ABSTRACT
Introduction
Toxoplasma gondii (T.gondii) is a widespread protozoan with significant economic losses and public health importance. But so far, the protective effect of reported DNA-based vaccines fluctuates widely, and no study has demonstrated complete protection.
Areas covered
This review provides an inclusive summary of T. gondii DNA vaccine antigens, adjuvants, and some other parameters. A total of 140 articles from 2000 to 2021 were collected from five databases. By contrasting the outcomes of acute and chronic challenges, we aimed to investigate and identify viable immunological strategies for optimum protection. Furthermore, we evaluated and discussed the impact of several parameters on challenge outcomes in the hopes of developing some recommendations to assist better future horizontal comparisons among research.
Expert opinion
In the coming five years of research, the exploration of vaccine cocktails combining invasion antigens and metabolic antigens with genetic adjuvants or novel DNA delivery methods may offer us desirable protection against this multiple stage of life parasite. In addition to finding a better immune strategy, developing better in silico prediction methods, solving problems posed by variables in practical applications, and gaining a more profound knowledge of T.gondii-host molecular interaction is also crucial towards a successful vaccine.
1. Introduction
Toxoplasmosis is a widespread disease caused by opportunistic infection of Toxoplasma gondii (T. gondii), one of the most common pathogenic protozoa in the world. T. gondii infects approximately one-third of the human population on earth [Citation1]. Usually, healthy individuals do not exhibit obvious clinical symptoms but it can cause severe damage to the fetus or immunocompromised patients such as those with Acquired immunodeficiency syndrome (AIDS) [Citation2,Citation3]. In particular, it can cause toxoplasmic encephalitis and retinochoroiditis in humans and abortion in animals [Citation2,Citation3]. Toxoplasmosis is one of the main causes of death in immunocompromised and malignant tumor patients but also it can lead to a devastating effect on the livestock’s health and production, particularly Small domestic ruminants. This can happen as a result of direct loss due to livestock’s death or indirect loss due to reproductive disorders such as stillbirth, abortion, and low surviving rate of the offspring [Citation4–7].
Due to the possible harm of T. gondii, a variety of drug treatments have been proposed. Since Sabin and Warren reported the anti-toxoplasmic effect of sulfadiazine in 1942 and Eyles and Coleman found the synergistic impact of pyrimethamine in 1953, the combination of these two drugs had been considered as the basis for the treatment of Toxoplasmosis [Citation8,Citation9]. In addition, clindamycin, atovaquone, clarithromycin, spiramycin, and azithromycin were also reported as anti-toxoplasmic drugs [Citation9]. However, these medications showed some disadvantages which cannot be ignored, namely, long course of treatment and significant side effects. Moreover, due to the chemical inaccessibility of the blood-brain barrier, drugs are always ineffective in the elimination of the persistent infection of T. gondii in the central nerve system. Hence, due to the current absence of ideal therapeutic drugs, vaccines will be our primary choice for the control of T. gondii.
Many vaccines have been developed to protect against T. gondii infection, including live attenuated, inactivated, subunit, and DNA vaccines. The killed or inactivated vaccine of T. gondii acts by destroying some functions of the parasite through heat or chemical treatment but it was not effective enough in any infection model used [Citation10]. The subunit vaccines stimulate the host’s immune system by exposing antigenic proteins of T. gondii. Existing studies have shown that it lacks immunogenicity, and it is necessary to choose appropriate adjuvants to compensate for this shortcoming [Citation11]. In addition, it has safety problems such as allergic reactions.
Attenuated T. gondii vaccines are now considered the most promising type of vaccine which provides complete protection. Toxovax, the only commercial T. gondii vaccine, is also an attenuated vaccine made from live T. gondii S48 strain to protect susceptible breeding ewes. Attenuated vaccines are often made using life cycle defective mutants, virulence factor deletion mutants, or nutrient auxotrophic mutants. Among them, attenuated vaccines made from auxotrophic mutants are considered more promising [Citation12]. However, although the attenuated vaccine has an excellent immune effect, the risk of reverting back to a complete virulence strain cannot be ignored [Citation10]. Together with the disadvantage of short-shelf life and its inefficiency in protecting against different Toxoplasma strains resulted in labeling it as none an ideal vaccine for wide application.
To avoid the above-mentioned safety issues while trying to achieve a better immune effect, many researchers worked hard towards a more advantageous type of vaccine, the so-called ‘DNA vaccine.’ A great merit of DNA vaccines is that they are highly malleable and can be designed with one or more T. gondii genes at our will. So, in theory, we could design vaccines that elicit more significant Th1 immune responses against T. gondii infection and, hopefully, even tailor a vaccine that brings complete protection against all three life stages of T. gondii [Citation13,Citation14]. Additionally, the DNA sequence of the recombinant plasmid has been thoroughly studied to avoid any potential risks and can be massively produced at a fairly low cost [Citation14]. On top of that, DNA vaccines would cause no allergic reaction, and therefore, the vaccination route can be flexibly adapted. Since they are non-livings, they do not even require cold-chain storage and hence, it could be easier to have them stored and transported [Citation15]. To sum up, in theory, DNA vaccines can achieve better immune effects through purposeful design and avoid safety issues while saving costs in production, storage, and transportation [Citation15].
However, there is still a long way and some challenges to develop a DNA vaccine for T. gondii. So far, there have been many attempts using either one gene alone or multi-gene in combination, but none has shown significantly better outcomes than other vaccines. Moreover, various parameter selections by different studies made the horizontal comparison between studies impossible. Therefore, in this review, while introducing major antigens and addressing the effects of these DNA vaccines, we try to analyze some of the involved parameters, to bring some insights into the DNA vaccine research.
2. Methods
2.1. Information source and search
In this review, with time restrictions set from 2000 to January 2021, five databases (PubMed, Google Scholar, Science Direct, Scopus and Web of Science) were searched for articles discussing the protective efficacy of T. gondii DNA vaccines. We applied the search term ‘Toxoplasma gondii’ and ‘DNA vaccine’ for the search of conventional DNA vaccine studies, and search terms ‘Bioinformatics analysis,’ ‘in-silico,’ ‘Epitope,’ ‘Toxoplasma gondii’ and ‘DNA vaccine’ for studies adapted bioinformatic analysis.
2.2. Study selection and data collection
After searching, all initially included papers were accessed and discerned by authors based on their titles and abstracts. Since we wish to evaluate the impact of parameters on the vaccine’s protective efficacy, those that did not use mice as animal models in parasite challenge trials, papers without clearly stated key variables (such as immunization dose), and papers that did not use plasmids as the vector were excluded. In total, more than 140 results were collected. Required data from these articles, such as antigens, antigen combinations, adjuvants (if applicable), immunization dosage, results, parasite strain and mice strain challenged, were extracted into Excel sheets. These data were then organized into four separate tables, depending on whether the antigens were combined and whether the challenge was acute or chronic (). Only papers with significant results reported were mentioned in the main body, but all collected results can be found in summarizing Tables ().
Table 1. Protective effect of single-gene DNA vaccine against acute infection of Toxoplasma gondiia.
Table 2. Protective effect of single-gene DNA vaccine against chronic infection of Toxoplasma gondiib.
Table 3. Protective effect of multi-gene DNA vaccine against acute infection of Toxoplasma gondiic.
Table 4. Protective effect of multi-gene DNA vaccine against chronic infection of Toxoplasma gondiid.
Table 5. Protective effect of multi-epitope DNA vaccine design base on in silico analysise.
2.3. Data synthesis
To help visualize our results, plots representing protective efficacy on brain cyst reduction, survival extension in days, average survival rate and counts of adapted antigens were produced from date we present in tables. And due to overwhelming variables, no statistical comparison was made on our plots. Plots are made by the software GraphPad Prism v8.0.2.263.
3. Results and discussion
3.1. Antigens
According to the data we gathered., invasion-related proteins, such as Surface antigens (SAGs), Dense granule proteins (GRAs), Rhoptry proteins (ROPs), and Microneme proteins (MICs), are the most often employed antigen options for DNA vaccination studies (). SAGs, MICs and ROPs are directly involved in the invasion [Citation53–60], while GRAs play a role after the invasion [Citation58,Citation59]. Besides these four, there has been an increasing number of studies that attempted metabolism-related proteins, such as Heat shock protein (HSP) and Calcium-dependent protein kinase (CDPK) [Citation61–69], as target antigens for DNA vaccines in recent years.
Figure 1. Illustration of common antigens for T. gondii DNA vaccine. The asterisk (*) indicates commonly used antigens.
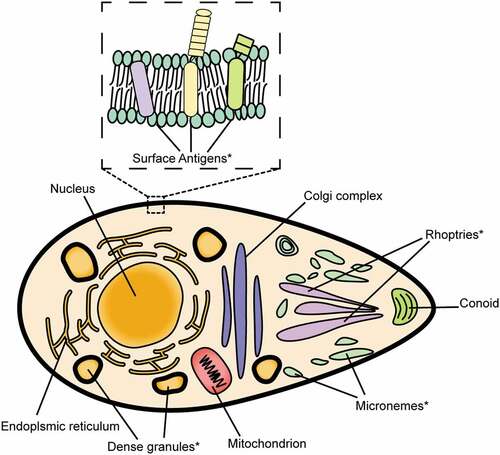
3.1.1. Surface antigens (SAGs)
SAGs are highly expressed in tachyzoites of T. gondii and are considered the first component that makes contact with the host cells during the invasion process. Due to their essential roles in the adhesion and pathogenesis of the parasite, they considered to be promising candidates for toxoplasmosis vaccine design.
Among numerous proteins of the SAG family, SAG1 is the primary surface antigen responsible for the tachyzoites’ host cells invasion [Citation70–72]. Because of its high antigenicity, SAG1 has been used for the development of many types of vaccines [Citation53–55,Citation73]. However, SAG1ʹs effect is not as significant as researchers expected when it is solely administered. Most of the results can only lead to a fewer than 10 days survival extension in acute infection and a less than 35% decrease in brain cyst formation in chronic infection [Citation74–80]. The only successful sole SAG1 immunization trial was recently reported by Liu et al [Citation81]., with a 60% protection rate against RH strain acute infection by co-injecting genetic adjuvant Interleukin-18 (IL-18) to elicit a greater Th1 response. Compared to the sole administration of SAG, the use of SAG1 in combination with other antigens showed relatively better results. Six out of seventeen multi-antigen vaccines adapting SAG1 showed partial life-long protection after the acute challenge, with survival rates ranging from 20% to 87% [Citation7,Citation74,Citation79,Citation82].
In addition to the most well-studied antigen, SAG1, other antigens from the SAG family, SAG2, SAG4 and SAG5 have also been studied for their potential as DNA vaccine antigens. It was reported that sole-gene vaccine adapting SAG2C, SAG2X, or tri-gene vaccine adapting SAG2C+SAG2D+SAG2X could provide more than 70% brain cyst decrease in chronic infection [Citation83]. The sole use of SAG4 brings 66% brain cysts reduction, whereas the combinate use of SAG5B and SAG5C brings 70% brain cysts reduction [Citation84,Citation85].
In sum, SAG1 vaccines can offer only poor protection against either acute or chronic challenges. When SAG1 is inoculated with other antigens (or adjuvants), it results in better protection against acute challenges. SAG2, SAG4 and SAG5, however, can bring relatively better chronic protection, as most of the results reach more than 70% cyst reduction.
3.1.2. Microneme proteins (MICs)
MICs are one of the three apical secretory organelles of T. gondii which play essential roles during the invasion. Nineteen types of MICs have been discovered so far, and they express in both tachyzoite, bradyzoite, and sporozoite [Citation86]. MICs are the first functional secretory organelle during the invasion [Citation58,Citation59]. MICs can easily recognize the host cells and combine with their receptors on the cell surface, facilitating the subsequent adhesion and penetration [Citation56,Citation87]. Moreover, MICs can serve as the escorters for other MIC proteins (e.g. MIC1, MIC3, MIC4) as well as ROP proteins [Citation88–90].
