ABSTRACT
Introduction: The successful development of two broadly protective vaccines targeting Neisseria meningitidis serogroup B (MenB); 4CMenB and rLP2086, is the most significant recent advance in meningococcal disease prevention.
Areas covered: Here we review the principles underlying the development of each vaccine and the novel methods used to estimate vaccine coverage. We update clinical and post-licensure experience with 4CMenB and rLP2086.
Expert commentary: The immunogenicity and acceptable safety profile of 4CMenB and rLP2086 has been demonstrated in clinical trials. Continuing uncertainties exist around the appropriate age groups to be immunized, the degree and duration of efficacy, and the impact on nasopharyngeal carriage which has implications for strategies to interrupt transmission and maximize herd protection effects. Universal vaccination programs such as those undertaken in Quebec and the United Kingdom are providing important information on these issues. The potential for MenB vaccines to prevent infection by other serogroups appears promising, and the impact of MenB vaccines on other pathogenic neisserial species with similar surface proteins warrants further investigation.
1. Introduction
The most significant recent advance in meningococcal disease prevention has been the successful development of two broadly protective protein-based vaccines targeting Neisseria meningitidis serogroup B (MenB). The 4-component MenB protein vaccine (4CMenB; Bexsero, GSK, Siena, Italy) is licensed in the United States (US) as a two-dose schedule in 10–25 year olds, and for use in all ages up to 50 years, starting from 2 months of age in Europe, Australia, Canada, and some countries in South America. The second vaccine, bivalent rLP2086 (Trumenba, Pfizer, Philadelphia, PA, USA) is licensed in the US as a two- or three-dose schedule in 10–25 year olds.
Neisseria meningitidis is an encapsulated gram-negative diplococcus and is an important cause of septicemia and meningitis in all age groups, with the highest disease incidence in infants <1 year of age [Citation1]. Humans are the only host and the meningococcus is a common commensal in the nasopharynx, transmitted from person-to-person via oral contact or respiratory secretions. Carriage prevalence varies with age, peaking (23.7%) in adolescents and young adults in industrialized countries, but may be substantially higher (up to 71%) in closed communities including residential colleges and military barracks [Citation2,Citation3], Rarely, the bacterium invades the mucosa and enters the blood stream, causing invasive meningococcal disease (IMD) and meningitis when meningococci traverse the blood–brain barrier. IMD epidemics occur when a population with low underlying immunity is exposed to a virulent strain to which it has not been exposed previously [Citation4]. Mortality due to IMD can reach 10–20% despite antibiotics and intensive supportive care, and long-term disability in survivors is not uncommon [Citation5].
The polysaccharide capsule of the meningococcus is a major virulence factor and differences in the chemical composition of the polysaccharide allow the differentiation of 12 serogroups. Six serogroups (A, B, C, W, Y, and X) cause the majority of endemic and epidemic IMD [Citation6], but the distribution of these serogroups varies geographically, temporally, and is affected by vaccination programs [Citation7]. Serogroup A caused the majority of epidemic IMD in sub-Saharan Africa until the recent introduction of MenA-conjugate vaccines. Serogroup Y contributes to IMD in the US and Scandinavia [Citation1,Citation8,Citation9]. Serogroup X causes outbreaks of IMD in the African meningitis belt [Citation10]. Serogroup W (MenW) causes relatively few cases of IMD in the US and Europe, with the exception of England, Wales, Argentina and Chile where a steep rise in MenW disease due to the ST11 clonal complex occurred after 2010, such that MenW now causes approximately 25–50% of IMD in those countries [Citation8,Citation11,Citation12].
During the late twentieth century, meningococcus serogroup C (MenC) was one of the most frequent causes of IMD in children and adolescents in industrialized countries, accounting for a substantial proportion of endemic disease and causing periodic outbreaks [Citation6]. Countries with established MenC vaccination programs, such as the United Kingdom (UK), Australia, Canada, and several regions in Europe, observed marked decreases in IMD due to MenC after vaccine introduction; in these countries MenB now causes the majority of IMD [Citation8,Citation13–Citation16]. MenB accounted for approximately 68% of cases in Europe (2012), 80% of cases in Australia (2014) and 28% of IMD cases in the US (2014, Citation8,Citation13,Citation17]. Incidence data available from the US and Europe show that the highest incidence of MenB IMD occurs in children aged <1 year [Citation8,Citation17].
Currently available meningococcal conjugate vaccines contain polysaccharides from one (C or A), two (C and Y) or four (A, C, W, and Y) serogroups, which are chemically conjugated to protein carrier molecules. The development of a broadly protective polysaccharide-based MenB vaccine was considered not to be feasible because its chemical composition is identical to a poly-sialic acid present in human glycoproteins such as fetal neural adhesion molecules, resulting in poor immunogenicity and the theoretical risk of developing auto-antibodies [Citation18].
The first vaccines used to prevent MenB IMD were detergent-extracted outer membrane vesicle (OMV) vaccines. OMVs originate from outer membrane and consist mainly of outer membrane proteins (OMPs), lipopolysaccharide, phospholipids and proteins. Detergent extraction reduces the amount of toxic lipopolysaccharide (LPS), but at the same time removes proteins that are weakly surface associated (e.g. lipoproteins). The dominant antigen of OMV is the major porin protein (PorA). PorA displays high antigenic variability, and multiple serosubtypes exist that show limited cross-reactivity between strains [Citation19]. Thus, because PorA is highly immunogenic, serum bactericidal activity (SBA) induced by OMV based-vaccines is only effective against the homologous strain [Citation20]. Three detergent-extracted OMV vaccines have been manufactured and used since the 1980s for the control of persisting MenB epidemics caused by a single clone. These vaccines have been used successfully in Norway, Cuba, Chile, New Zealand, and Normandy [Citation20–Citation25]. Vaccination induced SBA against the homologous (clonal) strain in all age groups, and contributed to attenuation of the outbreaks [Citation26].
The variability among PorA presents a challenge for the development of a broadly protective OMV vaccine. Multi-valent detergent-extracted OMV vaccines containing multiple PorA types have been proposed, and a hexavalent OMV vaccine was tested in clinical trials [Citation27]. An additional approach to OMV development uses detoxification of LPS based on genetic modification. This method has advantages over detergent extraction because it avoids loss of potentially immunogenic proteins and permits hyper-expression of other antigens, thereby potentially broadening the protective response. Some of these candidate OMVs have been tested in pre-clinical or phase I trials [Citation28–Citation31].
An alternative vaccination strategy relies on the identification of relevant surface antigens capable of inducing a broad seroprotective immune response. Two vaccines, 4CMenB and rLP2086, each using a different approach for antigen identification, have been developed and licensed for use with the aim of providing universal protection against MenB. Here, we review the principles underlying the development of 4CMenB and rLP2086 and the novel methods which have been used to estimate vaccine coverage. We update clinical and post-licensure experience with 4CMenB and rLP2086 and identify knowledge gaps where further investigation is ongoing.
2. Approaches to 4CMenB and rLP2086 target antigen identification
2.1. 4CMenB
Given the high level of genetic and antigenic diversity displayed by meningococci, identification of a single protein capable of providing universal protection was considered unlikely. The identification of suitable protein candidates and the development of 4CMenB proceeded via a process now known as ‘Reverse Vaccinology’, in which the complete genome sequence of a pathogenic strain of MenB (MC58) was analyzed and used to identify proteins suitable for further investigation as vaccine candidates. Of the 2158 genes present in the complete MC58 genome [Citation32], 570 were predicted in silico to encode for proteins either secreted or localized to the outer membrane. Of these, 350 were successfully expressed and purified as recombinant proteins in E. coli, and 28 were found to induce a functional SBA response [Citation33,Citation34]. The final antigen candidates were selected on the basis of their ability to induce SBA to homologous and heterologous strains, or to convey protection in a mouse challenge model [Citation33]. The three genome-derived Neisseria antigens (GNA) meeting these criteria were neisserial heparin binding antigen (NHBA or GNA 2132) [Citation35], fHbp (also known as GNA 1870 and LP2086) [Citation36], and Neisseria adhesin A (NadA or GNA 1994) [Citation37].
Following the successful application of Reverse vaccinology to MenB, this approach has been used extensively to identify potential antigens for several different pathogens [Citation38].
2.2. rLP2086
A more traditional proteomic approach was applied for the identification of potential vaccine candidates by the Pfizer group. Briefly, a mixture of soluble OMPs obtained after detergent extraction was fractionated and purified, and the resulting fractions were used to immunize mice to identify proteins capable of inducing SBA against a range of meningococcal strains [Citation39]. Through this process, the protein referred to as LP2086 (later named fHbp) was identified as potential candidate. The bivalent rLP2086 vaccine contains equal amounts of two fHbp variants, obtained in a lipidated form and selected on the principle that a combination of genetically different fHbp variants should induce broad coverage against the majority of clinical MenB strains [Citation40].
3. Antigen features and molecular epidemiology
3.1. Factor H binding protein (fHbp; GNA 1870 or LP2086)
fHbp is a surface lipoprotein expressed by the vast majority of MenB strains [Citation41]. Because fHbp was identified independently by two different groups [Citation36,Citation42], different nomenclature systems currently coexist: variant 1 (subfamily B) and variants 2 and 3 (subfamily A) [Citation39]. The three variants are serologically distinct and do not induce cross protective antibodies, although some low cross-reactivity exists between variants 2 and 3 in Subfamily A [Citation43,Citation44]. Pre-clinical studies have demonstrated that SBA mediated by anti-fHbp antibodies is not only affected by the genetic diversity of the antigen expressed by the different strains, but also by variations in levels of protein expression [Citation36]. Thus, SBA mediated by anti-fHbp antibodies against an individual strain will depend upon the variant of fHbp antigen that is expressed, and the level of its expression. FHbp shows very wide genetic diversity, with 1233 alleles and 972 peptides described as of October 2016 [Citation45]. The distribution of fHbp variants differs geographically and by age [Citation42,Citation43]. Variant 1 (Subfamily B) contained in 4CMenB and rLP2086 is found in at least 65% of MenB isolates globally, 61% of US clinical MenB strains and predominates in MenB clinical isolates from Europe, Western Australia and Brazil [Citation41,Citation46–Citation48]. Variants 2 and 3 (subfamily A) are found more frequently in carrier isolates, isolates from infants and the elderly with IMD, and predominates in South Africa [Citation49,Citation50].
