ABSTRACT
Introduction
Numerous analyses demonstrate substantial health-economic impacts of primary vaccine effects (preventing or mitigating clinical manifestations of the diseases they target), but vaccines may also be associated with secondary effects, previously known as nonspecific, heterologous, or off-target effects.
Areas covered
We define key concepts to distinguish primary and secondary vaccine effects for health economic analyses, summarized terminology used in different fields, and perform a systematic review of health economic analyses focused on secondary vaccine effects (SVEs).
Expert opinion
Health economists integrate evidence from multiple fields, which often use incomplete or inconsistent definitions. Like regulators and policy makers, health economists require high-quality evidence of specific effects. Consistent with the limited evidence on mechanisms of action for SVEs, the associated health economic literature remains highly limited, with 4 studies identified by our systematic review. The lack of specific and well-controlled evidence that supports quantification of specific SVEs limits the consideration of these effects in vaccine research, development, regulatory, and recommendation decisions and health economic analyses.
1. Introduction
1.1. Context
Vaccines represent a multibillion dollar global industry [Citation1] and provide substantial health and economic benefits [Citation2,Citation3]. Notably, however, the market for vaccines generally includes relatively few buyers (i.e. oligopsony) and few producers (i.e. oligopoly) [Citation1,Citation4]. This occurs due to product complexity and the relatively small economic margins for many vaccines. For some vaccines, some purchasers (e.g. governments, UNICEF [Citation5], Pan American Health Organization revolving fund [Citation6]) can demand low prices, which leads manufacturers to seek economies of scale and face relatively few incentives for innovation [Citation4]. For new vaccines, including those that target emerging pathogens (e.g. COVID-19), manufacturers that succeed in developing licensed vaccines benefit from high initial profits, which offset their at-risk research, development, and initial production costs. High initial profits will also support further investments in additional production capacity that may help to meet the needs of lower-margin markets in low- and middle-income countries. Some countries may fund the production of their own vaccines as nonprofit manufacturers, although scale economies do not work for smaller countries [Citation7]. In addition, since vaccines are generally perishable products with relatively long production lead times and numerous regulatory requirements [Citation8], vaccine manufacturers face considerable challenges with respect to making product changes and managing supply chains [Citation4]. Notably, although clinical administration of vaccines and proper supply chain management (after shipment from the manufacturer) depend on others (e.g. health-care systems and vaccine providers), vaccine manufacturers incur the liability and reputation risks for their products. Stakeholders downstream of manufacturers in the vaccine enterprise further expand the market value of vaccines (e.g. health-care systems and providers generate revenues by distributing and administering vaccines to individuals).
The research, development, and commercialization process for vaccines involves many stages and requires a substantial body of evidence to support regulatory decisions, which includes the performance of high-quality randomized controlled trials that demonstrate both safety and efficacy [Citation8]. Only a small fraction of vaccine candidates progress through the extensive, long, and expensive process to gain licensure [Citation9,Citation10]. National regulatory authorities (NRAs) license a vaccine in the country of manufacture for a primary disease target, indication, target population, vaccine formulation, and dose schedule (e.g. [Citation11]). Licensure covers one (or more) specific manufacturing facility(ies) that apply for the license, operate under good manufacturing practice (GMP), and demonstrate consistent production quality to ensure vaccine purity, safety, and potency. In addition, the World Health Organization (WHO) prequalifies some nationally licensed vaccines for use in other countries [Citation12]. Regulatory criteria for licensure and prequalification of vaccines include clear demonstration of individual health benefits (i.e. efficacy) in well-controlled, sufficiently powered, and scientifically valid studies. The comprehensive evidence base must show no substantial risks (i.e. demonstrate safety) for the specific indication and target population [Citation8]. However, NRAs recognize that different identifiable human subpopulations, such as children, the elderly, or immunocompromised individuals, may experience physiologically different responses to a given vaccine product and they consider this during licensure.
In the event of uncertainty about safety, the effectiveness evidence must suggest substantial expected individual benefits that exceed identified potential risks [Citation8], and any safety signals may lead to label restrictions and contraindications [Citation13]. Restriction and contraindications for individuals with identified clinical and/or demographic characteristics are generally based on the concept that while the vaccine should be licensed due to expected overall net benefits for the specific target population and indicated use, some individuals (in the restricted or contraindicated group) may incur adverse consequences that outweigh their potential benefits. Overall, the tolerance for potentially harmful effects of vaccines remains extremely low due to their preventive use in healthy people (and predominantly children).
Once regulators grant a license for a vaccine, decisions by public health agencies to adopt and use the vaccine will likely consider recommendations made by national immunization technical advisory groups (NITAGs) (e.g. [Citation14]) and/or the WHO Strategic Advisory Group of Experts on Immunization (SAGE) [Citation15]. NITAGs generally consider the incidence of the target disease and threat posed by the pathogen, which affect the benefit-risk evaluation for the vaccine product for their specific population. Varying burdens of disease and other factors lead to real differences in risks and benefits of specific vaccine products for different populations, and countries rationally establish and maintain different national vaccine policies and schedules. For example, licensed oral poliovirus vaccine (OPV) and Bacillus Calmette–Guérin (BCG) remain foundational in national immunization schedules in many countries despite their lack of use for the general population other countries (e.g. the US, UK, and other high-income countries). Current recommendations in the US contraindicate live vaccines in individuals with ‘generalized malignant neoplasm, transplantation, immunosuppressive or radiation therapy … depending on the immune status,’ in individuals with B lymphocyte deficiencies, T lymphocyte deficiencies, phagocytic deficiencies (undefined or accompanied by defects in T-cell and NK cell dysfunction), and HIV/AIDS [Citation16].
While vaccines are developed, evaluated, and licensed for their primary disease target (i.e. antigen-specific or pathogen-specific effects), like any pharmaceutical product, vaccines may also lead to secondary health impacts (harmful or beneficial). For any beneficial effects for secondary disease targets, licensure would require ‘repurposing’ (or ‘repositioning’ or ‘rescue’) [Citation17]. Notably, the active agent in BCG vaccine has been repurposed as a therapeutic agent (i.e. intravesical BCG) for the treatment of non-muscle invasive bladder cancer [Citation18], and studies continue to explore the use of some vaccine active agents as drugs for oncolytic virotherapy (e.g. [Citation19]). Arguably, off-label use of licensed vaccines for a secondary disease target could potentially play a role during an emerging pandemic before the availability of disease-specific vaccines or therapies [Citation17,Citation20]. However, large-scale off-label use of licensed vaccines remains unlikely given the limitations in vaccine supply and potential medicolegal consequences in the absence of documented efficacy (particularly for no-longer-licensed vaccines [Citation21]). Ultimately, the actual vaccine use of any vaccine product depends on the values and preferences of private and public purchasers, and considerations of both real and perceived risks, costs, and benefits of the vaccine compared to the alternatives, including doing nothing or using of other products.
1.2. Role of health economic analyses and motivation for this review
Health economists, and other policy and risk analysts, perform studies to support vaccine use decisions for various stakeholders by characterizing health and economic outcomes. These studies depend on evidence of specific effects, and health economists review and integrate the available evidence from multiple fields. Well-performed health economic analyses can help to ensure consistent use of definitions and assumptions and will highlight inconsistent uses by different stakeholders. Poorly performed and nontransparent health economic analyses can contribute to confusion. Despite guidelines that seek to standardize terms, methods, and input assumptions for health economic analyses and ensure transparency about these (e.g. [Citation22]), considerable variability exists, in part due to differences in scope and framing. Increasing focus by health economists on quantifying the full benefits of vaccines using dynamic models over the last 20 years (e.g. [Citation23–25]) includes recognition of ‘indirect’ effects [Citation26,Citation27]. For example, indirect effects include the prevention and/or reduction of adverse health outcomes for individuals other than vaccine recipients (e.g. protection due to ‘population immunity’ or ‘herd immunity’ (e.g. [Citation23,Citation28,Citation29]) and other externalities (e.g. [Citation30]). A recent health economic framework proposed classifications of indirect effects as ‘internal’ or ‘external’ to the intervention recipient, and included ‘internal – biological – non-specific effects’ and ‘external – health systems – side effects’ in the framework [Citation27]. However, in addition to using the term ‘side effects’ in a different way than commonly understood, that framework [Citation27] did not address issues related to immunological classification of the effects in terms relevant to clinical immunologists or basic scientists, and did not explicitly consider the information obtained as part of vaccine pharmacovigilance (e.g. vaccine-associated adverse events).
Increased focus on understanding the mechanisms of action of vaccines and immunological classification of these prompted a recent workshop, which began a discussion of clinical, scientific, economic and regulatory issues related secondary vaccine effects [Citation31]. The lack of standardized terminology and broadly accepted definitions within and across fields create substantial challenges for analysts seeking to quantify health and economic impacts of human vaccines [Citation32–40] This motivated us to identify these different fields and their definitions and concepts, and to specify our definitions and key concepts in Section 2. We then performed a systematic review of quantitative health economic analyses of secondary vaccine effects and discussed insights in the remaining sections.
2. Key concepts and definitions
Economic analyses depend on high-quality evidence and can only quantify risks, costs, and benefits well for specific effects demonstrated by evidence, which leads to the need to evaluate and integrate evidence from multiple parallel fields. summarizes some definitions from several relevant fields, and highlights the fact that definitions and usage vary within and across fields. also provides our definitions for the terms that we use in this review to clearly define our focus.