MIC1 and MIC4 are soluble adhesins involved in the parasite’s attachment [Citation88]. Previous research found that they can provide fairly strong protection against acute T. gondii infection. In combination with Freund’s incomplete adjuvant, both can bring up to a 50% survival rate when administered solely and up to a 65% survival rate when co-injected [Citation91]. However, their performance against chronic infections was mediocre, leading to only less than 60 percent brain cyst reduction [Citation91].
MIC2 is a protein involved in the gliding motility of T. gondii and the attachment to the host cell surface [Citation92]. Dautu et al., reported that DNA vaccines made from MIC2 could bring 40% and 37.5% survival rates to immunized BALB/c and C57BL/6 mice, respectively, by gene gun immunization [Citation93].
MIC3 has all the above-mentioned MICs properties and has been reported to have terrific immune reactivity [Citation86,Citation87,Citation94,Citation95]. The protection provided by MIC3 DNA vaccines are less stable than that of the other antigens. Under the condition of single gene immunization without adjuvant, one study had reported a 39% survival rate under 10,000 RH Tachyzoite challenge, while another study had reported only a 6-day prolongation under 1,000 RH Tachyzoite challenge [Citation96,Citation97]. In cocktail immunization with other antigens, 10 and 12 days of prolongation and a 10% survival rate were reported [Citation96,Citation98,Citation99].
MIC6 is the escorter of MIC1 and MIC4 protein which was previously discussed [Citation88]. MIC6 DNA vaccines could provide a relatively good effect in the protection of mice against acute infection. The highest survival rate reported was 40%, while the remaining results were a survival extension of 10–20 days [Citation91]. Moreover, when co-administered with the MIC1 and MIC4 cocktail, the vaccine’s protection can be further enhanced, with 75% of mice surviving the acute challenge and 68% of brain cysts reduction in chronic infection [Citation91]. Other cocktails made from MIC6 can also prolong survival length by 29 days and reduce brain cysts by 65% and 80% [Citation100,Citation101].
MIC8, MIC11, and MIC13 are all proteins involved in the adhesion process. DNA vaccines made from MIC8 and MIC13 resulted in prolonged survival of 6–27 days in acute challenge and a 25–64% cyst reduction in chronic challenge [Citation102,Citation103]. Meanwhile, MIC11 vaccines achieved 17% protection against acute infections [Citation104].
In general, DNA vaccines made from the MIC family have good immunogenicity. MIC types 1/2/3/4/6/11 can all confer lifelong protection against acute infection when solely inoculated. Moreover, when MICs are made into a cocktail vaccine, they can confer excellent results in both acute and chronic challenge trials, with MIC6 showing excellent potential for multi-gene DNA vaccines against T. gondii.
3.1.3. Rhoptry proteins (ROPs)
Rhoptry is also one of the three apical secretory organelles of Toxoplasma with a long, slender, secreting neck. ROPs are closely related to the virulence and pathogenesis of the parasite [Citation57,Citation60]. They participate in the invasion process and are involved in the parasitophorous vacuole (PV) generation [Citation57,Citation105,Citation106]. As it is expressed in most life-cycle stages and can activate classical responses due to its pathogenesis, there is a growing interest in constructing a DNA vaccine base on ROPs and using ROPs as a drug target [Citation107].
ROP1 is a component involved in cell invasion. It promotes parasite invasion and penetration in vitro [Citation60]. ROP1-adapted DNA vaccines can reduce cysts in chronic infections by up to 65% and increase survival rates against acute infections by up to 50% [Citation108,Citation109]. When it was co-injected with GRA7, along with genetic adjuvant Interleukin-12 (IL-12), a 50% survival rate was reported [Citation108].
ROP2, ROP4, ROP5, ROP7, ROP8, and ROP18 all belong to the ROP2 family, and most of them involve the PV’s normal function [Citation60]. Although all of these have been considered for DNA vaccines and quite many results have been reported, only a few were considered promising. ROP2/4/5/7/8 brings only less than a 5-days survival extension and less than 60% cyst reduction in the acute and chronic challenge when they are solely injected [Citation75,Citation110–115]. The only exception comes from ROP8, resulting in a 50% survival rate after a 1,000 RH Tachyzoite challenge [Citation116]. However, in cocktail DNA vaccines, ROP5 and ROP7 have become much more protective as they can bring 75% brain cyst reduction to chronically infected mice [Citation113].
ROP18 is the only antigen that can better protect against acute infection than its ROP2 family kins. In acute challenge trials, a 9 to 48 days survival extension in sole administration, and 12 to 30 days survival extension in cocktail administration were observed [Citation99,Citation115,Citation117,Citation118]. Meanwhile, in the chronic challenge, cocktail vaccines that include ROP18 can bring reductions ranging from 64% to 80% [Citation115,Citation117].
ROP13 is a soluble protein that might be injected into a host cell’s cytoplasm after it is proteolytically digested [Citation60]. It can prolong the length of survival by 49 days in mice challenged with 1,000 RH tachyzoites [Citation119]. On the other hand, ROP16 is a crucial virulence factor that causes the pathological response [Citation119]. DNA vaccines made from ROP16 can lead to a 13–27 days survival length prolongation [Citation120,Citation121].
Interestingly, ROP38, a protein that regulates the expression of host transcription factors and and cell proliferation, can bring pretty good protection against chronic challenges, despite its protection efficacy in the acute challenge being the worst among the ROP family [Citation60,Citation122]. Further, due to the limited sequence variation among strains, the ROP38 gene was thought to be a promising candidate for vaccination against toxoplasmosis [Citation60]. For other antigens (ROP9, ROP21, ROP22, ROP35 and ROP54), please see tables () for more information.
In short, ROP1 and ROP7 are good candidates against acute infections. For chronic infections, except ROP38ʹs sole administration can reach good chronic protection, multiple gene combinations of ROPs were also associated with a high reduction rate of chronic infections.
3.1.4. Dense granule proteins (GRAs)
Same as the two proteins mentioned above, GRAs are also one of the three apical secretory organelles of Toxoplasma and play an essential role in T. gondii’s invasion. However, unlike the other two organelles, GRAs are widely distributed throughout the cell and display their effect during and after the invasion [Citation58,Citation59]. GRAs are mainly involved in the forming of PV. They enhance the parasite’s survival and proliferation rate by acting as the modifier of the PV’s interior environment and are essential compositions of the tubule-vesicular network (TVN) of the membranous structure within the PV [Citation123,Citation124]. Since GRAs are widespread and essential proteins in T. gondii’s invasion, they are considered one of the most promising targets for DNA vaccines.
GRA1 is a protein expressed in both tachyzoite and bradyzoite and was reported to have great physiological importance in host cell invasion [Citation123]. However, DNA vaccines made from GRA1 can only provide no more than 6-day prolongation in acute challenge, and only 31% cyst reduction was observed in chronic challenge [Citation79,Citation98,Citation125]. Similarly, GRA2, GRA5 and GRA8 also displayed low protective effects against acute challenges, with both results reporting less than 4-day prolongation [Citation80,Citation126,Citation127].
GRA4 is a protein that can be found in the lumen of the PV [Citation128]. It would associate with the mature vacuole network later in T. gondii invasion by forming a solid interaction with GRA6, another dense granule protein closely associated with the network [Citation123,Citation129]. Their combination would then interact with GRA2 and is speculated to transport proteins and nutrients in the vacuole [Citation123]. GRA4 and GRA6 had been tried as DNA vaccines as well. DNA vaccines adapting GRA4 had reported a 62% survival rate against acute challenge, while the vaccines adapting GRA6 had also reached a 40% survival rate without adjuvant and 53% survival rate when co-administered with 1% LMS (adjuvant) [Citation7,Citation130].
In GRAs family, GRA7 is the most well-explored protein for its DNA vaccine potential. It would be secreted into the vacuolar network and extensions protruding in the cytoplasm after host cell invasion [Citation123]. In the acute challenge, GRA7 DNA vaccines had led to 4–16 days of survival extension and reached up to 15% survival rate when co-injected with adjuvant IL-12 [Citation77,Citation108,Citation112,Citation120]. In chronic challenges, 55%-80% brain cyst reduction was reported [Citation108,Citation125]. When GRA7 was made into a cocktail DNA vaccine, its efficacy was much enhanced, with up to 50% survival rate and 89% cyst formation reduction [Citation108,Citation125].
DNA vaccines made from the remaining antigens provide only moderate protection. GRA14, GRA16, GRA17, GRA23, GRA24, and GRA25 are all involved in maintaining the function of PV [Citation123]. When they were solely administered, GRA24 showed the best results bringing a 25-day survival extension against acute challenge [Citation131]. Despite, its protection efficacy had improved when they were made into multi-gene vaccines, it only provided no more than 18 days of survival extension and 55% cyst reduction [Citation132]. For more detailed results, please see tables ().
In general, GRA4/6/7 of the GRA family are relatively promising genes. Although the number of genes providing lifelong protection is not as large as MICs, however, GRAs can provide a higher protection rate than the MIC family. GRA4 and GRA6 vaccines can confer a survival rate ranging from 40–64%, which is almost the highest rate of protection achieved among all solely administered vaccines. GRA4 and GRA7 are promising candidates to be made into a cocktail vaccine, leading to 33%-75% survival rate and 63%-89% cyst formation reduction.
3.1.5. T. gondii heat shock proteins (TgHSP)
HSPs were reported to play a role in post-translational processes in T. gondii [Citation62]. HSPs are one of the most conservative genes among organisms, and it has excellent immunogenicity [Citation133]. For instance, HSP70 can induce adaptive and innate immune reactions by interacting with DCs and natural killer cells [Citation134]. Hence, vaccines made from HSPs have been reported to be more effective against chronic infections. The best protection has been achieved by mice inoculation with HSP70 by gene gun immunization, which resulted in a reduction of 75% T. gondii load in mice organs, and up to 60% reduction in 12 weeks P.I, as previously reported [Citation63]. In addition, DNA vaccines made from HSP40 and HSP60 were reported to bring a 62% and 48% reduction of brain cysts in chronic Pru strain infection [Citation61,Citation62]. However, the effect of HSPs on acute infection was not pronounced. The best result was from HSP60, which extended the mice’s survival by 19 days [Citation61].
3.1.6. T. gondii’s calcium-dependent protein kinase (TgCDPK)
CDPK family is a group of enzymes that primarily regulate the body’s calcium signal cascades and gliding motility [Citation66,Citation135–137]. Several studies have adapted CDPKs as DNA vaccines’ antigens, but only moderate protection was reported. In trials against acute and chronic infections, the best protection results both come from CDPK1 vaccines, with 14–20 days of extension and 46%-74% brain cyst reduction [Citation64,Citation138]. CDPK2, CDPK3 and CDPK5, on the other hand, provide only 4–17 days of prolongation and a 39%-54% reduction [Citation65,Citation67,Citation68].
3.1.7. T. gondii’s Rhomboid (TgROM)
Rhomboid proteases belong to the serine protease family and are expressed during the invasion. They were reported to affect the cleavage of MICs and, therefore, influence the immunogenicity and virulence of the parasite [Citation139,Citation140]. DNA vaccines based on ROM4 lead to quite deviated results against acute challenges, with the worst result bringing only about 5 days of prolongation while the best result provides a 20% survival rate [Citation141–143]. As for the ROM5 vaccines, 13.3% of acute challenge protection and 72% of brain cyst reduction were reported [Citation141].
3.1.8. T. gondii apical membrane antigen (TgAMA)
AMA1 is an indispensable part of the moving junction complex (MJ) and is one of the crucial proteins involved in host cell invasion [Citation144,Citation145]. Immunization with the AMA1 gene by gene gun led to 37.5% and 60% survival in the challenged C57BL/6 and BALB/c mice [Citation93].
