Abstract
Pterostilbene (PST) is a naturally derived stilbene compound in grapes, blueberries, and other fruits. It is also a natural dietary compound with a wide range of biological activities such as antioxidant, anti-inflammatory, antitumor, and so on. Structural modifications based on the chemical scaffold of the pterostilbene skeleton are of great importance for drug discovery. In this study, pterostilbene skeletons were used to design novel anti-inflammatory compounds with high activity and low toxicity. A total of 30 new were found and synthesised, and their anti-inflammatory activity and safety were screened. Among them, compound E2 was the most active (against NO: IC50 = 0.7 μM) than celecoxib. Further studies showed that compound E2 exerted anti-inflammatory activity by blocking LPS-induced NF-κB/MAPK signalling pathway activation. In vivo experiments revealed that compound E2 had a good alleviating effect on acute colitis in mice. In conclusion, compound E2 may be a promising anti-inflammatory lead compound.
Introduction
Inflammation is a complex defense mechanism of organisms. It is an essential and complex physiological process in response to tissue damage and pathogen infection.Citation1,Citation2 It has been found that a moderate inflammatory response can improve the resistance of the organism and is essential for the maintenance of immune balance.Citation3,Citation4 However, excessive or persistent inflammation can have many negative consequences, including tissue damage, organ function loss, organ failure, promote tumour formation and eventual death.Citation5,Citation6 More and more researchers pay attention to inflammatory response due to its complex and diverse pattern and its role in various diseases.Citation7,Citation8 It was confirmed that chronic inflammation shares the same molecular targets and signalling pathways as tumours.Citation9 These targets and signalling were thought to be causative factors in chronic diseases; examples include inflammatory bowel diseases (IBD),Citation10 rheumatoid arthritis (RA),Citation11 asthma,Citation12 types II diabetes,Citation13 cardiovascular diseases (CVD),Citation14 Parkinson’s disease (PD)Citation15 and Alzheimerimer’s disease (AD).Citation16
The innate immune system uses pattern recognition receptors (PRR) to detect self and non-self-pathogens.Citation17,Citation18 Toll-like receptors (TLRs) in the PRR subtype play an essential role in innate immunity by regulating pro-inflammatory cytokine, chemokine, B cell, and T cell activation.Citation19 Thus, inhibitors that target TLRs signalling pathways are considered attractive drug targets for inhibiting inflammatory responses.Citation20 Toll-like receptor 4 (TLR4) can recognise bacterial LPS and transmit a variety of signalling pathways, including NF-κB and MAPK pathways; they produce pro-inflammatory mediators such as Nitric Oxide (NO), prostaglandin E2 (PGE2) and Pro-inflammatory cytokines such as TNF-α, IL-1β, and IL-6.Citation21 Studies have confirmed that inhibition of these pathways can attenuate the inflammatory response in disease;Citation22 some natural products have been reported to exert anti-inflammatory activity by blocking the NF-κB and MAPK signalling pathways.Citation23–25
Although nonsteroidal anti-inflammatory drug aids (NSAIDs) such as celecoxib, and ibuprofen are readily available and effective.Citation26 However, they have significant side effects, especially gastrointestinal injury, which limits their clinical application.Citation27,Citation28 With increasing attention paid to bioactive ingredients in foods, these substances can provide additional health benefits beyond basic nutritional needs.Citation29 Among these identified bioactive components, dietary compounds have been studied the most, and there is increasing evidence of their potential role in the prevention of chronic diseases in humans.Citation30,Citation31 A large amount of clinical data has proven that bioactive ingredients, as part of our diet, have good safety and multiple biological activities, but the movement tends to be weak. Therefore, structural optimisation of dietary compounds, which may have few side effects, has become the focus of contemporary research and is of great significance for discovering anti-inflammatory drugs.
Pterostilbene (trans-3,5-dimethoxy-4-hydroxystilbene) is a natural product with a stilbene skeleton that is widely found in edible blueberries, grapes, and medicinal plants and possesses a wide range of pharmacological activities, including anti-inflammatory, anticancer, antioxidant, and antimicrobial properties, and it is also a common functional dietary compound.Citation32–35 In recent years, there has been increasing evidence of poor oral bioavailability and weak bioactivity of pterostilbene, which limits its clinical use.Citation36 Therefore, optimising pterostilbene is very important for the development of the pharmacological properties of pterostilbene. The current study found that replacing the hydroxyl group of pterostilbene can improve its biological activity (PIF-9; ).Citation37 Therefore, pterostilbene derivatives obtained by structural modification may have enhanced anti-inflammatory effects.
Molecular hybridisation is a common strategy in drug design, and hybrid molecules usually exhibit better activity and lower toxicity.Citation38 The group has been working on the structural optimisation of the pterostilbene skeleton for years.Citation39 To novel pterostilbene derivatives with high efficiency and low toxicity, in this study, two series of 30 compounds with pterostilbene-1H-1, 2, 3 triazole structure were synthesised through a cycle of coordination design-synthetic architectural relationship (SAR)-structure optimisation (). We first introduced the triazole structure into the structure of pterostilbene to synthesise the D1-D17 series of compounds, and the dominant substituent at the R1 position was identified by conformational relationship analysis. A series of compounds E1-E14 were synthesised by introducing the amide structure based on triazole; in vitro activity screening showed that most of the compounds possessed suitable biological activities, among which compound E2 had the most potent inhibitory effect. Further studies showed that compound E2 was able to inhibit the phosphorylation of key proteins in the MAPK and NF-κB signalling pathways. In vivo experiments also showed that compound E2 had a good alleviating effect on DSS-induced acute colitis in mice. In conclusion, we believe this work provides a reference for the development of drugs based on the pterostilbene skeleton.