The amount of fHbp expressed by different MenB strains is genetically determined and can vary at least 15-fold. Isolates from variant 2 and 3 (subfamily A) express considerably lower levels of fHpb than those belonging to variant 1 (subfamily B) [Citation51]. Although fHbp is expressed by almost all N. meningitidis strains studied to date [Citation52], a recent epidemiological analysis of US strains has shown that a proportion of MenC isolates belonging to the hypervirulent ST11 clone carry a truncated form of the fhbp gene [Citation53].
Recombinant fHbp induced SBA against a broad range of strains and passive protection against homologous and heterologous meningococcal challenge in animal models [Citation40]. Convalescent serum from humans found to contain detectable anti-fHbp antibodies supports the expression of fHbp during IMD [Citation54].
fHbp binds human Factor H, a major regulator of the complement pathway [Citation55,Citation56] (). Factor H binding results in down-regulation of the alternative complement pathway, reduced complement-mediated killing and improved bacterial survival in serum [Citation57,Citation58]. Anti-fHbp antibodies induce SBA, but may also have a secondary function in preventing Factor H binding by the meningococcus, thereby allowing activation of the alternative complement pathway leading to increased susceptibility to complement-mediated killing [Citation57,Citation58].
Table 1. Antigen features and biological role.
Studies in animal models transgenic for human factor H [Citation74,Citation75] and more recent studies in non-human primates [Citation76,Citation77] have raised the interesting hypothesis that interaction of wild-type fHbp with factor H could impair its immunogenicity by decreasing access to vaccine epitopes, and therefore non-factor H binding forms of fHbp might be more immunogenic in humans. Data generated in preclinical studies have shown that in some instances anti-fHbp antibodies might be capable of dislodging factor H from fHbp [Citation39,Citation58,Citation78]; on the other hand, data so far available from human studies are not enough to confirm or dismiss this possibility and, therefore, the validity of this hypothesis needs clinical confirmation by immunizing in parallel with factor H binding and factor H non-binding forms of fHbp. Crystallographic data are today available for all the three main fHbp variants, and the X-ray structure of the complex between fHbp and the domains 6 and 7 of human factor H has also allowed fine characterization of the residues involved in factor H binding [Citation56,Citation79] ().
Figure 1. Structural characterization and molecular epidemiology of antigens included in MenB vaccines. Upper panels: the three dimensional structures of the full length fHbp variant 1, the C-terminal portion of NHBA peptide 2, and the N-terminal portion of NadA variant 5 are reported. A schematic representation of the OMV architecture (PorA being the immunodominant antigen) is also shown.
Lower panels: split tree representations of the genetic variability of each of the three main protein antigens, plus PorA are shown.Footnote: Green and red circles indicate antigen variants present in 4CMenB and rLP2086, respectively.
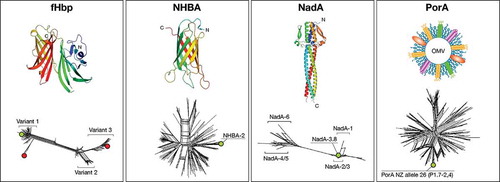
3.2. Neisseria heparin binding protein (NHBA; GNA 2132)
NHBA is a surface-exposed lipoprotein that is expressed by most meningococcal strains, including commensal Neisserial species and all MenB strains tested so far [Citation46,Citation61] (). NHBA is highly variable and 872 alleles and 677 peptides had been identified as of October 2016 (, ). There appears to be substantial regional variation in the distribution of NHBA peptides [Citation62–Citation64]; however, cross-protection across a variety of NHBA peptides has been described [Citation34]. The three-dimensional structure of the C-terminal region of NHBA reveals beta-barrel folding, which highlights interesting similarities with the corresponding domain of fHbp as well as with other outer membrane lipoproteins [Citation80] (). In the absence of the bacterial capsule, heparin binding mediated by NHBA is associated with increased survival of N. meningitidis in human serum and might facilitate adherence to host tissues by binding to heparan sulfate proteoglycans [Citation35]. Recently, it has been found that NHBA expression is temperature dependent and higher at 30°C and 32°C, suggesting that NHBA might be up-regulated in the nasopharyngeal niche and be involved in the attachment to epithelial cells and colonization [Citation81]. NHBA is recognized by sera from patients convalescing from IMD and induces SBA that correlates with the level of NHBA expression [Citation35]. Antibodies to fHbp and NHBA appear to work co-operatively to induce SBA [Citation65].
3.3. Neisserial adhesin A (NadA; GNA1994)
NadA is an autotransporter molecule that mediates adhesion to human epithelium and is thought to promote nasopharyngeal colonization and invasion [Citation37]. The recently solved three dimensional structure of a major portion of NadA reveals a trimeric fold, composed by an elongated coiled-coil stalk which sustains an N-terminal head-like domain, possibly involved in binding to epithelial cells (). It is likely that the role of NadA extends beyond adhesion because it can bind and activate macrophages and dendritic cells, bind human beta1 integrins and the extracellular chaperone human heat shock protein Hsp90 [Citation67–Citation70].
Unlike fHbp and NHBA, the NadA gene is present in up to 50% of all meningococcal strains, including three out of four hypervirulent clones (ET-5, ET-37, and cluster A4) [Citation46,Citation71]. Six variants exist of which NadA1, NadA2, and NadA3 are highly immunogenic and induce cross-reactive SBA responses [Citation71,Citation72]. NadA4 is associated with carriage strains and NadA5 is found on a single clonal complex (ST-269) [Citation66]. The level of NadA expression is regulated by the repressor protein NadR, and the level of expression varies markedly (up to 100-fold) between isolates [Citation73]. Interestingly, anti-NadA antibodies are detectable in IMD convalescent sera. Furthermore, Fagnocchi et al., showed that although sera from immunized infants was not able to induce complement-mediated bacterial killing of a selected MenB strain in vitro, the same sera passively protected infant rats by bacteremia from the same strain in a challenge model [Citation73]. These data suggest that NadA expression during IMD may be different than its expression under in vitro conditions, possibly due to inhibition of NadR regulatory activity in vivo, that is mediated by products of inflammation or signals present in saliva [Citation73]. This means that estimates of NadA-mediated vaccine coverage are likely to be underestimated, and that during infection, any strain carrying nadA gene could be susceptible to NadA-specific antibodies induced by vaccination.
4. Vaccine composition and pre-clinical data supporting formulation
The first candidate rMenB vaccine formulation contained three main recombinant protein antigens plus aluminum hydroxide. To improve immunogenicity and to simplify large-scale manufacturing [Citation33,Citation34], NHBA and fHbp were fused, respectively, to GNA1030 and GNA 2091 accessory antigens (proteins that did not induce broadly bactericidal antibodies but conveyed protection in animal models [Citation34]). This multicomponent vaccine was evaluated in preclinical studies and found to induce SBA against 78% of diverse clinical MenB isolates from U.S.A., U.K., Europe, Australia and other countries (N = 85). When the same preclinical serum was used for passive immunization of infant rats, it prevented meningococcemia in all immunized animals, compared with 100% bacteremia in controls that received phosphate buffered saline (PBS) [Citation34].
The final 4CMenB vaccine contains the fHbp-GNA2091 fusion protein (fHbp variant 1.1), NadA variant 3.1, the NHBA-GNA1030 fusion protein (NHBA peptide 2), and the OMV obtained from the NZ 98/254 strain (B:4:P1.7–2,4; ST-42 [cc41/44]), which was previously used successfully to curtail the outbreak in New Zealand [Citation66] (). The three recombinant proteins (rMenB) and 4CMenB (containing OMV) were tested separately in phase 2 trials. 4CMenB was selected after a phase 2 study in infants showing that the inclusion of the OMV component improved immunogenicity against heterologous strains as well as P1.4 homologous strains, possibly due to an adjuvant effect of OMV, to the presence of minor antigenic OMV components, or synergy between antibodies against OMV and recombinant antigens [Citation82]. The four vaccine components were selected with the aim of producing a highly immunogenic vaccine capable of providing broad protection against the majority of IMD caused by MenB. All MenB IMD strains contain genes for at least one 4CMenB component, and the majority contain genes for more than one, potentially providing multiple targets for vaccine-induced antibodies. By ensuring that each strain is targeted by more than one component, the risk of vaccine-escape mutants is also reduced.
Table 2. Composition of 4CmenB and rLP2086 [Citation83,Citation84].
rLP2086 contains two lipidated variants of fHbp; variant 3.45 (subfamily A05) and variant 1.55 (subfamily B01) (). The lipidated form was selected because of evidence in mice of improved immunogenicity compared to non-lipidated forms [Citation42]. In view of the cross-protection provided by fHbp variants within the same subfamily, it was considered that one variant from each fHbp subfamily could be enough to induce immunity against most strains of meningococcus. This is supported by a study in which sera from rabbits immunized with rLP2086 killed 87 out of 100 clinical MenB isolates expressing different fHbp variants [Citation59]. Significantly, the level of killing was not correlated to the fHbp genotype, but to the level of fHbp expression [Citation39,Citation59].This feature of the rLP2086 vaccine was exploited in the development of a method to predict vaccine coverage (see below). By contrast, a different study using non-lipidated fHbp showed that in a mouse model, SBA differed substantially between each variant group tested, indicating that beside expression levels, small amino-acid differences between variant proteins may also influence immunogenicity [Citation44].
5. Correlates of protection and assessment of immunogenicity
The measurement of the SBA of antibodies using human complement as the complement source is widely accepted as a surrogate marker of protection against meningococcal disease [Citation85]. For hSBA the accepted cut-off for protection is a titer of 4 [Citation86]; confirmed by post-licensure experience with MenC vaccines in the UK [Citation87,Citation88], and now extrapolated to other serogroups to support licensure of multivalent conjugate vaccines [Citation89].
The demonstration of immunogenicity was approached in different ways during the development of 4CMenB and rLP2086 (). 4CMenB is a multicomponent vaccine able to elicit bactericidal antibodies against the four key antigens combined therein. In order to assess the immunogenicity of each of the 4CMenB components, it was necessary to measure antigen-specific SBA through the use of N. meningitidis strains selectively recognized by antibodies against each of the antigen [Citation90]. The four antigen specific selected strains were not matched to any of the other three antigens to ensure that each strain only measured the contribution of one antigen (). These ‘indicator’ strains were isolated from cases of IMD and were used in hSBA assays to provide evidence of the functional antibody response to each of the four vaccine antigens [Citation90]. These strains have been evaluated and accepted by regulatory agencies for this purpose. The four strains are genetically diverse (); they belong to different clonal complexes, but are not considered to be representative of disease-causing isolates.