3.1.9. Cyclophilin of T. gondii (TgCyP)
CyP is believed to be a protein with plenty of functions that expresses within the prokaryotic and eukaryotic cells [Citation146]. In protozoan parasites, it displays the functions of peptidyl-prolyl cis-trans isomerase (PPIase). It can modify and regulate other enzymes, and affect the folding of proteins [Citation147]. A study established by Gong et al., demonstrated that CyP DNA vaccines could bring 37.5% of protection under 500 RH tachyzoite challenge [Citation146]. The authors suggested the high challenge survival rate is due to Cyp’s Interleukin-12 (IL-12) stimulating nature.
3.1.10. T. gondii Profilin (TgPF) & Toxofilin & Nucleoside triphosphate hydrolase-II (NTpase-II)
PF is a protein involved in the parasite’s gliding motility [Citation148,Citation149]. To date, all DNA vaccine studies on PF have focused on chronic infections. While it was solely administered, only a 40–50% of cyst reduction was observed, but when it was added into the ROP16 + ROP18 + MIC6 + CDPK3 vaccine cocktail, it can increase the vaccine’s efficacy from 38 % to 80 % reduction in cyst formation [Citation82,Citation150]. Vaccines made from Toxofilin and NTpase-II were also reported to be effective against chronic challenges. Co-injection of Toxofilin with Alum+MPLA adjuvant could lead to 76% cyst reduction, while NTpase-II itself can lead to 78% cyst reduction [Citation151,Citation152].
3.1.11. In silico analysis
There has been increasing application of bioinformatics analysis in the field of life sciences, and more and more researchers have also applied this new method to help screen antigenic epitopes in DNA vaccine research. Vaccines developed based on the results of this in silico epitope analysis are often referred to as multi-epitope DNA vaccines, harboring characteristics of both traditional DNA vaccines and polypeptide vaccines [Citation153]. Adapting in silico epitope prediction can give us a general picture of the structures and functions of an antigen without risking cultivating the pathogens. And it is less time-consuming and can save us more cost compared to traditional vaccinology research [Citation154]. More importantly, it can also reveal some properties, such as the antigenic variation, genetic polymorphisms, pathogenesis and immune mechanisms of an antigen, and therefore provide us with crucial data to allow us to select and synthesize some of the best candidates into a new vaccine [Citation154].
Immunodominant T and B cell epitope prediction is the primary method used in silico prediction [Citation155]. Accurate identification of both T and B cell epitopes is one of the most critical requirements in constructing a successful vaccine [Citation156]. In T. gondii DNA vaccine research, T and B cell epitope analysis usually refers to linear B-cell prediction, MHC-I prediction and MHC-II prediction in specific [Citation111]. Across numerous studies, alleles used for MHC-I and MHC-II binding epitope prediction are remarkably similar. MHC class I molecules are chosen from the alleles H2-Db, H2-Dd, H2-Kb, H2-Kd, H2-Kk, and H2-Ld, whereas MHC class II molecules are chosen from the alleles H2-IAb, H2-IAd, and H2-IEd. After prediction, predicted epitopes would go through in vivo evaluation and the most immunogenic epitopes will be selected based on the results [Citation157]. These multi-epitope peptides would then be constructed with selected regions for MHC-I, MHC-II, and B-cell epitopes. And eventually, be made into multi-epitope vaccines and evaluated [Citation111].
Theoretically, compared to conventional vaccinology techniques, a correctly developed multi-epitope vaccine expressing T-cell or/and B-cell epitopes would demonstrate a large increase in both cellular and humoral immune responses and further lengthen survival duration [Citation157]. However, in reality, only moderate results of efficacy enhancement were reported. Although almost every in silico study had reported a strong cellular and humoral immune response, only a maximum of 11 days of survival prolongation after acute challenges were observed [Citation158]. A complete list of results can be found in .
In general, all bioinformatic predicted vaccines’ survival prolongation is indistinguishable from traditional vaccinology methods, indicating a limited effect of in silico prediction in optimizing a vaccine’s protection performance. According to Khan et al., in order to achieve a desired outcome, it is imperative to correctly identify both T and B cell epitopes and to choose a potent method of delivering those epitopes [Citation156]. Therefore, in order to ameliorate the situation, future applications will require a better adjuvant, a modified inoculation technique, or a better prediction strategy, particularly in a complex animal model.
3.1.12. Brief summary on antigens
In sum, the results of monogenic DNA vaccine immunization vary widely. When against chronic challenges, vaccines that adapted ROPs can confer the greatest average cyst reduction of all antigens (). For protective effect in against acute challenge, the MIC group are more likely to provide life-long protection () or yield greater prolongation in survival length (), while the GRA group has the highest average survival rate reported (). For more detailed information, please see . And a full list of results can be found in tables ().
Figure 2. Results of protective efficacy of single-antigen DNA vaccine studies, sorted by antigen type. A, Box plot indicating the average brain cyst reduction rate for each antigen group after chronic challenge. B, Bar chart that counts the number of results that achieve partial protection for each antigen group. C, Box plot indicating the average survival extension in days for each antigen group after acute challenge. D, Box plot indicating the average survival rate (if applicable) for each antigen group after acute challenge. MIC = Microneme proteins; SAG = Surface antigen proteins; GRA = Dense granule proteins; ROP = Rhoptry proteins.
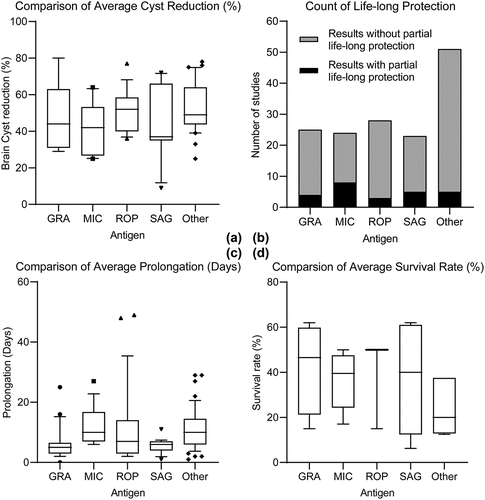
As for multi-gene vaccination, a better result was observed than that of single-gene vaccination. Most genes, when combined with other genes, can work better than they do alone. The best outcomes reported were the combination of SAG1+ GRA4+ Granulocyte-macrophage colony-stimulating factor (GM-CSF) and GRA1+ GRA7, leading to an 87% survival rate in the acute challenge, and 89% cyst reduction in the chronic challenge, respectively. Nevertheless, we found that the high survival outcome is primarily due to the low challenge dose. After sorting out these lower-dose results, we can still notice that the vaccine cocktail GRA7+ ROP1, SAG1+ GRA1, SAG1+ SAG3 and SAG1+ GRA1+ ROP2+ GRA4+ SAG2C+SAG2X performed better in the acute challenge trials [Citation74,Citation79,Citation108,Citation159]. In terms of protection against chronic infection, vaccines that employ SAG2, SAG5, GRA7 and PF can also provide a promising rate of cyst reduction in addition to multi-gene vaccine adapting ROPs mentioned above. A complete list of results can be found in tables ()
3.2. Adjuvants
Since DNA vaccines are often weak in stimulating specific immunity, and their efficacy is always affected by some parameters such as adjuvants.
Currently, there are several ways for adjuvants to augment the DNA vaccine’s immunogenicity. They boost vaccines’ efficacy by enlarging the magnitude and time of DNA expression or by boosting DNA delivery into the host cells or antigen-presenting cells [Citation60,Citation160,Citation161]. Additionally, adjuvants can increase a plasmid’s immunostimulatory qualities by attracting immune cells to the injection area and preventing DNAses from destroying the plasmid [Citation15,Citation60,Citation160–162]. Adjuvants used in T. gondii DNA vaccine can generally be categorized into two groups: genetic adjuvants and conventional adjuvants.
Interleukins are cytokines that stimulate Th1 response in the host. Given that the Th1 immune response is essential for preventing toxoplasma infection, many studies on T. gondii DNA vaccines had adapted interleukins as a genetic adjuvant. IL-12 is one of the most well-studied adjuvants, which enhances Th1 cellular responses and promotes NK cell activity. A study by Quan et al. reported that it could increase the survival rate by 17% in GRA7+ ROP1 multi-gene vaccines and up to 15% in GRA7 single-gene vaccines [Citation108]. However, no significant enhancement was observed in the rest of the studies involving IL-12 [Citation108,Citation110,Citation163,Citation164]. IL-18 is another interleukin that can activate NK cells and enhance Th1-type immunity, increasing the survival rate by 20% when co-administered with SAG1 and leading to a 26% more cyst reduction when co-administered with ROP13 [Citation81,Citation119]. IL-15 and IL-33 had also been co-administered with Profilin and ROP5+ ROP18, respectively, but only moderate enhancements were demonstrated (Profilin: +0 days; ROP5+ ROP18: +7 days) [Citation82,Citation115]. In addition to the sole use of interleukins, there are also some studies using a combination of two interleukins as genetic adjuvants. IL-7+ IL-15 help increases the efficacy of CDPK1, providing an additional 5 days of survival against tachyzoite infection and a 28% more reduction in cyst formation [Citation138]. IL-15+ IL-21 had also shown an enhancement when co-injected with MIC8 and CDPK1, leading to 3 ~ 16 days increase for the acute challenge and a further 27 ~ 39% reduction in cyst formation in the chronic challenge [Citation64,Citation102].
Costimulatory molecule B7-2 (B7-2) is another genetic adjuvant that was adapted by researchers in T. gondii DNA vaccines. It contributed only an additional 5 ~ 6 days of survival under 1,000 RH tachyzoite challenge when co-injected with GRA7, ROP16, and GRA7+ ROP16 [Citation120]. A2/B subunits of cholera toxin (pCTXA2/B) is a potent adjuvant that can induce a significant mucosal immune response. When co-administered with SAG1+ SAG3, an additional 20% of survival in the acute challenge was reported [Citation74]. Hepatitis B surface antigen (HbsAg), a genetic adjuvant made from the hepatitis B virus (HBV), is also promising as it can result in an additional 20% survival rate against acute challenges when co-injected with SAG1+ ROP2 [Citation165].
Except genetic adjuvants, conventional adjuvants have also been employed as co-administered adjuvants for DNA vaccines against T. gondii. Aluminum hydroxide gel (alum), one of the most commonly used adjuvants, was reported to lead to 13 more days of survival in the acute challenge when co-injected with Toxofilin. But alum’s other results were less reported, with either no significant improvement or even less than 5 days of survival rate [Citation76,Citation166]. Calcium Phosphate Nanoparticles (CaP-NPs), another commonly used adjuvant, however, had only displayed a poor enhancement of 0 ~ 1 days prolongation in acute challenge with ROM4 & GRA14 [Citation143,Citation163]. However, Levamisole (LMS), a less commonly used adjuvant, displayed somewhat promising results compared to alum and CaP-NPs. Co-injection of Levamisole with GRA6 lead to an additional 13% survival rate in against acute challenge [Citation130]. For more adjuvants’ detailed results, see tables ().
In sum, genetic adjuvants (IL-12, IL-18, pCTXA2/B and HbsAg) and conventional adjuvants (Levamisole) are of great potential in augmenting the efficacy of T. gondii DNA vaccine. Co-inoculation with plasmids encoding cytokines is one of the finest ways to increase the immunogenicity of DNA vaccines. Because the cytokine is expressed and acts at the site of antigen expression, systemically administered cytokines will have a lower risk of cytokine toxicity [Citation15]. Additionally, there are perks including simplicity, ease of the cloning process, and cost savings [Citation60].
3.3. Other parameters
Besides antigen and adjuvant, other parameters, for instance, route of immunization, mice strain and dosage would all be important factors affecting the outcome of the final survival challenge. Due to these widely differentiated parameter selections in different studies, a horizontal comparison is almost impossible. Here, we briefly review the impact of these parameters on vaccine protection to provide a basis for further future studies.