Materials and methods
General procedures
All reagents and chemicals were obtained commercially (Bide Pharmatech Ltd, China). The solvents were dried and used according to standard procedures, and all chemical reactions were monitored by thin layer chromatography (TLC) and observed under 254 and 365 nm UV irradiation on TLC plates or by potassium permanganate staining. Compounds were purified by column chromatography on silica gel (200 – 300 mesh).1H, 13CNRM resonance spectra were measured on a Bruker AM-300 (1H, 400 MHz; 13C, 100 MHz) or Agilent DD2 600 MHz (1H, 600 MHz; 13C, 151 MHz) with TMS as an internal standard. The solvent was deuterated chloroform (CDCl3 or DMSO-d6), and the chemical shifts were in ppm. In addition, all chemical shifts are given in parts per million relative to tetramethylsilane (TMS). Mass spectra were determined using an LTQ-Orbitrap XL (Thermo Fisher, USA).
Detailed synthesis procedures
Preparation of Intermediate A: Weighed pterostilbene (10 mmol, 2.56 g) in a 50 ml flask, added 20 ml of acetonitrile solvent, to be dissolved, and then added 12 mmol of bromine substituent, anhydrous K2CO3 (10 mmol, 1.38 g), the reaction was carried out under the condition of 60 °C for 16 h, and TLC detected the response until the reaction was complete, and then remove the anhydrous K2CO3. Separated by silica gel column chromatography (PE: EA = 4:1), the desired solution was concentrated to give white solid A, yielding 95.3%.
Preparation of Intermediate B: Weigh intermediate A (5 mmol, 1.35 g) and dissolve it in 20 ml of acetonitrile. Add 0.75 ml of N, N-dimethylformamide, and 3 ml of POCl3 dropwise under ice bath conditions. After the reaction, adjust the reaction liquid to be neutral and slightly alkaline, and stir until the crude product is separated, filter by suction and collect the filter cake, and separate by silica gel column chromatography (DCM: PE = 3:1). The yellow solid collected is B, and the yield is 78.8%.
Preparation of Intermediate C: Intermediate A was synthesised by dissolving astragalus and the compounds replacing the R1 hydroxyl group in acetonitrile, and adding K2CO3. Intermediate A was synthesised by adding POCl3 dropwise with acetonitrile and a small amount of DMF in an ice bath to obtain synthesis B. Then, intermediate B (5 mmol, 1.49 g) was dissolved in 15 ml of CH3NO2, and ammonium acetate (10 mmol, 0.77 g) was added, heated, and refluxed in 100 °C oil bath. Then intermediate B (5 mmol, 1.49 g) was dissolved in 15 ml of CH3NO2, ammonium acetate (10 mmol, 0.77 g) was added, and the reaction was heated to reflux at 100 °C in an oil bath. TLC detected the response until the reaction was complete.
Procedure for the Preparation of Compounds D1 − D17. Intermediate C (2 mmol, 0.68 g) was weighed and dissolved in 20 ml of DMF solvent, NaN3 (3 mmol, 0.195 g) was added slowly under ice bath condition, and p-TsOH (1 mmol, 0.172 g) was added carefully. The reaction was carried out at room temperature and detected by TLC. After the response was complete, it was extracted with water and dichloromethane, the organic phase was collected, an appropriate amount of anhydrous sodium sulphate was added to remove moisture, and the crude product was concentrated, separated by silica gel column chromatography (petroleum ether: ethyl acetate = 4:1), and recrystallised with ethyl acetate to obtain the target product D1-D17 as a white solid.
Procedure for the Preparation of Compounds E1−E14. Weigh 1 mmol of intermediates D1-D14, dichloromethane was used as a solvent, and after the white solid was dissolved entirely, 1.5 ml of triethylamine was added slowly dropwise. Then ethyl isocyanate (1.1 mmol, 87 μL) was added and detected by TLC. The organic phase was extracted, collected, dried, and concentrated to obtain the crude product and separated by thin-layer chromatography to get the target products E1-E14, respectively.
X-ray diffraction
Crystallographic data for compound D11 were collected on a Bruker Smart 1000 CCD area detector diffractometer. Equipped with Mo Кα (0.71073 Å) radiation using u-scan mode. An empirical absorption correction was applied to the data. The structure was solved by direct method and refined by full-matrix least-squares on F2. All non-hydrogen atoms were derived from the experimental setup and then anisotropically refined. All hydrogen atoms were created at idealised positions and were assigned fixed isotropic thermal parameters that were 1.2 times the equivalent isotropic U of the atoms to which they were attached and allowed to ride on their respective parent atoms. The contribution of these hydrogen atoms is included in the structure factor calculations.
Cell culture
RAW264.7 cell line was purchased from BeNa Culture Collection (China), were cultured in high-glucose Dulbecco’s Modified Eagle Medium (DMEM, Hyclone, USA) with 5% foetal bovine serum (Wisent, Canada), and 100 U/mL penicillin and 100 μg/mL streptomycin (Beyotime, China) at 37 °C in a humidified environment with 5% CO2.