Figure 2. Indicator and test strain selection to assess immunogenicity of 4CMenB and rLP2086. The two tables report the main features of the selected strains, including antigen variant, clonal complex (CC) and sequence type (ST) (when available), and country of origin. The tree is an MLST-based representation of MenB strain epidemiology. The main ST types are represented as colored spheres, whose dimension is proportional to the frequency of that ST type. The most prevalent CCs are shown with different colors. 4CMenB indicator strains are indicated with arrows, according to their ST types. rLP2086 test strains are only indicated according to their CC characterization, as ST type is not known.
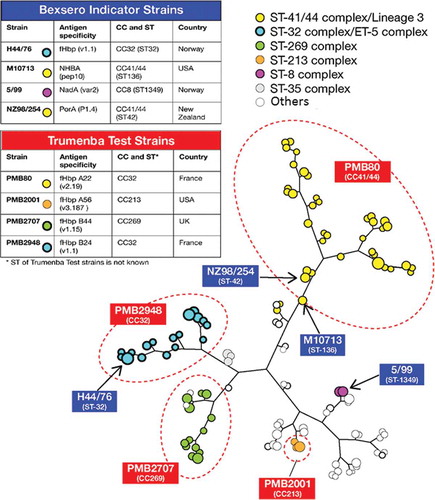
For rLP2086, four MenB strains expressing different amount of fHbp were used as test strains [Citation41] to represent fHbp subfamilies A and B [Citation91] (). These strains were used to indicate the breadth of the immune response induced by rLP2086. As for the indicator strains used to assess 4CMenB, the rLP2086 test strains are genetically diverse (), are used to measure the functional antibody response induced, but are not representative of the breadth of coverage.
6. Estimates of vaccine coverage
The genetic and phenotypic diversity of surface structures on individual strains of MenB means that estimates of the coverage likely to be provided by MenB protein vaccines in a given geographic region would require testing a very large numbers of strains. This is not feasible using hSBA because of the requirement for large volumes of sera and of human complement. Two different, novel methods to predict vaccine coverage have been developed for 4CMenB and rLP2086, respectively.
For 4CMenB, a high-throughput in vitro assay was developed called the meningococcal antigen typing system (MATS) (). MATS combines conventional genotyping for PorA with a specialized sandwich ELISA that determines phenotypic expression and cross-reactivity (or relative potency, RP) of fHbp, NadA, and NHBA on individual strains [Citation92,Citation93]. To mitigate the need to perform hSBA on large panels of isolates, the MATS method established a minimum level of RP for each antigen, named the positive bactericidal threshold (PBT) that predicts whether a given MenB isolate would be susceptible to killing in hSBA by antibodies induced by 4CMenB [Citation92].
Figure 3. Schematic representation of the MATS and MEASURE assays, which are employed for coverage estimates of 4CMenB and rLP2086, respectively. MATS combines conventional genotyping for PorA with a specialized sandwich ELISA approach, which uses polyclonal antibodies raised against each recombinant protein antigen to determine level of antigen expression and cross-reactivity on individual strains under examination (value is expressed as relative potency, RP, versus a reference strain). hSBA testing of a consistent number of MenB strains using pooled serum from infants vaccinated with 4CMenB allowed the definition of a minimum level of RP (named PBT, positive bactericidal threshold) that predicts whether a given strain would be susceptible to killing by antibodies induced in infants by 4CMenB. The MEASURE assay is based on the use of a mouse monoclonal antibody targeting a conserved fHbp epitope and is able to measure the amount of fHbp expressed by any given strain. The relationship between fHbp surface expression and susceptibility to killing in the SBA was assessed by hSBA testing of rabbit and adult human sera on a panel of diverse MenB strains. A fluorescence reactivity threshold was defined to predict strains potentially killed by antibodies raised against rLP2086.
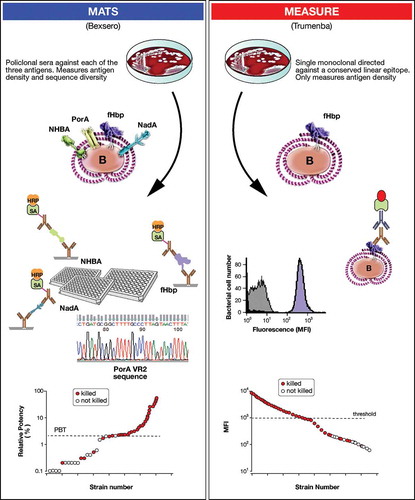
MATS is a valuable tool allowing prediction of 4CMenB coverage in individual countries using panels of regionally representative strains. Predicted coverage afforded by 4CMenB has been estimated at 78% in five countries in Europe, 73% in England and Wales [Citation94], 89.2% in Greece [Citation95], 66% in Canada [Citation96] and 91% in the US [Citation97]. In each country, the highest contribution to coverage was from fHbp and NHBA. In a study that used 40 reference strains representative of IMD isolates in England and Wales during 2007 and 2008, predicted coverage by MATS was 70%, while 88% of strains were killed in the hSBA assay indicating that MATS is a conservative predictor of strain coverage [Citation98]. Similar observations were made in a study using sera from Spain [Citation99]. A strong relationship between seroprotection predicted using pooled versus individual sera in hSBA assays has been demonstrated [Citation100].
Predictions of coverage afforded by rLP2086 are estimated using the novel MEningococcal Antigen SURface Expression (MEASURE) assay, which uses flow cytometry to assess fHbp surface expression and accessibility of fHbp to antibodies on whole bacteria [Citation39] (). This measure is based on the observation by Jiang et al. that SBA is dependent on fHbp expression rather than on the type of variant [Citation59]. A monoclonal pan-fHbp antibody (MN86-994-11) is used to measure surface expression of fHbp. MN86-994-11 is thought to recognize all fHbp variants and subvariants. Strains with surface expression values above a threshold of 1000 (mean fluorescent intensity units) are generally killed in SBA assays. The assay appears to be independent of strain encapsulation. Although no estimates of coverage are provided, original data with MEASURE would indicate that approximately 13% of strains have a fHbp expression below the threshold needed for killing by anti-fHbp antibodies [Citation59].Currently there is no information about the MEASURE data obtained on strain panels from different geographic regions.
7. Clinical experience with MenB vaccines
7.1. 4CMenB
The clinical development of 4CMenB was conducted in different age groups, ranging from 2 months to 50 years of age. In early studies hSBA was evaluated in panels of 7–15 genetically and geographically diverse MenB strains [Citation82,Citation101,Citation102]. Later studies focused on the immune response to selected indicator strains. Key features of phase 2 and 3 studies are summarized in (infants and children) and (adolescents and adults).
Table 3. Phase 2 and 3 studies of immunogenicity conducted with 4CMenB in healthy infants and children.
Table 4. Phase 2 and 3 studies conducted with 4CMenB in adolescents and adults.
7.1.1. Immunogenicity in infants and children
Vaccination of infants with 4CMenB in a three-dose primary plus booster schedule, with or without co-administration with other routinely administered pediatric vaccines, was assessed in five clinical studies () [Citation82,Citation106–Citation108,Citation116,Citation117]. In a large pivotal study, 84–100% of infants achieved hSBA titers ≥4 against the indicator strains after the third dose of a series administered at 2, 4, and 6 months, increasing from 95% to 100% after a booster dose at 12 months of age [Citation106]. The immune response to 4CMenB was also shown to be consistently high in other studies using 2, 3, 4 or 2.5, 3.5, 5 month schedules, followed by a booster given 6–20 months later ().
One study assessed the immune response to 4CMenB administered through an accelerated schedule, initiated at 3 months of age [Citation108,Citation109]. The percentages of infants with hSBA titers ≥4 across the indicator strains after the second dose received at 5 months of age, ranged from 44% to 100% when 4CMenB was administered alone and from 68% to 97% when co-administered with meningococcal serogroup C vaccine [Citation108,Citation109]
Three studies assessed two-dose primary vaccination schedule in toddlers aged from 6 to 18 months of age [Citation101,Citation108,Citation109,Citation118]. Two doses of 4CMenB were immunogenic in this age group, with 93–100% of subjects achieving hSBA titers ≥4 across indicator strains.
Four studies assessed two-dose catch-up regimens in children between 2 and 10 years of age [Citation103,Citation105,Citation106,Citation119]. In one such study, 94% of children achieved hSBA titers ≥4 across strains [Citation108], which was consistent with results from earlier smaller studies ().
One follow-up study of immunogenicity showed persistence of hSBA titers up to 28 months after the last vaccine dose in infants and children [Citation104]. Despite waning of antibodies after priming in infancy, booster vaccination induced marked rises in hSBA GMTs, indicative of an anamnestic response [Citation104].
One recently reported study demonstrated that 4CMenB is immunogenic in children with complement deficiencies and splenic dysfunction [Citation120].
Co-administration of 4CMenB with other antigens included in recommended pediatric vaccines (diphtheria, tetanus, acellular pertussis, Haemophilus influenzae type b, inactivated poliomyelitis, hepatitis B, heptavalent pneumococcal conjugate, meningococcal C conjugate, rotavirus, measles, mumps, rubella, and varicella) did not impact the overall immune response to either vaccine [Citation82,Citation106,Citation107,Citation116,Citation117].
7.1.2. Immunogenicity in adolescents and adults
The immunogenicity of 4CMenB administered to adolescents and adults was assessed in three studies in subjects aged between 11 and 24 years, and one study in 18–50 year olds (). In the large pivotal study in adolescents the percentage of subjects with hSBA titers ≥4 against the indicator strains after the second dose ranged from 98% to 100% across schedules (either 0 and 1 month, 0 and 2 months, or 0 and 6 months) [Citation111–Citation113]. Evidence of an early response was demonstrated in the same study where hSBA titers ≥4 ranged from 93% to 96% after the first dose [Citation111]. Antibody persistence data at 18–23 months after the second dose showed hSBA titers ≥4 ranging from 77% to 94% across groups [Citation112]. Further studies performed in adolescents and adults showed an overall high immune response after two doses of 4CMenB against the same strains and an additional four strains [Citation110,Citation114,Citation115,Citation121].
7.1.3. Safety
In clinical trials 4CMenB was associated with local and systemic reactions that were transient and largely of mild-to-moderate severity. In infants, local and systemic reactions appeared to be more frequent when 4CMenB was co-administered with other vaccines [Citation106,Citation117]. Elevated body temperature (≥38°C) was reported more frequently after co-administration of 4CMenB with routine pediatric vaccines than after intercalated administration, although rates of medical attendance after vaccination and fever-related serious adverse events (SAEs) were low [Citation106,Citation117,Citation122]. Prophylactic administration of paracetamol significantly reduces the occurrence of fever, particularly fever ≥38.5°C, without influencing the immunogenicity of either 4CMenB or the co-administered routine vaccines [Citation107,Citation123], and is recommended by the UK and Australian authorities when 4CMenB is administered to infants. The OMV vaccine component, shown to induce fever when used in New Zealand infants [Citation124], is likely a contributing factor to the reactogenicity observed after 4CMenB.