Table 1. Definitions of some terms associated with different fields/domains and our definitions for terms related to defining economics of secondary vaccine effects
2.1. Clinical immunology/basic science
We begin with the clinical immunology terminology of primary vaccine effects (PVEs) and secondary vaccine effects (SVEs) from a recent workshop of subject-matter experts [Citation31]. shows the components of the immune system associated with PVEs and SVEs. PVEs represent activation of the antigen-specific (or pathogen-specific) systemic or local adaptive immunity subsequent to the administration of a vaccine (, right panel). As such, PVEs represent the classical immunobiology of vaccines as the inducers of memory in the adaptive immune system. SVEs represent the set of all other immunological responses to a vaccine that are not a direct consequence of the antigen-specific (or pathogen-specific) activation of the adaptive immunity (, left panels). In the existing literature, SVEs are also variably known as heterologous, off-target, pathogen agnostic, non-antigen specific, nonspecific, or non-specific effects [Citation31]. Classification of specific observed health outcomes requires evidence, knowledge, or assumptions about immunological mechanisms of action to assign them to PVEs related to adaptive immunity (i.e. antigen-specific activation of B and T lymphocytes) or SVEs related to activation and/or training of the innate immunity or a combination of multiple pathways () [Citation31]. For example, protection from measles morbidity and mortality are the PVEs for the measles vaccine, and reduction in all-cause mortality in children who have received the measles vaccine suggests potential SVEs of the measles vaccine [Citation32].
Figure 1. Schematic overview of the underlying mechanisms for primary and secondary vaccine effects. Primary vaccine effects (PVEs) are almost exclusively under the umbrella of the adaptive immunity. As shown in the right panel, these effects are mediated through antigen-dependent activation of naïve B and T cells, ultimately resulting in production of antibodies by mature plasma cells, as well as the development of CD4+ helper and CD8+ cytotoxic T cells. Memory B and T cells (not shown) expedite production of activated B and T cells during subsequent exposures to the same antigen. The immunological underpinnings of Secondary Vaccine Effects (SVEs) are not as well established, but emerging evidence points largely to activation and/or training of the antigen-independent innate immunity. Activation of the innate immunity (middle panel) is complex and involves multiple systems, cell types, and chemical signals, a review of which is beyond the scope of this manuscript. Most importantly, the emerging field of the trained immunity (left panel) is thought to be the reason behind the enhanced immune response to various pathogens observed after administration of a specific vaccine. Innate immune memory is best described for cells of the myeloid lineage, including the bone marrow progenitors, through epigenetic modifications, metabolic reprogramming and enhanced cytokine production, collectively giving the innate immune system a so-called ‘defensive’ posture against pathogens. A minor component of SVEs may also be mediated secondarily through the adaptive immune system because of antibody cross-reactivity or antigen-mimicry.
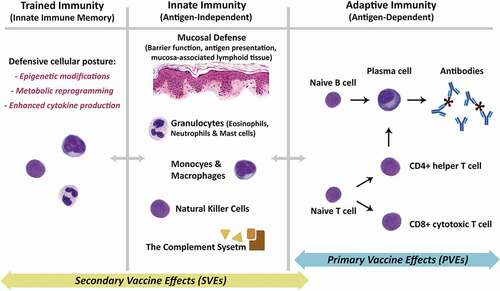
The relationships between individual mechanisms of action and specific health outcomes are often uncertain and variable (for individuals and over time) and can be complex. Specifically, while some mechanisms of action for some diseases and vaccines are well-known, other mechanisms or immunological pathways are unknown or uncertain. In particular, mechanisms of action for SVEs remain an active area of research [Citation31]. Uncertainties about mechanisms of action are further complicated by real differences in genetics, environment, and other factors that affect immunological development and responses in any given vaccine recipient (a concept referred to as ‘personalized vaccinology’ [Citation33]). These differences may lead to observed or expected inter-individual variability in outcomes (e.g. individuals with specific immunodeficiencies may interact differently with pathogens and vaccines than immunocompetent individuals) and intra-individual variability in outcomes as a function of time (e.g. immune immaturity in the neonatal period, waning of immunity after antigen exposure, and immunosenescence with age).
2.2. Patient safety and pharmacovigilance
As noted above, recognizing the potential for real differences in patient- and population-based outcomes, NRAs and NITAGs consider vaccine safety. For vaccines to receive and maintain licensure, vaccines must be proven safe, and any clinically significant signals or symptoms generally must occur with much lower probability and/or severity following receipt of the vaccine compared to the experience of infection. After licensure, manufacturers, regulators, policy makers, and clinicians seek to identify potential side effects that are temporally associated with receipt of a vaccine, and to evaluate any that may pose harm. Serious adverse events (e.g. life-threatening or resulting in significant morbidity) that occur with a significantly higher probability in vaccinated individuals compared to unvaccinated individuals can lead to (additional) contraindications or restrictions for the usage of the vaccine in a specific population [Citation13]. With respect to interpretation of the available evidence, the signal for some effects may be below detection levels in clinical trials and some epidemiological studies due to study design and/or small sample sizes. Clinical trials and field studies may also focus on the net efficacy in trial participants or net effectiveness of the intervention in the field without consideration of the mechanisms leading to the observed overall outcome. Thus, actionable evidence of some rare effects may only become discernable after sufficient numbers of individuals receive the vaccine (e.g. intussusception risk associated with Rotashield™ [Citation34], vaccine-associated enhanced disease [Citation35]). For live vaccines, for which the mechanisms of vaccine action (and therefore clinical signs and symptoms) can mimic the target pathogen that they are intended to prevent, this may imply some adverse events similar to the target disease. For example, measles vaccine may induce fever and rash, and in very rare instances, OPV may cause vaccine-associated paralytic polio (VAPP) [Citation36,Citation37].
In spite of significant international efforts to standardize terminology and definitions [Citation38,Citation39], NRAs, researchers, medical and scientific journals, and others continue to use inconsistently defined terms. Overall, the evolution of the patient safety and pharmacovigilance fields created a legacy of numerous definitions for relevant terms (e.g. side effects, adverse (drug) reactions, adverse events, etc.) used ambiguously and interchangeably by some (). For example, the common term of ‘side effects’ may imply only harm to some, whereas for others it implies both harmful and beneficial effects. We do not use these terms due to issues with terminology ( does not include entries in the rightmost column).
Post-licensure, vaccine pharmacovigilance programs continue to monitor and track adverse events following immunization (AEFI) according to WHO guidelines [Citation38]. By definition, AEFIs describe observed unintended temporally associated harmful effects, and are not necessarily causality linked to the vaccine or immunization. The designation of an outcome as an AEFI also does not require association with an immunological mechanism of action. Not surprisingly and by design, AEFIs generally remain unclassified as either PVEs and/or SVEs, because the focus is on the temporal association of the observed outcomes in patients independent of mechanisms of action and without attribution of causality. In addition, upon identification of clinically or policy actionable AEFIs, the evidence may refer to the specific clinical outcome (e.g. VAPP) and not specifically call these out as AEFIs or include AEFI as a searchable term. Finally, the process of delivering and administering vaccines may itself pose some risks that would not exist but for the vaccines, which may lead to vaccine-associated adverse events not related to the active agent in the vaccine. For example, administration of some vaccines may cause injury (or immunization errors) due to reconstitution errors [Citation48], contamination [Citation49], and/or injection errors [Citation50]. Although some may not recognize these as a potential source of AEFIs, we include them in our definition of AEFIs, which we view as consistent with a broad interpretation of the WHO definition of the term (). Consideration of all vaccine-related adverse events (independent of linkage to the biological contents of the vaccine) may play an important role in vaccine injury compensation programs (e.g. [Citation51]). We also propose a definition for beneficial effects following immunization (BEFIs) in , which also represent unintended effects, to contrast with the pharmacovigilance focus that by definition is on harmful effects.
2.3. Epidemiology (observational evidence, including incidental findings)
Although AEFIs are a well-defined concept and tracked through a systematic program of vaccine licensure and stewardship, no such systematic program exists for tracking and reporting all observed unexpected or unintended events after vaccination. Results from epidemiological and observational studies, including incidental findings from natural experiments and clinical trials, spurred a large body of literature of potential SVEs with increasing use of the term ‘non-specific effects’ (with or without the hyphen). Over the past decade, these efforts led to multiple attempts at recognition and coordination through the WHO [Citation32,Citation40,Citation52,Citation53]. However, continued lack of consensus in terminology, definitions, and tracking methodologies, and major limitations of the existing evidence base leads to its use for hypothesis generation only and not for policy or decision making [Citation31,Citation32,Citation40,Citation52–54]. A key contributing issue is that the immunological basis of SVEs is not well understood, although SVEs can potentially be explained by training of the innate immunity, cross-reactivity of the adaptive immunity and/or various other host–microbiome interactions () [Citation31]. Notably, in contrast to observations of potential beneficial SVEs, assertions of potential harmful SVEs have led to substantial investments (i.e. time and money) in studies to test hypotheses, particularly for hypotheses that threaten the use of a vaccine for its primary disease target. The substantial investments in well-controlled, sufficiently powered, and scientifically valid studies that debunk hypotheses of harmful potential SVEs or nonspecific effects of vaccines (e.g. [Citation55–60],) represent an underappreciated vaccine enterprise cost.