MTT assay
RAW264.7 cells (1 × 104 cells/well) were seeded into a 96-well plate and cultured overnight. Then the compound (20 µM) was added and co-cultured for 24 h. Next, add 20 μl of MTT solution (concentration of 5 mg/mL) and incubate for 4 h. Finally, the medium was removed, DMSO (150 μL) was added to each well, and absorbance at 492 nm was read in a microplate reader.
Assay for NO production
RAW264.7 cells (7 × 104 cells/well) were seeded into a 48-well plate and cultured overnight. RAW264.7 cells were pre-treated with different concentrations of compounds for 1 h and incubated with LPS (055:B5, Sigma, USA) (0.5 µg/mL) for 24 h. The supernatant was collected and assayed for NO production using Griess reagent (Beyotime, China).
Western blot
The RAW264.7 cells (2 × 106 cells) were seeded in 6-well plates and cultured overnight. RAW264.7 cells were pre-treated with compound E2 (2, 1, 0.5 µM) for 1 h, then treated with LPS (0.5 µg/mL) for 24 h. Cells were lysed in 300–400 μL of RIPA (Beyotime China). Different concentrations of SDS-PAGE were selected for electrophoresis and then transferred to PVDF membranes (Millipore Immobilon-P, USA). The primary antibodies anti-IκB antibody (2859s), anti-Phospho IκB antibody (2859s), anti-NF-κB antibody (13346s), anti-Phospho NF-κB antibody (8242s) were purchased from Cell Signalling (USA). Antibodies were diluted with antibody dilution solution (Beyotime, China) at a ratio of 1:1000. Anti-ERK antibody (YT1625), anti-Phospho ERK antibody (YP0101), anti-p38 antibody (YT3513), anti-Phospho p38 antibody (YP0338), anti-JNK antibody (YT2440) anti-Phospho JNK antibody (YP0157) were purchased from Immunoway (USA). Antibodies were diluted with antibody dilution solution (Beyotime, China) at a ratio of 1:1000. Anti-β-actin antibody (1:1000, 250136) was purchased from Zen-bioscience (China). Blots with antibodies were incubated overnight at 4 °C followed by HRP-conjugated secondary antibodies for 1 h. The bands were detected with an enhanced chemiluminescence reagent western blotting detection system (Tanon, China). The experiment was repeated three times, and the gray value of the protein was calculated each time using Image-J software, and the protein expression was expressed as mean ± SD.
DSS-induced colitis model and treatment
Male C57BL/6 mice, 16–18 g, purchased from Skbex Biology (China). Mice were randomly divided into the following five groups (10 per group): Normal group, DSS treatment group, positive drug (Sulfasalazine 200 mg/kg) group, and compound E2 (10 mg/kg and 20 mg/kg) treatment group. Acute colitis was induced in C57BL/6 by administration of 5% (wt/vol) DSS in drinking water (days 1–7). Compound E2 (10 mg/kg and 20 mg/kg) and Sulfasalazine were dissolved in an aqueous solution containing 0.5% sodium carboxymethylcellulose (CMC-Na) and administered orally from day 1 to day 7. The normal group and DSS-treated group were also given the same volume of CMC-Na by gavage. The mice were anaesthetised and sacrificed on the eighth day, and the mouse colons were dissected for subsequent pathological analysis.
ELISA assay
Blood samples were centrifuged to collect serum and cytokines levels were assayed using ELISA kits (TNF-α, IL-1β, and IL-6, Elabscience, China) according to the manufacturer’s instructions.
Real-time PCR
Total RNA was isolated from tissues using TransZol Up reagent (BOSTER Biological Technology Co. Ltd, China). Using the HiScript Q RT SuperMix kit (BOSTER Biological Technology Co. Ltd, China), high-quality RNA (approximately 1 μg) Reverse transcription into cDNA. TNF-α, IL-1β, IL-6, COX-2, iNOS, and TLR4 mRNA expressions were determined. β-actin was introduced as the normalisation for RT-qPCR. The primer sequences are listed in Table S1.
Statistic analysis
Statistical analysis was performed in GraphPad Prism using one-way ANOVA. Data were expressed as mean ± standard deviation (SD) and were considered statistically significant when p < 0.05.
Results and discussion
Chemistry
The synthetic pathway employed to prepare the target compounds was depicted in Scheme 1. Then, the reaction of p-toluenesulfonic acid (p-TsOH) mediated 1,3-dipolar cycloaddition of nitroolefins (C) with NaN3 was applied for the synthesis of the intermediates pterostilbene-NH-1,2,3-triazoles (D1-D17) in good yield. The reaction of selected NH-1,2,3-triazole intermediates with commercially available isocyanates gave the target compounds E1-E14. All compounds were purified by recrystallisation or column chromatography with good yields. The structures of all the target compounds were elucidated by detailed 1H NMR, 13C NMR, HRMS (ESI) spectra.
Crystal structure of compound D11
Compound D11 was further determined by the X-ray crystallography, see . Compound D11: (C25H23N3O3)2⋅H2O, triclinic, space group: p-1; a = 7.6094(7), b = 12.0172(13), c = 23.743(2) (Å); α = 95.297(8), β = 93.309(8), γ = 93.745(8) (°), V = 2152.8(4) nm3, T = 293.0(5) K, Z = 2, Dc = 1.303 g/cm3, F(000) = 892, Reflections collected = 13183, Indep. reflns = 13183, Refns obs. [I > 2σ(I)] = 8282, Goodness-of-fit on F2 = 1.067, Parameters = 584, R1, wR2 (all data) = 0.1344/0.3036, R1, wR2 [I > 2σ (I)] = 0.0947/0.2639, Large peak/hole (e. Å) = 0.409/-0.38, CCDC. No: 2288371.