In adolescents, transient pain at injection site was the most commonly reported reaction, but fever was uncommon [Citation111,Citation114].
Clinical data are supplemented by experience in more than 17,000 university students in the US, more than 500,000 UK infants who received 4CMenB since the commencement of the national immunization program in September 2015 (considering the birth cohort and >90% coverage [Citation125,Citation126]), and in more than 43,000 vaccinees between 2 months and 20 years of age in the Saguenay-Lac-Saint-Jean region of Quebec [Citation123,Citation127]. The findings from active and passive safety surveillances for adverse events conducted during those vaccination campaigns are in line with the clinical safety database, with an observed lower incidence of fever. In addition, no safety signal has been identified [Citation123,Citation128].
7.1.4. Carriage
The interruption of meningococcal transmission is recognized as a critical factor in the success of immunization programs [Citation129]. A UK study conducted in 2954 subjects aged 18–24 year olds did not detect a significant effect of 4CMenB on carriage of MenB strains specifically, although there was a trend toward lower carriage which did not reach statistical significance due to the low number of events [Citation115]. Interestingly, the carriage of other serogroups (BCWY, mainly serogroup Y), was significantly reduced (26.6%) during the year following vaccination, due to reduction in acquisition of these serogroups.
7.2. rLP2086
7.2.1. Immunogenicity in adolescents and young adults
summarizes the key features of phase 2 and 3 studies conducted in adolescents and adults. Early studies in 11–40-year-old subjects used different homologous and heterologous MenB strains [Citation130,Citation131]. Later studies used four standard heterologous test MenB strains for hSBA testing.
Across large phase 2 studies, the percentages of subjects aged 10–18 years with hSBA titers greater or equal to the lower limit of quantitation (LLQ) for indicator strains after a third rLP2086 dose was consistently high, ranging from 81.5% to 100% when administered as a 0, 2, and 6-month schedule (). Percentages of subjects achieving hSBA titers ≥LLQ were lower after two doses administered 2 months apart (range: 54.5–98.5%) ().
Table 5. Phase 2 and 3 studies conducted with rLP2086.
In a phase 2 study in which adolescents aged 11–18 years received three doses of rLP2086 in either a 0, 1, 6 or 0, 2, 6-month schedule, or, two doses given 6 months part, the percentage of subjects with hSBA titers ≥1:8 were comparable between the two-dose and the three-dose series with 70–98% of adolescents with hSBA titers ≥LLQ for each of the four test strains () [Citation138].
Investigation of a further 10 test strains in large phase 3 studies showed that whilst a substantial proportion of subjects achieved hSBA titers ≥LLQ after two doses, further increases were observed after a third vaccination [Citation136,Citation137].
Persistence data indicate that after a three-dose schedule in adolescents aged 11–18 years, 20.4–59.0% of subjects sustained hSBA titers >LLQ for the four indicator strains for up to 4 years [Citation139]. Co-administration of rLP2086 with reduced antigen content diphtheria-tetanus-acellular pertussis (Tdap)-inactivated polio vaccine, 4-valent human papillomavirus vaccine (HPV4), and Tdap and quadrivalent meningococcal vaccine did not impact the overall immune response to either vaccine [Citation133–Citation135].
7.2.2. Safety
The clinical development of rLP2086 in infants was suspended after the early termination of a phase 1/2 study in which high rates of fever were observed among individuals receiving low doses of vaccine [Citation140]. A previous study in toddlers in which fever was reported in 0–40.9% of toddlers receiving any rLP2086 dose, had not raised such concerns [Citation141].
In clinical trials in adolescents and adults rLP2086 was associated with local and systemic reactions that were transient and largely of mild-to-moderate severity. In adolescents transient pain at injection site was the most commonly reported reaction.
In the largest phase 3 placebo-controlled study designed to assess the tolerability and safety, and in particular the occurrence of less common adverse events, rLP2086 confirmed to be safe and well tolerated in individuals from 10 to 40 years of age [Citation130,Citation131].
7.2.3. Carriage
Clinical studies evaluating the impact of rLP2086 on nasophayngeal carriage in adolescents are yet to be reported [Citation142]. Recently, the results of a carriage study conducted during vaccination campaigns at Providence College and at the University of Oregon have shown no impact of the vaccine on the carriage and acquisition of meningococcal strains in this group of subjects [Citation143].
8. Effectiveness
The effectiveness of 4CMenB was evaluated in two studies, the first one in Saguenay Lac Saint Jean, a small region of Quebec in Canada, and the second one in the United Kingdom. So far there are no data on the effectiveness of LP2013.
The incidence of MenB IMD in Saguenay Lac Saint Jean was higher than in the rest of Canada (2.6/100,000 population among 15–19 year olds in 2011 versus 0.7/100,000 in 15–19 year olds across Canada) [Citation16]. In May 2014, a campaign commenced to vaccinate the population from 2 month to 20 years of age. More than 43,000 individuals were immunized [Citation123]. Since the vaccination started, no IMD cases were reported in the vaccinated population, while two cases were reported in unvaccinated individuals, and IMD continued to be reported in the surrounding regions [Citation144]. It is tempting to speculate that 4CMenB not only induced complete direct protection on vaccinees, but very likely induced herd immunity that protected the infants born after May 2014 that were not part of the vaccination campaign. Starting on the 1st September 2015, 4CMenB was introduced in the National Immunization Program in the United Kingdom and given to all newborns according to a 2, 4, and 12 months schedule [Citation122,Citation145]. The decision to implement a two-dose primary schedule was based on evidence from other vaccines and from preliminary data not published at the time [Citation145]. It was considered that age and the interval between doses were likely to have a greater impact on antibody titers than the number of doses administered. Compliance with vaccination has been more than 90% [Citation146], and by June 2016, when approximately 500,000 infants had received two doses, the first analysis of safety and effectiveness was reported. The overall effectiveness against IMD measured at approximately six months after the second vaccination, was of 83%, and the efficacy against vaccine-preventable strains was 94% [Citation147,Citation148]. The UK campaign will continue for three additional years and will provide the opportunity to investigate effectiveness after the booster dose at 12 months of age.
Vaccine effectiveness has been shown indirectly in outbreak settings. In 2015, rLP2086 was used during a MenB IMD outbreak at Providence College, Rhode Island, and was used along with 4CMenB in an outbreak at the University of Oregon [Citation127]. 4CMenB has been used to control MenB outbreaks at University and college campuses in New Jersey and California [Citation128,Citation149,Citation150]. In these settings, no cases of MenB disease have been reported in vaccinated individuals. During a recent outbreak in Santa Clara County, no cases of MenB disease were identified in after implementation of the 4CMenB vaccination campaign (as of May 2016) [Citation150].
Information from a 2013 meningococcal serogroup B outbreak at Princeton University raised questions about 4CMenB coverage based on lower-than expected hSBA responses to the outbreak strain among vaccinated individuals (66% of 499 individuals who received two doses of vaccine were seropositive) [Citation151]. However, while the presence of bactericidal antibodies is an indication of protection, the absence of bactericidal antibodies is not an indication of susceptibility [Citation152]. Indeed, the United Kingdom estimate of 82.9% vaccine effectiveness confirms that protection conferred by 4CMenB is high and broad [Citation148]. Parikh et al. reported high vaccine effectiveness against all laboratory-confirmed cases of disease, irrespective of the predicted strain coverage using MATS [Citation148]. Basta et al. commented on the importance of such population-based studies in providing essential evidence about the impact of newly developed vaccines and vaccination programs [Citation151].
9. Regulatory status and recommendations for use
4CMenB received a positive approval from the Committee for Medicinal Products for Human Use in November 2012 and was licensed in the EU in January 2013. 4CMenB is currently approved in 39 countries including Europe, Australia, Canada, and several countries in South America. US approval was received in 2015. 4CMenB is licensed for use from the age of 2 months in Europe and several other countries in a three-dose schedule with booster, and for 10–25 year olds as a two-dose schedule in the US.
Clinical recommendations for use of 4CMenB made by at least 14 countries encompass the immunization of at-risk groups such as infants, travelers, close contacts of cases of IMD, and those with underlying medical conditions that put them at increased risk [Citation149]. To date, majority of these clinical recommendations are not linked to funding.
As well as the UK, 4CMenB has recently been recommended for routine schedule inclusions in Ireland (for infants from late 2016 [Citation153]) and in Italy [Citation154]. Nationwide expansion of 4CMenB funding in Italy follows its use since 2014 in eight regions and one province for children under 1 year of age [Citation154].
rLP2086 was licensed in the United States in October 2014 as a three-dose schedule in 10–25 year olds. In April 2016 rLP2086 was approved in the US for use in a two-dose schedule (administered at 0 and 6 months) and the three-dose schedule was relaxed to allow administration of the second dose between 1 and 2 months post-dose 1. rLP2086 was submitted for review in Europe in 2016, with a decision expected in early 2017. At this time rLP2086 has not been included in the national immunization program of any country.
4CMenB and rLP2086 are both recommended for use in the 10–25 year age group in the US. Both vaccines have a ‘Category A’ recommendation (recommended for all persons in an age-based or risk-factor-based group) for use in individuals aged 10 years or more who are at increased risk for IMD due to complement deficiencies, anatomic or functional asplenia, routine laboratory exposure to isolates of N. meningitidis, and during a MenB outbreak [Citation155]. Under a ‘Category B’ recommendation (recommendation made for individual clinical decision making), both vaccines may be used in 16–23 year olds (preferred age 16–18 years) [Citation156].
4CMenB can be used as two doses given at least one month apart (0,1 or 0, 2 or 0,6 schedules are all acceptable), while for rLP2086, three doses given at 0, 1–2, and 6 months should be used for persons at increased risk and during outbreaks. When administered to healthy adolescents who are not at increased risk for IMD, two doses of rLP20186 should be administered at 0 and 6 months, except if the second dose is given at <6 months, in which a third dose should be given 6 months after the first dose.