3.3.1. Route of immunization
Among the results we have collected, most studies have adapted conventional injection methods (i.e. intramuscular, subcutaneous, or intraperitoneal) for immunization. Of these, intramuscular is the most widely used method due to its simplicity and the fact that it confers fairly general immunity, compared to the poor immune outcome from other methods such as s.c and i.p [Citation63]. However, intramuscular injection is not the best method for DNA vaccine delivery. According to previous studies, intramuscular injection causes only mild humoral and cell-mediated immunological reactions because it was reported to be inadequately scattered, ineffectually expressed, and speedily decomposed by lysosomes and DNAses [Citation60,Citation160,Citation167].
To overcome these shortcomings by conventional syringe injection, some novel administration methods have been proposed. The most popular novel approach in the study of a T. gondii DNA vaccine so far has been the gene gun vaccination, and it seems promising. When it comes to producing cytotoxic T lymphocytes (CTL) and specific antibodies in an animal model, gene gun administration is more dependable and repeatable than intramuscular inoculation [Citation168]. Better reproductivity of the results means a more stable effect of immunization and hence, leads to greater potential for future clinical application of DNA vaccination [Citation168]. From the results by Dautu et al., and Mohamed et al., 12.5–40% survival rate in acute challenges and 35–75% cyst reduction in chronic challenges were observed [Citation63,Citation93].
In addition to gene gun vaccination, some other delivery methods, such as electroporation, nanoparticles, microneedles and liposomes, have all shown good potential [Citation162]. But so far, no study has applied them in delivering T. gondii DNA vaccine, and gene gun injection has only been used in single-gene immunization. We believe these methods have great potential and are worth exploring in the future.
3.3.2. Mice strain
Mice are the most frequently used animal model in evaluating the protective effect of a vaccine against acute or chronic T. gondii infection. They were chosen not only because they are easy to manipulate and cheap in maintenance, but also because their immunology was well-studied and their genome has high homogeneity to that of humans [Citation60,Citation169,Citation170].
BALB/c, C57BL/6 and C3H/HeJ are three commonly used strains in vaccine studies. BALB/c were considered naturally resistant to T. gondii, and always observed a relatively lower cyst burden and higher survival rate than C57BL/6 and C3H/HeJ [Citation171,Citation172]. The decrease in the prevalence of brain cysts and encephalitis in mice is mediated by the Ld gene in the D region of the H2 complex. Mice that carries the b or k allele (C57BL/6 and C3H/HeJ) would experience a more serious encephalitis during the chronic T. gondii infection, whereas BALB/c mice with d allele would not [Citation169,Citation173]. Additionally, BALB/c mice have a propensity for a Th2 type response, as evidenced by higher IgG1 titers following vaccination and higher IL-4 production following infection, whereas a more prominent Th1 response was seen in C3H/HeJ mice [Citation174].
Unlike the low susceptibility nature of BALB/c, C3H/HeJ and C57BL/6 were considered to have intermediate and high susceptibilities, respectively [Citation171,Citation175]. C3H/HeJ mice were reported to synthesize a significantly higher concentration of IFN-γ and IL-2 in response to immunization than C57BL/6 mice. However, many challenged mice would still succumb due to the parasite proliferation despite the higher IFN-γ expression [Citation174].
As for C57BL/6, they had a higher mortality rate than C3H/HeJ mice. According to the study by Vercammen et al., an unregulated pathogenic Th1-type response is the cause of the elevated mortality in infected C57BL/6 mice [Citation174]. And DNA vaccination may increase the production of IFN-γ even further, thereby aggravating immunopathology instead of providing protection [Citation174]. On the other hand, weak antigen-specific spleen cell proliferation had also been reported after immunization in C57BL/6 mice [Citation171]. This might further explain why C57BL/6 mice showed the highest mortality rate when compared to two other strains. A consistent result was reported by Dautu et al., with BALB/c mice having a higher survival rate than C57BL/6 mice [Citation93].
Thus, the selection of mice strains has a significant influence on the challenge results. The genetic characteristics of the mice strain are the first determinant, and their post-immunization response can be a double-edged sword. Although C3H/HeJ reported higher IFN-γ expression and a more significant Th1 response than BALB/c, their survival expectations may not be as high as those of BALB/c. Furthermore, a higher immune response may induce immunopathology, as in the C57BL/6 mice, and eventually increase mice mortality.
3.3.3. Immunization dose
The selection of this parameter is relatively uniform across studies. A total of 100 μg plasmid(s) are most commonly used. Studies have shown that higher doses are not always better at yielding protection and that sometimes even 10 μg can provide better immunity than 100 μg [Citation176]. At this order of magnitude, the effect of blindly increasing the dose is relatively insignificant and sometimes even counterproductive. Rather than that, we should worry about the low transfection rate of a large plasmid and difficulties in translating a large protein [Citation177].
3.3.4. Challenge dose
1000 RH tachyzoites and 10/20 Pru cysts are most commonly used in acute and chronic challenges. At this point, we should look not only at the number but also at parasite vitality. Hence, the preparation of tachyzoites and cysts has become the most important factor other than quantity. For tachyzoite, parasites that still proliferating within the cell would have higher vitality than that of the parasite released. Hence, to ensure the quality of the parasites is uniformly high, we recommend first discarding the cell culture supernatant, then lysate the cultured cells to release parasites with higher vitality for the upcoming challenge. The same principle for cysts, a similar volume and number of cysts used in chronic infection is always preferred.
4. Conclusion
Toxoplasmosis is a widespread disease caused by T. gondii, a protozoan parasite. T. gondii poses a threat to human health and, meanwhile, brings economic losses to our animal husbandry industry. Humans have been searching for preventive or therapeutic approaches against T. gondii for decades. DNA vaccines, labeled as safe, inexpensive and highly malleable, seem to be a promising candidate among the options. But so far, no DNA vaccine can bring complete protection against T. gondii. This review sought to compare the results of considerable vaccine studies and sorts out antigens and adjuvants with a better effect so far in terms of protective efficacy.
For sole gene immunization, vaccines that employ MICs would yield a better outcome in reaching partial life-long protection (33%) or prolongation in survival length (average 12 days). GRAs vaccines, however, have the highest average survival rate while ROP group yields the greatest average brain cyst reduction.
Abbreviations that appeared in the review:
The best results among all papers come from multi-gene vaccine cocktails adapting SAG1+ GRA4+ GM-CSF and GRA1+ GRA7, leading to an 87% survival rate in the acute challenge, and 89% cyst reduction in the chronic challenge, respectively [Citation7,Citation125]. However, we suspect the high survival outcome of the SAG1+ GRA4+ GM-CSF vaccine might result from the low dose of acute challenge. And hence we consider the combinations of GRA7+ ROP1, SAG1+ GRA1, SAG1+ SAG3 and SAG1+ GRA1+ ROP2+ GRA4+ SAG2C+SAG2X to be the more credible best result of the acute challenge.
Besides GRA1+ GRA7, multi-gene cocktail vaccines adapting SAGs and ROPs also appear to be promising against chronic infection as they are relatively more stable in chronic protective efficacy. The antigen PF with ROP16+ ROP18+ MIC6+ CDPK3 cocktail was an impressive attempt to combat chronic challenges. When PF was added to the cocktail, the cyst reduction rate skyrocketed from 38% to 80% [Citation101].
Furthermore, an appropriate selection of adjuvants can bring approximately a 20% increase in survival and cyst reduction. Among all results, genetic adjuvants (IL-12, IL-18, PCTXA2/B and HbsAg) and conventional adjuvants (Levamisole) can best enhance existing vaccines.
This review also discussed variables in the challenge trial to provide a basis for comparison. Studies have demonstrated that the BABL/C and C57BL/6 strains are the least and most susceptible to T. gondii infection, respectively [Citation171,Citation172]. Also, although intramuscular injection is the most widely used method, it is not considered the best fit for DNA vaccines [Citation60,Citation160,Citation167]. And hence in the future, it will be necessary to explore new routes of inoculation to bring the best effect of DNA vaccines.
Taken together, vaccines that employ multi-antigen, genetic adjuvants are more likely to be potent candidates. And variables such as the choice of mice strain and route of immunization would have a certain impact on the outcome of the challenge trial.
5. Expert opinion
T. gondii is a protozoan parasite with a wide range of hosts. So far, researchers have tried different types of Toxoplasma vaccines, including recombinant vaccines, DNA vaccines, subunit vaccines, live attenuated vaccines, and nanoparticle vaccines [Citation178,Citation179]. Of these, only the live attenuated vaccine can offer desirable and effective protection against T. gondii [Citation180]. However, existing attenuated vaccines have the disadvantages of being expensive, causing side effects and short shelf-life [Citation11]. To overcome these shortcomings, researchers have turned their attention to the live attenuated mutants, among which the nutrient auxotrophic mutant was considered to be the most promising [Citation12]. But due to the adverse effects it might cause and its risk of reverting back to a pathogenic strain, it is still destined not an ideal vaccine to be used in humans [Citation10].
Although progress has been made in DNA vaccines against T. gondii in recent years, no studies have yet reported complete protection. The most well-studied antigens: SAGs, MICs, GRAs and ROPs, are all directly related to the parasite’s invasion process. Although some researchers believe antigens either located on the surface (SAGs) of, or secreted (MICs, GRAs and ROPs) by the parasite are necessary elements for a successful vaccine, adapting these antigens alone is not enough to provide desirable protection, as proven by studies in recent two decades [Citation177]. Hence, it is necessary to expand the scope of our exploration beyond these four invasion- related antigens to, for instance, metabolism-related antigens. Since T. gondii has three different life stages, combining invading antigen & metabolic antigen into a cocktail is a hopeful strategy to offer a broader and better protective effect than using the invading antigen alone. And in real case, there has also been increasing evidence indicating the introduction of metabolic antigens can indeed further improve the protection efficacy. On top of that, implementing appropriate genetic adjuvants is also be of crucial and shown good potential in many studies, as we mentioned above.
In the coming five years, future research exploring the combination of different-stage/function antigens with adjuvants still holds the best chance to bring desirable protection. The pattern of adapting invasion antigens + metabolic antigens + genetic adjuvants might offer us optimal protection against this multiple-life-stage parasite. Tools that have become more convenient and well-developed in recent years, such as bioinformatics analysis, will definitely greatly speed up this in-by-inch search. With the progress of other variables, such as the exploration of novel administration methods for better DNA delivery to induce better protection, there is still a fairly good chance that one day we can develop a DNA vaccine that can provide full protection against T.gondii.
Nevertheless, it is still unlikely to see such a vaccine within the coming five years, as we refer to the past 20 years of T. gondii DNA vaccine research. Currently, T. gondii DNA vaccines are far from laboratory application, and there is even a longer way to go before they can be implemented into practical application. First and foremost, the immunogenicity of current DNA vaccines against T. gondii is poor due to DNA vaccines’ inability to mimic the T. gondii cell invasion process as the attenuated vaccine does. These built-in properties will not change no matter how many years of research. All we can do, for the time being, is to make up for this weakness from other aspects within our reach, such as the improvement of immune strategies. Theoretically, the introduction of in silico analysis can generate broader immune responses against multiple antigens in a small delivery vehicle, optimize the vaccine design to elicit desired immunity response, and mimic the processing and presentation of antigens during a natural infection [Citation153]. However, as noted, the effect of the in silico prediction has only a limited impact on enhancing the survival outcome. The prolongation of survival length is comparable to that of most other general traditional vaccine studies, suggesting predictions did not significantly contribute to the further escalation of protection rates. To maximize the impact of in silico research, adjuvants, delivery techniques, and epitope analyses must be developed or modified. Secondly, in addition to the factors that affect our vaccines, the sex of the vaccinated, decreased vaccine effectiveness in larger-size animals, and T. gondii prevalence strain in different regions are all critical variables to consider [Citation169,Citation179,Citation181,Citation182]. It is conceivable that there will be many more obstacles to overcome if there is ever a vaccine that can make it to its clinical trials before application. And lastly, unclear T. gondii molecular/ pathogenesis mechanisms in different species is another challenge that should be addressed. Different hosts use different mechanisms to control T.gondii. For instance, in mouse, the parasite’s virulence is mediated by ROP5-ROP18, while in human infection, ROP5 and ROP18 are not virulence factors and virulence determinants are unknown [Citation183,Citation184].
