ABSTRACT
Introduction: Pneumococcal diseases (including septicemia, meningitis, pneumonia, and upper respiratory infections) constitute a major public health problem. The World Health Organization recommends pneumococcal conjugate vaccine immunization of young children worldwide.
Areas covered: We reviewed evidence on the effects of the 10-valent pneumococcal non-typeable Haemophilus influenzae protein D conjugate vaccine (PHiD-CV), which is used in childhood immunization programs in over 45 countries or regions. The effectiveness of PHiD-CV against invasive pneumococcal disease (IPD), pneumonia, and acute otitis media was assessed. We also present its effect on pneumococcal nasopharyngeal carriage (NPC) and indirect effects (herd protection) among unvaccinated individuals.
Expert commentary: Results from randomized, double-blind trials and post-marketing studies in various countries provide evidence of the protective efficacy, effectiveness, and impact of PHiD-CV against pneumococcal diseases. Data from different geographic locations also show reductions in NPC of vaccine pneumococcal serotypes, laying the foundation for indirect protection against pneumococcal disease. In countries where PHiD-CV is included in childhood immunization programs, there are signs of herd protection for vaccine serotypes among unvaccinated individuals. Although increases in non-vaccine serotype IPD and NPC rates were observed, there was an overall reduction of pneumococcal disease.
1. Introduction
The World Health Organization (WHO) recommends pneumococcal conjugate vaccine (PCV) immunization of young children in all countries [Citation1]. Since 2009, the seven-valent pneumococcal CRM-conjugate vaccine (7vCRM; Prevenar/Prevnar, Pfizer Inc.) has been replaced by the 10-valent pneumococcal non-typeable Haemophilus influenzae protein D conjugate vaccine (PHiD-CV; Synflorix, GSK) or 13-valent pneumococcal CRM-conjugate vaccine (PCV13; Prevenar13/Prevnar13, Pfizer Inc.) in almost all national childhood immunization programs that include PCV immunization.
PHiD-CV is licensed in more than 135 countries and used in immunization programs in more than 45 countries or regions. Although prescribing information differs by country, PHiD-CV can be indicated for the active immunization of infants and children up to 5 years of age against invasive pneumococcal disease (IPD), pneumonia, and acute otitis media (AOM) caused by vaccine pneumococcal serotypes and vaccine-related serotype 19A as well as AOM due to non-typeable H. influenzae (NTHi). PHiD-CV contains pneumococcal polysaccharides of serotypes 1, 4, 5, 6B, 7F, 9V, 14, and 23F conjugated to carrier NTHi protein D, serotype 18C conjugated to tetanus toxoid, and serotype 19F conjugated to diphtheria toxoid [Citation2]. The immunogenicity of PHiD-CV, including protective functional immune responses and immunologic memory, and its safety profile are well established [Citation1,Citation3–Citation5].
We reviewed clinical evidence of the protective efficacy and effectiveness of PHiD-CV against pneumococcal diseases that has accumulated since first approval in 2008 from a broad range of clinical trial and post-marketing settings. We also examined the effect of PHiD-CV on pneumococcal nasopharyngeal carriage (NPC) and its indirect effects among unvaccinated individuals (herd protection). The effect of PHiD-CV on the carriage of NTHi and on AOM due to NTHi has been reviewed recently [Citation6], and is therefore not discussed here. Rather than a systematic review, this article is an expert commentary on clinical efficacy and effectiveness data on PHiD-CV published in the last 10 years. A ‘Focus on the Patient’ section () contextualizes, highlights, and discusses the impact of the primary findings of our review.
2. Studies that evaluated the clinical effectiveness of PHiD-CV
Evidence on the clinical efficacy/effectiveness of PHiD-CV comes from both randomized controlled trials (RCTs) () and post-marketing observational studies (). While RCTs generally have high internal validity by controlling for confounding variables, post-marketing effectiveness and impact studies are often suitable for long-term evaluations in ‘real-life’ settings with high vaccination coverage. With adequate power, post-marketing studies can be the most feasible way to quantify population effects and long-term impact, including indirect effects [Citation7]. However, the strengths and weaknesses of particular study designs must be taken into account when comparing and interpreting results.
Table 1. Characteristics of clinical trials that evaluated the impact of PHiD-CV.
Table 2. Characteristics of post-marketing effectiveness and impact studies of PHiD-CV. Effectiveness studies compared vaccinated and unvaccinated individuals exposed to the same PHiD-CV vaccination program while impact studies compared the same population before and after the introduction of PHiD-CV in the national immunization program.
Three double-blind RCTs have been conducted with clinical disease outcomes: the Finnish Invasive Pneumococcal disease (FinIP) trial [Citation10], AOM and NPC study 053 nested within FinIP [Citation11], and the Clinical Otitis Media and PneumoniA Study (COMPAS) in Latin America [Citation12] (). The cluster-randomized FinIP field trial was designed to evaluate vaccine effectiveness rather than efficacy. Over 47,000 children aged 2–18 months were enrolled to receive PHiD-CV or hepatitis vaccines as control in 3 + 1 (three primary doses and one booster dose) or 2 + 1 infant schedules, or in 2 + 1 or 2-dose catch-up schedules [Citation10]. In COMPAS, an efficacy study which was conducted in Argentina, Panama, and Colombia, approximately 24,000 infants received PHiD-CV or hepatitis control vaccine in a 3 + 1 regimen [Citation12]. Several other clinical trials assessed impact on pneumococcal NPC [Citation13–Citation15] ().
Figure 2. Countries in which studies were conducted to assess the efficacy or effectiveness of PHiD-CV against invasive pneumococcal disease (IPD), pneumonia, and acute otitis media (AOM).
aAntimicrobial purchases and tympanostomy tube placement in Finland; AOM-related diagnoses and surgical procedures in Sweden; antimicrobial purchases in Iceland.bStudy 053 was not powered to evaluate AOM end points and the assessment was affected by a randomization error.COMPAS: Clinical Otitis Media and PneumoniA Study; FinIP: Finnish Invasive Pneumococcal disease trial; 7vCRM: 7-valent pneumococcal CRM-conjugate vaccine; 2 + 1/3 + 1/3 + 0, PHiD-CV infant vaccination schedules (two or three primary doses with or without booster dose).
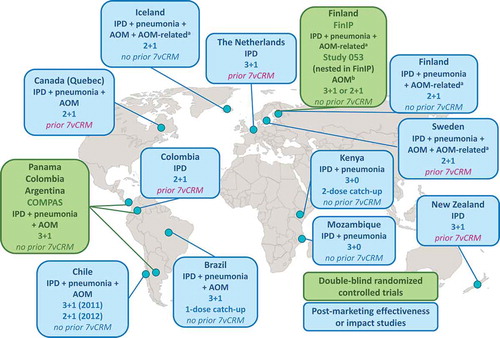
Post-marketing effectiveness or impact studies of PHiD-CV have been carried out in high-, middle-, and low-income settings (, ). In all countries, PHiD-CV was the first PCV included in the national immunization program, apart from in the Netherlands, Sweden, Colombia, Canada (Quebec), Australia (Northern Territory), and New Zealand, where 7vCRM was used previously.
3. Effectiveness against pneumococcal diseases in vaccinated children
3.1. Culture-confirmed IPD
The effectiveness and efficacy of PHiD-CV against culture-confirmed IPD (i.e. positive for Streptococcus pneumoniae in culture of any normally sterile body fluid) was investigated in the FinIP and COMPAS clinical trials (). The primary and secondary objectives of the FinIP trial were to demonstrate vaccine effectiveness against culture-confirmed IPD due to vaccine serotypes (VT IPD) in children who received at least one PHiD-CV dose before 7 months of age in a 3 + 1 or 2 + 1 schedule [Citation10]. In the intent-to-treat analysis, no cases of VT IPD occurred after the first vaccine dose in the PHiD-CV 3 + 1 infant group and one case occurred 12 days after the first dose in the 2 + 1 group compared with 12 cases in the control group. Vaccine effectiveness against VT IPD was thus 100% (95% confidence interval [CI]: 83, 100) and 92% (95% CI: 58, 100) with the 3 + 1 and 2 + 1 infant schedules, respectively. For culture-confirmed IPD irrespective of serotype, vaccine effectiveness was 93% (95% CI: 75, 99) with the 3 + 1 and 2 + 1 infant schedules combined. In COMPAS, vaccine efficacy against VT IPD with a 3 + 1 schedule was also 100% (95% CI: 77, 100) () [Citation12]. No cases of VT IPD were reported in the PHiD-CV group compared with 18 cases in the control group, while culture-confirmed IPD irrespective of serotype was reported in seven children in the PHiD-CV group versus 21 in the control group (vaccine efficacy 67%, 95% CI: 22, 86) during mean follow-up of 33 months.
Table 3. Efficacy and effectiveness of PHiD-CV against invasive pneumococcal disease (IPD) in vaccinated or vaccine-eligible infants and young children.
This RCT evidence is augmented by data from post-marketing effectiveness and impact studies conducted in Finland, the Netherlands, Iceland, Sweden, Brazil, Canada, Kenya, Mozambique, and New Zealand (). The impact of PHiD-CV against IPD has also been estimated in Brazil, Chile, and Colombia using data collected by the Latin American laboratory network for passive surveillance of bacterial pneumonia and meningitis pathogens (SIREVA II) [Citation16]. While these data provide useful information on serotype distribution during particular periods of time, caution is advised in the analysis of the impact of vaccination since SIREVA II is a voluntary system and completeness of reporting varies among countries [Citation17]. Analyses of the laboratory-based data are also limited by a lack of demographic and clinical information.
In Finland, PHiD-CV 2 + 1 vaccination was added to the infant immunization program in September 2010. Nationwide, population-based IPD rates among vaccine-eligible children (born up to 6 years after PHiD-CV introduction and regardless of whether they had received PHiD-CV or not) were compared to those from historical season- and age-matched cohorts before its introduction [Citation18,Citation19]. Information on IPD cases was retrieved from the national infectious diseases register. This showed a 94% (95% CI: 92, 96) reduction in VT IPD while the overall IPD rate was reduced by 79% (95% CI: 74, 83) among vaccine-eligible children aged 3–78 months [Citation19]. Other studies of the national vaccination program in Finland showed vaccine effectiveness of 98% (95% CI: 72, 100) against VT IPD during 3 years of follow-up [Citation20], a 46% decline in cases of pneumococcal meningitis between 1995–1999 and 2010–2014 [Citation21], and a 76% decrease in invasive pneumococcal isolate numbers between 2009 and 2014 [Citation22] in young children.