10. Expert commentary
For the first time in history two vaccines offer broad protection against IMD caused by MenB. These vaccines, together with the already licensed tetravalent conjugate vaccines against serotypes A,C,Y, and W, offer the possibility to reach a new milestone in history of medicine and eliminate meningococcal disease globally. However, this requires global vaccine recommendations and deployment, and this is still challenging. Although the number of recommendations for MenB vaccination is steadily increasing and the number of countries considering implementation is growing, several key questions still need to be addressed. The most important question is whether the vaccine is cost-effective. Although IMD is devastating, it is relatively rare. Because of this, most of the time official cost-effectiveness calculations conclude that MenB vaccines are not cost-effective, because cost-effectiveness evaluations favor vaccines against milder but more frequent diseases. On the other hand, parents and community members generally disagree with the official ‘cost-effectiveness’ approach because they prioritize vaccination for severe diseases that may cause death or permanent sequelae for which there is no second chance, over vaccination against mild diseases that are not life threatening.
Other outstanding questions are the appropriate age groups to be immunized, the degree and duration of efficacy, and importantly, the impact on nasopharyngeal carriage which has implications for strategies to interrupt transmission and maximize herd protection effects. Universal vaccination programs such as those undertaken in Quebec and in the UK will continue to provide important information on these issues.
Finally, both Men B vaccines are composed by proteins that are present also in other meningococcal serogroups and Neisseria species, and 4CMenB has been reported to induce bactericidal antibodies against serogroup X strains from Africa [Citation157], as well as MenW strains from England, Wales, Germany, France and Brazil [Citation158–Citation160]. The MenZB vaccine, which is one of the components of 4CMenB has also been reported to protect from infection with N. gonorrhoeae [Citation161].
11. Five-year view
IMD is a rare disease and large-scale programs are the best way to generate evidence needed to support effectiveness, safety, carriage and wider impacts on non-B serogroups and other Neisserial pathogens. The UK mass immunization program is already providing, and will continue to provide real-world evidence of the potential for 4CMenB to protect against diverse circulating strains. The next five years will provide info on the effectiveness and impact of MenB vaccines in protecting the individuals at highest risk of disease; infants, young children and adolescents. Additional data on carriage impact will inform vaccination strategy, and the potential for indirect protection and population-level impacts. The utility of MATS and MEASURE as predictors of vaccine coverage will be validated. The role of these assays will expand if they are capable of predicting the impact of vaccination on nasopharyngeal carriage and if they can be effectively used to track vaccine impact and changes in MenB epidemiology [Citation162].
Key issues
Two new vaccines offer broad protection against IMD caused by MenB for the first time. 4CMenB and rLP2086 were developed using different antigen-selection methods and each used novel ways to overcame challenges associated with demonstrating immunogenicity and estimating coverage.
4CMenB combines four components, each targeting a different mechanism involved in MenB survival and disease development. One hundred percent of invasive MenB strains contain genes for at least one component and the majority of invasive MenB strains contain genes for more than one component, potentially providing multiple targets for vaccine-induced antibodies and potentially protecting against invasive MenB strains even when the expression of one antigen is low or antigenically different.
rLP2086 combines fHbp variants selected from each of the two major subfamilies on the principle that combining a representative fHbp from each subfamily should induce broad coverage against the majority of invasive MenB strains. Most invasive MenB strains contain genes for fHbp, although the level of expression can be variable. The recent report [Citation53] that MenC strains carrying a truncated form of the fHbp gene are emerging, suggests that the fHbp gene is dispensable and that vaccination with one antigen alone may select vaccine escape mutants.
Further large-scale studies will provide information on the impact of MenB vaccines on carriage, and their wider impact on non-B serogroups and other Neisserial pathogens.
Declaration of interest
All authors are employees of the GSK group of companies. D Toneatto, V Masignani, M Pizza and R Rappuoli hold shares in the GSK group of companies as part of his/her employee remuneration. The authors have no other relevant affiliations or financial involvement with any organization or entity with a financial interest in or financial conflict with the subject matter or materials discussed in the manuscript apart from those disclosed. Writing assistance was provided by Joanne Wolter (Independent medical writer on behalf of GSK) and editorial and coordination assistance was provided by Maria Ana De la Grandiere (XPE Pharma & Science on behalf of GSK) and funded by GlaxoSmithKline Biologicals SA.
Trademarks
Bexsero is a trademark of the GSK group of companies. Trumenba is a trademark of Pfizer Inc.
Acknowledgments
The authors wish to thank Phil Watson, Kumaran Vadivelu and Patricia Novy for their critical review of the paper.
Additional information
Funding
References
- Cohn AC, MacNeil JR, Harrison LH, et al. Changes in Neisseria meningitidis disease epidemiology in the United States, 1998-2007: implications for prevention of meningococcal disease. Clin Infect Dis. 2010;50:184–191.
- Christensen H, May M, Bowen L, et al. Meningococcal carriage by age: a systematic review and meta-analysis. Lancet Infect Dis. 2010;10:853–861.
- Soriano-Gabarró M, Wolter J, Hogea C, et al. Carriage of Neisseria meningitidis in Europe: a review of studies undertaken in the region. Expert Rev Anti Infect Ther. 2011;9:761–774.
- Pollard AJ, Ochnio J, Ho M, et al. Disease susceptibility to ST11 complex meningococci bearing serogroup C or W135 polysaccharide capsules, North America. Emerg Infect Dis. 2004;10:1812–1815.
- Baccarini C, Ternouth A, Wieffer H, et al. The changing epidemiology of meningococcal disease in North America 1945-2010. Hum Vaccines Immunother. 2013;9:162–171.
- Harrison LH, Trotter CL, Ramsay ME. Global epidemiology of meningococcal disease. Vaccine. 2009;27(Suppl 2):B51–B63.
- Khatami A, Pollard AJ. The epidemiology of meningococcal disease and the impact of vaccines. Expert Rev Vaccines. 2010;9:285–298.
- European Center for Disease Prevention and Control. Surveillance report. Surveillance of invasive bacterial diseases in Europe. 2012.
- Bröker M, Emonet S, Fazio C, et al. Meningococcal serogroup Y disease in Europe: continuation of high importance in some European regions in 2013. Hum Vaccines Immunother. 2015 Jun 2;11(9):2281–2286.
- Agnememel A, Hong E, Giorgini D, et al. Neisseria meningitidis Serogroup X in Sub-Saharan Africa. Emerg Infect Dis. 2016;22:698–702.
- Ladhani SN, Beebeejaun K, Lucidarme J, et al. Increase in endemic Neisseria meningitidis capsular group W sequence type 11 complex associated with severe invasive disease in England and Wales. Clin Infect Dis. 2015;60:578–585.
- Campbell H, Saliba V, Borrow R, et al. Targeted vaccination of teenagers following continued rapid endemic expansion of a single meningococcal group W clone (sequence type 11 clonal complex), United Kingdom 2015. Euro Surveil. 2015;20(28). pii: 21188.
- Australian Government Department of Health Office of Health Protection. Meningococcal - Australian Meningococcal Surveillance Programme annual reports [Internet]; [2016 Jul 18]. Available from: http://www.health.gov.au/internet/main/publishing.nsf/content/cda-pubs-annlrpt-menganrep.htm
- Wormsbecker AE, Wong K, Jamieson FB, et al. Epidemiology of serogroup C and Y invasive meningococcal disease (IMD) in Ontario, 2000-2013: vaccine program impact assessment. Vaccine. 2015;33:5678–5683.
- Miller E, Salisbury D, Ramsay M. Planning, registration, and implementation of an immunisation campaign against meningococcal serogroup C disease in the UK: a success story. Vaccine. 2001;20(Suppl 1):S58–S67.
- The recommended use of the multicomponent meningococcal B (4CMenB) vaccine in Canada - Public Health Agency of Canada [Internet]; [ cited 2016 Apr 4]. Available from: http://www.phac-aspc.gc.ca/naci-ccni/mening-4cmenb-exec-resum-eng.php
- Centers for Disease Control and Prevention. Active Bacterial Core Surveillance (ABCs) [Internet]; [ cited 2016 Jun 27]. Available from: http://www.cdc.gov/abcs/reports-findings/surv-reports.html
- Finne J, Leinonen M, Mäkelä PH. Antigenic similarities between brain components and bacteria causing meningitis. Implications for vaccine development and pathogenesis. Lancet. 1983;2:355–357.
- Urwin R, Russell JE, Thompson EAL, et al. Distribution of surface protein variants among hyperinvasive meningococci: implications for vaccine design. Infect Immun. 2004;72:5955–5962.
- Tappero JW, Lagos R, Ballesteros AM, et al. Immunogenicity of 2 serogroup B outer-membrane protein meningococcal vaccines: a randomized controlled trial in Chile. Jama. 1999;281(16):1520–1527.
- Sierra GV, Campa HC, Varcacel NM, et al. Vaccine against group B Neisseria meningitidis: protection trial and mass vaccination results in Cuba. NIPH Ann. 1991;14:195–207–210.
- Caron F, Du Châtelet IP, Leroy J-P, et al. From tailor-made to ready-to-wear meningococcal B vaccines: longitudinal study of a clonal meningococcal B outbreak. Lancet Infect Dis. 2011;11:455–463.
- Oster P, Lennon D, O’Hallahan J, et al. MeNZB: a safe and highly immunogenic tailor-made vaccine against the New Zealand Neisseria meningitidis serogroup B disease epidemic strain. Vaccine. 2005;23:2191–2196.
- Boslego J, Garcia J, Cruz C, et al. Efficacy, safety, and immunogenicity of a meningococcal group B (15: P1.3)outer membrane protein vaccine in Iquique, Chile. Chilean National Committee for Meningococcal Disease. Vaccine. 1995;13:821–829.
- Fredriksen JH, Rosenqvist E, Wedege E, et al. Production, characterization and control of MenB-vaccine “Folkehelsa”: an outer membrane vesicle vaccine against group B meningococcal disease. NIPH Ann. 1991;14:67–79–80.
- Holst J, Martin D, Arnold R, et al. Properties and clinical performance of vaccines containing outer membrane vesicles from Neisseria meningitidis. Vaccine. 2009;27(Suppl 2):B3–B12.
- Poolman JT, Richmond P. Multivalent meningococcal serogroup B vaccines: challenges in predicting protection and measuring effectiveness. Expert Rev Vaccines. 2015;14:1277–1287.
- Kaaijk P, Van Straaten I, Van De Waterbeemd B, et al. Preclinical safety and immunogenicity evaluation of a nonavalent PorA native outer membrane vesicle vaccine against serogroup B meningococcal disease. Vaccine. 2013;31:1065–1071.
- Rots NY, Kleijne D Safety of a nonavalent meningococcal serogroup B vaccine in healthy adult volunteers in a randomised, controlled, single blind study. 16th International Pathogenic Neisseria Conference; 2008 Sept 7-12; Rotterdam, The Netherlands.