In the next five years, the call for using uniform parameters as much as possible to enable horizontal comparisons is another key to accelerate the development of this field. We here suggest unifying variables in studies as much as possible to allow horizontal comparison. Obviously, such unification would make it more straightforward to identify better combination through direct comparison of different studies. And moreover, normalizing the variables would, as a result, make the results of studies more credible, rather than obtaining a falsely high rate of protection by lowering the challenge dose. To allow horizontal comparison, we suggest the following control strategy as a reference; 1) use a moderate dosage of 1,000 RH tachyzoites and 10 PRU cysts with similarly high vitality in acute and chronic challenges. 2) try avoid C57BL/6 mice and use moderate susceptible mice strain C3H/HeJ as the animal model instead, and 3) i.m. a total of 100 μg plasmid(s) or 2 μg gene gun vaccination per shot during vaccination, at 0, 2 and 4 weeks.
To sum up, for this parasite with multiple life stages, the strategy of combining invasion antigens and metabolic antigens with adjuvants or novel inoculation methods may confer the optimal protection we desire. In the next five years, in addition to finding a better immune strategy, we will also need to keep on developing better in silico prediction methods, solve problems posed by variables in practical applications, and gain a more profound knowledge of T.gondii-host molecular interaction. On top of that, facilitating a general consensus on key variables of challenge trials is crucial, as this would allow better horizontal comparison and, ultimately, further speed up the research.
Article highlights
For sole-gene vaccination, MICs and GRAs are the most promising against acute challenges while ROPs confer the best protection against that of chronic.
For multi-gene vaccination, cocktails with SAGs, GRAs and ROPs perform better in the acute challenge. And cocktails with ROPs, SAGs, GRA7 and T. gondii Profilin can greatly improve cyst reduction in chronic challenges.
In silico analysis can undoubtedly accelerate the exploration of multi-epitope DNA vaccines. However, currently, only a limited impact on further the escalation of survival length after the challenge was observed, and improvement toward a better approach is needed.
Genetic adjuvants (e.g. IL-12, IL-18, A2/B subunits of cholera toxin and Hepatitis B surface antigen) and conventional adjuvants (Levamisole) are of great potential in augmenting the efficacy of T. gondii DNA vaccine.
DNA vaccine delivery by conventional syringe injections is considered to be mediocre in inducing immune responses. Hence, some studies have adapted novel inoculation methods such as gene gun, to optimize the vaccine’s efficacy. Gene gun’s high reproductivity in inducing immune responses is considered to have great potential for future clinical application of DNA vaccination.
The selection of mice strains has a significant influence on the challenge results. Mice strain BALB/c, C3H/HeJ and C57BL/6 are categorized as low, intermediate and high susceptibility to T. gondii infection, respectively. C57BL/6 mice have always observed higher mortality.
Immunization and challenge dose would affect the outcome of the challenge trial with no doubt. Besides dosage, the quality of the plasmid, as well as the parasite vitality, are issues to be concerned.
Declaration of interests
The authors have no relevant affiliations or financial involvement with any organization or entity with a financial interest in or financial conflict with the subject matter or materials discussed in the manuscript. This includes employment, consultancies, honoraria, stock ownership or options, expert testimony, grants or patents received or pending, or royalties.
Reviewer disclosures
Peer reviewers on this manuscript have no relevant financial or other relationships to disclose.
Author contributions
All authors have substantially contributed to the conception and design of the review article and interpreting the relevant literature, and been involved in writing the review article or revised it for intellectual content.
Correction Statement
This article has been corrected with minor changes. These changes do not impact the academic content of the article.
Additional information
Funding
References
- Halonen SK, Weiss LM. Toxoplasmosis. Handb Clin Neurol. 2013;114:125–145. .
- Chowdhury MN. Toxoplasmosis: a review. J Med. 1986;17(5–6):373–396.
- Saadatnia G, Golkar M. A review on human toxoplasmosis. Scand J Infect Dis. 2012;44(11):805–814.
- Boughattas S, Bergaoui R, Essid R, et al. Seroprevalence of Toxoplasma gondii infection among horses in Tunisia. Parasit Vectors. 2011 Nov 22;4(1):218.
- Wang M, Wang YH, Ye Q, et al. Serological survey of Toxoplasma gondii in Tibetan mastiffs (Canis lupus familiaris) and yaks (Bos grunniens) in Qinghai, China. Parasit Vectors. 2012 Feb 13;5(1):35.
- Yang N, Mu MY, Li HK, et al. Seroprevalence of Toxoplasma gondii infection in slaughtered chickens, ducks, and geese in Shenyang, northeastern China. Parasit Vectors. 2012 Oct 18;5(1):237.
- Mevelec MN, Bout D, Desolme B, et al. Evaluation of protective effect of DNA vaccination with genes encoding antigens GRA4 and SAG1 associated with GM-CSF plasmid, against acute, chronical and congenital toxoplasmosis in mice. Vaccine. 2005 Aug 22;23(36):4489–4499.
- Dubey JP. The history of Toxoplasma gondii–the first 100 years. J Eukaryot Microbiol. 2008 Nov-Dec;55(6):467–475.
- Ji-Long S, Li Y. Prevalence and fundamental researches of prevention and treatment of toxoplasmosis in China: an overview. Zhongguo Xue Xi Chong Bing Fang Zhi Za Zhi. 2019 Apr 2;31(1):71–76.
- Kur J, Holec-Gasior L, Hiszczyńska-Sawicka E. Current status of toxoplasmosis vaccine development. Expert Rev Vaccines. 2009 Jun;8(6):791–808.
- Liu Q, Singla LD, Zhou H. Vaccines against Toxoplasma gondii: status, challenges and future directions. Hum Vaccin Immunother. 2012 Sep;8(9):1305–1308.
- Fox BA, Bzik DJ. De novo pyrimidine biosynthesis is required for virulence of Toxoplasma gondii. Nature. 2002 Feb 21;415(6874):926–929.
- Ghaffarifar F. Strategies of DNA vaccines against toxoplasmosis. Rev Med Microbiol. 2015;26(3):88–90.
- Yang H, Wang FY. Research progress of DNA vaccines. Chin Anim Husbandry Vet Med. 2013;40(1):72–76.
- Saade F, Petrovsky N. Technologies for enhanced efficacy of DNA vaccines. Expert Rev Vaccines. 2012 Feb;11(2):189–209.
- Ahmadpour E, Sarvi S, Hashemi Soteh MB, et al. Enhancing immune responses to a DNA vaccine encoding Toxoplasma gondii GRA14 by calcium phosphate nanoparticles as an adjuvant. Immunol Lett. 2017 May;185:40–47.
- Hu LY, Zhang NZ, Zhang FK, et al. Resistance to chronic Toxoplasma gondii infection induced by a DNA vaccine expressing GRA16. Biomed Res Int. 2017;2017:1295038.
- Zhu WN, Wang JL, Chen K, et al. Evaluation of protective immunity induced by DNA vaccination with genes encoding Toxoplasma gondii GRA17 and GRA23 against acute toxoplasmosis in mice. Exp Parasitol. 2017 Aug;179:20–27.
- Peng GH, Yuan ZG, Zhou DH, et al. Toxoplasma gondii microneme protein 6 (MIC6) is a potential vaccine candidate against toxoplasmosis in mice. Vaccine. 2009 Nov 5;27(47):6570–6574.
- Liu MM, Yuan ZG, Peng GH, et al. Toxoplasma gondii microneme protein 8 (MIC8) is a potential vaccine candidate against toxoplasmosis. Parasitol Res. 2010 Apr;106(5):1079–1084
- Chen J, Zhou DH, Li ZY, et al. Toxoplasma gondii: protective immunity induced by rhoptry protein 9 (TgROP9) against acute toxoplasmosis. Exp Parasitol. 2014 Apr;139:42–48.
- Wang HL, Wang YJ, Pei YJ, et al. DNA vaccination with a gene encoding Toxoplasma gondii rhoptry protein 17 induces partial protective immunity against lethal challenge in mice. Parasite. 2016;23:4.
- Zhang Z, Li Y, Wang M, et al. Immune protection of rhoptry protein 21 (ROP21) of Toxoplasma gondii as a DNA vaccine against toxoplasmosis. Front Microbiol. 2018;9:909.
- Zhang Z, Li Y, Xie Q, et al. The molecular characterization and immunity identification of rhoptry protein 22 of Toxoplasma gondii as a DNA vaccine candidate against toxoplasmosis. J Eukaryot Microbiol. 2019 Jan;66(1):147–157
- Zhang Z, Li Y, Liang Y, et al. Molecular characterization and protective immunity of rhoptry protein 35 (ROP35) of Toxoplasma gondii as a DNA vaccine. Vet Parasitol. 2018 Aug 30;260:12–21. DOI:10.1016/j.vetpar.2018.06.016.
- Yang WB, Zhou DH, Zou Y, et al. Vaccination with a DNA vaccine encoding Toxoplasma gondii ROP54 induces protective immunity against toxoplasmosis in mice. Acta Trop. 2017 Dec;176:427–432.
- Lu G, Zhou A, Meng M, et al. Alpha-galactosylceramide enhances protective immunity induced by DNA vaccine of the SAG5D gene of Toxoplasma gondii. BMC Infect Dis. 2014 Dec 20;14(1):3862.
- Li J, Huang X, Zhang G, et al. Immune response and protective efficacy against homologous challenge in BALB/c mice vaccinated with DNA vaccine encoding Toxoplasma gondii actin depolymerizing factor gene. Vet Parasitol. 2011 Jun 30;179(1–3):1–6.
- Zhao G, Zhou A, Lu G, et al. Identification and characterization of Toxoplasma gondii aspartic protease 1 as a novel vaccine candidate against toxoplasmosis. Parasit Vectors. 2013 Jun 14;6(1):175.
- Huang SY, Chen K, Wang JL, et al. Evaluation of protective immunity induced by recombinant calcium-dependent protein kinase 1 (TgCDPK1) protein against acute toxoplasmosis in mice. Microb Pathog. 2019 Aug;133:103560.
- Zhao G, Zhou A, Lv G, et al. Toxoplasma gondii cathepsin proteases are undeveloped prominent vaccine antigens against toxoplasmosis. BMC Infect Dis. 2013 May 7;13(1):207.
- Han Y, Zhou A, Lu G, et al. DNA Vaccines encoding toxoplasma gondii cathepsin C 1 induce protection against toxoplasmosis in mice. Korean J Parasitol. 2017 Oct;55(5):505–512
- Hassan IA, Wang S, Xu L, et al. DNA vaccination with a gene encoding Toxoplasma gondii Deoxyribose Phosphate Aldolase (TgDPA) induces partial protective immunity against lethal challenge in mice. Parasit Vectors. 2014 Sep 8;7(1):431.
- Wang S, Wang Y, Sun X, et al. Protective immunity against acute toxoplasmosis in BALB/c mice induced by a DNA vaccine encoding Toxoplasma gondii elongation factor 1-alpha. BMC Infect Dis. 2015 Oct 24;15(1):448.
- Chen J, Huang SY, Li ZY, et al. Protective immunity induced by a DNA vaccine expressing eIF4A of Toxoplasma gondii against acute toxoplasmosis in mice. Vaccine. 2013 Mar 25;31(13):1734–1739.