Toxicity of compounds to RAW264.7 cells
First, we measured the cytotoxicity of all synthesised compounds in RAW 264.7 cells by MTT assay to ensure their safety and avoid giving false-positive results for subsequent experiments. The results showed that all synthesised compounds exhibited good protection at concentrations of 20 μM compared to the control (). Therefore, we selected the compounds for further evaluation of their anti-inflammatory activities.
Inhibitory effect on LPS-induced NO production in RAW264.7 cells
When the body undergoes an immune response, macrophages release many inflammatory factors, of which NO production is closely associated with various inflammatory diseases.Citation40 Therefore, we used the Griess method to detect the effect of the compounds on NO production in LPS-treated RAW 264.7 cells. In vitro activity screening of the synthesised compounds (D1-D17) showed that most compounds exhibited some inhibitory effect on NO release (). The activity results showed that compounds containing alkyne substitutions at R1 (D4 and D5) showed good inhibition of NO. when R1 substitutions were alkane (D1-D3), ether bonds (D6-D7), carbamates (D8-D9) and Aromatic ring (D11-D17)were unfavourable. The above results suggest that the compounds containing the structure of pterostilbene-1H-1, 2, 3-triazole have some anti-inflammatory activity and do not exhibit cytotoxicity; The SAR analysis shows that when the R1 substituent contains alkynes, the anti-inflammatory effect is most prominent, so the alkyne substitution at the fixed R1 position can further optimise the structure.
Figure 4. Inhibitory effects of the synthetic compounds (D1-D17) on LPS-induced NO production. ***P < 0.001 Compared with LPS treated group. Cel: celecoxib; as a positive control.
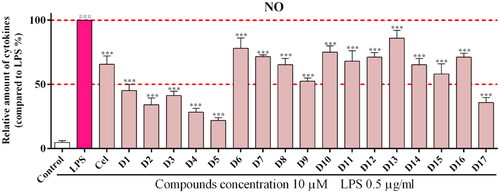
Amides are widely used in drug design due to their easy metabolism and hydrolysis in the body by enzymes in the kidneys, liver, and other organs. To further improve the activity and physicochemical properties of the compounds, we introduced amide structures based on D1-D17 series compounds. Other groups were introduced at R2 to design and synthesise the E1-E13 series compounds. The structures and activities of the designed compounds are shown in . The activity of the compounds changes when different alkyl groups are utilised in place of R2, with propyl substitution being the most active (E2). Extending the length (E4) or increasing the area (E3, E5) of substituent groups reduces the compounds’ activity. Fixing the substitution of R2 and replacing the alkynyl group of R1 (E11-E13) decreased the compound activity, again showing the importance of the alkynyl group for increasing the compound activity. The experimental results showed that after two rounds of structural optimisation, most compounds exhibited high biological activity, with compound E2 having the most prominent activity. Therefore, compound E2 was selected for subsequent research.
Table 1. Compounds E1-E13 inhibitory activity against NO release.
Effect of compound E2 on the expression of inflammatory proteins
To further investigate the anti-inflammatory mechanism of E2, we examined the effect of E2 on the expression of relevant inflammatory proteins. iNOS is an inducible enzyme that mediates NO production during inflammatory progression.Citation41 Analogously, cyclooxygenase 2 (COX-2) is the key enzyme for PGE2 biosynthesis.Citation42 Inhibition of COX-2 protein is mainly used to alleviate pain symptoms, infection, fever, etc. Expression of IL-6 and TNF-α protein is associated with various inflammatory diseases, and inhibition of the secretion of related proteins and factors is currently used to treat colitis, rheumatoid arthritis, and inflammatory gastrointestinal tract diseases. The experiment results revealed that inflammatory protein expression increased substantially in the LPS-induced group compared to the blank group. The expression of iNOS, COX2, IL-6, and TNF-α protein was significantly inhibited in cells treated with different concentrations of compound E2 (). These results suggest that E2 can mitigate the inflammatory effects by decreasing the iNOS, COX2, IL-6, and TNF-α protein expression.
Effect of compound E2 on NF-κB inflammatory signalling pathway
Nuclear factor-κB is critical in various cellular activities, including inflammatory responses, viral replication, apoptosis, and proliferation.Citation43 Studies have shown that NF-κB effectively induces gene expression of cytokines, adhesion molecules, chemokine, and acute-phase response proteins; it also has a vital role in regulating the gene expression of various enzymes involved in the amplification and persistence of inflammatory responses. In addition, the occurrence and development of autoimmune diseases, chronic inflammation-related diseases, certain neurological diseases, allergic reactions, and the body’s rejection of grafts are closely related to the over-activation of NF-κB. Therefore, inhibiting the activation of the NF-κB signalling pathway is vital for treating diseases. With the degradation of IκB, NF-κB translocates to the nucleus and activates target gene transcription. We used western blot to determine the effects of compound E2 on the phosphorylation of P65 and IκB proteins at different concentrations and to analyse the mechanism of its anti-inflammatory effects. The results showed that compound E2 inhibited the phosphorylation of IκB and p65 proteins at different concentrations, inhibiting the activation of the NF-κB pathway (). These results suggest that compound E2 exerts anti-inflammatory effects by inhibiting the abnormal activation of the NF-κB signalling pathway.