2.4. Health economics
Existing health economic guidelines (e.g. [Citation22]), check lists (e.g. [Citation61]), and frameworks (e.g. [Citation27]) seek to address the lack of standardization. Some guidelines for economic analyses recognize the potential importance of including AEFIs [22, see section 5.2.2], and some health economic analyses recognize AEFIs in vaccine-specific analyses (e.g. [Citation62–64]). Several systematic reviews [Citation65–67] reported the consideration of AEFIs in health economic analyses (e.g. 5 studies that included immunization side effects or nonspecific effects [Citation65], 52 studies that included AEFIs for influenza vaccines [Citation66], and 14 studies [Citation37,Citation68–80] that characterized VAPP for OPV [Citation67]). Notably, the systematic review with the broadest framing [Citation65] included only one study identified by each of the other systematic reviews [Citation66,Citation67]. This most likely resulted from the limited scope of the search, which did not include AEFIs or specific AEFIs (e.g. VAPP) in the search terms. Although AEFIs may play a relatively small role in the overall health and economic impacts for most licensed vaccines [Citation65,Citation66], some AEFIs have substantially affected the licensure, recommendations, and use for some vaccines (e.g. VAPP for OPV, intussusception for Rotashield™ [Citation34]).
Motivated by the recent workshop on SVEs [Citation31], we performed a systematic review of quantitative health economic studies that specifically focused on SVEs, which we refer to as health economics of SVEs (eSVEs). Despite advances in current technology, we recognize the challenges of classifying all of the specific health outcomes associated with pathogens, diseases, and the vaccines that target them with specific mechanisms of action [Citation31]. We start by defining the health economics of primary vaccine effects (ePVEs) to ensure transparent distinction between ePVEs and eSVEs. We define ePVEs to include any and all health outcomes associated with the pathogen with sufficient evidence for quantification and that may be prevented or reduced by vaccine use as intended for its primary disease target and all quantifiable associated AEFIs and BEFIs. Thus, by definition, we set aside the issue of whether the mechanisms of action of AEFIs and BEFIs would lead to their classification by clinical immunologists as PVEs or SVEs and include all health outcomes with sufficient evidence for quantification associated with a vaccine and its administration for its primary disease target as ePVEs. We evaluate eSVEs as any unintended health outcomes characterized by the health economic study authors as related to SVEs (and include implied SVEs due to ‘non-specific’ effects of the vaccine).
3. Systematic literature review
3.1. Process
A perspective published in March 2021 indicated awareness of only one eSVE [Citation81]. We performed a systematic review to identify all quantitative health economic analyses of SVEs (i.e. eSVEs) published in the English language through 1 November 2021. summarizes the process we followed and records identified at each step, and provides the specific search terms for database queries. We searched both PubMed™ and Web of Science™ databases for publications focused on human vaccines and/or immunizations (Query #1). We then used Query #2 to capture records with any reference to health economics, economic evaluations, and cost(s) combined with benefit(s) or effect(s) or effectiveness to identify records with quantitative health economic analyses. Combining the results of queries #1 and #2, we identified potential quantitative health economic analyses of vaccines. We then executed query #3 to identify records with any reference to nonspecific, off-target, heterologous, pathogen-agnostic, or unintended effects. After combining queries #1, #2 and #3, we applied filters to exclude records identified as ‘Other Animals’ (i.e. not humans) and to include only English language records to obtain the eSVEs for human vaccines for each database.
Figure 2. Search strategy, exclusions and eligibility criteria used to identify quantitative health economic analyses of secondary vaccine effects (eSVEs) through 1 November 2021.
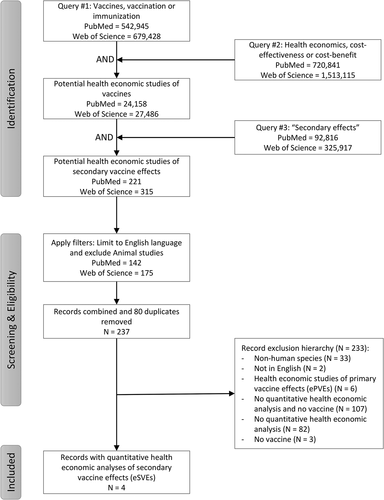
Table 2. Detailed literature search strategy to retrieve records related to quantitative health economic evaluations of vaccine secondary effects
We combined the records from both databases and removed the duplicates. We then reviewed the titles, abstracts and/or full texts of the final 237 records identified to find papers that provided quantitative estimates of health and economic costs, effectiveness, and/or benefits characterized by the study authors as SVEs or effects other than for the target disease. With the a priori knowledge that the majority of putative SVEs for humans relate to 4 specific vaccines (i.e. BCG, OPV, MMR and DTP) [Citation32,Citation52,Citation81], we also verified that adding these as specific search terms did not change the records we obtained.
Consistent with different types of evidence of eSVEs, economic analyses can vary from simplistic screening studies to full quantification of risks, costs, and benefits to support policy decisions related to using vaccines for SVEs (or supplemental to the primary target effects). In general, screening studies seek to qualitatively inform discussions about further exploration and investment of resources often relying on hypothetical bounding assumptions. However, given their scope, screening studies come with substantial limitations and they generally do not limit their estimates to the high-quality evidence that would be required by NRAs, NITAGs, or other public decision makers for action. In contrast, full economic analyses intended to support public policy should perform rigorous assessments of the quality of the evidence base, and transparently include inputs and assumptions based on high-quality evidence (i.e. well-controlled, randomized controlled trials performed specifically to document the effectiveness of the vaccine or well-controlled, highly-powered and replicated epidemiological studies). Recognizing the different motivations for economic analyses, we characterized the scope and nature of the quantitative health economic analyses for eSVEs that we identified in our search.
3.2. Findings
Review of the 237 unique records identified in our systematic search identified 4 published eSVE studies [Citation21,Citation82–84]. Of the 233 papers that did not meet the inclusion criteria, we identified 6 papers that provided quantitative health economic analysis results related to ePVEs [Citation85–90]. shows reason for exclusion of the remaining 227 excluded records in the hierarchical order we used (top to bottom in the exclusion box). Notably, among the excluded papers, some provided frameworks or reviews [Citation27,Citation65], some did not find sufficient evidence to support the inclusion of SVEs in economic analyses (e.g. [Citation59,Citation91]), some mentioned but did not quantify potential detrimental SVEs (e.g. [Citation54]), and some suggested the need for the consideration of the broader effects of the disease targeted by a vaccine (e.g. [Citation92–94]).
The earliest of the 4 health economic analyses that met the inclusion criteria as a eSVE, published in 2000, presented a screening analysis for ‘illustrative purposes only’ that used multiple simplifying assumptions (e.g. 100% coverage, no consideration of vaccine administration costs, effectiveness based on overall mortality reduction estimates that arguably includes both PVEs and potential SVEs) [Citation82]. The analysis suggested substantial potential economic impacts of achieving universal coverage with vaccines in Papua New Guinea due to SVEs [Citation82]. The second study assumed that a dose of measles vaccine led to a 46% reduction in all-cause mortality risk in Guinea-Bissau for a period of 4 years [Citation83]. This study concluded that the inclusion of all-cause mortality (instead of measles mortality alone) provided a better measure of the ‘true effect’ of measles vaccine due to potential SVEs [Citation83]. The framing of this cost-effectiveness analysis could be interpreted as quantifying the additional benefits associated with preventing the mortality associated with the wide range of sequelae induced by measles infections, which differs from a focus on SVEs [Citation95,Citation96]. Both of these two analyses [Citation82,Citation83] lack estimates of effectiveness for specific SVEs that differ from and unambiguously exclude the ePVEs.
The third study quantified the health and economic impacts of reintroducing OPV into the US population in response to COVID-19 [Citation21], which had been proposed as an intervention based on SVEs [Citation97]. The analysis did not find sufficient evidence to support any quantification of effectiveness for COVID-19 prevention by OPV or any non-polio benefits associated with giving OPV to US population in 2021 [Citation21]. The analysis highlighted the end of all use of OPV in the US in 2000 due to the risks of VAPP, which provides a notable example of a vaccine removed from the market. In the US, the risks of using OPV became unacceptable by the year 2000 following a decade in which VAPP represented the only reported cases of polio (see in [Citation98]), and immunization with inactivated poliovirus vaccine (IPV) did not come with this risk [Citation37,Citation99]. As such, no vaccine manufacturers offered licensed OPV for use in the US after 2000, which made it unavailable for any clinical use or stockpiling [Citation80] and made the option of using it in 2020 for COVID-19 response infeasible [Citation21]. Based on the review of the available evidence related to OPV effects on SVEs relevant to the US population, the analysis estimated 0 quantifiable health benefits for the intervention [Citation21]. However, using prior knowledge and evidence of AEFIs for OPV, that analysis estimated of on the order of 1–41 expected cases of VAPP that could occur with OPV use in the US in 2020 for COVID-19, depending on the type of OPV used [Citation21]. The analysis also estimated net financial costs on the order of $4.4 billion (US$2019) [Citation21]. Thus, this analysis found that not reintroducing OPV in the US dominated, and showed no justification for repurposing OPV in the US for COVID-19 [Citation21].
The final included eSVE evaluated the cost-effectiveness of disregarding the restrictive vial-opening policy for BCG vaccine in Guinea-Bissau for children aged 0–4 years old considering only tuberculosis mortality and hospitalizations to represent PVEs and all-cause mortality and hospitalizations as an indication of SVEs [Citation84]. The mechanisms of action for BCG to reduce all-cause mortality remain unknown, and because ‘it is unrealistic to assume complete protection against all-cause mortality’ the analysis assumes ‘leaky’ BCG vaccine effectiveness for all-cause mortality [Citation84]. The analysis finds that based on expected tuberculosis mortality and hospitalizations alone, disregarding the restrictive vial-opening policy for BCG vaccine is not cost-effective, but the policy becomes cost-effective for the assumed BCG effectiveness on all-causes of mortality and hospitalization [Citation84].