- Koeberling O, Ispasanie E, Hauser J, et al. A broadly-protective vaccine against meningococcal disease in sub-Saharan Africa based on generalized modules for membrane antigens (GMMA). Vaccine. 2014;32:2688–2695.
- Keiser PB, Biggs-Cicatelli S, Moran EE, et al. A phase 1 study of a meningococcal native outer membrane vesicle vaccine made from a group B strain with deleted lpxL1 and synX, over-expressed factor H binding protein, two PorAs and stabilized OpcA expression. Vaccine. 2011;29:1413–1420.
- Tettelin H, Saunders NJ, Heidelberg J, et al. Complete genome sequence of Neisseria meningitidis serogroup B strain MC58. Science. 2000;287:1809–1815.
- Pizza M, Scarlato V, Masignani V, et al. Identification of vaccine candidates against serogroup B meningococcus by whole-genome sequencing. Science. 2000;287:1816–1820.
- Giuliani MM, Adu-Bobie J, Comanducci M, et al. A universal vaccine for serogroup B meningococcus. Proc Natl Acad Sci USA. 2006;103:10834–10839.
- Serruto D, Spadafina T, Ciucchi L, et al. Neisseria meningitidis GNA2132, a heparin-binding protein that induces protective immunity in humans. Proc Natl Acad Sci U S A. 2010;107:3770–3775.
- Masignani V, Comanducci M, Giuliani MM, et al. Vaccination against Neisseria meningitidis using three variants of the lipoprotein GNA1870. J Exp Med. 2003;197:789–799.
- Capecchi B, Adu-Bobie J, Di Marcello F, et al. Neisseria meningitidis NadA is a new invasin which promotes bacterial adhesion to and penetration into human epithelial cells. Mol Microbiol. 2005;55:687–698.
- Adu-Bobie J, Capecchi B, Serruto D, et al. Two years into reverse vaccinology. Vaccine. 2003;21:605–610.
- McNeil LK, Zagursky RJ, Lin SL, et al. Role of factor H binding protein in Neisseria meningitidis virulence and its potential as a vaccine candidate to broadly protect against meningococcal disease. Microbiol Mol Biol Rev. 2013;77:234–252.
- Pillai S, Howell A, Alexander K, et al. Outer membrane protein (OMP) based vaccine for Neisseria meningitidis serogroup B. Vaccine. 2005;23:2206–2209.
- Murphy E, Andrew L, Lee K-L, et al. Sequence diversity of the factor H binding protein vaccine candidate in epidemiologically relevant strains of serogroup B Neisseria meningitidis. J Infect Dis. 2009;200:379–389.
- Fletcher LD, Bernfield L, Barniak V, et al. Vaccine potential of the Neisseria meningitidis 2086 lipoprotein. Infect Immun. 2004;72:2088–2100.
- Pajon R, Beernink PT, Harrison LH, et al. Frequency of factor H-binding protein modular groups and susceptibility to cross-reactive bactericidal activity in invasive meningococcal isolates. Vaccine. 2010;28:2122–2129.
- Seib KL, Brunelli B, Brogioni B, et al. Characterization of diverse subvariants of the meningococcal factor H (fH) binding protein for their ability to bind fH, to mediate serum resistance, and to induce bactericidal antibodies. Infect Immun. 2011;79:970–981.
- Neisseria factor H binding protein sequence typing [Internet]; [updated 2016 Jul 18; cited 2016 Jul 19]. Available from: http://pubmlst.org/neisseria/fHbp/
- Wang X, Cohn A, Comanducci M, et al. Prevalence and genetic diversity of candidate vaccine antigens among invasive Neisseria meningitidis isolates in the United States. Vaccine. 2011;29:4739–4744.
- Boan P, Metasan N, Tempone S, et al. Neisseria meningitidis porA, fetA and fHbp gene distribution in Western Australia 2000 to 2011. BMC Infect Dis. 2014;14:686.
- De Filippis I, De Lemos APS, Hostetler JB, et al. Molecular epidemiology of Neisseria meningitidis serogroup B in Brazil. PLoS One. 2012;7:e33016.
- Hoiseth SK, Murphy E, Andrew L, et al. A multi-country evaluation of Neisseria meningitidis serogroup B factor H-binding proteins and implications for vaccine coverage in different age groups. Pediatr Infect Dis J. 2013;32:1096–1101.
- Mothibeli KM, Du Plessis M, Von Gottberg A, et al. Distribution of factor H binding protein beyond serogroup B: variation among five serogroups of invasive Neisseria meningitidis in South Africa. Vaccine. 2011;29:2187–2192.
- Biagini M, Spinsanti M, De Angelis G, et al. Expression of factor H binding protein in meningococcal strains can vary at least 15-fold and is genetically determined. Proc Natl Acad Sci USA. 2016;113:2714–2719.
- Lucidarme J, Tan L, Exley RM, et al. Characterization of Neisseria meningitidis isolates that do not express the virulence factor and vaccine antigen factor H binding protein. Clin Vaccine Immunol. 2011;18:1002–1014.
- Chang H, Vuong J, Kretz C, et al. Molecular epidemiology of Neisseria meningitidis serogroup B vaccine antigens in the United States, 2009-2014. Abstract 122. 20th International Pathogenic Neisseria Conference; 2016 Sep 4–9; Manchester, UK.
- Ala’aldeen DAA, Flint M, Oldfield NJ, et al. Human antibody responses to the meningococcal factor H binding protein (LP2086) during invasive disease, colonization and carriage. Vaccine. 2010;28:7667–7675.
- Madico G, Welsch JA, Lewis LA, et al. The meningococcal vaccine candidate GNA1870 binds the complement regulatory protein factor H and enhances serum resistance. J Immunol Baltim Md 1950. 2006;177:501–510.
- Schneider MC, Prosser BE, Caesar JJE, et al. Neisseria meningitidis recruits factor H using protein mimicry of host carbohydrates. Nature. 2009;458:890–893.
- Welsch JA, Ram S, Koeberling O, et al. Complement-dependent synergistic bactericidal activity of antibodies against factor H-binding protein, a sparsely distributed meningococcal vaccine antigen. J Infect Dis. 2008;197:1053–1061.
- Giuntini S, Reason DC, Granoff DM. Complement-mediated bactericidal activity of anti-factor H binding protein monoclonal antibodies against the meningococcus relies upon blocking factor H binding. Infect Immun. 2011;79:3751–3759.
- Jiang H-Q, Hoiseth SK, Harris SL, et al. Broad vaccine coverage predicted for a bivalent recombinant factor H binding protein based vaccine to prevent serogroup B meningococcal disease. Vaccine. 2010;28:6086–6093.
- Brehony C, Wilson DJ, Maiden MCJ. Variation of the factor H-binding protein of Neisseria meningitidis. Microbiol Read Engl. 2009;155:4155–4169.
- Muzzi A, Mora M, Pizza M, et al. Conservation of meningococcal antigens in the genus Neisseria. Mbio. 2013;4:e00163–113.
- Tsang RS, Law DK, Gad RR, et al. Characterization of invasive Neisseria meningitidis from Atlantic Canada, 2009 to 2013: with special reference to the nonpolysaccharide vaccine targets (PorA, factor H binding protein, Neisseria heparin-binding antigen and Neisseria adhesin A). Can J Infect Dis Med Microbiol. 2015;26:299–304.
- Law DKS, Lefebvre B, Gilca R, et al. Characterization of invasive Neisseria meningitidis strains from Québec, Canada, during a period of increased serogroup B disease, 2009-2013: phenotyping and genotyping with special emphasis on the non-carbohydrate protein vaccine targets. BMC Microbiol. 2015;15:143.
- Abad R, Medina V, Stella M, et al. Predicted strain coverage of a new meningococcal multicomponent vaccine (4CMenB) in Spain: analysis of the differences with Other European Countries. PLoS One. 2016;11:e0150721.
- Vu DM, Wong TT, Granoff DM. Cooperative serum bactericidal activity between human antibodies to meningococcal factor H binding protein and neisserial heparin binding antigen. Vaccine. 2011;29:1968–1973.
- Serruto D, Bottomley MJ, Ram S, et al. The new multicomponent vaccine against meningococcal serogroup B, 4CMenB: immunological, functional and structural characterization of the antigens. Vaccine. 2012;30(Suppl 2):B87–B97.
- Montanari P, Bozza G, Capecchi B, et al. Human heat shock protein (Hsp) 90 interferes with Neisseria meningitidis adhesin A (NadA)-mediated adhesion and invasion. Cell Microbiol. 2012;14:368–385.
- Mazzon C, Baldani-Guerra B, Cecchini P, et al. IFN-gamma and R-848 dependent activation of human monocyte-derived dendritic cells by Neisseria meningitidis adhesin A. J Immunol Baltim Md 1950. 2007;179:3904–3916.
- Franzoso S, Mazzon C, Sztukowska M, et al. Human monocytes/macrophages are a target of Neisseria meningitidis Adhesin A (NadA). J Leukoc Biol. 2008;83:1100–1110.
- Nägele V, Heesemann J, Schielke S, et al. Neisseria meningitidis adhesin NadA targets beta1 integrins: functional similarity to Yersinia invasin. J Biol Chem. 2011;286:20536–20546.
- Comanducci M, Bambini S, Caugant DA, et al. NadA diversity and carriage in Neisseria meningitidis. Infect Immun. 2004;72:4217–4223.
- Bambini S, De Chiara M, Muzzi A, et al. Neisseria adhesin A variation and revised nomenclature scheme. Clin Vaccine Immunol CVI. 2014;21:966–971.
- Fagnocchi L, Biolchi A, Ferlicca F, et al. Transcriptional regulation of the nadA gene in Neisseria meningitidis impacts the prediction of coverage of a multicomponent meningococcal serogroup B vaccine. Infect Immun. 2013;81:560–569.
- Rossi R, Granoff DM, Beernink PT. Meningococcal factor H-binding protein vaccines with decreased binding to human complement factor H have enhanced immunogenicity in human factor H transgenic mice. Vaccine. 2013;31:5451–5457.
- Beernink PT, Shaughnessy J, Braga EM, et al. A meningococcal factor H binding protein mutant that eliminates factor H binding enhances protective antibody responses to vaccination. J Immunol Baltim Md 1950. 2011;186:3606–3614.
- Granoff DM, Costa I, Konar M, et al. Binding of complement factor H (FH) decreases protective Anti-FH binding protein antibody responses of Infant Rhesus Macaques immunized with a meningococcal Serogroup B vaccine. J Infect Dis. 2015;212:784–792.