Figure 6. Inhibition of NF-κB inflammatory signalling pathway by compound 8. RAW264.7 was pre-treatment with compound 8 (2 µM, 1 µM, 0.5 µM) for 1 h, and then exposed to LPS (500 ng/mL) for 30 min. Bay11-7082 (1 µM) is an NF-κB signalling pathway inhibitor. *** P < 0.001 compared with LPS treated group.
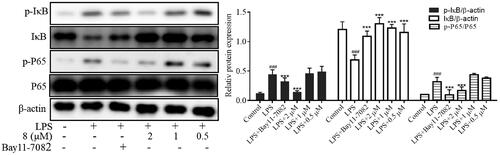
Effect of compound E2 on the inflammatory signalling pathway of MAPKs
Mitogen-activated protein kinases (MAPKs) are critical signalling systems and major pathways that mediate cellular responses, such as the regulation of apoptosis, growth, maturation, proliferation, differentiation, and other processes, and play a crucial role in the maintenance of normal physiological processes in the body.Citation44 In addition, increasing evidence suggests that phosphorylation of MAPK family proteins (including ERK, p38, and JNK) triggers the regulation of the NF-κB signalling pathway during inflammation. The activated MAPK can enter the nucleus and induce up-regulation of transcription levels of various inflammatory mediators. Therefore, the MAPK signalling pathway was considered a potential target for anti-inflammatory therapy. Here, LPS-stimulated cells exhibited significantly increased levels of JNK, p38, and ERK phosphoproteins, which upregulated the production of TNF-α, IL-6, iNOS, and COX2. Therefore, the levels of phosphorylated ERK, p38, and JNK were investigated to explore the anti-inflammatory mechanism of E2 further. We also found that compound E2 inhibits the expression of p-p38, p-ERK, and p-JNK in a dose-dependent manner (). In summary, compound E2 exerts anti-inflammatory effects by blocking the activation of NF-kB and MAPK signalling pathways.
In vivo efficacy of compound E2
Inflammatory bowel disease (IBD) is an idiopathic inflammatory disease of the intestine characterised by autoimmune dysfunction, including ulcerative colitis (UC) and Crohn’s disease (CD). IBD's pathogenesis is complex and associated with genetic susceptibility and microbial ecological dysregulation.Citation45 Although its aetiology is unknown, studies have confirmed that the abnormally activated NF-κB and MAPK pathways promote intestinal barrier disruption and persistent inflammation.Citation46 Finding effective therapeutic agents is currently a hot issue in clinical research. Therefore, we chose the DSS-induced acute colitis model in mice to test the bioactivity of compound E2 in vivo. The experiment was divided into a normal group, model group (DSS), high-dose group (DSS + 20 mg/kg), low-dose group (DSS + 10 mg/kg), and sulfasalazine (DSS + 200 mg/kg). During the 7-day experiment, the control group mice drank normal water, while the other experimental group mice drank water solutions containing 5% DSS. The mice’s body weight, diarrhoea, bloody stools, and disease indices were recorded daily throughout the experiment. From day 4–7, most model group mice began to develop significant pasty faeces to liquid blood stools, accompanied by weight loss and increased disease index. Compared with the model mice, the treatment and sulfasalazine groups alleviated the weight loss and increased the disease index (). At the end of the experiment, blood was taken from the eyes of the mice, and serum was collected for subsequent testing. Mice were executed and dissected, and compound E2 significantly attenuated DSS treatment-induced shortening of colon length (). Histopathological analysis showed that the model group had an incomplete colonic mucosa with massive infiltration of neutrophils, reduced cupping, loss of crypt foci, and muscular edoema with partial neutrophil infiltration (). The number of colonic cups and crypt foci was significantly greater in the treatment group than in the model group. In the acute colitis model, compound E2 significantly improved these symptoms. These results suggest compound E2 has a better therapeutic effect on DSS-induced acute colitis in mice.
Figure 8. Compound E2 alleviates DSS-induced colitis in mice. (a) Mice show bloody stools (b) Weight change; (c) DAI was calculated; (d, e) Measuring the length of the colon; (f) Histopathological analysis of mouse colon. Data are represented as mean and SEM. Sulfasalazine (SASP) was used as a positive control drug. Compared with the Normal group, ### P < 0.001; Compared with DSS treated group, *** P < 0.001.
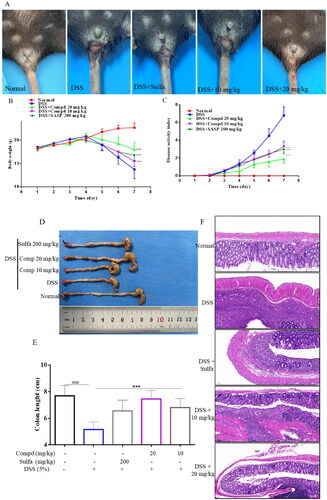
Myeloperoxidase (MPO) is both a mediator of oxidative stress and a marker of systemic inflammation that promotes the formation of oxidising substances and the production of free radicals and a variety of reactive substances.Citation47 By day 7, E2 at 20 mg/kg and sulfasalazine significantly reduced MPO, respectively (). To evaluate the anti-inflammatory effect of E2, the level of cytokines TNF-α, IL-1β, and IL-6 in the serum of mice was tested. It can be observed that DSS notably increased the levels of TNF-α, IL-1β, and IL-6 in serum, whereas administration of E2 significantly decreased the levels of these pro-inflammatory cytokines (). These results suggest that compound E2 inhibits DSS-induced systemic inflammation in mice.