PHiD-CV was introduced to the immunization program in Brazil between March and September 2010 as a 3 + 1 infant schedule or single catch-up dose at 12–23 months of age. A matched case-control study showed PHiD-CV effectiveness of 84% (95% CI: 66, 92) against VT IPD [Citation23]. In a subsequent indirect cohort analysis, in which the vaccination status of cases with VT IPD was compared with that of children with non-vaccine serotype (NVT) disease [Citation24], the adjusted effectiveness of PHiD-CV against VT IPD was 73% (95% CI: 44, 87) among children who received at least one vaccine dose. This is consistent with an analysis of Brazilian surveillance data, which found VT IPD decreased by 62% in children aged 12–23 months between 2008–2009 and 2011–2013 [Citation25]. Moreover, a systematic review of studies of pneumococcal meningitis in children conducted in Brazil showed vaccine effectiveness varied from 13% to 88% [Citation26]. Among children younger than 5 years, one study showed a reduction in VT IPD of 83% for meningitis and 87% for non-meningitis between 2005–2009 and 2014–2015 [Citation27].
PHiD-CV was also the first PCV in immunization programs in Iceland (2 + 1 infant schedule) [Citation28,Citation29], Kenya (3 + 0 infant and 2-dose catch-up in children under 5 years old) [Citation30], and Mozambique (3 + 0 infant schedule) [Citation31,Citation32] (). Surveillance of cases in young children pre- and post-introduction showed decreases in both VT IPD (87% in Iceland, 96% in Kenya, 96% in Mozambique) and overall IPD (82% and 93% in Iceland, 78% in Kenya, 73% in Mozambique) ().
In Quebec, the Netherlands, and New Zealand, PHiD-CV was preceded by 7vCRM and (in Quebec and New Zealand) eventually replaced by PCV13, limiting the interpretation of surveillance data [Citation33–Citation38]. In Sweden, counties could switch from 7vCRM to either PHiD-CV or PCV13 [Citation39]. There are indications however that, in each setting, PHiD-CV was effective in reducing VT IPD and overall IPD compared to either historical surveillance or incidence data from the 7vCRM time period (), although we note that the authors of the Swedish study are in the process of reanalyzing their data following receipt of additional information [Citation40].
PCVs may also confer protection against IPD caused by vaccine-related serotypes, particularly 6A and 19A related to serotypes 6B and 19F in 7vCRM and PHiD-CV. While the immunogenicity of PHiD-CV against 6A and 19A is not as robust as against the 10 vaccine serotypes, immunoglobulin G (IgG) and functional antibody responses against serotype 6A are similar and against 19A are higher when comparing PHiD-CV to 7vCRM, possibly because of differences in 19F conjugation chemistry [Citation2]. In comparison with PCV13, which contains serotype 19A, the IgG antibody response with PHiD-CV is lower against serotype 19A, although in studies including both PCV13 and PHiD-CV, 46–94% of infants administered three or four doses of PHiD-CV had antibody concentrations of at least 0.2 µg/mL against serotype 19A [Citation41,Citation42]. While cross-protection against IPD caused by serotype 6A was reported in the 7vCRM era [Citation43], and recently following PHiD-CV vaccination [Citation19], 19A IPD was not prevented by 7vCRM. Antibiotic resistance, clonal changes, and capsular switching might also have contributed to the emergence of 19A as a major invasive pneumococcal serotype [Citation44]. In the FinIP and COMPAS trials, too few IPD cases were caused by individual vaccine-related serotypes (in FinIP, two in a control group; in COMPAS, two in PHiD-CV group and one in control group) to allow conclusions to be drawn regarding serotype-specific vaccine effectiveness or efficacy [Citation10,Citation12]. In the case-control study in Brazil, the point estimate for effectiveness against IPD caused by serotype 19A (82%, 95% CI: 11, 96) in children under 5 years old was similar to that reported for VT IPD (84%, 95% CI: 66, 92) [Citation23]. In the related indirect cohort analysis of VT IPD versus IPD due to NVT (NVT IPD), effectiveness among children who received at least one PHiD-CV dose was 71% (95% CI: 17, 90) against IPD caused by serotype 19A and 51% (95% CI: −52, 84) against 6A IPD [Citation24]. The population-based study in Finland showed a 26% (95% CI: −13, 51) reduction of 19A IPD and 95% (95% CI: 75, 100) reduction of 6A IPD in vaccine-eligible children aged 6 years or younger [Citation19] (). The surveillance follow-up from Finland suggests protection against serotype 19A may be age-dependent, with protection evident up to 24 months of age only (). However, the limited number of 19A or 6A IPD cases included in each analysis must be taken into account when interpreting these data as well as broad CIs associated with point estimates of reductions.
In Quebec and New Zealand, increases in 19A IPD were observed during the 7vCRM era followed by a decline, after PHiD-CV was introduced, in children under 5 years old in Quebec [Citation33,Citation34], and in children under 2 years old in New Zealand [Citation35] (). In the Netherlands, incidences of 19A IPD in children younger than 2 years started to decrease at the end of the 7vCRM time period and this decline continued with PHiD-CV introduction [Citation36]. Among these countries, 6A IPD cases were reported in New Zealand only but there were too few to draw any conclusions [Citation35].
These decreases in invasive disease caused by vaccine-related serotype 19A in children immunized with PHiD-CV in post-marketing observational studies constituted evidence supporting the addition of protection against pneumococcal diseases caused by serotype 19A to the Synflorix label by European and other regulatory authorities [Citation45].
3.2. Clinically suspected IPD
Culture-confirmed IPD is a highly specific end point that does not capture all IPD cases for various reasons, most often because of suboptimal sensitivity of laboratory- and hospital-based detection that can be reduced further by prior antimicrobial treatment. The effect of PHiD-CV against an alternative case definition, clinically suspected IPD, has been investigated in Finland by estimating the effect of PHiD-CV against non-laboratory-confirmed IPD or unspecified sepsis. One of the assessments was part of the FinIP trial [Citation46]; the other had a vaccine-probe study design and assessed population-based rates of suspected IPD among vaccine-eligible children during the first 3 years after the vaccine was introduced [Citation47] (). In both studies, all inpatient and outpatient care notifications compatible with IPD or unspecified sepsis were collected from the national hospital discharge register. Laboratory-confirmed IPD cases (i.e. culture-confirmed or positive for S. pneumoniae in nucleic acid or antigen detection tests) were excluded.
In the FinIP trial assessment, vaccine effectiveness against patient-file verified, non-laboratory-confirmed IPD for 3 + 1 and 2 + 1 schedule groups combined during intent-to-treat follow-up was 71% (95% CI: 52, 83) and the absolute rate reduction (i.e. the vaccine-preventable incidence) was 142 (95% CI: 91, 191) episodes per 100,000 person-years [Citation46]. For the more sensitive case definition of register-based non-laboratory-confirmed IPD or unspecified sepsis, vaccine effectiveness was 50% (95% CI: 32, 63) and the absolute rate reduction was 207 (95% CI: 127, 286) episodes per 100,000 person-years. Therefore, although the effectiveness of PHiD-CV against culture-confirmed IPD was higher (93% [95% CI: 75, 99] for 3 + 1 and 2 + 1 groups combined), the vaccine-preventable incidence was much lower (61 episodes per 100,000 person-years) [Citation10] than with the suspected IPD case definition.
In the population-based study, there were 3124 episodes of register-based non-laboratory-confirmed IPD or unspecified sepsis among children aged 3–42 months [Citation47]. The relative rate reduction due to PHiD-CV immunization was 34% (95% CI: 29, 39) and the absolute reduction was 122 (95% CI: 100, 144) episodes per 100,000 person-years. This was more than twofold higher than for culture-confirmed IPD (50 per 100,000 person-years) in the same study population [Citation18]. Similar results have been reported by another study group in Finland [Citation48].
The investigators in Finland highlighted poor sensitivity of the blood culture test, which was estimated to be 27% [Citation46], as the main reason for the additional disease burden detected through hospital discharge register data. A considerable proportion of true IPD cases that were diagnosed by hospital pediatricians were negative in blood culture [Citation46,Citation47]. These first reports of PCV effectiveness against clinically suspected IPD and its impact during an infant immunization program therefore imply that the public health value of PCVs against IPD has been underestimated.
3.3. Pneumonia
Evidence of the efficacy and effectiveness of PHiD-CV against all-cause pneumonia in the first years of life came from the COMPAS and FinIP trials (). In previous efficacy trials, PCVs prevented more community-acquired pneumonia (CAP) cases than those captured by the consolidated CAP case definition proposed by the WHO [Citation49]. The COMPAS investigators therefore introduced an expanded case definition as the primary end point to capture consolidated CAP and all X-ray cases with non-consolidated infiltrates and elevated C-reactive protein levels. The primary objective of COMPAS was to demonstrate vaccine efficacy against likely bacterial CAP (B-CAP), defined as radiologically confirmed CAP with alveolar consolidation or pleural effusion on the chest X-ray, or non-alveolar infiltrates and C-reactive protein ≥40 µg/mL [Citation12]. After a mean follow-up of 26 months for the intent-to-treat analysis, vaccine efficacy was 18% (95% CI: 6, 29) against B-CAP and 23% (95% CI: 9, 36) against WHO-defined consolidated CAP. In the per-protocol analysis (primary objective results), after a mean follow-up of 23 months, vaccine efficacy was 22% (95% CI: 8, 34) and 26% (95% CI: 8, 40), respectively.
Table 4. Efficacy and effectiveness of PHiD-CV against pneumonia in vaccinated or vaccine-eligible infants and young children in countries where PHiD-CV was the first PCV introduced in the national immunization program.
Vaccine efficacy against WHO-defined CAP with alveolar consolidation in COMPAS was consistent with the 21–37% efficacy reported in per-protocol cohorts in other PCV studies [Citation50–Citation54]. As in previous PCV trials, point estimates of efficacy were lower against pneumonia than against IPD mostly due to lower specificity of the pneumonia end point since its etiology involves a spectrum of bacterial and viral pathogens [Citation55].
In the FinIP trial, vaccine effectiveness was calculated against different case definitions of pneumonia [Citation56,Citation57]. Among those with hospital-diagnosed pneumonia with WHO-defined consolidation, vaccine effectiveness was 47% (95% CI: 24, 64) following the 3 + 1 schedule and 43% (95% CI: 19, 61) following the 2 + 1 schedule [Citation57]. Effectiveness of PHiD-CV against radiologically confirmed pneumonia (consolidated or non-consolidated pattern) was 28% (95% CI: −0.5, 48) and 29% (95% CI: 0.6, 49), for the respective schedules [Citation57].
Effectiveness against pneumonia in the FinIP trial was also estimated using hospital discharge register data only (International Classification of Diseases [ICD-10] diagnoses compatible with pneumonia) [Citation56]. With 3 + 1 and 2 + 1 schedules starting before 7 months of age, the effectiveness of PHiD-CV against any hospital-diagnosed pneumonia was 25% (95% CI: 3, 43) and 28% (95% CI: 6, 45), respectively, with similar effectiveness against hospital-treated primary pneumonia, defined as overnight hospitalization and a final primary diagnosis compatible with pneumonia. These register-based case definitions captured a much higher number of cases preventable by the vaccine than definitions with radiological confirmation according to WHO definitions.