- Granoff DM, Giuntini S, Gowans FA, et al. Enhanced protective antibody to a mutant meningococcal factor H-binding protein with low-factor H binding. JCI Insight. 2016;1:e88907.
- Seib KL, Scarselli M, Comanducci M, et al. Neisseria meningitidis factor H-binding protein fHbp: a key virulence factor and vaccine antigen. Expert Rev Vaccines. 2015 Jun;14(6):841–859.
- Johnson S, Tan L, Van Der Veen S, et al. Design and evaluation of meningococcal vaccines through structure-based modification of host and pathogen molecules. PLoS Pathog. 2012;8:e1002981.
- Esposito V, Musi V, De Chiara C, et al. Structure of the C-terminal domain of Neisseria heparin binding antigen (NHBA), one of the main antigens of a novel vaccine against Neisseria meningitidis. J Biol Chem. 2011;286:41767–41775.
- Lappann M, Otto A, Brauer M, et al. Impact of moderate temperature changes on Neisseria meningitidis adhesive phenotypes and proteome. Infect Immun. 2016 Nov 18;84(12):3484–3495.
- Findlow J, Borrow R, Snape MD, et al. Multicenter, open-label, randomized phase II controlled trial of an investigational recombinant meningococcal serogroup B vaccine with and without outer membrane vesicles, administered in infancy. Clin Infect Dis. 2010;51:1127–1137.
- Bexsero® (Meningococcal Group B Vaccine). Prescribing information [Internet]; [ cited 2016 Jul 19]. Available from: http://www.fda.gov/downloads/BiologicsBloodVaccines/Vaccines/ApprovedProducts/UCM431447.pdf
- Trumenba® (Meningococcal Group B Vaccine) [Internet]; [ cited 2016 Jul 19]. Available from: http://www.fda.gov/downloads/BiologicsBloodVaccines/Vaccines/ApprovedProducts/UCM421139.pdf. Bexsero® (Meningococcal Group B Vaccine). Prescribing information. 2015.
- Borrow R, Carlone GM, Rosenstein N, et al. Neisseria meningitidis group B correlates of protection and assay standardization–international meeting report Emory University, Atlanta, Georgia, United States, 16-17 March 2005. Vaccine. 2006;24:5093–5107.
- Goldschneider I, Gotschlich EC, Artenstein MS. Human immunity to the meningococcus. II. Development of natural immunity. J Exp Med. 1969;129:1327–1348.
- Borrow R, Andrews N, Goldblatt D, et al. Serological basis for use of meningococcal serogroup C conjugate vaccines in the United Kingdom: reevaluation of correlates of protection. Infect Immun. 2001;69:1568–1573.
- Andrews N, Borrow R, Miller E. Validation of serological correlate of protection for meningococcal C conjugate vaccine by using efficacy estimates from postlicensure surveillance in England. Clin Diagn Lab Immunol. 2003;10(5):780–786.
- World Health Organization. Meningococcal vaccines: WHO position paper, November 2011. Wkly Epidemiol Rec. 2011;86:521–539.
- Giuliani MM, Biolchi A, Serruto D, et al. Measuring antigen-specific bactericidal responses to a multicomponent vaccine against serogroup B meningococcus. Vaccine. 2010;28:5023–5030.
- Zlotnick GW, Jones TR, Liberator P, et al. The discovery and development of a novel vaccine to protect against Neisseria meningitidis serogroup B disease. Hum Vaccines Immunother. 2015;11:5–13.
- Donnelly J, Medini D, Boccadifuoco G, et al. Qualitative and quantitative assessment of meningococcal antigens to evaluate the potential strain coverage of protein-based vaccines. Proc Natl Acad Sci USA. 2010;107:19490–19495.
- Plikaytis BD, Stella M, Boccadifuoco G, et al. Interlaboratory standardization of the sandwich enzyme-linked immunosorbent assay designed for MATS, a rapid, reproducible method for estimating the strain coverage of investigational vaccines. Clin Vaccine Immunol. 2012;19:1609–1617.
- Vogel U, Taha M-K, Vazquez JA, et al. Predicted strain coverage of a meningococcal multicomponent vaccine (4CMenB) in Europe: a qualitative and quantitative assessment. Lancet Infect Dis. 2013;13:416–425.
- Tzanakaki G, Hong E, Kesanopoulos K, et al. Diversity of Greek meningococcal serogroup B isolates and estimated coverage of the 4CMenB meningococcal vaccine. BMC Microbiol. 2014;14:111.
- Bettinger JA, Scheifele DW, Halperin SA, et al. Diversity of Canadian meningococcal serogroup B isolates and estimated coverage by an investigational meningococcal serogroup B vaccine (4CMenB). Vaccine. 2013;32:124–130.
- Medini D, Stella M, Wassil J. MATS: global coverage estimates for 4CMenB, a novel multicomponent meningococcal B vaccine. Vaccine. 2015;33:2629–2636.
- Frosi G, Biolchi A, Lo Sapio M, et al. Bactericidal antibody against a representative epidemiological meningococcal serogroup B panel confirms that MATS underestimates 4CMenB vaccine strain coverage. Vaccine. 2013;31:4968–4974.
- Abad R, Biolchi A, Moschioni M, et al. A large portion of meningococcal antigen typing system-negative meningococcal strains from Spain is killed by Sera from adolescents and infants immunized with 4cmenb. Clin Vaccine Immunol. 2015;22:357–360.
- Budroni S, Kleinschmidt A, Boucher P, et al. Pooled-sera hSBA titres predict individual seroprotection in infants and toddlers vaccinated with 4CMenB. Vaccine. 2016;34:2579–2584.
- Snape MD, Dawson T, Oster P, et al. Immunogenicity of two investigational serogroup B meningococcal vaccines in the first year of life: a randomized comparative trial. Pediatr Infect Dis J. 2010;29:e71–e79.
- Toneatto D, Ismaili S, Ypma E, et al. The first use of an investigational multicomponent meningococcal serogroup B vaccine (4CMenB) in humans. Hum Vaccin. 2011;7:646–653.
- McQuaid F, Snape MD, John TM, et al. Persistence of specific bactericidal antibodies at 5 years of age after vaccination against serogroup B meningococcus in infancy and at 40 months. Can Med Assoc J. 2015;187:E215–E223.
- Snape MD, Saroey P, John TM, et al. Persistence of bactericidal antibodies following early infant vaccination with a serogroup B meningococcal vaccine and immunogenicity of a preschool booster dose. Can Med Assoc J. 2013;185:E715–E724.
- McQuaid F, Snape MD, John TM, et al. Persistence of bactericidal antibodies to 5 years of age after immunization with serogroup B meningococcal vaccines at 6, 8, 12 and 40 months of age. Pediatr Infect Dis J. 2014;33:760–766.
- Vesikari T, Esposito S, Prymula R, et al. Immunogenicity and safety of an investigational multicomponent, recombinant, meningococcal serogroup B vaccine (4CMenB) administered concomitantly with routine infant and child vaccinations: results of two randomised trials. Lancet. 2013;381:825–835.
- Prymula R, Esposito S, Zuccotti GV, et al. A phase 2 randomized controlled trial of a multicomponent meningococcal serogroup B vaccine (I). Hum Vaccines Immunother. 2014;10:1993–2004.
- Torres F, Safadi MAP, Martinez A, et al. Immunogenicity and safety of a 2 or 3 dose primary series of meningococcal serogroup b vaccine in infants, and a 2-dose catch-up series in children. Abstract presented at 34th meeting of the European Society for Pediatric Infectious Diseases; 2016 May 10–14; Brighton, UK.
- Safadi MAP, Weckx L, Moreira Junior E, et al. Immunogenicity and safety of concomitant administration of meningococcal serogroup B (4CMenB) and serogroup C (MenC-CRM) vaccines in infants: A phase 3b, randomized controlled trial. Vaccine. 2017 Mar 18;1–8. doi:10.1016/j.vaccine.2017.03.002
- Kimura A, Toneatto D, Kleinschmidt A, et al. Immunogenicity and safety of a multicomponent meningococcal serogroup B vaccine and a quadrivalent meningococcal CRM197 conjugate vaccine against serogroups A, C, W-135, and Y in adults who are at increased risk for occupational exposure to meningococcal isolates. Clin Vaccine Immunol. 2011;18:483–486.
- Santolaya ME, O’Ryan ML, Valenzuela MT, et al. Immunogenicity and tolerability of a multicomponent meningococcal serogroup B (4CMenB) vaccine in healthy adolescents in Chile: a phase 2b/3 randomised, observer-blind, placebo-controlled study. Lancet. 2012;379:617–624.
- Santolaya ME, O’Ryan M, Valenzuela MT, et al. Persistence of antibodies in adolescents 18-24 months after immunization with one, two, or three doses of 4CMenB meningococcal serogroup B vaccine. Hum Vaccines Immunother. 2013;9:2304–2310.
- O’Ryan M, Stoddard J, Toneatto D, et al. A multi-component meningococcal serogroup B vaccine (4CMenB): the clinical development program. Drugs. 2014;74:15–30.
- Perrett KP, McVernon J, Richmond PC, et al. Immune responses to a recombinant, four-component, meningococcal serogroup B vaccine (4CMenB) in adolescents: a phase III, randomized, multicentre, lot-to-lot consistency study. Vaccine. 2015;33:5217–5224.
- Read RC, Baxter D, Chadwick DR, et al. Effect of a quadrivalent meningococcal ACWY glycoconjugate or a serogroup B meningococcal vaccine on meningococcal carriage: an observer-blind, phase 3 randomised clinical trial. Lancet. 2014;384:2123–2131.
- Esposito S, Prymula R, Zuccotti GV, et al. A phase 2 randomized controlled trial of a multicomponent meningococcal serogroup B vaccine, 4CMenB, in infants (II). Hum Vaccines Immunother. 2014;10:2005–2014.
- Gossger N, Snape MD, Yu L-M, et al. Immunogenicity and tolerability of recombinant serogroup B meningococcal vaccine administered with or without routine infant vaccinations according to different immunization schedules: a randomized controlled trial. Jama. 2012;307:573–582.
- Snape MD, Voysey M, Finn A, et al. Persistence of bactericidal antibodies after infant serogroup B meningococcal immunization and booster dose response at 12, 18 or 24 months of age. Pediatr Infect Dis J. 2016;35:e113–e123.
- Vesikari T, Prymula R, Merrall E, et al. Meningococcal serogroup B vaccine (4CMenB): booster dose in previously vaccinated infants and primary vaccination in toddlers and two-year-old children. Vaccine. 2015;33:3850–3858.