Figure 9. Compound E2 inhibits pro-inflammatory factor expression and MPO levels. (a) Detection of MPO activity in colon tissue. (b − d) ELISA determined the cytokine levels of TNF-α, IL-1β, and IL-6. Values are expressed as a mean ± SEM. ### P < 0.001 compared with the Normal group; *** P < 0.001 Compared with DSS treated group.
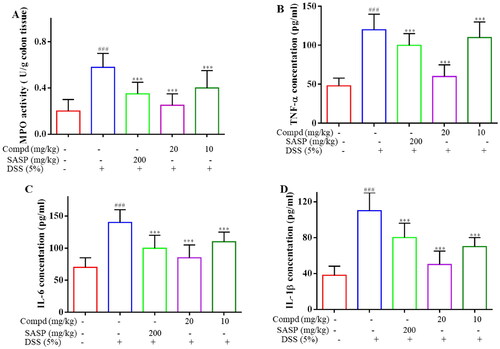
Furthermore, the intestinal tight junction-related mRNA expression results are shown in . Data showed that DSS significantly decreased the mRNA expression levels of TNF-α, IL-1β, IL-6, iNOS, COX-2, and TLR4 in the colon tissue, and these changes were alleviated by compound E2 treatment. To further explore the mechanism of compound E2, the COX-2, p-NF-κB, and p-Stat3 signalling pathway-related proteins were evaluated by Western blot. As shown in , DSS significantly increased the levels of COX-2, p-NF-κB, and p-Stat3 expression, while these trends were decreased by compound E2 treatment. Summarising the above experimental results, we believe that compound E2 can inhibit the transcription of inflammatory proteins and the activation of inflammatory pathways in DSS-induced mouse colon tissues, thereby exerting a better therapeutic effect on acute colitis.
Figure 10. Compound E2 treatment downregulated the Inflammatory proteins and signalling pathway in acute DSS-induced colitis. (A-F) TNF-α, IL-1β, IL-6, iNOS, COX-2 and TLR4 mRNA levels in colon tissues. (G-J) Representative Western blot images of colonic COX-2, p-NF-κB, p-Stat3, and β-actin proteins. Compared with Normal group, ### P < 0.001; Compared with DSS treated group, *** P < 0.001.
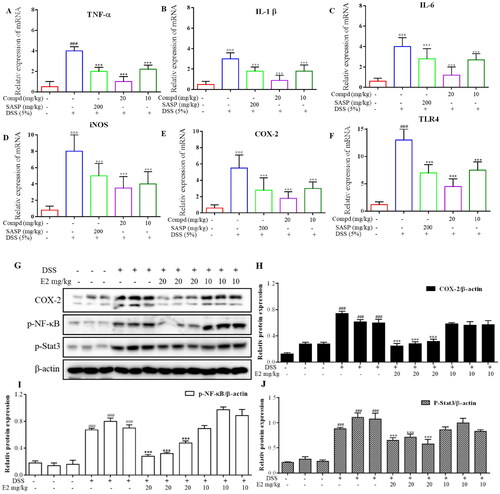
In summary, we synthesised 30 new pterostilbene derivatives based on the combination principles. The structures were confirmed by 1H NMR and 13C NMR, HR-MS, and some compounds were conformationally confirmed by X-ray single-crystal diffraction technique. Next, we determined their anti-inflammatory activities in LPS-treated RAW 264.7 cells in vitro. The results showed that the two series of compounds exhibited good safety, and most of the compounds had good anti-inflammatory activity. In the D1-D17 series, structure-activity relationship studies have found that compounds D4 and D5 containing unsaturated double alkyne substitutions at R1 have more prominent inhibitory effects on NO than other compounds, with D4 (IC50= 8.07 ± 2.08 μM) and D5 (IC50= 5.43 ± 0.96 μM). Further structural optimisation was carried out by fixing the best substituent at R1 and introducing a different substituted amide structure at R2, and 13 compounds were designed and synthesised again. Most of the compounds in the new series showed better inhibition of NO release than those in the D1-D17 series, with compound E2 (IC50= 0.7 ± 0.15 μM) exhibiting the most significant inhibition. Mechanistic studies showed that compound E2 exerted anti-inflammatory effects by inhibiting NF-κB/MAPK signalling pathway activation. In vivo experiments showed that this compound E2 had a better safety profile and better therapeutic effects on DSS-induced acute colitis in mice. In conclusion, compound E2 is a structural modification of the natural product pterostilbene, which could serve as a promising anti-inflammatory lead compound and deserves further investigation.
Supplemental Material
Download PDF (2.6 MB)Disclosure statement
The authors confirm that this article’s content has no conflict of interest.
Additional information
Funding
References
- Watson N, Ding B, Zhu X, Frisina RD. Chronic inflammation – inflammaging – in the ageing cochlea: a novel target for future presbycusis therapy. Ageing Res Rev. 2017;40:1–13.