3.3. Additional observations
Based on arguments made about the potential benefits of SVEs of live virus vaccines for COVID-19 [Citation97], some randomized clinical trials registered in 2020 (e.g. NCT04445428) began testing the hypothesis that OPV would prevent COVID-19 disease, but they did not lead to published results as of late 2021. Similarly, nearly 20 randomized clinical trials began in 2020 to demonstrate SVEs of BCG in preventing and/or treating COVID-19 [Citation100], and none of these led to actionable results as of late 2021. In the meantime, multiple specific vaccines for COVID-19, with safety and efficacy demonstrated by clinical trials, received emergency use authorization in late 2020 and full licensure in 2021 for their PVEs. Not surprisingly, the US did not and does not plan to use OPV in its response to COVID-19. The rapid development of highly effective COVID-19 antigen-specific vaccines made the option of using and testing other vaccines for their SVEs irrelevant for COVID-19.
We also note that due to the licensed use of intravesical BCG as a therapeutic agent for some bladder cancers, our search did not identify health economic analyses related to this use [Citation101,Citation102] as eSVEs. These studies did not characterize the intravesical BCG as a vaccine (or mention ‘vaccine’ or ‘immunization’). The mechanism of action for BCG use as a therapeutic agent to treat bladder cancer involves immunomodulatory effects that could be characterized as SVEs, if the definition of SVEs is broadened to include vaccines as reagents that may be administered in non-vaccine formulations. Nevertheless, both of these economic studies represent cost-effectiveness analyses for the specific intervention of using intravesical BCG for bladder cancer as the primary disease target [Citation101,Citation102], and none of the cost-effectiveness studies for BCG that we reviewed simultaneously considered the benefits of BCG vaccine use for tuberculosis prevention (PVEs) and for therapeutic use of intravesical BCG for some bladder cancer treatments. Given the lack of characterization of intravesical BCG as a vaccine in these studies or their characterization of the effects as SVEs, we did not separately include these as studies of eSVEs. Our interpretation aligns with the concept that SVEs represent outcomes observed with the routine use of the vaccine in an immunization program targeting the primary effect, not any and all potential uses of the biological components of the vaccine.
4. Discussion
Despite the limited number of studies, we identified several issues that complicate health economic analyses for eSVEs, which we categorize according to specific themes. We suggest that these issues could prove important as research on SVEs evolves and as health economists seek to properly characterize them.
4.1. Limited eSVE study scope despite multiple health outcomes
As noted above, vaccines tend to target pathogens responsible for substantial public health burdens of disease with the aim of prevention of clinical illness, hospitalization, and/or death, and in some cases to interrupt infectious disease transmission. As such, infectious diseases targeted by vaccines often involve multiple mechanisms of systemic or organ-specific damage and therefore affect numerous health outcomes (endpoints). For example, measles infection may cause substantial morbidity with a wide range of sequelae (e.g. fever, rash, otitis media, diarrhea, pneumonia, encephalitis, blindness, etc.), and may lead to mortality shortly after illness, or delayed due to comorbidities, immunosuppression, or subacute sclerosing panencephalitis [Citation62,Citation92,Citation96,Citation103].
The health outcomes selected for any quantitative health economic analysis will determine the overall estimated health and economic risks, costs, and benefits attributed to the vaccine. For example, since measles virus may affect multiple biological pathways, involve multiple mechanisms of action, and induce multiple health outcomes, health economic analyses should ideally characterize the effects of measles interventions on all of these outcomes [Citation62,Citation64]. Capturing the full benefits of vaccines requires using health outcome measures such as disability-adjusted-life-years (DALYs) that account for both mortality and morbidity [Citation22]. However, health economic analyses may consider one or more of the most severe health outcome (e.g. only mortality prevention for measles vaccine [Citation104]). In addition, some health economic studies that report outcomes in DALYs may include only mortality, which means that the use of a potentially integrative health outcome metric alone (e.g. DALYs) does not provide sufficient evidence of inclusion of all relevant health outcomes [Citation104]. The framing of single outcome health economic studies may reflect screening or bounding analyses (e.g. studies that focus on quickly evaluating whether adoption of an intervention is warranted due to substantial benefits for the most severe outcome alone). However, studies that focus on mortality benefits from an intervention with short-term follow-up (e.g. [Citation82,Citation83]) will systematically underestimate the benefits, costs, and risks that relate to the wide range and longer-term sequelae associated with significant vaccine-preventable diseases. For example, one modeling study [Citation96] demonstrated that longer term immunomodulation induced by measles infection increases overall childhood infectious disease mortality, not just mortality shortly after infection [Citation92]. As such, previous studies of overall or all-cause mortality that attributed an observed reduction in mortality after vaccination to beneficial SVEs of measles vaccine (e.g. [Citation105]), mostly likely include some of the specific effects of preventing measles infections and all of their sequelae (i.e. ePVEs), instead of or in addition to eSVEs [Citation96]. Similarly, if studies of eSVEs rely on estimates of effectiveness that may include effects unrelated to the infectious disease or vaccine (i.e. neither ePVEs nor eSVEs, like mortality from physical injury), then analysts need to clearly distinguish the effects of eSVEs from those attributable to ePVEs or other non-vaccine related effects. Thus, studies that focus on all-cause or overall mortality (e.g. [Citation82–84]) may misattribute mortality reduction benefits of non-vaccine health interventions to the vaccine, while simultaneously failing to attribute the mortality reduction benefits of the vaccines from their PVEs in the ePVE. We suggest that studies of eSVEs need to separately account for all of the relevant health outcomes, including morbidity and long-term effects, and ensure clear distinction of ePVEs, eSVEs, and other unrelated effects (if any) when interpreting the evidence, or clearly state their framing as a major limitation and subject to bias [Citation32].
4.2. Uncertain mechanisms of action and complications related to measurement of secondary vaccine effects
The nature of evidence related to vaccine mechanisms of action impacts decisions and the characterization of the risks, costs, and benefits of vaccines in health economic analyses. With use and clinical experience, research may reveal variability in human genetics and/or immunity states that explain different interactions and effects for some individuals with respect to vaccines (e.g. differential roles of mechanisms of action due to functional pathways or enzymes). When evidence demonstrates identifiable risk factors for potential adverse effects of vaccines, then this may lead to the listing of counterindications for the vaccines [Citation13,Citation16]. However, the nature and quality of the evidence for potential SVEs as of 2021 (e.g. small and highly selected cohorts, variable findings between and within studies dependent on analysis groups, and insufficient prospective testing of specific hypotheses) limit their actionability [Citation31,Citation32,Citation40,Citation52–54]. Actionability on potential SVEs will and should require the collection of confirmatory evidence (e.g. reproducible, well-controlled, sufficiently powered, and scientifically valid population-specific studies (e.g. [Citation55–60])) prior to the consideration of regulatory and policy changes by NRAs, NITAGs, and public health authorities.
As evidence evolves for the existence of clinically significant SVEs, health economists will need to increasingly consider these in health economic analyses. Recent evidence suggests that such effects might operate through the induction of innate immune responses, which may lead to lasting changes through metabolic and epigenetic programming of myeloid cells (, left panel). The existing literature particularly calls out the induction or activation of innate immunity or various other forms of ‘boosting’ or ‘training’ the immune system (e.g. [Citation106,Citation107]). As research leads to improved characterization of variability in human responses to vaccine, analysts may need to explicitly consider both variability and uncertainty to support vaccine risk management [Citation108]. Improved understanding of how vaccines work may help to better target vaccine recommendations (e.g. [Citation109]) and development, so research that identifies immunological risk factors linked to specific mechanisms of action may prove clinically actionable. Targeting vaccines (a precision medicine concept) may help minimize individual risks and maximize individual benefits, although added complexity may increase health system and vaccine use costs and decrease overall coverage.
4.3. Incomplete accounting for the full value of vaccines
Health economic modeling can support characterization of the full benefits of vaccines, if framed for that purpose (e.g. for polio vaccines see [Citation37,Citation72,Citation110]). All analyses that seek to quantify the broad or full health and economic impacts of vaccines should consider all of the relevant health outcomes affected and sufficiently supported by evidence. This becomes a necessity for economic analyses that seek to characterize SVEs. This may change if and when an existing vaccine becomes repurposed or repositioned for deployment under an alternative indication (e.g. a therapeutic drug), depending on the interpretation of that use as either vaccine-related or not (e.g. BCG). (As highlighted above, we did not include intravesical BCG as a vaccine, but other studies may do so.)
Discussions of nonspecific effects of vaccines may reflect the tendency of some vaccine effectiveness studies and health economic analyses to insufficiently account for the full health benefits of vaccines, in part due to exclusive focus on a single high-impact health endpoint (e.g. mortality or hospitalization) and their exclusion of other endpoints (e.g. morbidity). We suggest that any analyses that report potential SVEs, including observational studies, should also simultaneously report or estimate both the PVEs and any outcomes attributable to other causes that may also be affecting the population (e.g. other interventions that affect baseline mortality). We further suggest that the totals for each attributable component need to sum to the overall total, and that when summed, these cannot exceed the total incidence of the observed outcome. Full accounting for all health outcomes and benefits of vaccines may eliminate many issues raised by observational studies that have suggested potential SVEs.