The effectiveness of PHiD-CV against pneumonia in RCTs is corroborated by evidence from post-marketing studies conducted in countries with no prior 7vCRM use (Finland, Iceland, Brazil, Chile, Kenya, and Mozambique) () [Citation58–Citation69]. In a nationwide study in Finland, PHiD-CV vaccination led to a relative reduction in the rate of hospital-treated primary pneumonia of 23% (95% CI: 18, 28) among vaccine-eligible children [Citation62]. In Goiânia, Brazil, population-based surveillance studies conducted in 2-year periods before and after PHiD-CV introduction showed a relative rate reduction for X-ray confirmed pneumonia of 23% (95% CI: 22, 24) among hospitalized children younger than 36 months, with the highest reduction in children younger than 24 months (25%, 95% CI: 25, 26) [Citation64]. A study of national hospital data in Brazil found that admission rates for pneumonia among children aged less than 4 years fluctuated before 2010 but decreased steadily in the 2 years after PHiD-CV introduction, decreasing by 13% between 2002–2009 and 2011–2012 [Citation63]. In a nationwide case-control study of Chilean infants, the protective effect of PHiD-CV on all-cause pneumonia hospitalizations and deaths increased when more specific case definitions were used; vaccine effectiveness was 11% (95% CI: 9, 14) when all pneumonia hospitalizations and deaths were considered and 21% (95% CI: 17, 24) when viral pneumonia was excluded [Citation59].
In Quebec, where 7vCRM was used previously, a study of the frequency of hospital admission for lower respiratory tract infection (mainly bronchiolitis and pneumonia) among children younger than 2 years showed a slightly lower hospitalization risk in the PHiD-CV time period as compared with the 7vCRM time period, although the difference was not significant [Citation70]. In Sweden, an analysis of pneumonia hospitalizations during the pre-7vCRM era and following PHiD-CV introduction showed a reduction in hospitalization risk of 21% (incidence rate ratio 0.79, 95% CI: 0.68, 0.93) [Citation71].
3.4. Acute otitis media
Evidence for the protective effect of PHiD-CV against AOM has been generated in the RCTs and in countries that included PHiD-CV in the national immunization program. The efficacy and effectiveness of PHiD-CV against AOM or AOM-related end points were investigated in COMPAS (Panama only), FinIP, and study 053 (). In COMPAS, in the intent-to-treat analysis, the efficacy of PHiD-CV was 19% (95% CI: 4, 31) against first episodes of clinically confirmed AOM (C-AOM) and 70% (95% CI: 30, 87) against C-AOM caused by vaccine pneumococcal serotypes [Citation12]. Further analysis of the level of protection showed positive point estimates for efficacy against C-AOM in children younger than 24 months (24%, 95% CI: 9, 37) and against moderate (18%, 95% CI: −6, 36) and severe (33%, 95% CI: −21, 62) C-AOM episodes [Citation72]. As with pneumonia, point estimates of efficacy against AOM were lower than against IPD, probably due to its complex etiology and because it is a mucosal disease [Citation73].
Table 5. Efficacy and effectiveness of PHiD-CV against acute otitis media (AOM) or AOM-related end points in vaccinated or vaccine-eligible infants and young children in countries where PHiD-CV was the first PCV introduced in the national immunization program.
In the FinIP trial, the effectiveness of PHiD-CV was assessed against outpatient prescriptions of antimicrobial drugs recommended for AOM by national treatment guidelines [Citation74] and against tympanostomy tube placement (TTP) [Citation75]. The children had been randomized to receive PHiD-CV in two-thirds of clusters or control hepatitis vaccine in one-third of clusters and mean follow-up for the infant cohort was 24 months. Over 90% of all outpatient antimicrobials prescribed were those recommended for AOM [Citation74]. The average number of purchases per person-year was 1.43 in the PHiD-CV clusters and 1.55 in the control combined 3 + 1 and 2 + 1 groups. Vaccine effectiveness was 8% (95% CI: 1, 14) against outpatient purchases of antimicrobial drugs recommended for AOM. From this, it was estimated that more than 12,000 antimicrobial purchases and pharmacy costs of over €200,000 could be averted every year with PHiD-CV immunization in children younger than 24 months in Finland. In the analysis of TTPs, 4369 were reported in over 30,500 children and vaccine effectiveness was 13% (95% CI: −2, 26) for the combined 3 + 1 and 2 + 1 group, with similar nonsignificant estimates when each schedule was evaluated separately [Citation75]. Interestingly, examination of data from other PCV trials shows an inverse relationship between vaccine effectiveness and incidence of TTP [Citation76–Citation78]; with more liberal indications for the TTP procedure, vaccine effectiveness tends to be lower. The 14% (95% CI: −4, 29) decline in the FinIP study for the 3 + 1 group compares favorably with the 4% (95% CI: −19, 23) reduction for the 7vCRM vaccine in the Finnish Otitis Media trial, which was the study that reported the highest incidence of TTP [Citation75,Citation77].
In study 053, nested within FinIP, vaccine effectiveness was assessed against AOM diagnosed by physicians and subsequently reported by parents to study personnel in children who received the 3 + 1 or 2 + 1 infant schedules [Citation11]. There was a trend toward a reduced number of infants with at least one parentally reported AOM episode and toward a reduction in all AOM episodes with both schedules, although the reliance on parental reporting limits interpretation of these results.
Post-marketing data from Finland, Sweden, Iceland, Chile, and Brazil suggest PHiD-CV immunization has an impact on the burden of AOM in young children (). In Finland, population-based evaluation of the impact of PHiD-CV in a 2 + 1 schedule showed a relative reduction in TTP rate of 15% (95% CI: 13, 17) and an absolute rate reduction of 860 per 100,000 person-years [Citation79]. Moreover, an analysis of the incidence of outpatient purchases of antimicrobials showed a sharp decrease after PHiD-CV introduction, with a relative rate reduction of 18% (95% CI: 17, 18) and absolute rate reduction of 20,000 per 100,000 person-years [Citation79]. An analysis of young children in Sweden found significant reductions in incidences of AOM-related diagnoses and surgical procedures when comparing 2-year periods before and after PHiD-CV or PCV13 introduction [Citation80]. The incidence of AOM decreased among outpatients by 39% (95% CI: 39, 40) and among hospital admissions by 42% (95% CI: 36, 46), while ventilation tube insertions decreased by 18% (95% CI: 15, 20) and myringotomies by 15% (95% CI: 4, 25). The decline in outpatient AOM and ventilation tube insertions was more pronounced in counties that used PHiD-CV compared to those using PCV13, with risk ratios (PHiD-CV:PCV13) in 2014 of 0.75 (95% CI: 0.73, 0.78) for AOM ambulatory care and 0.78 (95% CI: 0.72, 0.83) for ventilation tube insertions. Another analysis of otitis media in Sweden also found declines in incidence rates in children aged 5 years or younger in two Swedish counties following either PHiD-CV or PCV13 introduction [Citation81]. Vaccine effectiveness was estimated as 10% (95% CI: 4, 15) for PHiD-CV and 2% (95% CI: −3, 8) for PCV13.
In Iceland, the number of primary care visits for AOM among children aged under 3 years was assessed, comparing vaccine-eligible to vaccine-ineligible children [Citation82]. Hazard ratio estimates showed a 22% (95% CI: 12, 31) impact on AOM, with crude incidence rates of 38 and 44 per 100 person-years in vaccine-eligible and vaccine-ineligible children, respectively. Another study in Iceland reported vaccine effectiveness of 20% (95% CI: 15, 25) against prescriptions of antimicrobials used in the treatment of AOM among children younger than 3 years, when vaccine-eligible cohorts were compared with ineligible children [Citation83]. A nested case-control study in Chile estimated the frequency of AOM among children younger than 24 months in the 4 years before and after PHiD-CV introduction [Citation84]. From 1907 emergency department cases with AOM compared to controls with any other discharge diagnosis, it was shown that PHiD-CV decreased the risk of developing AOM in this age group, with an odds ratio of 0.66 (95% CI: 0.60, 0.72). In Goiânia, the impact on outpatient visits for otitis media was calculated from records of children aged 2–23 months covered by the public health-care system between August 2008 and July 2015 [Citation85]. The monthly rate of otitis media visits fell by 43% (95% CI: 41, 45) after PHiD-CV introduction, taking into account rates of outpatient visits due to other causes. In contrast, in a study conducted in Quebec, the introduction of PHiD-CV after 7vCRM did not lead to reduced physicians’ claims for otitis media in comparison to the 7vCRM era [Citation86].
4. Impact on NPC
Transmission of S. pneumoniae occurs via respiratory secretions mainly from asymptomatic NPC of pneumococci. Carriage is particularly common in young children [Citation87,Citation88] and studies of close contacts of cases with pneumococcal disease (mostly of mild mucosal infections in children) suggest that exposure to and colonization with pneumococci are predisposing events to disease [Citation89]. A systematic review of PCV studies (mostly of seven- or nine-valent PCVs) concluded that carriage of vaccine serotypes was reduced following PCV immunization [Citation90], although replacement with NVTs may mitigate the impact of PCVs on the nasopharyngeal bacterial community [Citation91]. Data on the impact of PHiD-CV on pneumococcal NPC in children are available from four randomized controlled studies (COMPAS, study 053, study 027 in the Netherlands, and study 070 in Kenya) [Citation11,Citation13,Citation15,Citation72], a non-randomized open study in the Czech Republic (study 014) [Citation14], and population-based studies in Kenya, Brazil, and Iceland [Citation92–Citation96] (Supplementary Table 1).
In COMPAS, study 053, and study 014, which compared PHiD-CV to hepatitis or quadrivalent meningococcal vaccine controls in 3 + 1 or 2 + 1 schedules, PHiD-CV vaccination was followed by a consistent reduction in vaccine serotype NPC (VT NPC) (). In COMPAS and study 053, which were both randomized, estimates of vaccine efficacy varied but tended to be highest after the booster dose [Citation11,Citation72]. In all three studies, trends for increased NVT carriage were seen at some time points post-booster [Citation11,Citation14,Citation72]. In contrast to observations with PCVs of lower serotype valency [Citation89], increases in NVT carriage were generally smaller than decreases in VT NPC at the same time points, leading to an overall reduction in pneumococcal NPC at most time points versus control (–)). Study 027 was conducted in the Netherlands, where 7vCRM had been introduced in the national immunization program 2 years before the study started [Citation15]. No differences were observed in VT, NVT, or overall pneumococcal NPC when comparing PHiD-CV to 7vCRM vaccination ()). In the RCT conducted in Kenya (study 070), children aged 12–59 months received two doses of PHiD-CV (60 or 180 days apart) or two doses of hepatitis A control vaccine [Citation13]. VT NPC began to drop within 1 month of the first PHiD-CV dose and the reduction was statistically significant 60 days after vaccination (16% VT NPC in both PHiD-CV groups versus 30% in control, p < 0.001). At day 180, vaccine efficacy against VT NPC was 40% (95% CI: 4, 62) for children who had received two doses of PHiD-CV. Carriage of NVT pneumococci was not significantly different in PHiD-CV recipients compared to controls at any time point. In the largest RCT assessing carriage (study 053), there was a significant reduction in the carriage of vaccine-related serotype 19A in Finnish children immunized with the 3 + 1 schedule and a less pronounced nonsignificant reduction in the 2 + 1 schedule group [Citation11], while no consistent decline or increase in serotype 19A NPC was observed in the three smaller RCTs. However, none of these studies had statistical power for serotype-specific assessments.