- Medzhitov R. Origin and physiological roles of inflammation. Nature. 2008;454(7203):428–435.
- Geiger SS, Essers MAG. Inflammation’s epigenetic footprint in hematopoietic stem cells. Cell Stem Cell. 2020;26(5):611–612.
- Cain D, Kondo M, Chen H, Kelsoe G. Effects of acute and chronic inflammation on B-cell development and differentiation. J Invest Dermatol. 2009;129(2):266–277.
- Fullerton JN, O'Brien AJ, Gilroy DW. Lipid mediators in immune dysfunction after severe inflammation. Trends Immunol. 2014;35(1):12–21.
- Han J, Ulevitch RJ. Limiting inflammatory responses during activation of innate immunity. Nat Immunol. 2005;6(12):1198–1205.
- Clark JD, Flanagan ME, Telliez JB. Discovery and development of Janus kinase (JAK) inhibitors for inflammatory diseases. J Med Chem. 2014;57(12):5023–5038.
- Willems S, Zaienne D, Merk D. Targeting nuclear receptors in neurodegeneration and neuroinflammation. J Med Chem. 2021;64(14):9592–9638.
- Salkeni MA, Naing A. Interleukin-10 in cancer immunotherapy: from bench to bedside. Trends Cancer. 2023;9(9):716–725.
- Lee SH, Kwon JE, Cho ML. Immunological pathogenesis of inflammatory bowel disease. Intest Res. 2018;16(1):26–42.
- Schneider K, Arandjelovic S. Apoptotic cell clearance components in inflammatory arthritis. Immunol Rev. 2023;28:126–135.
- Ding Z, Mulder J, Robinson MJ. The origins and longevity of IgE responses as indicated by serological and cellular studies in mice and humans. Allergy. 2023;78(12):3103–3117.
- Berbudi A, Rahmadika N, Tjahjadi AI, Ruslami R. Type 2 diabetes and its impact on the immune system. Curr Diabetes Rev. 2020;16:442–449.
- Liberale L, Badimon L, Montecucco F, Lüscher TF, Libby P, Camici GG. Inflammation, aging, and cardiovascular disease: JACC review topic of the week. J Am Coll Cardiol. 2022;79(8):837–847.
- Tansey MG, Wallings RL, Houser MC, Herrick MK, Keating CE, Joers V. Inflammation and immune dysfunction in Parkinson disease. Nat Rev Immunol. 2022;22(11):657–673.
- Calsolaro V, Edison P. Neuroinflammation in Alzheimer’s disease: current evidence and future directions. Alzheimers Dement. 2016;12(6):719–732.
- Couto D, Zipfel C. Regulation of pattern recognition receptor signalling in plants. Nat Rev Immunol. 2016;16(9):537–552.
- Brubaker SW, Bonham KS, Zanoni I, Kagan JC. Innate immune pattern recognition: a cell biological perspective. Annu Rev Immunol. 2015;33(1):257–290.
- Aghamiri SH, Komlakh K, Ghaffari M. Toll-like receptors (TLRs) and their potential therapeutic applications in diabetic neuropathy. Int Immunopharmacol. 2022;102:1083–1098.
- Wang J, Zhang J, Wang J, Hu X, Ouyang L, Wang Y. Small-molecule modulators targeting toll-like receptors for potential anticancer therapeutics. J Med Chem. 2023;66(10):6437–6462.
- Chen LZ, Sun WW, Bo L, Wang JQ, Xiu C, Tang WJ, Shi JB, Zhou HP, Liu XH. New arylpyrazoline-coumarins: synthesis and anti-inflammatory activity. Eur J Med Chem. 2017;138:170–181.
- Zhang Y, Liang X, Bao X, Xiao W, Chen G. Toll-like receptor 4 (TLR4) inhibitors: current research and prospective. Eur J Med Chem. 2022;235:114291–114301.
- Li R, Zhou Y, Zhang S, Li J, Zheng Y, Fan X. The natural (poly)phenols as modulators of microglia polarization via TLR4/NF-kappaB pathway exert anti-inflammatory activity in ischemic stroke. Eur J Pharmacol. 2022;914:174660–174668.
- Bian M, Gong G, Lei P, Du H, Bai C, Wei C, Quan Z, Ma Q. Design, synthesis, and in vitro and in vivo biological evaluation of limonin derivatives for anti-inflammation therapy. J Agric Food Chem. 2021;69(45):13487–13499.
- Deng R, Wang F, Wang L, Xiong L, Shen X, Song H. Advances in plant polysaccharides as antiaging agents: effects and signaling mechanisms. J Agric Food Chem. 2023;71(19):7175–7191.
- Ramos-Inza S, Ruberte AC, Sanmartín C, Sharma AK, Plano D. NSAIDs: old acquaintance in the pipeline for cancer treatment and prevention horizontal line structural modulation, mechanisms of action, and bright future. J Med Chem. 2021;64(22):16380–16421.
- Bindu S, Mazumder S, Bandyopadhyay U. Nonsteroidal anti-inflammatory drugs (NSAIDs) and organ damage: a current perspective. Biochem Pharmacol. 2020;180:114147–114155.
- Schjerning AM, McGettigan P, Gislason G. Cardiovascular effects and safety of (non-aspirin) NSAIDs. Nat Rev Cardiol. 2020;17(9):574–584.