Discussions of beneficial SVEs remains dominated by observational studies (‘natural experiments’), which prior systematic reviews characterized as at high risk of bias [Citation32,Citation52]. As concluded in one systematic review, ‘the quality of the evidence, however, does not provide confidence in the nature, magnitude, or timing of nonspecific immunological effects after vaccination with BCG, diphtheria, pertussis, tetanus, or measles containing vaccines nor the clinical importance of the finding’ [Citation52]. In addition, controlled studies that explored hypotheses raised about beneficial nonspecific effects related to the timing and order of vaccines have not supported these hypotheses in high-income countries [Citation59], just as they did not support claims of adverse nonspecific effects [Citation57,Citation58]. As one excluded study from our systematic review suggests, issues of either unique characteristics of studied population in Guinea-Bissau and/or bias in the statistical methods used also warrant consideration [Citation111]. The limited current understanding of mechanisms of action of SVEs suggests that their inclusion in economic analyses should not be expected in the near future, since rigorous analyses will likely not find sufficient evidence of effectiveness in the general population for their consideration [Citation54,Citation112]. We suggest that more attention to the potential beneficial effects of SVEs would help health economists better capture these, such that ePVEs could actually include both AEFIs and BEFIs.
Overall, we find that the ambiguity of proposed SVEs implies a low bar for evidence, which makes them non-actionable clinically, and not a topic generally considered in quantitative health economic analyses. Although observations and reporting of potential SVEs, particularly those proliferated in popular or social media, may persuade some fraction of the population, evidence that falls short of the quality and type expected for the primary vaccine licensure will not likely satisfy the evidentiary requirements for consideration by vaccine manufacturers, regulators, health systems, or purchasers. In the absence of sufficiently powered, prospective, and randomized controlled trials that demonstrate specific effects of vaccines for endpoints that differ from the ePVEs, we see no basis for the inclusion of the consideration of potential SVEs in most health economic analyses. In the event that secondary mechanisms of action for vaccine emerge as clinically important, then the formal process of repurposing the vaccine (or its active agent) for those specific uses offers a better option than promoting unquantifiable and nonspecific secondary benefits. Historically, our collective failure to identify and reject low-quality, poorly-controlled, non-reproducible studies and data highlights the treacherous landscape of ‘secondary effects’ for medical interventions, including vaccines and other pharmaceutical products. Since only evidence of specific effects is actionable, we suggest that all use of the term ‘non-specific effects’ (with or without the hyphen) should stop so as to avoid unintended consequences (e.g. misperceptions of vaccine risks, increases in vaccine hesitancy).
4.4. Potential interactions between uses of the vaccine for different disease targets
The inclusion of eSVEs in economic analyses for some vaccines may add some complexity, depending on the nature of the effect and disease target. Specifically, the significance and role of the health endpoints associated with the secondary effects depends on the relationships between the health outcomes for the disease targets and indications for use. The distinctions between ‘primary’ and ‘secondary’ disease targets for ePVEs and eSVEs must be clear. As noted above, our search did not identify health economic analyses related to the use of intravesical BCG [Citation101,Citation102] as eSVEs. Thus, health economists who seek to characterize the full values of specific active agents used in vaccines may need to consider more than one disease target, particularly for emerging oncolytic virotherapies. When the vaccine health outcomes and vaccine indication for an SVE differ completely from the ePVEs, then this would likely require a new license for the indication, and economic analyses for the disease targets could be performed completely independently, as occurs now for BCG.
Alternatively, if the health outcomes differ for the disease targets but use the same dose schedule in the same target populations, then this could mean that economists need to account for additional benefits. One theoretical example in a country that already uses licensed OPV in campaigns to control polio, OPV campaigns could potentially benefit from the slowing of transmission of a similar pandemic virus due to SVEs. Presumably, the benefits of these campaigns for polio already warrant their performance, so any potential benefits associated with SVEs for the pandemic virus could potentially increase the benefits at no additional costs. However, performing OPV campaigns during a pandemic could potentially increase the chances of spread of the pandemic virus, which could lead to net overall harm [Citation21,Citation113]. Health economists evaluating the use of vaccines for pandemics need to consider the dynamics of the emerging disease, and the effects of non-pharmaceutical interventions and specific vaccines that target the pandemic pathogen [Citation114,Citation115].
In the event that the health outcomes and indications for use of a vaccine for all PVEs and SVEs for different disease targets align completely, then this may mean that use of the vaccine for the secondary disease target would not change the nature of vaccine use, but it would still require licensure for the specific indication. The addition of strains to an existing vaccine or creating a combination vaccine from two existing licensed vaccines differ from this concept, but represent somewhat close concepts. The current portfolio of vaccines includes a number of combination formulations, which reflects the preferences of manufacturers, health systems, parents, and vaccine recipients to minimize the number of injections and required immunization contacts. The licensure of combination formulations requires vaccine manufacturers to demonstrate the safety and efficacy of the combined product in clinical trials, with noninferior efficacy of each component of the combination vaccine relative to the individual component vaccines. The trend of increased licensure and use of combination vaccines, along with simultaneous administration of multiple vaccines during a single visit, allowed for sharing of administration costs and supported simpler management of vaccine supply chains. However, with the shift to combination vaccines, options for giving single-component vaccines remain limited, and making changes to immunization schedules represents a costly endeavor.
Finally, if the use of the vaccine for a secondary disease target changes its use for the primary disease target, then health economists may need to consider these effects. For relatively minor effects associated with a secondary disease target, the resource investments required to support repurposing may not likely develop, so economic analyses for the primary disease target may need to consider off-label use of for a secondary disease target as an externality. As demonstrated by one of the studies in our review [Citation21], economic analyses for repurposing a no-longer-licensed vaccine may be simplified by not needing to consider PVEs of the vaccine and may benefit from any evidence from prior evidence of AEFIs (or BEFIs).
4.5. Real differences between developed vs developing countries
Discussions of SVEs should recognize that real differences exist in vaccine risks, costs, and benefits for developed and developing countries. These differences occur due to different disease burdens, severity of endpoints, treatment, vaccines used, costs, risks, liability, markets, values and preferences, incentives and other factors, which can lead to justifiably divergent recommendations for the use of specific vaccines (e.g. [Citation116]) and other health interventions (e.g. [Citation117]). For example, in some countries the high incidence of diseases such as yellow fever, cholera, or tuberculosis means that vaccines that target these diseases represent good options for adoption and use, because they provide health benefits that exceed the risks and with reasonable economic trade-offs. However, other countries with low incidence of the same diseases will neither need nor want these vaccines (except perhaps for travelers going to the high incidence areas). In addition, the benefits of vaccines may vary with respect to outcomes in specific populations. For example, greater baseline mortality and morbidity risks may change the importance of comorbidity outcomes [Citation95]. Similarly, in populations in which individuals with primary immune deficiencies survive beyond infancy (e.g. in developed countries), live virus vaccines (e.g. OPV and BCG) pose significantly greater relative risks than in populations in which these individuals do not survive. Finally, countries consistently demonstrate different preferences with respect to investments in health. Wealthier countries spend greater fractions of wealth on health (e.g. much higher percentages of gross domestic product on health services) [Citation118], and are much more likely to make substantial investments in research and development of specific vaccines and other health interventions than to rely on secondary effects of existing tools.
These differences play out in complex and imperfect vaccine market (section 1.1), for which economic incentives matter. Incentives for repurposing vaccines for secondary disease targets and other innovations include new licensure and the associated market protection, potential market share, and price premiums. We suggest that health economic analyses for SVEs will need to be population specific.
5. Expert opinion
Vaccines have been historically developed, studied and used for their primary antigen-specific effects, but there has been increasing recent interest in potential SVEs. Building on a recent workshop that focused on clinical and immunological opportunities and challenges of SVEs, our finding of a few quantitative health economic analyses for secondary vaccine effects (or eSVEs) suggests a signal of increasing interest in research on SVEs. However, key challenges to increased consideration of SVEs in health economic analyses remains insufficient understanding of the mechanisms of secondary action for vaccines and the lack of clinically actionable evidence. Increasing research in this area may improve the evidence based related to the mechanisms of action for vaccines and our understanding of reasons for variability in human immunological responses that may support improved targeting for the development of candidates for new vaccines and/or therapies. However, improved understanding will require careful accounting that separates eSVEs from economics of primary vaccine effects (or ePVEs), particularly for diseases characterized by a broad clinical spectrum of sequelae. We expect that some research related to mechanisms of action for vaccines may provide insights that better explain some ePVEs for diseases with multiple health endpoints.
A key limitation in our review is the lack of standard terminology and definitions in the field of SVEs. We made an attempt to address this issue by reviewing the existing definitions and clarifying our definitions. However, the lack of standardized terminology will continue to create challenges with respect to interpretation and use of evidence from different fields and studies. This issue can become an even larger problem when addressing a potential causal relationship between vaccines and SVEs, a topic that is beyond the scope of our review, but critical in future clinical and basic science studies of SVEs.
Research related to SVEs as a public health measure may create some additional challenges for clinicians. The concept of immunomodulation for therapy or disease prevention in specific scenarios is not new or unique to vaccines. However, managing pharmaceutical immunomodulation as a public health intervention requires rigorous clinical validation and proof of safety, efficacy, and effectiveness in the same manner as routine vaccine development. Studies that promote the public health benefits of SVEs must account for the nature of individual risks or the concepts of ‘potential harm’ and ‘preventable harm.’ In clinical practice, we strive not to treat individuals with modalities that pose preventable or even potential harms to the individual, unless the benefits clearly outweigh the risks to the individual. For example, multiple studies have demonstrate beneficial effects of cigarette smoking on ulcerative colitis (UC), likely through some form of immunomodulation (reviewed in [Citation119]). Despite these convincing positive findings with respect to smoking, clinical guidelines for UC do not recommend smoking to individuals who have UC, to those with genetic predisposition to UC, or to the general population to prevent UC [Citation120]. Here, an intervention that clearly produces a positive or beneficial ‘secondary effect’ poses a preventable potential harm to individuals and/or the society at large, so an intervention such as smoking will ever be acceptable as a modality to address a public health concern, even in the context of devastating global pandemic. Thus, the expectations for licensure and for public health policy include substantial evidence of specific benefits for all exposed individuals, and not theoretical potential nonspecific benefits for some individuals within the population.