Figure 3. Impact of PHiD-CV vaccination on nasopharyngeal carriage of vaccine pneumococcal serotypes (VT) and non-vaccine serotypes (NVT) in (a) Panama (COMPAS) [Citation72], (b) Finland (study 053: AOM/carriage study nested in FinIP trial) [Citation11], (c) the Czech Republic (study 014: non-randomized study with age-matched controls) [Citation14], and (d) the Netherlands (study 027: randomized study of children immunized with PHiD-CV or 7vCRM) [Citation15].
*Pneumococcal serotypes included in PHiD-CV (serotypes 1, 4, 5, 6B, 7F, 9V, 14, 18C, 19F, and 23F).
![Figure 3. Impact of PHiD-CV vaccination on nasopharyngeal carriage of vaccine pneumococcal serotypes (VT) and non-vaccine serotypes (NVT) in (a) Panama (COMPAS) [Citation72], (b) Finland (study 053: AOM/carriage study nested in FinIP trial) [Citation11], (c) the Czech Republic (study 014: non-randomized study with age-matched controls) [Citation14], and (d) the Netherlands (study 027: randomized study of children immunized with PHiD-CV or 7vCRM) [Citation15].*Pneumococcal serotypes included in PHiD-CV (serotypes 1, 4, 5, 6B, 7F, 9V, 14, 18C, 19F, and 23F).](/cms/asset/6fabc8df-5426-4f5b-ae19-96c456019880/ierv_a_1516551_f0003_oc.jpg)
A cross-sectional survey in Goiânia revealed a trend for reduced pneumococcal NPC after PHiD-CV introduction [Citation92]. After less than a year, there was a significant reduction in VT NPC, with vaccine effectiveness of 36% (95% CI: 4, 57) among children who received two priming doses and 44% (95% CI: 14, 64) for those who received three priming doses in infancy. Other cross-sectional studies in Brazil reported effectiveness against VT carriage of 97% (95% CI: 89, 99), with no significant change in carriage of serotypes 6A and 19A [Citation93], and near elimination of VT carriage after 4 years of PHiD-CV vaccination, from approximately 45% to 2.5% carriage [Citation96,Citation97]. In Iceland, vaccine effectiveness for acquisition of VT NPC among children younger than 4 years was 94% (95% CI: 91, 96) and there was a reduction of 33% (95% CI: 1, 55) for serotypes 6A and 19A combined [Citation94].
Evidence is starting to emerge suggesting an indirect effect of PHiD-CV on VT NPC among unvaccinated individuals. A satellite study evaluated indirect effectiveness among older siblings of children who were vaccinated in the FinIP trial [Citation98]. Among 927 unvaccinated siblings aged 3–7 years in the PHiD-CV clusters, indirect vaccine effectiveness against VT NPC was 29% (95% CI: 6, 47) when compared to 518 unvaccinated siblings in the control clusters. Other evidence suggestive of reduced transmission of vaccine serotype pneumococci within the population comes from cross-sectional surveys conducted in Iceland [Citation94] and Kilifi, Kenya [Citation95]. In Iceland, nasopharyngeal samples taken from unvaccinated children aged 3–6 years were compared before and after PHiD-CV introduction [Citation94]. Vaccine effectiveness of 56% (95% CI: 44, 65) was reported against VT NPC acquisition, 44% (95% CI: 12, 64) against vaccine-related serotype 6A, and a nonsignificant reduction for 19A (17%, 95% CI: −28%, 46%). In the Kenyan study, carriage was assessed in around 500 individuals per year in the 2 years before and after PHiD-CV introduction [Citation95]. At least one PHiD-CV dose had been received by 69% (95% CI: 62, 76) of children aged less than 5 years in 2011 and by 79% (95% CI: 62, 76) in 2012. There was a 64% (95% CI: 49, 74) reduction in VT NPC among children younger than 5 years and 66% (95% CI: 38, 82) reduction among older individuals. This suggested a two-thirds reduction in the prevalence of VT NPC, not only in children eligible for vaccination but also in ineligible older individuals. There were also modest reductions (13% and 15%) in the carriage of pneumococci overall in both vaccine-eligible and non-eligible age groups. Among children younger than 5 years, there was no effect on carriage of vaccine-related serotypes 19A and 6A, although a recent analysis of data up to 2017 reported a significant increase in serotype 19A carriage prevalence in this age group [Citation99]. This subsequent analysis reported declines in VT carriage in all age groups assessed (<5 years, 5–17 years, ≥18 years) and a significant increase in NPC of NVT (NVT NPC) in children but not in adults.
5. Indirect effects
The indirect effects of PCVs against pneumococcal disease among unvaccinated individuals result from a reduction in pneumococcal carriage and therefore transmission [Citation100], although the quantitative relationship between carriage decrease and disease decrease is not known [Citation101]. Data suggestive of indirect effects of PHiD-CV against IPD, meningitis, and pneumonia are shown in (further information is provided in Supplementary Table 2).
Figure 4. Indirect effects of PHiD-CV on invasive pneumococcal disease (IPD), meningitis, and pneumonia in vaccine-ineligible children and adults estimated from post-marketing impact studies in Finland, the Netherlands, Iceland, and Brazil. Further information on the analyses conducted is provided in Supplementary Table 2.
diag: diagnosed; lab: laboratory; overall IPD: IPD due to any pneumococcal serotype; pneumococcal meningitis: meningitis due to any pneumococcal serotype; VT IPD: IPD due to vaccine serotypes; VT meningitis: meningitis due to vaccine serotypes; mo: months; y: years. Error bars represent 95% confidence intervals.
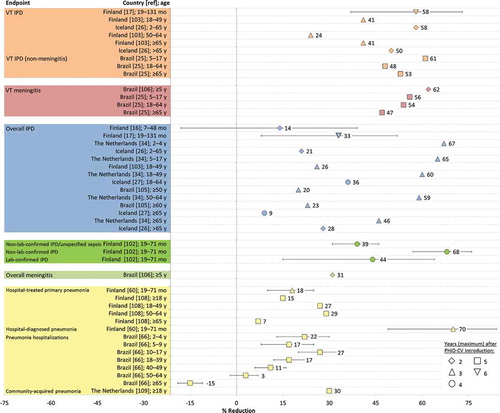
5.1. Indirect effects on IPD
After infant vaccination with PHiD-CV, herd protection against VT IPD is suggested from the FinIP trial and from post-marketing studies in Finland, Iceland, the Netherlands, and Brazil in the first years after the vaccine was introduced.
In Finland, the incidence of IPD among unvaccinated people aged 5 years or older was calculated from national register data and compared between the PHiD-CV and control clusters of the FinIP trial in 2011 and 2012 [Citation102,Citation103]. In 2011, with vaccination coverage of 29–61% in the PHiD-CV clusters, there was no consistent indirect effect against culture-confirmed IPD [Citation103] but there was a decrease of 29% (95% CI: −6, 35) in suspected non-confirmed IPD among the unvaccinated population [Citation102]. In 2012, the incidence of culture-confirmed VT IPD among the unvaccinated population was reduced by one-third [Citation103]. Because of a slight increase both in vaccine-related and other NVT IPD, the net reduction of IPD among the unvaccinated population was 8% (95% CI: −11, 23). It is noteworthy that these findings represent the first demonstration of indirect effects on clinical outcomes for any PCV in a trial setting.
In a nationwide observational study in Finland that compared IPD rates in a cohort of vaccine-eligible children with season-and age-matched cohorts before PHiD-CV introduction, reductions of 33% (95% CI: 8, 52) in culture-confirmed IPD and 58% (95% CI: 37, 73) in VT IPD were detected among 19- to 131-month-old children not eligible for PHiD-CV vaccination [Citation19]. Clinically suspected IPD in unvaccinated children, defined as non-laboratory-confirmed IPD, declined by 68% (95% CI: 57, 76) between 2007–2009 and 2012–2014 [Citation104]. There is also evidence of an indirect effect among the adult population in Finland [Citation105]. The incidence of VT IPD decreased by 24–41% in adult age groups in a one-year period post-introduction compared to a three-year period before PHiD-CV introduction. Rates of overall IPD decreased only for the 18–49 years age group because of increases in IPD caused by NVTs, including 19A, in older age groups.
In Iceland, 79 IPD cases were diagnosed in 2011–2013 in comparison with 116 in 2008–2010 (pre-vaccine period), with significant declines recorded for VT IPD in 2–65 years and over 65 years age groups [Citation28]. An analysis of 2014–2015 data showed continued declines in IPD cases for the 18–64 years age group but increases among those aged 65 years and older [Citation29]. National and regional data from Brazil also showed reductions in VT and overall IPD as well as in rates of meningitis in some vaccine-ineligible age groups up to 5 years after PHiD-CV implementation [Citation27,Citation68,Citation106–Citation108]. In the Netherlands, incidences of overall IPD, IPD due to serotypes contained in 7vCRM, and IPD caused by the three additional serotypes covered by PHiD-CV decreased in non-vaccinated age groups in the third year after PHiD-CV introduction, suggesting herd effects [Citation36]. Increases in the incidence of NVT IPD among non-vaccinated age groups were of smaller magnitude than reductions in VT IPD, leading to a net reduction in overall IPD, and the incidence of NVT IPD stabilized in the second and third years after PHiD-CV introduction, partially caused by a reduction in 19A IPD.
5.2. Indirect effects on pneumonia
FinIP clinical trial data showed no indirect effect against clinical pneumonia in the unvaccinated population each year from 2011 to 2013, apart from a lower incidence of empyema in the unvaccinated PHiD-CV clusters in 2011 [Citation109]. However, surveillance data from the national immunization program in Finland showed reductions in pneumonia rates in children not eligible for vaccination 2–3 years after PHiD-CV was introduced [Citation62]. Comparison of 2012–2013 data with those from historical season- and age-matched periods before its introduction showed a 18% (95% CI: 10, 25) reduction in hospital-treated primary pneumonia and a 70% (95% CI: 49, 84) reduction in hospital-diagnosed pneumococcal pneumonia, suggesting a herd effect.