- Borchers A, Pieler T. Programming pluripotent precursor cells derived from Xenopus embryos to generate specific tissues and organs. Genes (Basel)). 2010;1(3):413–426.
- Wu Q, Gao ZJ, Yu X, Wang P. Dietary regulation in health and disease. Signal Transduct Target Ther. 2022;7:240–252.
- Zampelas A, Magriplis E. Dietary patterns and risk of cardiovascular diseases: a review of the evidence. Proc Nutr Soc. 2020;79(1):68–75.
- Kim H, Seo KH, Yokoyama W. Chemistry of pterostilbene and its metabolic effects. J Agric Food Chem. 2020;68(46):12836–12841.
- McCormack D, McFadden D. A review of pterostilbene antioxidant activity and disease modification. Oxid Med Cell Longev. 2013;2013:575482–15.
- Koh YC, Ho CT, Pan MH. Recent advances in health benefits of stilbenoids. J Agric Food Chem. 2021;69(35):10036–10057.
- Wu WY, Ding XQ, Gu TT, Guo WJ, Jiao RQ, Song L, Sun Y, Pan Y, Kong LD. Correction to pterostilbene improves hepatic lipid accumulation via the MiR-34a/Sirt1/SREBP-1 pathway in fructose-fed rats. J Agric Food Chem. 2021;69(35):10405–10405.
- Lin WS, Leland JV, Ho CT, Pan MH. Occurrence, bioavailability, anti-inflammatory, and anticancer effects of pterostilbene. J Agric Food Chem. 2020;68(46):12788–12799.
- Fang M, Zou T, Yang X, Zhang Z, Cao P, Han J, Duan Y, Ruan BF, Li QS. Discovery of novel pterostilbene derivatives that might treat sepsis by attenuating oxidative stress and inflammation through modulation of MAPKs/NF-kappaB signaling pathways. Antioxidants. 2021;10(9):1333–1350.
- Wang C, Li J, Qu L, Tang X, Song X, Yang F, Chen X, Lin Q, Lin W, Zhou Y, et al. Discovery of D6808, a highly selective and potent macrocyclic c-met inhibitor for gastric cancer harboring MET gene alteration treatment. J Med Chem. 2022;65(22):15140–15164.
- Ruan B, Rong M, Ming Z, Wang K, Liu X, Deng L, Zhang X, Xu K, Shi C, Gao T, et al. Discovery of pterostilbene analogs as novel NLRP3 inflammasome inhibitors for potential treatment of DSS-induced colitis in mice. Bioorg Chem. 2023;133:106429–106434.
- Chen LZ, Shu HY, Wu J, Yu YL, Ma D, Huang X, Liu MM, Liu XH, Shi JB. Discovery and development of novel pyrimidine and pyrazolo/thieno-fused pyrimidine derivatives as potent and orally active inducible nitric oxide synthase dimerization inhibitor with efficacy for arthritis. Eur J Med Chem. 2021;213:113174–113185.
- Shi JB, Chen LZ, Wang BS, Huang X, Jiao MM, Liu MM, Tang WJ, Liu XH. Novel pyrazolo[4,3-d]pyrimidine as potent and orally active inducible nitric oxide synthase (iNOS) dimerization inhibitor with efficacy in rheumatoid arthritis mouse model. J Med Chem. 2019;62(8):4013–4031.
- Chen LZ, Wu J, Li K, Wu QQ, Chen R, Liu XH, Ruan BF. Novel phthalide derivatives: synthesis and anti-inflammatory activity in vitro and in vivo. Eur J Med Chem. 2020;206:112722–112731.
- Yuan Y, Lu L, Bo N, Chaoyue Y, Haiyang Y. Allicin ameliorates intestinal barrier damage via microbiota-regulated short-chain fatty acids-TLR4/MyD88/NF-kappaB cascade response in acrylamide-induced rats. J Agric Food Chem. 2021;69(43):12837–12852.
- Kou RW, Gao YQ, Xia B, Wang JY, Liu XN, Tang JJ, Yin X, Gao JM. Ganoderterpene A, a new triterpenoid from ganoderma lucidum, attenuates LPS-Induced inflammation and apoptosis via suppressing MAPK and TLR-4/NF-kappaB pathways in BV-2 cells. J Agric Food Chem. 2021;69(43):12730–12740.
- Zhang L, Yao X, Ma M, Ding Y, Zhang H, He X, Song Z. Protective effect of l-theanine against DSS-induced colitis by regulating the lipid metabolism and reducing inflammation via the NF-kappaB signaling pathway. J Agric Food Chem. 2021;69(47):14192–14203.
- Xia X, Lin H, Luo F, Wu X, Zhu L, Chen S, Luo H, Ye F, Peng X, Zhang Y, et al. Oryzanol ameliorates DSS-stimulated gut barrier damage via targeting the gut microbiota accompanied by the TLR4/NF-kappaB/NLRP3 cascade response in vivo. J Agric Food Chem. 2022;70(50):15747–15762.
- Frankish N, Sheridan H. 6-(methylamino)hexane-1,2,3,4,5-pentanol 4-(((1S,2S)-1-hydroxy-2,3-dihydro-1H,1'H-[2,2-biinden]-2-yl)methyl)benzoate (PH46A): a novel small molecule with efficacy in murine models of colitis. J Med Chem. 2012;55(11):5497–5505.