- Guo HT, Li ZY, Wang JL, et al. Immune responses induced by pVAX/TgERK7 against Toxoplasma gondii Infection in BALB/c Mice. Iran J Parasitol. 2019 Oct-Dec;14(4):552–562.
- Hassan IA, Wang S, Xu L, et al. Immunoglobulin and cytokine changes induced following immunization with a DNA vaccine encoding Toxoplasma gondii selenium-dependent glutathione reductase protein. Exp Parasitol. 2014 Nov;146:1–10.
- Wang S, Hassan IA, Liu X, et al. Immunological changes induced by Toxoplasma gondii Glutathione-S-Transferase (TgGST) delivered as a DNA vaccine. Res Vet Sci. 2015 Apr;99:157–164.
- Chen J, Huang SY, Zhou DH, et al. DNA immunization with eukaryotic initiation factor-2alpha of Toxoplasma gondii induces protective immunity against acute and chronic toxoplasmosis in mice. Vaccine. 2013 Dec 16;31(52):6225–6231.
- Cui X, Lei T, Yang D, et al. Toxoplasma gondii immune mapped protein-1 (TgIMP1) is a novel vaccine candidate against toxoplasmosis. Vaccine. 2012 Mar 16;30(13):2282–2287.
- Hassan IA, Wang S, Xu L, et al. Immunological response and protection of mice immunized with plasmid encoding Toxoplasma gondii glycolytic enzyme malate dehydrogenase. Parasite Immunol. 2014 Dec;36(12):674–683
- Zheng B, Ding J, Lou D, et al. The virulence-related MYR1 protein of toxoplasma gondii as a novel DNA vaccine against toxoplasmosis in mice. Front Microbiol. 2019;10:734.
- Yan HK, Yuan ZG, Petersen E, et al. Toxoplasma gondii: protective immunity against experimental toxoplasmosis induced by a DNA vaccine encoding the perforin-like protein 1. Exp Parasitol. 2011 May;128(1):38–43
- Zhao Y, Li ZY, Chen J, et al. Protective efficacy of pVAX-RON5p against acute and chronic infections of Toxoplasma gondii in BALB/c mice. Exp Parasitol. 2016 Apr;163:24–30.
- Liu Y, Cao A, Li Y, et al. Immunization with a DNA vaccine encoding Toxoplasma gondii Superoxide dismutase (TgSOD) induces partial immune protection against acute toxoplasmosis in BALB/c mice. BMC Infect Dis. 2017 Jun 7;17(1):403.
- Zheng B, Ding J, Chen X, et al. Immuno-efficacy of a T. gondii secreted protein with an altered Thrombospondin Repeat (TgSPATR) as a novel DNA vaccine candidate against acute toxoplasmosis in BALB/c mice. Front Microbiol. 2017;8:216.
- Zhou J, Wang L, Lu G, et al. Epitope analysis and protection by a ROP19 DNA vaccine against Toxoplasma gondii. Parasite. 2016;23:17.
- Lu G, Zhou J, Zhao YH, et al. DNA vaccine ROP29 from Toxoplasma gondii containing R848 enhances protective immunity in mice. Parasite Immunol. 2018 Oct;40(10):e12578
- Lu G, Wang L, Zhou A, et al. Epitope analysis, expression and protection of SAG5A vaccine against Toxoplasma gondii. Acta Trop. 2015 Jun;146:66–72.
- Naserifar R, Ghaffarifar F, Dalimi A, et al. Evaluation of immunogenicity of cocktail DNA vaccine containing plasmids encoding complete GRA5, SAG1, and ROP2 antigens of Toxoplasma gondii in BALB/C mice. Iran J Parasitol. 2015 Oct-Dec;10(4):590–598.
- Cao A, Liu Y, Wang J, et al. Toxoplasma gondii: vaccination with a DNA vaccine encoding T- and B-cell epitopes of SAG1, GRA2, GRA7 and ROP16 elicits protection against acute toxoplasmosis in mice. Vaccine. 2015 Nov 27;33(48):6757–6762.
- Khodadadi M, Ghaffarifar F, Dalimi A, et al. Immunogenicity of in-silico designed multi-epitope DNA vaccine encoding SAG1, SAG3 and SAG5 of Toxoplasma gondii adjuvanted with CpG-ODN against acute toxoplasmosis in BALB/c mice. Acta Trop. 2021 Apr;216:105836.
- Angus CW, Klivington-Evans D, Dubey JP, et al. Immunization with a DNA plasmid encoding the SAG1 (P30) protein of Toxoplasma gondii is immunogenic and protective in rodents. J Infect Dis. 2000 Jan;181(1):317–324
- Dzierszinski F, Mortuaire M, Cesbron-Delauw MF, et al. Targeted disruption of the glycosylphosphatidylinositol-anchored surface antigen SAG3 gene in Toxoplasma gondii decreases host cell adhesion and drastically reduces virulence in mice. Mol Microbiol. 2000 Aug;37(3):574–582
- Laguia-Becher M, Martin V, Kraemer M, et al. Effect of codon optimization and subcellular targeting on Toxoplasma gondii antigen SAG1 expression in tobacco leaves to use in subcutaneous and oral immunization in mice. BMC Biotechnol. 2010 Jul 15;10(1):52.
- Carruthers VB, Sibley LD. Sequential protein secretion from three distinct organelles of Toxoplasma gondii accompanies invasion of human fibroblasts. Eur J Cell Biol. 1997 Jun;73(2):114–123.
- El Hajj H, Lebrun M, Arold ST, et al. ROP18 is a rhoptry kinase controlling the intracellular proliferation of Toxoplasma gondii. PLoS Pathog. 2007 Feb;3(2):e14
- Joiner KA, Roos DS. Secretory traffic in the eukaryotic parasite Toxoplasma gondii: less is more. J Cell Biol. 2002 May 13;157(4):557–563.
- Ngo HM, Hoppe HC, Joiner KA. Differential sorting and post-secretory targeting of proteins in parasitic invasion. Trends Cell Biol. 2000 Feb;10(2):67–72.
- Foroutan M, Ghaffarifar F, Sharifi Z, et al. Rhoptry antigens as Toxoplasma gondii vaccine target. Clin Exp Vaccine Res. 2019 Jan;8(1):4–26
- Li ZY, Lu J, Zhang NZ, et al. Immune responses induced by HSP60 DNA vaccine against toxoplasma gondii infection in Kunming mice. Korean J Parasitol. 2018 Jun;56(3):237–245
- Li ZY, Lu J, Zhang NZ, et al. Immunization with plasmid DNA expressing heat shock protein 40 confers prophylactic protection against chronic Toxoplasma gondii infection in Kunming mice. Parasite. 2018;25:37.
- Mohamed RM, Aosai F, Chen M, et al. Induction of protective immunity by DNA vaccination with Toxoplasma gondii HSP70, HSP30 and SAG1 genes. Vaccine. 2003 Jun 20;21(21–22):2852–2861.
- Chen J, Li ZY, Huang SY, et al. Protective efficacy of Toxoplasma gondii calcium-dependent protein kinase 1 (TgCDPK1) adjuvated with recombinant IL-15 and IL-21 against experimental toxoplasmosis in mice. BMC Infect Dis. 2014 Sep 6;14(1):487.
- Chen K, Wang JL, Huang SY, et al. Immune responses and protection after DNA vaccination against Toxoplasma gondii calcium-dependent protein kinase 2 (TgCDPK2). Parasite. 2017;24:41.
- Morlon-Guyot J, Berry L, Chen CT, et al. The Toxoplasma gondii calcium-dependent protein kinase 7 is involved in early steps of parasite division and is crucial for parasite survival. Cell Microbiol. 2014 Jan;16(1):95–114
- Zhang NZ, Huang SY, Xu Y, et al. Evaluation of immune responses in mice after DNA immunization with putative Toxoplasma gondii calcium-dependent protein kinase 5. Clin Vaccine Immunol. 2014 Jul;21(7):924–929.
- Zhang NZ, Huang SY, Zhou DH, et al. Protective immunity against Toxoplasma gondii induced by DNA immunization with the gene encoding a novel vaccine candidate: calcium-dependent protein kinase 3. BMC Infect Dis. 2013 Oct 31;13(1):512.
- Zhang NZ, Xu Y, Wang M, et al. Vaccination with Toxoplasma gondii calcium-dependent protein kinase 6 and rhoptry protein 18 encapsulated in poly(lactide-co-glycolide) microspheres induces long-term protective immunity in mice. BMC Infect Dis. 2016 Apr 18;16(1):168.
- Mineo JR, McLeod R, Mack D, et al. Antibodies to Toxoplasma gondii major surface protein (SAG-1, P30) inhibit infection of host cells and are produced in murine intestine after peroral infection. J Immunol. 1993;150(9):3951–3964.
- Mineo JR, Kasper LH. Attachment of Toxoplasma gondii to host cells involves major surface protein, SAG-1 (P30). Exp Parasitol. 1994 Aug;79(1):11–20.
- Grimwood J, Smith JE. Toxoplasma gondii: the role of parasite surface and secreted proteins in host cell invasion. Int J Parasitol. 1996 Feb;26(2):169–173.
- Wang Y, Yin H. Research progress on surface antigen 1 (SAG1) of Toxoplasma gondii. Parasit Vectors. 2014 Apr 13;7(1):180.
- Cong H, Zhang M, Xin Q, et al. Compound DNA vaccine encoding SAG1/ SAG3 with A2/B subunit of cholera toxin as a genetic adjuvant protects BALB/c mice against Toxoplasma gondii. Parasit Vectors. 2013 Mar 13;6(1):63.
- Hoseinian Khosroshahi K, Ghaffarifar F, D’Souza S, et al. Evaluation of the immune response induced by DNA vaccine cocktail expressing complete SAG1 and ROP2 genes against toxoplasmosis. Vaccine. 2011 Jan 17;29(4):778–783.
- Maraghi S, Ghadiri AA, Tavalla M, et al. Evaluation of immunogenicity and protective effect of DNA vaccine encoding surface antigen1 (SAG1) of Toxoplasma gondii and TLR-5 ligand as a genetic adjuvant against acute toxoplasmosis in BALB/c mice. Biologicals. 2019 Nov;62:39–49.
- Mavi SA, Modarressi MH, Mohebali M, et al. Assessment of the immunogenicity and protective efficiency of a novel dual-promoter DNA vaccine, harboring SAG1 and GRA7 genes, from RH strain of Toxoplasma gondii in BALB/c mice. Infect Drug Resist. 2019;12:2519–2530.
- Meng M, He S, Zhao G, et al. Evaluation of protective immune responses induced by DNA vaccines encoding Toxoplasma gondii surface antigen 1 (SAG1) and 14-3-3 protein in BALB/c mice. Parasit Vectors. 2012 Nov 26;5(1):273.
- Wu XN, Lin J, Lin X, et al. Multicomponent DNA vaccine-encoding Toxoplasma gondii GRA1 and SAG1 primes: anti-Toxoplasma immune response in mice. Parasitol Res. 2012 Nov;111(5):2001–2009.
- Zhou H, Min J, Zhao Q, et al. Protective immune response against Toxoplasma gondii elicited by a recombinant DNA vaccine with a novel genetic adjuvant. Vaccine. 2012 Feb 27;30(10):1800–1806.
- Liu Q, Shang L, Jin H, et al. The protective effect of a Toxoplasma gondii SAG1 plasmid DNA vaccine in mice is enhanced with IL-18. Res Vet Sci. 2010 Aug;89(1):93–97
- Gao Q, Zhang NZ, Zhang FK, et al. Immune response and protective effect against chronic Toxoplasma gondii infection induced by vaccination with a DNA vaccine encoding profilin. BMC Infect Dis. 2018 Mar 7;18(1):117.