Another analysis, taking into account pre-vaccination trends in the disease, showed a 5% annual decrease (2011–2015) in rate of hospital-treated pneumonia in adults in Finland after PHiD-CV introduction in comparison with a 2% annual increase in 2004–2010 [Citation110]. A significant 7% decline was seen in persons aged 65 years or older (132 per 100,000), indicating 1456 fewer pneumonia hospitalizations annually, and the greatest relative rate reduction (29%) in adults aged 50–64 years. In the Netherlands, the proportion of pneumococcal CAP among hospitalized adult patients decreased from 37% in 2004–2006 to 26% during the period after PHiD-CV introduction in the childhood immunization program, while similar shifts were not observed for other pathogens contributing to the etiology of CAP [Citation111].
6. Expert commentary
We reviewed evidence on the efficacy/effectiveness of PHiD-CV against IPD, pneumonia, and AOM that has accumulated since its licensure. As well as several large RCTs, various population-based post-marketing studies have been conducted to measure the direct and indirect impacts of large-scale vaccination programs. This enables a comprehensive assessment of the overall impact of PHiD-CV in ‘real-life’ as well as clinical trial settings.
Immunization of infants and young children with PHiD-CV using different schedules and in different settings and populations has produced considerable reductions in the incidence of VT IPD and overall IPD. Evidence is also emerging for direct protection by PHiD-CV against IPD caused by vaccine-related serotypes, such as serotype 19A in immunized children, as already reflected in the vaccine label, and serotype 6A [Citation19,Citation24]. Protection against pneumonia and AOM was shown, including reduced antibiotic consumption for AOM in the FinIP trial and reduction of AOM- and pneumonia-related outcomes in Finland, Iceland, Sweden, and Brazil. Vaccine effectiveness estimates against various clinical end points in the FinIP trial were comparable for the infant 3 + 1 and 2 + 1 schedules. Also, the 3 + 0 schedule used in Kenya and Mozambique was effective in reducing the incidence of VT and overall IPD. However, equivalence of the 3 + 0 and 2 + 1 schedules, in terms of induction of immune memory, has not been determined.
Because disease etiology for pneumonia and AOM is variable and often not verified, lower point estimates of vaccine efficacy and effectiveness were expected against these mucosal diseases in comparison to IPD. However, given the considerable burden of pneumonia and AOM [Citation112,Citation113], and evidence of effectiveness against TTP and reduced outpatient antibiotic prescriptions, this represents substantial reductions from public health and health-care cost perspectives.
Indeed, as public health interventions, vaccination programs need to be evaluated based on public health relevance, i.e. the net benefits, not just changes due to individual serotypes or other fractions of overall disease burden. As an additional indication of the public health value of PHiD-CV [Citation114], FinIP trial data were used to estimate vaccine-preventable disease incidences (VPDIs) from differences in disease incidences between PHiD-CV and control clusters [Citation115]. Among infants vaccinated with the 3 + 1 or 2 + 1 schedule, VPDIs per 100,000 person-years were 75 (95% CI: 33, 116) for laboratory-confirmed IPD, 210 (95% CI: 100, 320) for non-laboratory-confirmed IPD, 271 (95% CI: 9, 533) for hospital-diagnosed pneumonia, 1143 (95% CI: −230, 2516) for any TTP, and 11,381 (95% CI: −579, 23,341) for any outpatient antimicrobial prescription. Corresponding numbers needed to vaccinate (number of children needed to be vaccinated to prevent one outcome episode during 2-year follow-up) were 671, 238, 185, 44, and 5, respectively. It will be interesting to compare these values from a European high-income country to those from other settings.
With reports of decreases in VT S. pneumoniae NPC, herd protection against VT pneumococcal diseases is expected with PHiD-CV. This is supported by a growing body of evidence from the FinIP trial and post-marketing studies, which suggests that PHiD-CV confers early herd protection against VT IPD in unvaccinated populations. However, full characterization of its indirect effects requires longer follow-up and additional data from other countries. Any influence of PCVs on the balance between potentially pathogenic bacterial species in the nasopharynx also requires further investigation. Moreover, although data suggest that PHiD-CV may decrease NTHi AOM and might protect against NTHi carriage [Citation6], more evidence is warranted.
Following 7vCRM introduction, consistent and significant decreases in both overall and VT IPD in children were sustained for 7 years, supporting the use of PCVs [Citation116]. Increases in NVT IPD, particularly due to serotype 19A, occurred in most countries [Citation116,Citation117], which was possibly due to vaccine pressure although antibiotic resistance and clonal spread might also have contributed [Citation44,Citation118]. With PHiD-CV, no evidence of serotype replacement disease in children was found in the RCTs, but trends for increased NVT NPC primarily after the booster dose were observed. Impact studies and surveillance data showed signs of serotype replacement in IPD, as expected, since this has been observed for other PCVs, although there was also evidence that replacement NVT may have lower invasive potential and greater antibiotic susceptibility compared with VT. In a surveillance study conducted in the Netherlands, while there was a modest reduction in pneumococcal NPC in 24-month-old children following PHiD-CV (49% versus 66% pre-vaccination), the overall IPD incidence declined from 20 to 6 per 100,000 in children aged under 5 years from pre-vaccination to 2014–2016, because replacement NVT showed lower invasive potential compared with VT [Citation119]. A recent study in Iceland reported greater susceptibility to penicillin among NVT than VT [Citation120], as well as declines in antimicrobial-resistant S. pneumoniae following PHiD-CV vaccination, and a similar observation was reported recently from Mozambique [Citation121]. This is consistent with reductions in the prevalence of antimicrobial-resistant pneumococci reported after the introduction of other PCVs [Citation122]. The magnitude and relevance of these observations must be assessed over a longer period. It is also important to consider the role of epidemiological factors, secular trends, and temporal changes in interventions when interpreting the results of impact studies that compare the same population before and after PCV introduction in immunization programs [Citation123].
In addition to PHiD-CV, the effectiveness and impact of PCV13 has been documented [Citation124], although randomized trials for clinical outcomes in children are lacking. PCV13 contains serotype 19A, and thus it is not surprising that PCV13 elicits a stronger immune response against this serotype than PHiD-CV [Citation125]. There have been no direct large-scale comparative studies between PHiD-CV and PCV13 although in Sweden both are used in infant vaccination programs, but in different counties around the country. Interestingly, similar reductions in pneumonia outcomes, and similar or more pronounced declines with PHiD-CV against AOM outcomes, have been observed between counties using different vaccination programs [Citation71,Citation80,Citation81].
7. Five-year view
PHiD-CV has been demonstrated to be an effective vaccine in high- and middle-income settings, which encourages its use in lower income countries where the pneumococcal disease burden is higher. This is supported by a recent assessment of the evidence generated so far, coordinated by the International Vaccine Access Center, which concluded that PHiD-CV and PCV13 can be considered global products, appropriate for any country setting, with comparable disease impact in routine use settings [Citation126]. Since 2010, more than 50 countries have been approved for GAVI support to add PCV vaccination to their national immunization programs. Some of the countries with the highest burdens of pneumonia mortality have introduced PHiD-CV (including Pakistan, Bangladesh, Nigeria, and Ethiopia) and there is an expectation that this will contribute to mortality reductions in those regions.
The increase in global PCV coverage and availability necessitates high-quality, population-based surveillance to monitor the rate of pneumococcal diseases both in vaccine-eligible children and in unvaccinated groups including the elderly. Pre- and post-vaccine introduction data are required to gather sufficient, reliable baseline data and draw meaningful conclusions. However, surveillance of long-term effects is required, particularly the extent to which vaccines induce herd protection and the relationship between colonization with new serotypes and replacement disease. It should also be kept in mind that changes in one serotype may have little relevance in a broader perspective.
In the future, conjugate formulations might be introduced to target pneumococcal serotypes that become more prevalent following infant immunization programs or vaccines containing pneumococcal proteins to provide serotype-independent protection. In the meantime, with widespread availability of high-valency PCVs, we can anticipate major reductions in pneumococcal disease and child deaths in many countries, including regions with the highest burden of disease.
Key issues
Evidence of the protective efficacy/effectiveness and impact of PHiD-CV against pneumococcal diseases is available from randomized, double-blind trials and from post-marketing studies in various countries.
Immunization of infants and young children with PHiD-CV using different schedules, and in different settings and populations, has led to considerable reductions in the incidence of VT IPD and overall IPD.
PHiD-CV was shown to be effective in preventing pneumonia and AOM caused by vaccine serotypes and there was evidence of an impact against various AOM-related end points, including TTP and antimicrobial purchases, which contribute substantially to childhood health-care costs.
PHiD-CV vaccination was followed by consistent reductions in VT NPC, with a delayed, relatively lower increase in NVT NPC in clinical studies, in contrast to observations with lower-valency PCVs.
A growing body of evidence suggests that PHiD-CV infant vaccination confers early herd protection against disease caused by vaccine pneumococcal serotypes in older children and adults.
Trademark statement
Synflorix is a trademark of the GSK group of companies. Prevenar/Prevnar and Prevenar13/Prevnar13 are trademarks of Pfizer Inc.
Declaration of interest
T Mrkvan is employed by the GSK group of companies and holds shares in the GSK group of companies. D Borys is employed by the GSK group of companies and holds shares in the GSK group of companies. S I Pelton is the principle investigator for the investigator-initiated research grants to Boston Medical Center from Pfizer. He receives honoraria for participation to international symposium from Pfizer as well. He also received honoraria for participation to advisory board from Pfizer, Sanofi-Pasteur, the GSK group of companies, and Seqirus. J Ruiz-Guiñazú is employed by the GSK group of companies and holds shares in the GSK group of companies. A.A Palmu is an employee of the department of public health solutions at the national institute for Health and Welfare, which has received research funding from the GSK group of companies for conduct of trials. The authors have no other relevant affiliations or financial involvement with any organization or entity with a financial interest in or financial conflict with the subject matter or materials discussed in the manuscript apart from those disclosed.
Reviewer disclosures
Peer reviewers on this manuscript have no relevant financial or other relationships to disclose.
Supplemental Material
Download MS Word (35.4 KB)Acknowledgments
Joanne Knowles provided writing assistance and Thomas Déplanque (XPE Pharma & Science c/o GSK) provided publication coordination and editorial support.
Supplementary material
Supplemental data for this article can be accessed here.
Additional information
Funding
Notes on contributors
Tomas Mrkvan
Tomas Mrkvan All authors contributed to the literature search and interpretation of data reviewed. All authors provided critical contributions during the development of the manuscript. All authors approve and take responsibility for the content of this manuscript.
References
- World Health Organization. Pneumococcal vaccines. WHO position paper - 2012. Wkly Epidemiol Rec. 2012;87:129–144.
- Prymula R, Schuerman L. 10-valent pneumococcal nontypeable Haemophilus influenzae PD conjugate vaccine: synflorix. Expert Rev Vaccines. 2009;8:1479–1500.
- Vesikari T, Wysocki J, Chevallier B, et al. Immunogenicity of the 10-valent pneumococcal non-typeable Haemophilus influenzae protein D conjugate vaccine (PHiD-CV) compared to the licensed 7vCRM vaccine. Pediatr Infect Dis J. 2009;28:S66–S76.
