silico study of n-hexane extract and essential oil of Guava leaves. Molecules. 2022;27(24):8979.
- De La Fuente G, Fontana M, Asnaghi V, Chiantore M, Mirata S, Salis A, Damonte G, Scarfì S. The remarkable antioxidant and anti-inflammatory potential of the extracts of the brown alga cystoseira amentacea var. stricta. Mar Drugs. 2021;19(1):2.
- Zbakh H, Zubía E, de los Reyes C, Calderón-Montaño JM, López-Lázaro M, Motilva V. Meroterpenoids from the brown alga cystoseira usneoides as potential anti-inflammatory and lung anticancer agents. Mar Drugs. 2020;18(4):207.
- Sahnoun M, Trabelsi S, Bejar S. Citrus flavonoids collectively dominate the α-amylase and α-glucosidase inhibitions. Biol. 2017;72(7):764–773.
- Enomoto H, Takahashi S, Takeda S, Hatta H. Distribution of flavan-3-ol species in ripe strawberry fruit revealed by matrix-assisted laser desorption/ionization-mass spectrometry imaging. Molecules. 2020;25(1):2373.
- Sun J, Liang F, Bin Y, Li P, Duan C. Screening non-colored phenolics in red wines using liquid chromatography/ultraviolet and mass spectrometry/mass spectrometry libraries. Molecules. 2007;12(3):679–693.
- Tine Y, Renucci F, Costa J, Wélé A, Paolini J. A method for LC-MS/MS profiling of coumarins in zanthoxylum zanthoxyloides (Lam.) B. Zepernich and timler extracts and essential oils. Molecules. 2017;22(1):174.
- Simirgiotis MJ, Benites J, Areche C, Sepúlveda B. Antioxidant capacities and analysis of phenolic compounds in three endemic nolana species by HPLC-PDA-ESI-MS. Molecules. 2015;20(6):11490–11507.
- Ads EN, Rajendrasozhan S, Hassan SI, Sharawy SMS, Humaidi JR. Phytochemical screening of different organic crude extracts from the stem bark of Ziziphus spina-christi (L.). biomedicalresearch. 2018;29(8):1645–1652.
- Barros L, Dueñas M, Dias MI, Sousa MJ, Santos-Buelga C, Ferreira ICFR. Phenolic profiles of cultivated, in vitro cultured and commercial samples of Melissa officinalis L. infusions. Food Chem. 2013;136(1):1–8.
- Farag MA, Gad HA, Heiss AG, Wessjohann LA. Metabolomics driven analysis of six Nigella species seeds via UPLC-qTOF-MS and GC-MS coupled to chemometrics. Food Chem. 2014;151:333–342.
- Li X, Xiao H, Liang X, Shi D, Liu J. LC-MS/MS determination of naringin, hesperidin and neohesperidin in rat serum after orally administrating the decoction of Bulpleurum falcatum L. and Fractus aurantii. J Pharm Biomed Anal. 2004;34(1):159–166.
- Patil SH, Kurlapkar DD, Gaikwad DK. Phytochemical characterization of natural dye extracted from Senna siamea Pods. Open Access Libr J. 2020;07(04):1–11.
- de Souza Mesquita LM, Rodrigues CFB, da Rocha CQ, Bianchim MS, Rodrigues CM, de Oliveira VM, Gaeta HH, Belchor MN, Toyama MH, Vilegas W. LC–ESI–IT-MS/MS and MALDI-TOF Approach: identification of natural polymers from Rhizophora mangle barks and determination of their analgesic and anti-inflammatory properties. Nat Prod Bioprospect. 2019;9(1):23–34.
- Ayoub IM, Korinek M, El-Shazly M, Wetterauer B, El-Beshbishy HA, Hwang T-L, Chen B-H, Chang F-R, Wink M, Singab ANB, et al. Activity of Chasmanthe aethiopica leaf extract and its profiling using LC/MS and GLC/MS. Plants. 2021;10(6):1118.
- Ben SR, Hamed AI, Mahalel UA, Al-Ayed AS, Kowalczyk M, Moldoch J, Oleszek W, Stochmal A. Tentative characterization of polyphenolic compounds in the male flowers of Phoenix dactylifera by liquid chromatography coupled with mass spectrometry and DFT. Int J Mol Sci. 2017;18(3):1–18.
- Chen G, Li X, Saleri F, Guo M. Analysis of flavonoids in Rhamnus davurica and its antiproliferative activities. Molecules. 2016;21(10):1275.
- Salih EYA, Fyhrquist P, Abdalla AMA, Abdelgadir AY, Kanninen M, Sipi M, Luukkanen O, Fahmi MKM, Elamin MH, Ali HA. LC-MS/MS tandem mass spectrometry for analysis of phenolic compounds and pentacyclic triterpenes in antifungal extracts of Terminalia brownii (Fresen). Antibiotics (Basel). 2017;6(4):37.
- Yao H, Chen B, Zhang Y, Ou H, Li Y, Li S, Shi P, Lin X. Analysis of the total biflavonoids extract from Selaginella doederleinii by HPLC-QTOF-MS and its in vitro and in vivo anticancer effects. Molecules. 2017;22(2):325.
- Lou J, Yu R, Wang X, Mao Z, Fu L, Liu Y, Zhou L. Alternariol 9-methyl ether from the endophytic fungus Alternaria sp. Samif01 and its bioactivities. Braz J Microbiol. 2016;47(1):96–101.
- López-Angulo G, Montes-Avila J, Díaz-Camacho SP, Vega-Aviña R, López-Valenzuela JÁ, Delgado-Vargas F. 2018. Comparison of terpene and phenolic profles of three wild species of Echeveria (Crassulaceae). J Appl Bot Food Qual. 91:145–154.