- Zhang M, Zhao L, Song J, et al. DNA vaccine encoding the Toxoplasma gondii bradyzoite-specific surface antigens SAG2CDX protect BALB/c mice against type II parasite infection. Vaccine. 2013 Sep 23;31(41):4536–4540.
- Lu G, Zhou J, Zhou A, et al. SAG5B and SAG5C combined vaccine protects mice against Toxoplasma gondii infection. Parasitol Int. 2017 Oct;66(5):596–602
- Zhou J, Wang L. SAG4 DNA and peptide vaccination provides partial protection against T. gondii infection in BALB/c mice. Front Microbiol. 2017;8:1733.
- Wang Y, Yin H. Research advances in microneme protein 3 of Toxoplasma gondii. Parasit Vectors. 2015 Jul 22;8(1):384.
- Cerede O, Dubremetz JF, Soete M, et al. Synergistic role of micronemal proteins in Toxoplasma gondii virulence. J Exp Med. 2005 Feb 7;201(3):453–463.
- Reiss M, Viebig N, Brecht S, et al. Identification and characterization of an escorter for two secretory adhesins in Toxoplasma gondii. J Cell Biol. 2001 Feb 5;152(3):563–578.
- Meissner M, Reiss M, Viebig N, et al. A family of transmembrane microneme proteins of Toxoplasma gondii contain EGF-like domains and function as escorters. J Cell Sci. 2002 Feb 1;115(Pt 3):563–574.
- Baldi DL, Andrews KT, Waller RF, et al. RAP1 controls rhoptry targeting of RAP2 in the malaria parasite Plasmodium falciparum. Embo J. 2000 Jun 1;19(11):2435–2443.
- Pinzan CF, Sardinha-Silva A, Almeida F, et al. Vaccination with recombinant microneme proteins confers protection against experimental toxoplasmosis in mice. PLoS One. 2015;10(11):e0143087.
- Brossier F, David Sibley L. Toxoplasma gondii: microneme protein MIC2. Int J Biochem Cell Biol. 2005 Nov;37(11):2266–2272.
- Dautu G, Munyaka B, Carmen G, et al. Toxoplasma gondii: DNA vaccination with genes encoding antigens MIC2, M2AP, AMA1 and BAG1 and evaluation of their immunogenic potential. Exp Parasitol. 2007 Jul;116(3):273–282
- Ismael AB, Hedhli D, Cerede O, et al. Further analysis of protection induced by the MIC3 DNA vaccine against T. gondii: CD4 and CD8 T cells are the major effectors of the MIC3 DNA vaccine-induced protection, both Lectin-like and EGF-like domains of MIC3 conferred protection. Vaccine. 2009 May 14;27(22):2959–2966.
- Xiang W, Qiong Z, Li-peng L, et al. The location of invasion-related protein MIC3 of Toxoplasma gondii and protective effect of its DNA vaccine in mice. Vet Parasitol. 2009 Dec 3;166(1–2):1–7.
- Fang R, Feng H, Hu M, et al. Evaluation of immune responses induced by SAG1 and MIC3 vaccine cocktails against Toxoplasma gondii. Vet Parasitol. 2012 Jun 8;187(1–2):140–146.
- Fang R, Nie H, Wang Z, et al. Protective immune response in BALB/c mice induced by a suicidal DNA vaccine of the MIC3 gene of Toxoplasma gondii. Vet Parasitol. 2009 Oct 14;164(2–4):134–140.
- Gong P, Cao L, Guo Y, et al. Toxoplasma gondii: protective immunity induced by a DNA vaccine expressing GRA1 and MIC3 against toxoplasmosis in BALB/c mice. Exp Parasitol. 2016 Jul;166:131–136.
- Qu D, Han J, Du A. Evaluation of protective effect of multiantigenic DNA vaccine encoding MIC3 and ROP18 antigen segments of Toxoplasma gondii in mice. Parasitol Res. 2013 Jul;112(7):2593–2599.
- Yan HK, Yuan ZG, Song HQ, et al. Vaccination with a DNA vaccine coding for perforin-like protein 1 and MIC6 induces significant protective immunity against Toxoplasma gondii. Clin Vaccine Immunol. 2012 May;19(5):684–689
- Zhang NZ, Gao Q, Wang M, et al. Immunization with a DNA vaccine cocktail encoding TgPF, TgROP16, TgROP18, TgMIC6, and TgCDPK3 genes protects mice against chronic toxoplasmosis. Front Immunol. 2018;9:1505.
- Li ZY, Chen J, Petersen E, et al. Synergy of mIL-21 and mIL-15 in enhancing DNA vaccine efficacy against acute and chronic Toxoplasma gondii infection in mice. Vaccine. 2014 May 23;32(25):3058–3065.
- Yuan ZG, Ren D, Zhou DH, et al. Evaluation of protective effect of pVAX-TgMIC13 plasmid against acute and chronic Toxoplasma gondii infection in a murine model. Vaccine. 2013 Jun 28;31(31):3135–3139.
- Tao Q, Fang R, Zhang W, et al. Protective immunity induced by a DNA vaccine-encoding Toxoplasma gondii microneme protein 11 against acute toxoplasmosis in BALB/c mice. Parasitol Res. 2013 Aug;112(8):2871–2877
- Dlugonska H. Toxoplasma rhoptries: unique secretory organelles and source of promising vaccine proteins for immunoprevention of toxoplasmosis. J Biomed Biotechnol. 2008;2008:632424.
- Bradley PJ, Sibley LD. Rhoptries: an arsenal of secreted virulence factors. Curr Opin Microbiol. 2007 Dec;10(6):582–587.
- Wei F, Wang W, Liu Q. Protein kinases of Toxoplasma gondii: functions and drug targets. Parasitol Res. 2013 Jun;112(6):2121–2129.
- Quan JH, Chu JQ, Ismail HA, et al. Induction of protective immune responses by a multiantigenic DNA vaccine encoding GRA7 and ROP1 of Toxoplasma gondii. Clin Vaccine Immunol. 2012 May;19(5):666–674
- Sonaimuthu P, Ching XT, Fong MY, et al. Induction of Protective Immunity against toxoplasmosis in BALB/c Mice Vaccinated with Toxoplasma gondii Rhoptry-1. Front Microbiol. 2016;7:808.
- Foroutan M, Barati M, Ghaffarifar F. Enhancing immune responses by a novel multi-epitope ROP8 DNA vaccine plus interleukin-12 plasmid as a genetic adjuvant against acute Toxoplasma gondii infection in BALB/c mice. Microb Pathog. 2020 Oct;147:104435.
- Foroutan M, Ghaffarifar F, Sharifi Z, et al. Vaccination with a novel multi-epitope ROP8 DNA vaccine against acute Toxoplasma gondii infection induces strong B and T cell responses in mice. Comp Immunol Microbiol Infect Dis. 2020 Apr;69:101413.
- Vazini H, Ghafarifar F, Sharifi Z, et al. Evaluation of immune responses induced by GRA7 and ROP2 genes by DNA vaccine cocktails against acute toxoplasmosis in BALB/c mice. Avicenna J Med Biotechnol. 2018 Jan-Mar;10(1):2–8.
- Wang L, Lu G, Zhou A, et al. Evaluation of immune responses induced by rhoptry protein 5 and rhoptry protein 7 DNA vaccines against Toxoplasma gondii. Parasite Immunol. 2016 Apr;38(4):209–217
- Zhang J, He S, Jiang H, et al. Evaluation of the immune response induced by multiantigenic DNA vaccine encoding SAG1 and ROP2 of Toxoplasma gondii and the adjuvant properties of murine interleukin-12 plasmid in BALB/c mice. Parasitol Res. 2007 Jul;101(2):331–338
- Zhu YC, He Y, Liu JF, et al. Adjuvantic cytokine IL-33 improves the protective immunity of cocktailed DNA vaccine of ROP5 and ROP18 against toxoplasma gondii infection in mice. Parasite. 2020;27:26.
- Parthasarathy S, Fong MY, Ramaswamy K, et al. Protective immune response in BALB/c mice induced by DNA vaccine of the ROP8 gene of Toxoplasma gondii. Am J Trop Med Hyg. 2013 May;88(5):883–887
- Chen Y, Yu M, Hemandez JA, et al. Immuno-efficacy of DNA vaccines encoding PLP1 and ROP18 against experimental Toxoplasma gondii infection in mice. Exp Parasitol. 2018 May;188:73–78.
- Yuan ZG, Zhang XX, Lin RQ, et al. Protective effect against toxoplasmosis in mice induced by DNA immunization with gene encoding Toxoplasma gondii ROP18. Vaccine. 2011 Sep 2;29(38):6614–6619.
- Wang PY, Yuan ZG, Petersen E, et al. Protective efficacy of a Toxoplasma gondii rhoptry protein 13 plasmid DNA vaccine in mice. Clin Vaccine Immunol. 2012 Dec;19(12):1916–1920
- Liu Q, Wang F, Wang G, et al. Toxoplasma gondii: immune response and protective efficacy induced by ROP16/GRA7 multicomponent DNA vaccine with a genetic adjuvant B7-2. Hum Vaccin Immunother. 2014;10(1):184–191.
- Yuan ZG, Zhang XX, He XH, et al. Protective immunity induced by Toxoplasma gondii rhoptry protein 16 against toxoplasmosis in mice. Clin Vaccine Immunol. 2011 Jan;18(1):119–124
- Xu Y, Zhang NZ, Tan QD, et al. Evaluation of immuno-efficacy of a novel DNA vaccine encoding Toxoplasma gondii rhoptry protein 38 (TgROP38) against chronic toxoplasmosis in a murine model. BMC Infect Dis. 2014 Sep 30;14(1):525.
- Nam HW. GRA proteins of Toxoplasma gondii: maintenance of host-parasite interactions across the parasitophorous vacuolar membrane. Korean J Parasitol. 2009 Oct;47(Suppl):S29–37.
- Mercier C, Dubremetz JF, Rauscher B, et al. Biogenesis of nanotubular network in Toxoplasma parasitophorous vacuole induced by parasite proteins. Mol Biol Cell. 2002 Jul;13(7):2397–2409
- Jongert E, de Craeye S, Dewit J, et al. GRA7 provides protective immunity in cocktail DNA vaccines against Toxoplasma gondii. Parasite Immunol. 2007 Sep;29(9):445–453
- Ching XT, Fong MY, Lau YL. Evaluation of the protective effect of deoxyribonucleic acid vaccines encoding granule antigen 2 and 5 against acute toxoplasmosis in BALB/c Mice. Am J Trop Med Hyg. 2017 Jun;96(6):1441–1447.
- Chu JQ, Huang S, Ye W, et al. Evaluation of protective immune response induced by a DNA vaccine encoding GRA8 against acute toxoplasmosis in a murine model. Korean J Parasitol. 2018 Aug;56(4):325–334
- Labruyere E, Lingnau M, Mercier C, et al. Differential membrane targeting of the secretory proteins GRA4 and GRA6 within the parasitophorous vacuole formed by Toxoplasma gondii. Mol Biochem Parasitol. 1999 Aug 20;102(2):311–324.
- Lecordier L, Moleon-Borodowsky I, Dubremetz JF, et al. Characterization of a dense granule antigen of Toxoplasma gondii (GRA6) associated to the network of the parasitophorous vacuole. Mol Biochem Parasitol. 1995 Mar;70(1–2):85–94
- Sun XM, Zou J, AE A, et al. DNA vaccination with a gene encoding Toxoplasma gondii GRA6 induces partial protection against toxoplasmosis in BALB/c mice. Parasit Vectors. 2011 Nov 9;4(1):213.
- Zheng B, Lou D, Ding J, et al. GRA24-based DNA vaccine prolongs survival in mice challenged with a virulent Toxoplasma gondii strain. Front Immunol. 2019;10:418.