- Knuf M, Pankow-Culot H, Grunert D, et al. Induction of immunologic memory following primary vaccination with the 10-valent pneumococcal nontypeable Haemophilus influenzae protein D conjugate vaccine in infants. Pediatr Infect Dis J. 2012;31:e31–e36.
- Silfverdal SA, Coremans V, François N, et al. Safety profile of the 10-valent pneumococcal non-typeable Haemophilus influenzae protein D conjugate vaccine (PHiD-CV). Expert Rev Vaccines. 2017;16:109–121.
- Clarke C, Bakaletz LO, Ruiz-Guinazu J, et al. Impact of protein D-containing pneumococcal conjugate vaccines on non-typeable Haemophilus influenzae acute otitis media and carriage. Expert Rev Vaccines. 2017;16:1–14.
- Hanquet G, Valenciano M, Simondon F, et al. Vaccine effects and impact of vaccination programmes in post-licensure studies. Vaccine. 2013;31:5634–5642.
- Leach AJ, Wigger C, Hare K, et al. Reduced middle ear infection with non-typeable Haemophilus influenzae, but not Streptococcus pneumoniae, after transition to 10-valent pneumococcal non-typeable H. influenzae protein D conjugate vaccine. BMC Pediatr. 2015;15:162.
- Almeida SC, Cassiolato AP, Amorim CS, et al. Increase in 19A and 6C serotypes after 5 years of PCV10 routine immunization in Brazil. 10th International Symposium on Pneumococci and Pnemococcal Diseases; 2016 Jun 26-30; Glasgow, UK.
- Palmu AA, Jokinen J, Borys D, et al. Effectiveness of the ten-valent pneumococcal Haemophilus influenzae protein D conjugate vaccine (PHiD-CV10) against invasive pneumococcal disease: a cluster randomised trial. Lancet. 2013;381:214–222.
- Vesikari T, Forsten A, Seppä I, et al. Effectiveness of the 10-valent pneumococcal non-typeable Haemophilus influenzae protein D-conjugated vaccine (PHiD-CV) against carriage and acute otitis media - a double-blind randomized clinical trial in Finland. J Pediatric Infect Dis Soc. 2016;5:237–248.
- Tregnaghi MW, Sáez-Llorens X, López P, et al. Efficacy of pneumococcal nontypable Haemophilus influenzae protein D conjugate vaccine (PHiD-CV) in young Latin American children: a double-blind, randomized controlled trial. PLoS Med. 2014;11:e1001657.
- Hammitt LL, Ojal J, Bashraheil M, et al. Immunogenicity, impact on carriage and reactogenicity of 10-valent pneumococcal non-typeable Haemophilus influenzae protein D conjugate vaccine in Kenyan children aged 1–4 years: a randomized controlled trial. PLoS One. 2014;9:e85459.
- Prymula R, Hanovcova I, Splino M, et al. Impact of the 10-valent pneumococcal non-typeable Haemophilus influenzae Protein D conjugate vaccine (PHiD-CV) on bacterial nasopharyngeal carriage. Vaccine. 2011;29:1959–1967.
- van den Bergh MR, Spijkerman J, Swinnen KM, et al. Effects of the 10-valent pneumococcal nontypeable Haemophilus influenzae protein D-conjugate vaccine on nasopharyngeal bacterial colonization in young children: a randomized controlled trial. Clin Infect Dis. 2013;56:e30–e39.
- Pan American Health Organization. SIREVA II regional reports [internet]. Washington (DC): PAHO; 2012 [cited 2017 Nov 29]. Available from: http://www.paho.org/hq/index.php?option=com_content&view=article&id=5536&Itemid=3966&lang=en
- Sartori AM, Nascimento Ade F, Yuba TY, et al. Methods and challenges for the health impact assessment of vaccination programs in Latin America. Rev Saude Publica. 2015;49:90.
- Jokinen J, Rinta-Kokko H, Siira L, et al. Impact of ten-valent pneumococcal conjugate vaccination on invasive pneumococcal disease in Finnish children - a population-based study. PLoS One. 2015;10:e0120290.
- Rinta-Kokko H, Palmu AA, Auranen K, et al. Long-term impact of 10-valent pneumococcal conjugate vaccination on invasive pneumococcal disease among children in Finland. Vaccine. 2018;36:1934–1940.
- Rinta-Kokko H, Auranen K, Palmu A, et al. Effectiveness of 10-valent pneumococcal conjugate vaccine (PCV10) against invasive pneumococcal disease (IPD) during ongoing national vaccination programme (NVP) in Finland. 9th International Symposium on Pneumococci & Pneumococcal Diseases; 2014 Mar 9–13; Hyderabad, India.
- Polkowska A, Toropainen M, Ollgren J, et al. Bacterial meningitis in Finland, 1995–2014: a population-based observational study. BMJ Open. 2017;7:e015080.
- Sihvonen R, Siira L, Toropainen M, et al. Streptococcus pneumoniae antimicrobial resistance decreased in the Helsinki Metropolitan Area after routine 10-valent pneumococcal conjugate vaccination of infants in Finland. Eur J Clin Microbiol Infect Dis. 2017;36:2109–2116.
- Domingues CM, Verani JR, Montenegro Renoiner EI, et al. Effectiveness of ten-valent pneumococcal conjugate vaccine against invasive pneumococcal disease in Brazil: a matched case-control study. Lancet Respir Med. 2014;2:464–471.
- Verani JR, Domingues CM, Moraes JC. Indirect cohort analysis of 10-valent pneumococcal conjugate vaccine effectiveness against vaccine-type and vaccine-related invasive pneumococcal disease. Vaccine. 2015;33:6145–6148.
- Andrade AL, Minamisava R, Policena G, et al. Evaluating the impact of PCV-10 on invasive pneumococcal disease in Brazil: A time-series analysis. Hum Vaccin Immunother. 2016;12:285–292.
- de Oliveira LH, Camacho LA, Coutinho ES, et al. Impact and effectiveness of 10 and 13-valent pneumococcal conjugate vaccines on hospitalization and mortality in children aged less than 5 years in Latin American countries: A systematic review. PLoS One. 2016;11:e0166736.
- Brandileone MC, Almeida SCG, Minamisava R, et al. Distribution of invasive Streptococcus pneumoniae serotypes before and 5 years after the introduction of 10-valent pneumococcal conjugate vaccine in Brazil. Vaccine. 2018;36:2559–2566.
- Erlendsdóttir H, Haraldsson A, Hrafnkelsson B, et al. An early reduction of invasive pneumococcal infections after PCV-10 immunisation. Poster session presented at: 9th International Symposium on Pneumococci and Pneumococcal Diseases; 2014 Mar 9–13; Hyderabad, India.
- Kristinsson KG, Erlendsdóttir H, Quirk S, et al. Effect of pneumococcal vaccination on invasive pneumococcal disease in Iceland 2009–2015. Poster session presented at: 26th European Congress of Clinical Microbiology and Infectious Diseases; 2016 Apr 9–12; Amsterdam, The Netherlands.
- KEMRI Wellcome Trust. Pneumococcal conjugate vaccine impact study (PCVIS) [internet]. Kenya: KWTRP; 2017 [cited 2017 Nov 29]. Available from: http://kemri-wellcome.org/programme/pcvis-2/
- Nhantumbo AA, Weldegebriel G, Katsande R, et al. Surveillance of impact of PCV-10 vaccine on pneumococcal meningitis in Mozambique, 2013–2015. PLoS One. 2017;12:e0177746.
- Massora S, Mucavele H, Lessa F, et al. Impact of 10-valent pneumococcal conjugate vaccine (PCV10) on invasive pneumococcal disease among children in Mozambique, 2008–2016. 11th International Symposium on Pneumococci and Pneumococcal Diseases 2018 Apr 15–19; Melbourne, Australia.
- De Wals P, Lefebvre B, Markowski F, et al. Impact of 2+1 pneumococcal conjugate vaccine program in the province of Quebec, Canada. Vaccine. 2014;32:1501–1506.
- Deceuninck G, De Serres G, Boulianne N, et al. Effectiveness of three pneumococcal conjugate vaccines to prevent invasive pneumococcal disease in Quebec, Canada. Vaccine. 2015;33:2684–2689.
- Institute of Environmental Science & Research Limited. Public health surveillance information for New Zealand public health action - invasive pneumococcal disease reports [internet]. New Zealand: Ministry of Health; 2014 [cited 2017 Nov 29]. Available from: https://surv.esr.cri.nz/surveillance/IPD.php
- Knol MJ, Wagenvoort GHJ, Sanders EAM, et al. Invasive pneumococcal disease 3 years after introduction of 10-valent pneumococcal conjugate vaccine, the Netherlands. Emerg Infect Dis. 2015;21:2040–2044.
- Netherlands Reference Laboratory for Bacterial Meningitis (AMC/RIVM). Pneumokokken [internet]. Amsterdam: RIVM; 2017 [cited 2017 Nov 29]. Available from: http://www.rivm.nl/Onderwerpen/P/Pneumokokken
- Knol MJ, Van der Ende A, Sanders L, et al. Vaccine effectiveness of 10-valent pneumococcal conjugate vaccine against invasive pneumococcal disease: indirect cohort design. 10th International Symposium on Pneumococci and Pneumococcal Diseases; 2016 Jun 26–30; Glasgow, UK.
- Naucler P, Galanis I, Morfeldt E, et al. Comparison of the impact of Pneumococcal Conjugate Vaccine 10 or Pneumococcal Conjugate Vaccine 13 on invasive pneumococcal disease in equivalent populations. Clin Infect Dis. 2017;65:1780–1789.
- Schooley RT. Expression of concern relating to Naucler et al. Clin Infect Dis. 2018. DOI:10.1093/cid/ciy316
- GSK clinical study register: study numbers 113994, 116485 [internet]. UK: GSK;2017 [cited 2017 Nov 29]. Available from: https://www.gsk-clinicalstudyregister.com/files2/529cf371-82b1-40c4-9bed-2e116b36e6ac and https://www.gsk-clinicalstudyregister.com/files2/116485%20-%20Clinical-Study-Result-Summary.pdf
- Odutola A, Ota MC, Antonio M, et al. Immunogenicity and safety of an investigational protein-based pneumococcal vaccine in infants following 3+0 and 2+1 vaccination schedules: a phase II randomized trial in the Gambia. 10th International Symposium on Pneumococci & Pneumococcal Diseases; 2016 Jun 26–30; Glasgow, UK.
- Park IH, Moore MR, Treanor JJ, et al. Differential effects of pneumococcal vaccines against serotypes 6A and 6C. J Infect Dis. 2008;198:1818–1822.
- Moore MR, Gertz RE Jr., Woodbury RL, et al. Population snapshot of emergent Streptococcus pneumoniae serotype 19A in the United States, 2005. J Infect Dis. 2008;197:1016–1027.