- Martinon-Torres F, Bernatowska E, Shcherbina A, et al. The immunogenicity of a serogroup B meningococcal vaccine (4CMenB) in children with complement deficiency, asplenia or splenic dysfunction. Abstract 122. 20th International Pathogenic Neisseria Conference, 2016 Sep 4–9; Manchester, UK.
- Block SL, Szenborn L, Daly W, et al. A comparative evaluation of two investigational meningococcal ABCWY vaccine formulations: results of a phase 2 randomized, controlled trial. Vaccine. 2015;33:2500–2510.
- Ladhani SN, Ramsay M, Borrow R, et al. Enter B and W: two new meningococcal vaccine programmes launched. Arch Dis Child. 2016;101:91–95.
- De Serres G, Gariepy M, Billard M, et al. Initial dose of a multicomponent serogroup B meningococcal vaccine in the saguenay– Lac-Saint-Jean Region, Québec, Canada: an Interim safety surveillance report, 2014.
- Oster P, O’Hallahan J, Aaberge I, et al. Immunogenicity and safety of a strain-specific MenB OMV vaccine delivered to under 5-year olds in New Zealand. Vaccine. 2007;25:3075–3079.
- Births in England and Wales - Office for National Statistics. http://www.ons.gov.uk/peoplepopulationandcommunity/birthsdeathsandmarriages/livebirths/bulletins/birthsummarytablesenglandandwales/2015
- Public Health England. Vaccine cuts cases of meningitis and septicaemia in UK infants - Press releases - GOV.UK. [Internet]; [ cited 2016 Oct 10]. Available from: http://www.ons.gov.uk/peoplepopulationandcommunity/birthsdeathsandmarriages/livebirths/bulletins/birthsummarytablesenglandandwales/2015
- Findlow J. Vaccines for the prevention of meningococcal capsular group B disease: what have we recently learned? Hum Vaccines Immunother. 2016;12:235–238.
- McNamara LA, Shumate AM, Johnsen P, et al. First use of a serogroup B meningococcal vaccine in the US in response to a university outbreak. Pediatrics. 2015;135:798–804.
- Vetter V, Baxter R, Denizer G, et al. Routinely vaccinating adolescents against meningococcus: targeting transmission & disease. Expert Rev Vaccines. 2016;15:641–658.
- Richmond PC, Marshall HS, Nissen MD, et al. Safety, immunogenicity, and tolerability of meningococcal serogroup B bivalent recombinant lipoprotein 2086 vaccine in healthy adolescents: a randomised, single-blind, placebo-controlled, phase 2 trial. Lancet Infect Dis. 2012;12:597–607.
- Marshall HS, Richmond PC, Nissen MD, et al. A phase 2 open-label safety and immunogenicity study of a meningococcal B bivalent rLP2086 vaccine in healthy adults. Vaccine. 2013;31:1569–1575.
- Ostergaard L, Lucksinger GH, Absalon J, et al. A phase 3, randomized, active-controlled study to assess the safety and tolerability of meningococcal serogroup B vaccine bivalent rLP2086 in healthy adolescents and young adults. Vaccine. 2016;34:1465–1471.
- Vesikari T, Wysocki J, Beeslaar J, et al. Immunogenicity, safety, and tolerability of bivalent rLP2086 meningococcal group B vaccine administered concomitantly with diphtheria, tetanus, and acellular pertussis and inactivated poliomyelitis vaccines to healthy adolescents. J Pediatr Infect Dis Soc. 2016;5:180–187.
- Senders S, Bhuyan P, Jiang Q, et al. Immunogenicity, tolerability and safety in adolescents of bivalent rLP2086, a meningococcal serogroup B vaccine, coadministered with quadrivalent human papilloma virus vaccine. Pediatr Infect Dis J. 2016;35:548–554.
- Muse D, Christensen S, Bhuyan P, et al. A phase 2, randomized, active-controlled, observer-blinded study to assess the immunogenicity, tolerability and safety of bivalent rLP2086, a meningococcal serogroup B vaccine, coadministered with tetanus, diphtheria and acellular pertussis vaccine and serogroup A, C, Y and W-135 meningococcal conjugate vaccine in healthy US adolescents. Pediatr Infect Dis J. 2016;35:673–682.
- Ostergaard L, Ward BJ, Beeslaar J et al. Phase 3 trial of immunogenicity of bivalent rLP2086, a meningococcal serogroup B vaccine, in young adults: bactericidal activity against a panel of antigenically diverse strains. Abstract 160 presented at 20th International Pathogenic Neisseria Conference; 2016 Sep 4–9; Manchester, UK.
- Vesikari T, Senders S, Absalon J et al. Phase 3 trial of immunogenicity of bivalent rLP2086, a meningococcal serogroup B vaccine, in adolescents: bactericidal activity against a panel of antigenically diverse strains. Abstract 166 presented at 20th International Pathogenic Neisseria Conference; 2016 Sep 4–9; Manchester, UK.
- Vesikari T, Østergaard L, Diez-Domingo J, et al. Meningococcal serogroup B Bivalent rLP2086 vaccine elicits broad and robust serum bactericidal responses in healthy adolescents. J Pediatr Infect Dis Soc. 2016;5:152–160.
- Marshall HS, Richmond PC, Beeslaar J et al. Persistence of meningococcal serogroup B–specific responses following vaccination with bivalent rLP2086. Abstract presented at 20th International Pathogenic Neisseria Conference; 2016 Sep 4–9. Manchester, UK.
- Martinon-Torres F, Gimenez-Sanchez F, Bernaola-Iturbe E, et al. A randomized, phase 1/2 trial of the safety, tolerability, and immunogenicity of bivalent rLP2086 meningococcal B vaccine in healthy infants. Vaccine. 2014;32:5206–5211.
- Marshall HS, Richmond PC, Nissen MD, et al. Safety and immunogenicity of a meningococcal B bivalent rLP2086 vaccine in healthy toddlers aged 18-36 months: a phase 1 randomized-controlled clinical trial. Pediatr Infect Dis J. 2012;31:1061–1068.
- Brendish NJ, Read RC. Neisseria meningitidis serogroup B bivalent factor H binding protein vaccine. Expert Rev Vaccines. 2015;14:493–503.
- Soeters HM, Whaley M, Wang X et al. Meningococcal carriage evaluation in response to a serogroup B meningococcal disease outbreak and mass vaccination campaign at a college — Rhode Island, 2015. Abstract 040 presented at 20th International Pathogenic Neisseria Conference; 2016 Sep 4–9; Manchester, UK.
- Centers for Disease Control and Prevention. Advisory Committee on Immunization Practices (ACIP). Summary Report February 24, 2016 Atlanta, Georgia [Internet]; [ cited 2016 Oct 14]. Available from: https://www.cdc.gov/vaccines/acip/meetings/downloads/min-archive/min-2016-02.pdf
- Ladhani SN, Campbell H, Parikh SR, et al. The introduction of the meningococcal B (MenB) vaccine (Bexsero®) into the national infant immunisation programme–New challenges for public health. J Infect. 2015;71:611–614.
- Wise J. MenB vaccine programme cuts cases of meningitis and septicaemia in infants. Bmj. 2016;354:i4839.
- Basta NE, Christensen H. 4CMenB vaccine effectiveness: reasons for optimism. Lancet. 2016 Dec 3;388(10061):2719–2721.
- Parikh SR, Andrews N, Beebeejaun K, et al. Effectiveness and impact of a reduced infant schedule of 4CMenB vaccine against group B meningococcal disease in England: a national observational cohort study. Lancet. 2016 Dec 3;388(10061):2775–2782.
- Watson PS, Turner DPJ. Clinical experience with the meningococcal B vaccine, Bexsero(®): prospects for reducing the burden of meningococcal serogroup B disease. Vaccine. 2016;34:875–880.
- Biswas HH, Han GS, Wendorf K, et al. Notes from the field: outbreak of serogroup B meningococcal disease at a university - California, 2016. Morb Mortal Wkly Rep. 2016;65:520–521.
- Basta NE, Mahmoud AAF, Wolfson J, et al. Immunogenicity of a meningococcal B vaccine during a university outbreak. N Engl J Med. 2016;375(3):220–228.
- Rappuoli R. Meningococcal B vaccine during a university outbreak. N Engl J Med. 2016;375:1594–1595.
- MenB and rotavirus vaccines - Ireland’s Health Service [Internet]; [ cited 2016 Aug 18]. Available from: http://www.hse.ie/eng/health/immunisation/news/menbrotavacc.html
- Signorelli C, Chiesa V, Odone A. Meningococcal serogroup B vaccine in Italy: state-of-art, organizational aspects and perspectives. J Prev Med Hyg. 2015;56:E125–E132.
- Folaranmi T, Rubin L, Martin SW, et al. Use of serogroup B meningococcal vaccines in persons aged ≥10 years at increased risk for serogroup B meningococcal disease: recommendations of the Advisory Committee on immunization practices, 2015. Morb Mortal Wkly Rep. 2015;64:608–612.
- MacNeil JR, Rubin L, Folaranmi T, et al. Use of serogroup B meningococcal vaccines in adolescents and young adults: recommendations of the advisory committee on immunization practices, 2015. Morb Mortal Wkly Rep. 2015;64:1171–1176.
- Hong E, Giuliani MM, Deghmane A-E, et al. Could the multicomponent meningococcal serogroup B vaccine (4CMenB) control Neisseria meningitidis capsular group X outbreaks in Africa? Vaccine. 2013;31:1113–1116.
- Abad R, López EL, Debbag R, et al. Serogroup W meningococcal disease: global spread and current affect on the Southern Cone in Latin America. Epidemiol Infect. 2014;142:2461–2470.
- Tomei S, Biolchi A, Brunelli B, et al. Potential coverage of the BEXSERO® MenB vaccine on non-B meningococci. Abstract P30. 19th International Pathogenic Neisseria Conference (IPNC); 2014 Oct 12–17; Asheville, NC.
- Ladhani SN, Giuliani MM, Biolchi A, et al. Effectiveness of meningococcal B vaccine against endemic hypervirulent Neisseria meningitidis W Strain, England. Emerg Infect Dis. 2016;22:309–311.
- Petoussis-Harris H, Paynter J, Morgan J, et al. Effectivness of a group B OMV meningococcal vaccine on gonorrhea in New Zealand - a case control study. Abstract 20th International Pathogenic Neisseria Conference; 2016 Sep 4–9; Manchester, UK.
- Domnich A, Gasparini R, Amicizia D, et al. Meningococcal antigen typing system development and application to the evaluation of effectiveness of meningococcal B vaccine and possible use for other purposes. J Immunol Res. 2015;2015:1–9.