With ongoing research on genetics and the specific mechanisms of action for vaccines, we may see increasing opportunities to target vaccines only to individuals who will benefit and prevent immunization of individuals who face risks. Recognition of this variability serves as the underlying premise of precision or personalized medicine. However, this would potentially lead to challenges for the development, testing, licensing, production, and/or management of vaccine supply chains, which imply higher product and system costs. In addition, as messages about differential vaccine risks and benefits emerge, this may affect use, purchase, and/or post-market experience and lead to decreased immunization coverage due to vaccine hesitancy as individuals worry more risks. Thus, while more information about mechanisms of action of vaccines may help clinicians to maximize individual benefits, this may come at the cost of greater market segmentation, higher product and system costs, and increased requirements for managing information about vaccine benefits and risks. For example, increased awareness of variability in human immunology may lead to more complicated public health messages related to vaccines (e.g. everyone should get a polio vaccine, but individuals with primary immunodeficiencies should only get IPV). Any increased segmentation of the market would likely imply more products each with smaller market share. These trends are largely in favor of increasingly specific vaccines and other therapeutics, and are not likely to support investment in nonspecific interventions.
Research that focuses on SVEs could potentially lead to the identification of new specific indications for existing (or obsolete) vaccines. If the mechanism of action relevant to the secondary effect leads to endpoints with health benefits that exceed those associated with the primary target, then these may lead to licensure and commercialization of the repurposed vaccine. However, repurposing a vaccine can change the anticipated market size (e.g. much smaller markets for cancer therapeutics than for general population vaccines). Understanding the mechanisms of action for vaccines may also inform the development of targeted therapeutic (non-vaccine) agents that can mimic the immunological activity induced by vaccines [Citation121]. We question whether pursuit of a better vaccine for tuberculosis should strive to provide both non-inferior effectiveness compared to BCG vaccine and non-inferior effectiveness for intravesical administration for bladder cancer treatment. We suspect that it may be better to decouple these clinical endpoints and identify separate superior specific products for a new tuberculosis vaccine and for treatment of bladder cancer.
As investigation into SVEs progresses, we must recognize that real unintended consequences can arise with increased visibility of potential SVEs, particularly if referred to as ‘non-specific’ or ‘off-target’ effects. Messages that suggest nonspecificity of a vaccine effect will increase public concern about inadequate quality of the evidence base supporting vaccine licensure and recommendations. Although this is in sharp contrast with the actual regulatory and review requirements for specific and high-quality evidence, public perception of these processes should not be put at risk. Current politicization of vaccines and the amplification of any discussions of vaccine risks/uncertainty by social media may contribute to the erosion of trust in the system and to the increase in vaccine hesitancy [Citation122,Citation123]. We need to avoid amplification of perspectives and analyses that undermine the system by implying effects that we cannot explain, and focus on specific, clinically actionable evidence, good economic and risk analyses, and clear communication.
Article highlights
Different disciplines use inconsistent and conflicting definitions and concepts that affect vaccine health economic analyses
Additional research on mechanisms of action of vaccines may improve attribution and accounting for their full benefits, risks, and costs
Health economic analyses for some vaccines may not quantify the full risks, costs, or benefits of the vaccine
Repurposing vaccines for secondary uses may complicate health economic analyses for their primary use
Health economic analyses for vaccines require careful interpretation of supporting evidence and transparent accounting and assumptions
Declaration of interest
The authors have no relevant affiliations or financial involvement with any organization or entity with a financial interest in or financial conflict with the subject matter or materials discussed in the manuscript. This includes employment, consultancies, honoraria, stock ownership or options, expert testimony, grants or patents received or pending, or royalties.
Reviewer disclosures
Peer reviewers on this manuscript have no relevant financial or other relationships to disclose.
Author contributions
Both authors participated equally in all elements of this review.
Acknowledgments
We presented some aspects of this work at a closed workshop hosted by the National Institute of Allergy and Infectious Diseases (NIAID), part of the U.S. National Institutes of Health (NIH), July 27-29, 2021, and we thank the participants of that workshop for helpful comments and discussion.
Additional information
Funding
References
- Shulman J, Ahsan R, O’Malley K, 2021. Understanding global vaccine economics and research and development. [cited 2021 Jun 21]. Available from: https://www.sabinaspengroup.org/app/uploads/2021/01/sabin-aspen-report-2021-shulman-3.pdf
- Roush SW, Murphy, TV, The Vaccine-Preventable Disease Table Working Group, et al. Historical comparisons of morbidity and mortality for vaccine-preventable diseases in the United States. JAMA. 2007;298(18):2155–2163.
- Zhou F, Shefer A, Wenger J, et al. Economic evaluation of the routine childhood immunization program in the United States, 2009. Pediatrics. 2014;133(4):577–585.
- Thompson KM, Orenstein WA, Hinman AR. An opportunity to incentivize innovation to increase vaccine safety in the United States by improving vaccine delivery using vaccine patches. Vaccine. 2020;38(25):4060–4065.
- UNICEF. 2021. Vaccines UNICEF supply division. [cited 2021 Sep 9]. Available from: https://www.unicef.org/supply/vaccines
- Pan American Health Organization. 2021. PAHO revolving fund. [cited 2021 Sep 9]. Available from: https://www.paho.org/en/revolvingfund
- Taylor P, 2011. Dutch government sells off vaccine facility. [cited 2021 Sep 9]. Available from: http://www.pharmafile.com/news/149629/netherlands-vaccine-institute-bilthoven-sale
- Baylor NW. The regulatory evaluation of vaccines for human use. Methods Mol Biol. 2016;1404:773–787.
- Watson M, Faron de Goër E. Are good intentions putting the vaccination ecosystem at risk? Hum Vaccin Immunother. 2016;12(9):2469–2474.
- Francis DP. Successes and failures: Worldwide vaccine development and application. Biologicals. 2010;38(5):523–528.
- Food and Drug Administration. 2021. Vaccines licensed for use in the United States. [cited 2021 Sep 9]. Available from: https://www.fda.gov/vaccines-blood-biologics/vaccines/vaccines-licensed-use-United-States
- World Health Organization. 2021. [cited 2021 Sep 9]. List of prequalified vaccines. Available from: https://extranet.who.int/pqweb/vaccines/list-prequalified-vaccines
- Centers for Disease Control and Prevention. Update: vaccine side effects, adverse reaction, contraindications, and precautions – recommendation of the Advisory Committee on Immunization Practices (ACIP). MMWR Morb Mortal Wkly Rep. 1996;45(RR–12):1–35.
- Centers for Disease Control and Prevention, 2021. ACIP vaccine recommendations and guidelines. [Cited 2021 Sep 9]. Available from: https://www.cdc.gov/vaccines/hcp/acip-recs/index.html
- World Health Organization, 2021. WHO vaccine position papers. [cited 2021 Sep 9]. Available from: https://www.who.int/teams/immunization-vaccines-and-biologicals/policies/position-papers
- Kroger A, Bahta L, Hunter P. 2021. General best practice guidelines for immunization. Best practices guidance of the Advisory Committee on Immunization Practices (ACIP), updated 4 May 2021. [cited 2021 Jun 21. Available from: https://www.cdc.gov/vaccines/hcp/acip-recs/general-recs/index.html
- Naylor A, Kauppi D, Schonfeld J. Therapeutic drug repurposing, repositioning and rescue part II: business review. Drug Discovery World.2015 (Spring) .57–72. .
- Fuge O, Vasdev N, Allchorne P et al, et al. Immunotherapy for bladder cancer. Res Rep Urol. 2015;7:65–79.
- Zhand S, Hosseini SM, Tabarraei A, et al. Oral poliovirus vaccine-induced programmed cell death involves both intrinsic and extrinsic pathways in human colorectal cancer cells. Oncolytic Virother. 2018;7:95–105.
- Chakraborty C, Sharma AR, Bhattacharya M, et al. Ongoing clinical trials of vaccines to fight against COVID-19 pandemic. Immune Netw. 2021;21(1):e5.
- Thompson KM, Kalkowsa DA, Badizadegan K. A health economic analysis for oral poliovirus vaccine to prevent COVID-19 in the United States. Risk Anal. 2021;41(2):376–386.
- World Health Organization. 2019. WHO guide for standardization of economic evaluations of immunization programmes, Edition II, October 2019. Initiative for Vaccine Research, Department of Immunization, Vaccine, and Biologicals: Geneva.
- Edmunds WJ, Medley GF, Nokes DJ. Evaluating the cost-effectiveness of vaccination programmes: a dynamic perspective. Stat Med. 1999;18(23):3263–3282.
- Beutels P. Economic evaluations of hepatitis B immunization: a global review of recent studies (1994-2000). Health Econ. 2001;10(8):751–774.
- Bos JM, Alphen L, Postma MJ. The use of modeling in the economic evaluation of vaccines. Expert Rev Pharmacoecon Outcomes Res. 2002;2(5):443–455.
- Silver MC, Neumann PJ, Ma S, et al. Frequency and impact of the inclusion of broader measures of value in economic evaluations of vaccines. Vaccine. 2021;39(46):6727–6734.
- Nymark L, Vassall A. A comprehensive framework for considering additional unintended consequences in economic evaluation. Cost Eff Resour Alloc. 2020;18(1):27.
- Scarbrough Lefebvre CD, Terlinden A, Standaert B. Dissecting the indirect effects caused by vaccines into the basic elements. Hum Vaccin Immunother. 2015;11(9):2142–2157.