- Synflorix, INN-Pneumococcal polysaccharide conjugate vaccine (adsorbed) [internet]. UK: European Medicines Agency; [ cited 2017 Nov 29]. Available from: http://www.ema.europa.eu/docs/en_GB/document_library/EPAR_-_Product_Information/human/000973/WC500054346.pdf
- Palmu AA, Jokinen J, Nieminen H, et al. Vaccine effectiveness of the pneumococcal Haemophilus influenzae protein D conjugate vaccine (PHiD-CV10) against clinically suspected invasive pneumococcal disease: a cluster-randomised trial. Lancet Respir Med. 2014;2:717–727.
- Palmu AA, Kilpi TM, Rinta-Kokko H, et al. Pneumococcal conjugate vaccine and clinically suspected invasive pneumococcal disease. Pediatrics. 2015;136:e22–e27.
- Laaksonen N, Rintamäki L, Korppi M. Pneumococcal vaccinations effectively prevent blood culture-negative infections that resemble occult pneumococcal bacteraemia or bacteraemic pneumococcal pneumonia at one to 36 months of age. Acta Paediatr. 2016;105:1487–1492.
- Madhi SA, Klugman KP. World Health Organisation definition of “radiologically-confirmed pneumonia” may under-estimate the true public health value of conjugate pneumococcal vaccines. Vaccine. 2007;25:2413–2419.
- Black SB, Shinefield HR, Ling S, et al. Effectiveness of heptavalent pneumococcal conjugate vaccine in children younger than five years of age for prevention of pneumonia. Pediatr Infect Dis J. 2002;21:810–815.
- Cutts FT, Zaman SM, Enwere G, et al. Efficacy of nine-valent pneumococcal conjugate vaccine against pneumonia and invasive pneumococcal disease in The Gambia: randomised, double-blind, placebo-controlled trial. Lancet. 2005;365:1139–1146.
- Klugman KP, Madhi SA, Huebner RE, et al. A trial of a 9-valent pneumococcal conjugate vaccine in children with and those without HIV infection. N Engl J Med. 2003;349:1341–1348.
- Lucero MG, Nohynek H, Williams G, et al. Efficacy of an 11-valent pneumococcal conjugate vaccine against radiologically confirmed pneumonia among children less than 2 years of age in the Philippines: a randomized, double-blind, placebo-controlled trial. Pediatr Infect Dis J. 2009;28:455–462.
- Hansen J, Black S, Shinefield H, et al. Effectiveness of heptavalent pneumococcal conjugate vaccine in children younger than 5 years of age for prevention of pneumonia: updated analysis using World Health Organization standardized interpretation of chest radiographs. Pediatr Infect Dis J. 2006;25:779–781.
- Loens K, Van Heirstraeten L, Malhotra-Kumar S, et al. Optimal sampling sites and methods for detection of pathogens possibly causing community-acquired lower respiratory tract infections. J Clin Microbiol. 2009;47:21–31.
- Kilpi T, Palmu AA, Puumalainen T, et al. Effectiveness of the 10-valent pneumococcal Haemophilus influenzae protein D conjugate vaccine (PHiD-CV10) against hospital-diagnosed pneumonia in infants - FinIP trial. 31st Annual Meeting of the European Society for Paediatric Infectious Diseases; 2013 May 28–Jun 1; Milan, Italy.
- Kilpi T, Jokinen J, Puumalainen T, et al. Effectiveness of pneumococcal Haemophilus influenzae protein D conjugate vaccine against radiologically confirmed pneumonia. 54th Interscience Conference on Antimicrobial Agents and Chemotherapy; 2014 Sep 5–9; Washington, DC.
- Afonso ET, Minamisava R, Bierrenbach AL, et al. Effect of 10-valent pneumococcal vaccine on pneumonia among children, Brazil. Emerg Infect Dis. 2013;19:589–597.
- Diaz J, Terrazas S, Bierrenbach AL, et al. Effectiveness of the 10-valent pneumococcal conjugate vaccine (PCV-10) in children in Chile: A nested case-control study using nationwide pneumonia morbidity and mortality surveillance data. PLoS One. 2016;11:e0153141.
- Fernández VJP, Goecke HC, von Borries C, et al. Incidence of admissions due to pneumonia in children under 24 months old before and after the introduction of the 10-valent pneumococcal conjugate vaccine into the National Immunization Program of Chile. Rev Chil Pediatr. 2015;86:168–172. Spanish.
- Minamisava R, Sgambatti S, Morais-Neto OL, et al. Impact of PCV10 introduction on pneumonia mortality rates in Brazil: a time series analysis. 9th International Symposium on Pneumococci & Pneumococcal Diseases; 2014 Mar 9–13; Hyderabad, India.
- Palmu AA, Rinta-Kokko H, Nohynek H, et al. Impact of ten-valent pneumococcal conjugate vaccine on pneumonia in Finnish children in a nation-wide population-based study. PLoS One. 2017;12:e0172690.
- Scotta MC, Veras TN, Klein PC, et al. Impact of 10-valent pneumococcal non-typeable Haemophilus influenzae protein D conjugate vaccine (PHiD-CV) on childhood pneumonia hospitalizations in Brazil two years after introduction. Vaccine. 2014;32:4495–4499.
- Sgambatti S, Minamisava R, Bierrenbach AL, et al. Early impact of 10-valent pneumococcal conjugate vaccine in childhood pneumonia hospitalizations using primary data from an active population-based surveillance. Vaccine. 2016;34:663–670.
- Sigurdsson S, Kristinsson KG, Erlendsdóttir H, et al. Decreased incidence of respiratory infections in children after vaccination with ten-valent pneumococcal vaccine. Pediatr Infect Dis J. 2015;34:1385–1390.
- Silaba M, Ooko M, Bottomley C, et al. The impact of 10-valent pneumococcal conjugate vaccine (PCV10) on the incidence of radiologically-confirmed pneumonia and on pneumonia hospitalizations among children in Kilifi, Kenya. 10th International Symposium on Pneumococci and Pneumococcal Diseases; 2016 Jun 26–30; Glasgow, UK.
- Verani J, Bigogo G, Muema S, et al. Pneumonia-related mortality among children in an urban slum in Nairobi, Kenya following 10-valent pneumococcal conjugate vaccine introduction. 10th International Symposium on Pneumococci and Pneumococcal Diseases; 2016 Jun 26–30; Glasgow, UK.
- Andrade AL, Afonso ET, Minamisava R, et al. Direct and indirect impact of 10-valent pneumococcal conjugate vaccine introduction on pneumonia hospitalizations and economic burden in all age-groups in Brazil: A time-series analysis. PLoS One. 2017;12:e0184204.
- Mucavele H, Massora S, Lessa F, et al. Impact of 10-valent pneumococcal conjugate vaccine on radiologically confirmed pneumonia among children in Mozambique 11th International Symposium on Pneumococci and Pneumococcal Diseases; 2018 Apr 15–19; Melbourne, Australia.
- Zhou Z, Deceuninck G, Boucher F, et al. Impact of two pneumococcal conjugate vaccines on hospitalizations for lower-tract respiratory infections (LRTI) in Quebec, Canada. 9th International Symposium on Pneumococci & Pneumococcal Diseases; 2014 Mar 9–13; Hyderabad, India.
- Berglund A, Ekelund M, Fletcher MA, et al. All-cause pneumonia hospitalizations in children <2 years old in Sweden, 1998 to 2012: impact of pneumococcal conjugate vaccine introduction. PLoS One. 2014;9:e112211.
- Sáez-Llorens X, Rowley S, Wong D, et al. Efficacy of 10-valent pneumococcal non-typeable Haemophilus influenzae protein D conjugate vaccine against acute otitis media and impact on nasopharyngeal carriage in Panamanian children - a randomized controlled trial. Human Vacc Immunother. 2017;13:1213–1228.
- Qureishi A, Lee Y, Belfield K, et al. Update on otitis media - prevention and treatment. Infect Drug Resist. 2014;7:15–24.
- Palmu AA, Jokinen J, Nieminen H, et al. Effect of pneumococcal Haemophilus influenzae protein D conjugate vaccine (PHiD-CV10) on outpatient antimicrobial purchases: a double-blind, cluster randomised phase 3–4 trial. Lancet Infect Dis. 2014;14:205–212.
- Palmu AA, Jokinen J, Nieminen H, et al. Effectiveness of the ten-valent pneumococcal conjugate vaccine against tympanostomy tube placements in a cluster-randomized trial. Pediatr Infect Dis J. 2015;34:1230–1235.
- Fireman B, Black SB, Shinefield HR, et al. Impact of the pneumococcal conjugate vaccine on otitis media. Pediatr Infect Dis J. 2003;22:10–16.
- Palmu AA, Verho J, Jokinen J, et al. The seven-valent pneumococcal conjugate vaccine reduces tympanostomy tube placement in children. Pediatr Infect Dis J. 2004;23:732–738.
- Prymula R, Peeters P, Chrobok V, et al. Pneumococcal capsular polysaccharides conjugated to protein D for prevention of acute otitis media caused by both Streptococcus pneumoniae and non-typable Haemophilus influenzae: a randomised double-blind efficacy study. Lancet. 2006;367:740–748.
- Palmu AA, Rinta-Kokko H, Nohynek H, et al. Impact of national ten-valent pneumococcal conjugate vaccine programme on reducing antimicrobial use and tympanostomy tube placements in Finland. Pediatr Infect Dis J. 2018;37:97–102.
- Gisselsson-Solen M. Trends in otitis media incidence after conjugate pneumococcal vaccination; a national observational study. Pediatr Infect Dis J. 2017;36:1027–1031.
- Talarico C, Feng Y, Medin E, et al. Impact of pneumococcal conjugate vaccines (PCVs) on otitis media incidence in Swedish children. 11th International Symposium on Pneumococci and Pneumococcal Diseases; 2018 Apr 15–19; Melbourne, Australia.
- Sigurdsson S, Eythorsson E, Hrafnkelsson B, et al. Reduction in all-cause acute otitis media in children less than three years of age in primary care following pneumococcal vaccination with PHiD-CV10: A whole population study. Clin Infect Dis. 2018. DOI:10.1093/cid/ciy233
- Eyþorsson ES, Sigurdsson S, Hrafnkelsson B, et al. Pneumococcal vaccination in Iceland: PHiD-CV10 effectiveness against AOM associated antimicrobial prescriptions in children under three years of age. 11th International Symposium on Pneumococci and Pneumococcal Diseases; 2018 Apr 15–19; Melbourne, Australia.
- Rosenblut A, Rosenblut M, Garcia K, et al. Frequency of acute otitis media in children under 24 months of age before and after the introduction of the 10-valent pneumococcal conjugate vaccine into the national immunization program in Chile. Pediatr Infect Dis J. 2018;37:132–134.