- Xu XP, Liu WG, Xu QM, et al. Evaluation of immune protection against Toxoplasma gondii infection in mice induced by a multi-antigenic DNA vaccine containing TgGRA24, TgGRA25 and TgMIC6. Parasite. 2019;26:58.
- Gupta RS, Golding GB. Evolution of HSP70 gene and its implications regarding relationships between archaebacteria, eubacteria, and eukaryotes. J Mol Evol. 1993 Dec;37(6):573–582.
- Kuppner MC, Gastpar R, Gelwer S, et al. The role of heat shock protein (hsp70) in dendritic cell maturation: hsp70 induces the maturation of immature dendritic cells but reduces DC differentiation from monocyte precursors. Eur J Immunol. 2001 May;31(5):1602–1609
- Lourido S, Shuman J, Zhang C, et al. Calcium-dependent protein kinase 1 is an essential regulator of exocytosis in Toxoplasma. Nature. 2010 May 20;465(7296):359–362.
- Lourido S, Tang K, Sibley LD. Distinct signalling pathways control Toxoplasma egress and host-cell invasion. Embo J. 2012 Dec 12;31(24):4524–4534.
- Uboldi AD, McCoy JM, Blume M, et al. Regulation of starch stores by a Ca(2+)-dependent protein kinase is essential for viable cyst development in Toxoplasma gondii. Cell Host Microbe. 2015 Dec 9;18(6):670–681.
- Chen J, Li ZY, Petersen E, et al. Co-administration of interleukins 7 and 15 with DNA vaccine improves protective immunity against Toxoplasma gondii. Exp Parasitol. 2016 Mar;162:18–23.
- Dowse TJ, Pascall JC, Brown KD, et al. Apicomplexan rhomboids have a potential role in microneme protein cleavage during host cell invasion. Int J Parasitol. 2005 Jun;35(7):747–756
- Brossier F, Jewett TJ, Sibley LD, et al. A spatially localized rhomboid protease cleaves cell surface adhesins essential for invasion by Toxoplasma. Proc Natl Acad Sci U S A. 2005 Mar 15;102(11):4146–4151.
- Zhang NZ, Xu Y, Wang M, et al. Protective efficacy of two novel DNA vaccines expressing Toxoplasma gondii rhomboid 4 and rhomboid 5 proteins against acute and chronic toxoplasmosis in mice. Expert Rev Vaccines. 2015;14(9):1289–1297.
- Han Y, Zhou A, Lu G, et al. Protection via a ROM4 DNA vaccine and peptide against Toxoplasma gondii in BALB/c mice. BMC Infect Dis. 2017 Jan 11;17(1):59.
- Rahimi MT, Sarvi S, Sharif M, et al. Immunological evaluation of a DNA cocktail vaccine with co-delivery of calcium phosphate nanoparticles (CaPNs) against the Toxoplasma gondii RH strain in BALB/c mice. Parasitol Res. 2017 Feb;116(2):609–616
- Santos JM, Ferguson DJ, Blackman MJ, et al. Intramembrane cleavage of AMA1 triggers Toxoplasma to switch from an invasive to a replicative mode. Science. 2011 Jan 28;331(6016):473–477.
- Hehl AB, Lekutis C, Grigg ME, et al. Toxoplasma gondii homologue of plasmodium apical membrane antigen 1 is involved in invasion of host cells. Infect Immun. 2000 Dec;68(12):7078–7086
- Gong P, Huang X, Yu Q, et al. The protective effect of a DNA vaccine encoding the Toxoplasma gondii cyclophilin gene in BALB/c mice. Parasite Immunol. 2013 Mar-Apr;35(3–4):140–146
- Fischer G, Aumuller T. Regulation of peptide bond cis/trans isomerization by enzyme catalysis and its implication in physiological processes. Rev Physiol Biochem Pharmacol. 2003;148:105–150.
- Koblansky AA, Jankovic D, Oh H, et al. Recognition of profilin by Toll-like receptor 12 is critical for host resistance to Toxoplasma gondii. Immunity. 2013 Jan 24;38(1):119–130.
- Plattner F, Yarovinsky F, Romero S, et al. Toxoplasma profilin is essential for host cell invasion and TLR11-dependent induction of an interleukin-12 response. Cell Host Microbe. 2008 Feb 14;3(2):77–87.
- Zhang NZ, Gao Q, Wang M, et al. Protective efficacy against acute and chronic toxoplasma gondii infection induced by immunization with the DNA vaccine TgDOC2C. Front Microbiol. 2018;9:2965.
- Song P, He S, Zhou A, et al. Vaccination with toxofilin DNA in combination with an alum-monophosphoryl lipid A mixed adjuvant induces significant protective immunity against Toxoplasma gondii. BMC Infect Dis. 2017 Jan 5;17(1):19.
- Zheng L, Hu Y, Hua Q, et al. Protective immune response in mice induced by a suicidal DNA vaccine encoding NTPase-II gene of Toxoplasma gondii. Acta Trop. 2017 Feb;166:336–342.
- Suhrbier A. Multi-epitope DNA vaccines. Immunol Cell Biol. 1997;75(4):402–408.
- Tong JC, Ren EC. Immunoinformatics: current trends and future directions. Drug Discov Today. 2009 Jul;14(13–14):684–689.
- Patronov A, Doytchinova I. T-cell epitope vaccine design by immunoinformatics. Open Biol. 2013 Jan 8;3(1):120139.
- Khan AM, Miotto O, Heiny AT, et al. A systematic bioinformatics approach for selection of epitope-based vaccine targets. Cell Immunol. 2006 Dec;244(2):141–147
- Hajissa K, Zakaria R, Suppian R, et al. Epitope-based vaccine as a universal vaccination strategy against Toxoplasma gondii infection: a mini-review. J Adv Vet Anim Res. 2019 Jun;6(2):174–182.
- Song PX, Yao SH, Yao Y, et al. Epitope analysis and efficacy evaluation of phosphatase 2C (PP2C) DNA Vaccine Against Toxoplasma gondii infection. J Parasitol. 2020 Aug 1;106(4):513–521.
- Cong H, Yuan Q, Zhao Q, et al. Comparative efficacy of a multi-epitope DNA vaccine via intranasal, peroral, and intramuscular delivery against lethal Toxoplasma gondii infection in mice. Parasit Vectors. 2014 Mar 31;7(1):145.
- Greenland JR, Letvin NL. Chemical adjuvants for plasmid DNA vaccines. Vaccine. 2007 May 10;25(19):3731–3741.
- Montomoli E, Piccirella S, Khadang B, et al. Current adjuvants and new perspectives in vaccine formulation. Expert Rev Vaccines. 2011 Jul;10(7):1053–1061
- Li L, Petrovsky N. Molecular mechanisms for enhanced DNA vaccine immunogenicity. Expert Rev Vaccines. 2016;15(3):313–329.
- Ahmadpour E, Sarvi S, Hashemi Soteh MB, et al. Evaluation of the immune response in BALB/c mice induced by a novel DNA vaccine expressing GRA14 against Toxoplasma gondii. Parasite Immunol. 2017 Apr;39(4):e12419
- Ghaffarifar F, Jafarimodrek M, Vazini H, et al. Assessment of DNA vaccine encoding Toxoplasma gondii microneme complete gene and IL-12 as adjuvant in BALB/c mice. Iran J Basic Med Sci. 2019 Aug;22(8):901–907
- Sun H, Li J, Xiao T, et al. Protective immunity induced by a DNA vaccine cocktail expressing TgSAG1, TgROP2, and the genetic adjuvant HBsAg against Toxoplasma gondii infection. Microb Pathog. 2020 Oct;147:104441.
- Roozbehani M, Falak R, Mohammadi M, et al. Characterization of a multi-epitope peptide with selective MHC-binding capabilities encapsulated in PLGA nanoparticles as a novel vaccine candidate against Toxoplasma gondii infection. Vaccine. 2018 Oct 1;36(41):6124–6132.
- Bureau MF, Naimi S, Torero Ibad R, et al. Intramuscular plasmid DNA electrotransfer: biodistribution and degradation. Biochim Biophys Acta. 2004 Jan 20;1676(2):138–148.
- Yoshida A, Nagata T, Uchijima M, et al. Advantage of gene gun-mediated over intramuscular inoculation of plasmid DNA vaccine in reproducible induction of specific immune responses. Vaccine. 2000 Mar 6;18(17):1725–1729.
- Garcia JL. Vaccination concepts against Toxoplasma gondii. Expert Rev Vaccines. 2009 Feb;8(2):215–225.
- Pifer R, Yarovinsky F. Innate responses to Toxoplasma gondii in mice and humans. Trends Parasitol. 2011 Sep;27(9):388–393.
- Dziadek B, Gatkowska J, Brzostek A, et al. Evaluation of three recombinant multi-antigenic vaccines composed of surface and secretory antigens of Toxoplasma gondii in murine models of experimental toxoplasmosis. Vaccine. 2011 Jan 17;29(4):821–830.
- Dziadek B, Gatkowska J, Grzybowski M, et al. Toxoplasma gondii: the vaccine potential of three trivalent antigen-cocktails composed of recombinant ROP2, ROP4, GRA4 and SAG1 proteins against chronic toxoplasmosis in BALB/c mice. Exp Parasitol. 2012 May;131(1):133–138
- Brown CR, Hunter CA, Estes RG, et al. Definitive identification of a gene that confers resistance against Toxoplasma cyst burden and encephalitis. Immunology. 1995 Jul;85(3):419–428.
- Vercammen M, Scorza T, Huygen K, et al. DNA vaccination with genes encoding Toxoplasma gondii antigens GRA1, GRA7, and ROP2 induces partially protective immunity against lethal challenge in mice. Infect Immun. 2000 Jan;68(1):38–45
- Echeverria PC, de Miguel N, Costas M, et al. Potent antigen-specific immunity to Toxoplasma gondii in adjuvant-free vaccination system using Rop2-Leishmania infantum Hsp83 fusion protein. Vaccine. 2006 May 8;24(19):4102–4110.
- Song X, Xu L, Yan R, et al. The optimal immunization procedure of DNA vaccine pcDNA–TA4–IL-2 of Eimeria tenella and its cross-immunity to Eimeria necatrix and Eimeria acervulina. Vet Parasitol. 2009;159(1):30–36.
- Warner RC, Chapman RC, Davis BN, et al. REVIEW OF DNA VACCINE APPROACHES AGAINST THE PARASITE TOXOPLASMA GONDII. J Parasitol. 2021 Nov 1;107(6):882–903.
- Javadi Mamaghani A, Fathollahi A, Spotin A, et al. Candidate antigenic epitopes for vaccination and diagnosis strategies of Toxoplasma gondii infection: a review. Microb Pathog. 2019 Dec;137:103788.
- Mamaghani AJ, Fathollahi A, Arab-Mazar Z, et al. Toxoplasma gondii vaccine candidates: a concise review. Ir J Med Sci. 2022 Apr;8:1–31.
- Buxton D, Innes EA. A commercial vaccine for ovine toxoplasmosis. Parasitology. 1995;110(Suppl):S11–6.
- Jongert E, Roberts CW, Gargano N, et al. Vaccines against Toxoplasma gondii: challenges and opportunities. Mem Inst Oswaldo Cruz. 2009 Mar;104(2):252–266
- Babiuk LA, Pontarollo R, Babiuk S, et al. Induction of immune responses by DNA vaccines in large animals. Vaccine. 2003 Jan 30;21(7–8):649–658.
- Behnke MS, Khan A, Wootton JC, et al. Virulence differences in Toxoplasma mediated by amplification of a family of polymorphic pseudokinases. Proc Natl Acad Sci U S A. 2011 Jun 7;108(23):9631–9636.
- Choi J, Biering SB, Hwang SQV. Interferon-inducible GTPases go to their target membranes via the LC3-conjugation system of autophagy. Small GTPases. 2017 Oct 2;8(4):199–207.