- Nymark LS, Sharma T, Miller A, et al. Inclusion of the value of herd immunity in economic evaluations of vaccines. A systematic review of methods used. Vaccine. 2017;35(49 Pt B):6828–6841.
- Andrews JR, Baker S, Marks F, et al. Typhoid conjugate vaccines: a new tool in the fight against antimicrobial resistance. Lancet Infect Dis. 2019;19(1):e26–e30.
- Joffe AM, Park E-C, Augustine A, et al. NIAID workshop on secondary vaccine effects. Nat Immunol. 2021;22(11):1363–1366.
- Higgins JP, Soares-Weiser K, López-López JA, et al. Association of BCG, DTP, and measles containing vaccines with childhood mortality: systematic review. BMJ. 2016;355:i5170.
- Poland GA, Ovsyannikova IG, Kennedy RB. Personalized vaccinology: a review. Vaccine. 2018;36(36):5350–5357.
- Centers for Disease Control and Prevention. Suspension of rotavirus vaccine after reports of intussusception–United States, 1999. MMWR Morb Mortal Wkly Rep. 2004;53(34):786–789.
- Munoz FM, Cramer JP, Dekker CL, et al. Vaccine-associated enhanced disease: case definition and guidelines for data collection, analysis, and presentation of immunization safety data. Vaccine. 2021;39(22):3053–3066.
- Duintjer Tebbens RJ, Pallansch MA, Kew OM, et al. Risks of paralytic disease due to wild or vaccine-derived poliovirus after eradication. Risk Anal. 2006;26(6):1471–1505.
- Thompson KM, Duintjer Tebbens RJ. Retrospective cost-effectiveness analyses for polio vaccination in the United States. Risk Anal. 2006;26(6):1423–1440.
- Council for International Organizations of Medical Sciences/World Health OrganizationDefinition and application of terms for vaccine pharmacovilance. [cited 2012 9 Sep]. Available from: https://cioms.ch/wp-content/uploads/2017/01/report_working_group_on_vaccine_LR.pdf
- World Health Organization, Causality assessment of an adverse event following immunization (AEFI): user manual for the revised WHO classification, 2nd ed., 2019 update. [cited 2021 9 Sep]. Available from: 2019 https://www.who.int/publications/i/item/causality-assessment-aefi-user-manual-2019
- World Health Organization, 2019. Non-specific effects of vaccines: questions and answers. [cited 2021 Sep 9]. Available from: https://www.who.int/immunization/research/implementation/NSE_questions_answers_March2019.pdf
- Management Sciences for Health and World Health Organization. 2007. Drug and therapeutics committee training course. [cited 2021 Sep 9]. Available from: https://www.who.int/medicines/technical_briefing/tbs/Participant-s-Guide-All-Sessions.pdf
- Food and Drug Administration, 2021. Finding and learning about side effects (adverse reactions). [cited 2021 Sep 9]. Available from: https://www.fda.gov/drugs/information-consumers-and-patients-drugs/finding-and-learning-about-side-effects-adverse-reactions
- World Health Organization. 2013. Vaccine safety basics learning manual. [cited 2021 Sep 9]. Available from: http://vaccine-safety-training.org/tl_files/vs/pdf/vsb-manual.zip
- World Health Organization. 1972. International drug monitoring: the role of national centres, technical report series 498. [cited 2021 Sep 9]. Available from: https://www.who-umc.org/media/2680/who-technical-report-498.pdf
- Centers for Disease Control and Prevention. 2021. Preventing and managing adverse reactions. [cited 2021 Sep 9]. Available from: https://www.cdc.gov/vaccines/hcp/acip-recs/general-recs/adverse-reactions.html
- Centers for Disease Control and Prevention. 2021. Understanding adverse events and side effects. [cited 2021 Sep 9]. Available from: https://www.cdc.gov/vaccinesafety/ensuringsafety/sideeffects/index.html.
- Code of Federal Regulations. 2020. Title 21 - food and drugs chapter 1 - Food and drug administration department of health and human services subchapter D - drugs for human use. [cited 2021 Sep 9]. Available from: https://www.accessdata.fda.gov/scripts/cdrh/cfdocs/cfcfr/cfrsearch.cfm?fr=312.32
- Cousins S. Contaminated vaccine deaths a serious setback for Syria. Lancet. 2014;384(9949):1172.
- Fortin J. 2017. Mishandled measles vaccine kills 15 children in South Sudan. [cited 2017 Jun 2]. Available from: https://www.nytimes.com/2017/06/02/international-home/vaccines-south-sudan.html
- Atanasoff S, Ryan T, Lightfoot R, et al. Shoulder injury related to vaccine administration (SIRVA). Vaccine. 2010;28(51):8049–8052.
- Thompson KM, Orenstein WA, Hinman AR. Performance of the United States Vaccine Injury Compensation Program (VICP): 1988-2019. Vaccine. 2020;38(9):2136–2143.
- Kandasamy R, Voysey M, McQuaid F, et al. Non-specific immunological effects of selected routine childhood immunisations: systematic review. BMJ. 2016;355:i5225.
- World Health Organization. 2014. Evidence based recommendations on non-specific effects of BCG, DTP-containing and measles-containing vaccines on mortality in children under 5 years of age. [cited 2021 Sep 9]. Available from: https://www.who.int/immunization/sage/meetings/2014/april/1_NSE_Backgroundpaper_final.pdf?ua=1
- Conklin L, Hviid A, Orenstein WA, et al. Vaccine safety issues at the turn of the 21st century. BMJ Glob Health. 2021;6(Suppl 2):e004898.
- Taylor B, Miller E, Farrington C, et al. Autism and measles, mumps, and rubella vaccine: no epidemiological evidence for a causal association. Lancet. 1999;353(9169):2026–2029.
- Jain A, Marshall J, Buikema A, et al. Autism occurrence by MMR vaccine status among US children with older siblings with and without autism. JAMA. 2015;313(15):1534–1540.
- Miller E, Andrews N, Waight P et al, et al. Bacterial infections, immune overload, and MMR vaccine. Measles, mumps, and rubella. Arch Dis Child. 2003;88(3):222–223.
- Stowe J, Andrews N, Taylor B, et al. No evidence of an increase of bacterial and viral infections following measles, mumps and rubella vaccine. Vaccine. 2009;27(9):1422–1425.
- Andrews N, Stowe J, Thomas SL, et al. The risk of non-specific hospitalised infections following MMR vaccination given with and without inactivated vaccines in the second year of life. Comparative self-controlled case-series study in England. Vaccine. 2019;37(36):5211–5217.
- Tielemans S, de Melker HE, Hahné SJM, et al. Non-specific effects of measles, mumps, and rubella (MMR) vaccination in high income setting: population based cohort study in the Netherlands. BMJ. 2017;358:j3862.
- Watts RD, Li IW. Use of checklists in reviews of health economic evaluations, 2010 to 2018. Value Health. 2019;22(3):377–382.
- Thompson KM, Odahowski CL. The costs and valuation of health impacts of measles and rubella risk management policies. Risk Anal. 2016;36(7):1357–1382.
- Thompson KM, Kalkowska DA. Potential future use, costs, and value of poliovirus vaccines. Risk Anal. 2021;41(2):349–363.
- Thompson KM, Badizadegan ND. Modeling the transmission of measles and rubella to support global management policy analyses and eradication investment cases. Risk Anal. 2017;37(6):1109–1131.
- Nymark LS, Miller A, Vassall A. Inclusion of additional unintended consequences in economic evaluation: a systematic review of immunization and tuberculosis cost-effectiveness analyses. Pharmacoecon Open. 2021;5(1):1–17.
- Fens T, de Boer PT, van Puijenbroek EP, et al. Inclusion of safety-related issues in economic evaluations for seasonal influenza vaccines: a systematic review. Vaccines (Basel). 2021;9(2):111.
- Thompson KM, Kalkowska DA. Review of poliovirus modeling performed from 2000-2019 to support global polio eradication. Expert Rev Vaccines. 2020;19(7):661–686.
- Miller MA, Sutter RW, Strebel PM et al, et al. Cost-effectiveness of incorporating inactivated poliovirus vaccine into the routine childhood immunization schedule. JAMA. 1996;276(12):967–971.
- Thompson KM, Duintjer Tebbens RJ. Eradication versus control for poliomyelitis: an economic analysis. Lancet. 2007;369(9570):1363–1371.
- Duintjer Tebbens RJ, Pallansch MA, Kew OM et al, et al. Uncertainty and sensitivity analyses of a decision analytic model for post-eradication polio risk management. Risk Anal. 2008;28(4):855–876.
- Thompson KM, Tebbens RJD, Pallansch MA, et al. The risks, costs, and benefits of possible future global policies for managing polioviruses. Am J Public Health. 2008;98(7):1322–1330.
- Duintjer Tebbens RJ, Pallansch MA, Cochi SL, et al. Economic analysis of the global polio eradication initiative. Vaccine. 2011;29(2):334–343.
- Duintjer Tebbens RJ, Pallansch MA, Cochi SL, et al. An economic analysis of poliovirus risk management policy options for 2013-2052. BMC Infect Dis. 2015;15(389). doi:https://doi.org/10.1186/s12879-015-1112-8
- Thompson KM, Duintjer Tebbens RJ. Health and economic consequences of different options for timing the coordinated global cessation of the three oral poliovirus vaccine serotypes. BMC Infect Dis. 2015;15(374). doi:https://doi.org/10.1186/s12879-015-1113-7
- Duintjer Tebbens RJ, Thompson KM. Uncertainty and sensitivity analysis of cost assumptions for global long-term poliovirus risk management. J Vaccines Vacc. 2016;7(5):339.