- Sartori AL, Minamisava R, Bierrenbach AL, et al. Reduction in all-cause otitis media-related outpatient visits in children after PCV10 introduction in Brazil. PLoS One. 2017;12:e0179222.
- De Wals P, Fratu R, Defay F, et al. Frequency of visits for otitis media in Quebec: face-to-nape comparison of children exposed to PCV-7 or PHiD-CV. 31st Meeting of the European Society for Paediatric Infectious Diseases; 2013 May 28–Jun 1; Milan, Italy.
- Bogaert D, De Groot R, Hermans PW. Streptococcus pneumoniae colonisation: the key to pneumococcal disease. Lancet Infect Dis. 2004;4:144–154.
- Adegbola RA, Deantonio R, Hill PC, et al. Carriage of Streptococcus pneumoniae and other respiratory bacterial pathogens in low and lower-middle income countries: a systematic review and meta-analysis. PLoS One. 2014;9:e103293.
- Simell B, Auranen K, Käyhty H, et al. The fundamental link between pneumococcal carriage and disease. Expert Rev Vaccines. 2012;11:841–855.
- Fleming-Dutra KE, Conklin L, Loo JD, et al. Systematic review of the effect of pneumococcal conjugate vaccine dosing schedules on vaccine-type nasopharyngeal carriage. Pediatr Infect Dis J. 2014;33(Suppl 2):S152–S160.
- Kwambana-Adams B, Hanson B, Worwui A, et al. Rapid replacement by non-vaccine pneumococcal serotypes may mitigate the impact of the pneumococcal conjugate vaccine on nasopharyngeal bacterial ecology. Sci Rep. 2017;7:8127.
- Andrade AL, Ternes YM, Vieira MA, et al. Direct effect of 10-valent conjugate pneumococcal vaccination on pneumococcal carriage in children Brazil. PLoS One. 2014;9:e98128.
- Brandileone MC, Zanella RC, Almeida SCG, et al. Effect of 10-valent pneumococcal conjugate vaccine on nasopharyngeal carriage of Streptococcus pneumoniae and Haemophilus influenzae among children in Sao Paulo, Brazil. Vaccine. 2016;34:5604–5611.
- Sigurdsson S, Erlendsdóttir H, Quirk SJ, et al. Pneumococcal vaccination: direct and herd effect on carriage of vaccine types and antibiotic resistance in Icelandic children. Vaccine. 2017;35:5242–5248.
- Hammitt LL, Akech DO, Morpeth SC, et al. Population effect of 10-valent pneumococcal conjugate vaccine on nasopharyngeal carriage of Streptococcus pneumoniae and non-typeable Haemophilus influenzae in Kilifi, Kenya: findings from cross-sectional carriage studies. Lancet Glob Health. 2014;2:e397–e405.
- Neves FPG, Cardoso NT, Snyder RE, et al. Pneumococcal carriage among children after four years of routine 10-valent pneumococcal conjugate vaccine use in Brazil: the emergence of multidrug resistant serotype 6C. Vaccine. 2017;35:2794–2800.
- Neves FP, Pinto TC, Correa MA, et al. Nasopharyngeal carriage, serotype distribution and antimicrobial resistance of Streptococcus pneumoniae among children from Brazil before the introduction of the 10-valent conjugate vaccine. BMC Infect Dis. 2013;13:318.
- Palmu AA, Toropainen M, Kaijalainen T, et al. Direct and indirect effectiveness of the 10-valent pneumococcal conjugate vaccine against carriage in a cluster randomized trial. Pediatr Infect Dis J. 2017;36:1193–1200.
- Hammitt LL, Akech DO, Morpeth SC, et al. Population impact of 10-valent pneumococcal conjugate vaccine (PCV) on nasopharyngeal carriage of Streptococcus pneumoniae in Kilifi, Kenya. 11th International Symposium on Pneumococci and Pneumococcal Diseases; 2018 Apr 15–19; Melbourne, Australia.
- Loo JD, Conklin L, Fleming-Dutra KE, et al. Systematic review of the indirect effect of pneumococcal conjugate vaccine dosing schedules on pneumococcal disease and colonization. Pediatr Infect Dis J. 2014;33(Suppl 2):S161–S171.
- Van Effelterre T, Moore MR, Fierens F, et al. A dynamic model of pneumococcal infection in the United States: implications for prevention through vaccination. Vaccine. 2010;28:3650–3660.
- Palmu AA, Jokinen J, Ruokokoski E, et al. Indirect impact of pneumococcal Haemophilus influenzae protein-D conjugate vaccine (PHiD-CV10) on suspected non-confirmed invasive pneumococcal disease (IPD) in cluster-randomized trial. 9th International Symposium on Pneumococci & Pneumococcal Diseases; 2014 Mar 9–13; Hyderabad, India.
- Kilpi T, Palmu AA, Ruokokoski E, et al. Indirect impact of pneumococcal Haemophilus influenzae protein-D conjugate vaccine (PHiD-CV10) on invasive pneumococcal disease (IPD) in a clinical trial. 8th World Congress of the World Society for Pediatric Infectious Diseases; 2013 Nov 19–22; Cape Town, South Africa.
- Palmu AA, Rinta-Kokko H, Nuorti JP, et al. A pneumococcal conjugate vaccination programme reduced clinically suspected invasive disease in unvaccinated children. Acta Paediatr. 2018. DOI:10.1111/apa.14335
- Nuorti JP, Rinta-Kokko H, Toropainen M, et al. Invasive pneumococcal disease among adults after routine infant conjugate vaccination in Finland - implications for vaccination policy. 9th International Symposium on Pneumococci & Pneumococcal Diseases; 2014 Mar 9–13; Hyderabad, India.
- Caierão J, Cunha G, d’Azevedo PA, et al. Distribution of pneumococcal serotypes after introduction of 10-valent conjugate vaccine: a study involving adults with invasive disease, Porto Alegre, Brazil. 8th International Symposium on Pneumococci and Pneumococcal Diseases; 2012 Mar 11–15; Iguaçu Falls, Brazil.
- Azevedo J, Soares Santos M, Santos Galvão V, et al. The burden of invasive pneumococcal disease among older patients in the era of conjugate vaccine. 9th International Symposium on Pneumococci & Pneumococcal Diseases; 2014 Mar 9–13; Hyderabad, India.
- Lobo PR, Azevedo J, Silva E, et al. Epidemiology of pneumococcal meningitis in the metropolitan region of Salvador, Brazil, before and after the introduction of PCV10. Poster session presented at: 9th International Symposium on Pneumococci and Pneumococcal Diseases; 2014 Mar 9–13; Hyderabad, India.
- Palmu A, Jokinen J, Puumalainen T, et al. Indirect impact of pneumococcal Haemophilus influenzae protein D-conjugate vaccine (PHiD-CV10) on hospital-diagnosed pneumonia in cluster-randomized trial. 33rd Annual Meeting of the European Society for Paediatric Infectious Diseases; 2015 May 12–16; Leipzig, Germany.
- Okasha O, Rinta-Kokko H, Palmu AA, et al. Population-level impact of infant 10-valent pneumococcal conjugate vaccination on adult pneumonia hospitalisations in Finland. Thorax. 2018;73:262–269.
- Vestjens SMT, Wagenvoort GHJ, Grutters JC, et al. Changes in pathogens and pneumococcal serotypes causing community-acquired pneumonia in The Netherlands. Vaccine. 2017;35:4112–4118.
- Monasta L, Ronfani L, Marchetti F, et al. Burden of disease caused by otitis media: systematic review and global estimates. PLoS One. 2012;7:e36226.
- Izadnegahdar R, Cohen AL, Klugman KP, et al. Childhood Pneumonia in Developing Countries. Lancet Respir Med. 2013;1:574–584.
- Gessner BD, Feikin DR. Vaccine preventable disease incidence as a complement to vaccine efficacy for setting vaccine policy. Vaccine. 2014;32:3133–3138.
- Palmu AA, Jokinen J, Nieminen H, et al. Vaccine-preventable disease incidence of pneumococcal conjugate vaccine in the Finnish invasive pneumococcal disease vaccine trial. Vaccine. 2018;36:1816–1822.
- Feikin DR, Kagucia EW, Loo JD, et al. Serotype-specific changes in invasive pneumococcal disease after pneumococcal conjugate vaccine introduction: a pooled analysis of multiple surveillance sites. PLoS Med. 2013;10:e1001517.
- Weinberger DM, Malley R, Lipsitch M. Serotype replacement in disease after pneumococcal vaccination. Lancet. 2011;378:1962–1973.
- Houseman C, Hughes GJ, Chapman KE, et al. Increased invasive pneumococcal disease, North East England, UK. Emerg Infect Dis. 2017;23:122–126.
- Vissers M, Wijmenga-Monsuur AJ, Knol MJ, et al. Increased carriage of non-vaccine serotypes with low invasive disease potential four years after switching to the 10-valent pneumococcal conjugate vaccine in The Netherlands. PLoS One. 2018;13:e0194823.
- Hjálmarsdóttir MÁ, Quirk SJ, Sveinsdóttir H, et al. Pneumococcal vaccination in Iceland: the effect on antimicrobial susceptibility and serotype distribution. 11th International Symposium on Pneumococci and Pneumococcal Diseases; 2018 Apr 15–19;Melbourne, Australia.
- Chauque A, Lessa F, Moiane B, et al. Declines in antimicrobial resistant (AMR)-Streptococcus pneumoniae after introduction of 10-valent pneumococcal conjugate vaccine (PCV10) in Mozambique. 11th International Symposium on Pneumococci and Pneumococcal Diseases; 2018 Apr 15–19; Melbourne, Australia.
- Kim L, McGee L, Tomczyk S, et al. Biological and epidemiological features of antibiotic-resistant Streptococcus pneumoniae in pre- and post-conjugate vaccine eras: a United States perspective. Clin Microbiol Rev. 2016;29:525–552.
- Shiragami M, Mizukami A, Leeuwenkamp O, et al. Reply to Farkouh RA et al. comment on “cost-effectiveness evaluation of the 10-valent pneumococcal non-typeable Haemophilus Influenzae protein D conjugate vaccine and 13-valent pneumococcal vaccine in Japanese children”. Infect Dis Ther. 2015;4:235–244.
- Esposito S, Principi N. Impacts of the 13-valent pneumococcal conjugate vaccine in children. J Immunol Res. 2015;2015:591580.
- Isturiz R, Sings HL, Hilton B, et al. Streptococcus pneumoniae serotype 19A: worldwide epidemiology. Expert Rev Vaccines. 2017;16:1007–1027.
- Cohen O, Knoll M, O’Brien K, et al. Pneumococcal conjugate vaccine (PCV) product assessment. April 2017 [internet]. Baltimore: IVAC; 2017 [cited 2017 Nov 29]. Available from: https://www.jhsph.edu/research/centers-and-institutes/ivac/resources/pcv-product-assessment-april-25-2017.pdf.