- Duintjer Tebbens RJ, Thompson KM. Comprehensive screening for immunodeficiency-associated vaccine-derived poliovirus: an essential OPV cessation risk management strategy. Epidemiol Infect. 2017;145(2):217–226.
- Duintjer Tebbens RJ, Thompson KM. The potential benefits of a new poliovirus vaccine for long-term poliovirus risk management. Future Microbiol. 2016;11(12):1549–1561.
- Duintjer Tebbens RJ, Thompson KM. Costs and benefits of including inactivated in addition to oral poliovirus vaccine in outbreak response after cessation of oral poliovirus vaccine use. Med Decis Making Policy & Practice. 2017;2(1):1–13.
- Tucker AW, Isaacs D, Burgess M. Cost-effectiveness of changing from live oral poliovirus vaccine to inactivated poliovirus vaccine in Australia. Aust N Z J Public Health. 2001;25(5):411–416.
- Jenkins PC, Modlin JF. Decision analysis in planning for a polio outbreak in the United States. Pediatrics. 2006;118(2):611–618.
- Chumakov K, Avidan MS, Benn CS, et al. Old vaccines for new infections: exploiting innate immunity to control COVID-19 and prevent future pandemics. Proc Natl Acad Sci U S A. 2021;118(21):e2101718118.
- Shann F. Immunization–dramatic new evidence. P N G Med J. 2000;43(1–2):24–29.
- Byberg S, Fisker AB, Thysen SM et al, et al. Cost-effectiveness of providing measles vaccination to all children in Guinea-Bissau. Glob Health Action. 2017;10(1):1329968.
- Thysen SM, Fisker AB, Byberg S et al, et al. Disregarding the restrictive vial-opening policy for BCG vaccine in Guinea-Bissau: impact and cost-effectiveness for tuberculosis mortality and all-cause mortality in children aged 0-4 years. BMJ Glob Health. 2021;6(8):e006127.
- Arnal JM, Frisas O, Garuz R, et al. Cost effectiveness of hepatitis A virus immunisation in Spain. Pharmacoecon. 1997;12(3):361–373.
- Chodick G, Lerman Y, Peled T et al, et al. Cost-benefit analysis of active vaccination campaigns against hepatitis A among daycare centre personnel in Israel. Pharmacoeconomics. 2001;19(3):281–291.
- Dye C. Making wider use of the world’s most widely used vaccine: bacille Calmette-Guerin revaccination reconsidered. J R Soc Interface. 2013;10(87):20130365.
- Waye A, Chuck AW, Jacobs P et al, et al. Prevnar 7 childhood immunization program and serotype replacement: changes in pneumococcal incidence and resulting impact on health care costs in Alberta (2003-2008). Drugs Real World Outcomes. 2015;2(2):153–161.
- Flasche S, Jit M, Rodríguez-Barraquer I, et al. The long-term safety, public health impact, and cost-effectiveness of routine vaccination with a recombinant, live-attenuated dengue vaccine (Dengvaxia): a model comparison study. PLoS Med. 2016;13(11):e1002181.
- Sandmann F, Ramsay M, Edmunds WJ et al, et al. How to prevent vaccines falling victim to their own success: intertemporal dependency of incidence levels on indirect effects in economic reevaluations. Value Health. 2021;24(10):1391–1399.
- Biggart R, Finn A, Marlow R. Lack of impact of rotavirus vaccination on childhood seizure hospitalizations in England - An interrupted time series analysis. Vaccine. 2018;36(31):4589–4592.
- Duke T, Mgone CS. Measles: not just another viral exanthem. 2003;361(9359):763–773.
- Arnoux S, Weinberger C, Gessner BD. Vaccine-preventable influenza disease burden from clinical trials of Vaxigrip - an inactivated split virion influenza vaccine - supports wider vaccine use. Vaccine. 2007;25(45):7720–7731.
- Jit M, Newall AT, Beutels P. Key issues for estimating the impact and cost-effectiveness of seasonal influenza vaccination strategies. Hum Vaccin Immunother. 2013;9(4):834–840.
- Cooper WO, Boyce TG, Wright PF, et al. Do childhood vaccines have non-specific effects on mortality? Bull World Health Organ. 2003;81(11):821–826.
- Mina MJ, Metcalf CJE, de Swart RL, et al. Long-term measles-induced immunomodulation increases overall childhood infectious disease mortality. Science. 2015;348(6235):694–699.
- Chumakov K, Benn CS, Aaby P, et al. Can existing live vaccines prevent COVID-19? Science. 2020;368(6496):1187.
- Thompson KM. Good news for billions of children who will receive IPV. Lancet Infecti Dis. 2015;15(10):1120–1122
- Centers for Disease Control and Prevention. Poliomyelitis prevention in the United States. Updated recommendations of the advisory committee on immunization practices. MMWR Morb Mortal Wkly Rep. 2000;49(RR–5):1–22.
- Gonzalez-Perez M, Sanchez-Tarjuelo R, Shor B, et al. The BCG vaccine for COVID-19: first verdict and future directions. Front Immunol. 2021;12:632478.
- Uchida A, Yonou H, Hayashi E, et al. Intravesical instillation of bacille Calmette-Guérin for superficial bladder cancer: cost-effectiveness analysis. Urology. 2007;69(2):275–279.
- Bachir BG, Dragomir A, Aprikian AG, et al. Contemporary cost-effectiveness analysis comparing sequential bacillus Calmette-Guerin and electromotive mitomycin versus bacillus Calmette-Guerin alone for patients with high-risk non-muscle-invasive bladder cancer. Cancer. 2014;120(16):2424–2431.
- Perry RT, Halsey NA. The clinical significance of measles: a review. J Infecti Dis. 2004;189(Supplement_1):S4–S16
- Thompson KM, Odahowski CL. Systematic review of health economic analyses of measles and rubella immunization interventions. Risk Anal. 2016;36(7):1297–1314.
- Aaby P, Bhuiya A, Nahar L et al, et al. The survival benefit of measles immunization may not be explained entirely by the prevention of measles disease: a community study from rural Bangladesh. Int J Epidemiol. 2003;32(1):106–116.
- Agrawal B. Heterologous immunity: role in natural and vaccine-induced resistance to infections. Front Immunol. 2019;10:2631.
- Marín-Hernández D, Nixon DF, Hupert N. Heterologous vaccine interventions: boosting immunity against future pandemics. Mol Med. 2021;27(1):54.
- Thompson KM, Graham JD. Going beyond the single number: using probabilistic risk assessment to improve risk management. Hum Ecol Risk Assess. 1996;2(4):1008–1034.
- Shukla R, Ramasamy V, Shanmugam RK et al, et al. Antibody-dependent enhancement: a challenge for developing a safe dengue vaccine. Front Cell Infect Microbiol. 2020;10:572681.
- Thompson KM, Kalkowska DA. An updated economic analysis of the global polio eradication initiative. Risk Anal. 2021;41(2):393–406.
- Bollaerts K, Verstraeten T, Cohet C. Observational studies of non-specific effects of Diphtheria-Tetanus-Pertussis vaccines in low-income countries: assessing the potential impact of study characteristics, bias and confounding through meta-regression. Vaccine. 2019;37(1):34–40.
- Thompson KM. Is introduction of IPV “Good news for billions of children”?–Author’s reply. Lancet Infect Dis. 2016;16(4):410.
- Thompson KM, Kalkowsa DA, Badizadegan K. No role for reintroducing OPV into the United States with respect to controlling COVID-19 [Response to the letter to the editor by Chumakov et al.]. Risk Anal. 2021;41(2):389–392.
- Thompson KM, Kalkowska DA, Badizadegan K. Hypothetical emergence of poliovirus in 2020: part 1. Consequences of policy decisions to respond using nonpharmaceutical interventions. Expert Rev Vaccines. 2021;20(4):465–481.
- Thompson KM, Kalkowska DA, Badizadegan K. Hypothetical emergence of poliovirus in 2020: part 2. Exploration of the potential role of vaccines in control and eradication. Expert Rev Vaccines. 2021;20(4):449–460.
- Thompson KM, Duintjer Tebbens RJ. National choices related to inactivated poliovirus vaccine, innovation, and the end game of global polio eradication. Expert Rev Vaccines. 2014;13(2):221–234.
- Badizadegan K, Vanlandingham DM, Hampton W et al, et al. Value of biopsy in a cohort of children with high-titer celiac serologies: observation of dynamic policy differences between Europe and North America. BMC Health Serv Res. 2020;20(1):962.
- Ochalek J, Claxton K, Lomas J, et al. Valuing health outcomes: developing better defaults based on health opportunity costs. Expert Rev Pharmacoecon Outcomes Res. 2021;21(4):729–736.
- Thomas T, Chandan JS, Li VSW et al, et al. Global smoking trends in inflammatory bowel disease: a systematic review of inception cohorts. PLOS ONE. 2019;14(9):e0221961.
- Rubin DT, Ananthakrishnan AN, Siegel CA, et al. ACG clinical guideline: ulcerative colitis in adults. Am J Gastroenterol. 2019;114(3):384–413.
- Hajjo R, Tropsha A. A systems biology workflow for drug and vaccine repurposing: identifying small-molecule BCG mimics to reduce or prevent COVID-19 mortality. Pharm Res. 2020;37(11):212.
- Broniatowski DA, Jamison AM, Qi S, et al. Weaponized health communication: Twitter bots and Russian trolls amplify the vaccine debate. Am J Public Health. 2018;108(10):1378–1384.
- Hotez PJ. Anti-science kills: from Soviet embrace of pseudoscience to accelerated attacks on US biomedicine. PLoS Biol. 2021;19(1):e3001068.