ABSTRACT
Introduction
Conventionally, vaccines are thought to induce a specific immune response directed against a target pathogen. Long recognized but poorly understood nonspecific benefits of vaccination, such as reduced susceptibility to unrelated diseases or cancer, are now being investigated and may be due in part to “trained immunity’.
Areas covered
We discuss ‘trained immunity’ and whether vaccine-induced ‘trained immunity’ could be leveraged to prevent morbidity due to a broader range of causes.
Expert opinion
The prevention of infection i.e. maintaining homeostasis by preventing the primary infection and resulting secondary illnesses, is the pivotal strategy used to direct vaccine design and may have long-term, positive impacts on health at all ages. In the future, we anticipate that vaccine design will change to not only prevent the target infection (or related infections) but to generate positive modifications to the immune response that could prevent a wider range of infections and potentially reduce the impact of immunological changes associated with aging. Despite changing demographics, adult vaccination has not always been prioritized. However, the SARS-CoV-2 pandemic has demonstrated that adult vaccination can flourish given the right circumstances, demonstrating that harnessing the potential benefits of life-course vaccination is achievable for all.
1. Introduction
It has long been recognized that the immune system ‘learns’ by adapting to new antigens on exposure, and responding to them as either potentially pathogenic, harmless, or somewhere on a spectrum in-between. The possible outcomes of this learning process – among them, immunity to infection, allergy, or tolerance – shape many aspects of our overall health. For some pathogens, a single infectious episode can lead to lifelong immunity, indicating that the immune system has learnt to effectively neutralize subsequent exposures to the same pathogen. The ability of the immune system to ‘learn’ underlies the concept of vaccination, whereby a small dose of a target antigen or modified pathogen teaches the immune system to recognize the same antigen/pathogen when exposed to it at a later date, and to reproduce a highly specific protective immune response that prevents disease on subsequent exposure.
Until relatively recently, the conventional view was that vaccines induced a specific immune response directed only against the pathogen included in the vaccine, or against closely related pathogens that shared similar antigens, and this philosophy underlaid vaccine design. Yet as far back as the 1800s, physicians observed that persons who had been inoculated against smallpox had reduced susceptibility to other unrelated diseases, such as measles, whooping cough, scarlet fever, and syphilis [Citation1]. Populations that receive Bacillus Calmette–Guérin (BCG) vaccine have reduced incidence of non-tuberculous respiratory infections, cancer, and lower overall mortality compared to unvaccinated populations, and this has been known since BCG vaccine was first used in the 1920s [Citation2,Citation3]. Indeed, the first explicit speculations of that BCG vaccine induced some sort of beneficial nonspecific immune response date from that period [Citation4]. These effects extend beyond infectious disease: BCG vaccine is an effective treatment for some urothelial cell cancers [Citation5], and it may help reduce the risk of type I and type 2 diabetes [Citation6,Citation7]. Similarly, it has been observed that rotavirus vaccination also appears to reduce the risk of some autoimmune diseases such as type 1 diabetes mellitus and celiac disease [Citation8,Citation9], although the extent of this benefit remains controversial. The mechanism by which vaccines that target a specific pathogen can reduce the risk of infection caused by a different one, or even reduce diseases due to a presumably unrelated process such as cancer or diabetes, has long been obscure. Several hypotheses have been advanced such as cross-reactivity or heterologous adaptive immunity [Citation10]. However, recent work suggests an important role for epigenetic re-programming of innate immune cells through a process known as ‘trained immunity’ [Citation11]. In this review, we summarize what is known about ‘trained immunity’ in the context of vaccination and consider briefly whether it could be leveraged to prevent morbidity due to a broader range of causes – for example, infection with SARS-CoV-2, or other viral pathogens with pandemic potential. We also discuss these issues in the light of the concept of ‘immune fitness’ and the wider role of vaccines in maintaining a ‘fighting fit’ immune system throughout life.
2. A refresher course in the immune response to infection
The immune response is generally divided broadly into what are called innate and adaptive responses [Citation12]. Invading pathogens are detected by the innate immune system through molecular sensing surveillance mechanisms. When exposed to a pathogen, innate immune cells such as macrophages, monocytes, neutrophils, and dendritic cells use soluble and cell-bound pattern recognition receptors (PRRs) to recognize specific immunostimulants that identify that pathogen as a threat [Citation13]. The targets of these PRRs (called PAMPS, or Pathogen-associated Molecular Patterns) are typically fragments of molecules such as nucleic acids or lipids that are common to many families of microorganisms, but which are absent or expressed in a very restricted fashion in the host. Signaling via PRRs initiates immune processes such as phagocytosis and inflammation. The inflammatory response includes the activation of complement, release of cytokines and other inflammatory mediators that recruit inflammatory cells to the site of infection. Phagocytosis is mediated by innate immune cells (macrophages, dendritic cells, and neutrophils). The movement of antigens to draining lymph nodes by dendritic cells, and uptake and presentation of antigens by antigen presenting cells (APCs), activate the initial phases of the adaptive response [Citation14] ().
The exact nature of innate immune response that occurs influences the activation of B cell and T cell subsets that determine the type of adaptive response that follows [Citation15]. Different PRRs recognize different PAMPs expressed by different classes of pathogens (viruses or bacteria) and the activation of different combinations of PAMPs leads to different responses by innate immune cells. For example, certain lipopolysaccharides common to bacterial membranes may provoke the activation of innate immune cell processes that promote the kind of inflammatory profile associated with bacterial infection (and sepsis). By contrast, recognition of certain nucleic acids, such as 5′ triphosphate RNA, which is uncommon in mammalian hosts, may promote immune pathways associated with anti-viral responses [Citation13]. These initial innate immune responses are not specific to individual pathogens but neither are they wholly nonspecific.
The kind of innate immune response that develops in the earliest stages of immune recognition can help shape the nature of the adaptive immune response that subsequently develops; for example, by altering the proportion of B cells that differentiate into plasma cells releasing into the bloodstream antigen-specific antibodies (immunoglobulin G [IgG]), and the proportion of those that migrate to mucosal surfaces such as the respiratory system or the gut and release IgA [Citation16]. This differential response leads to the development of the different populations of memory cells that are capable of responding rapidly and appropriately to subsequent exposures to that specific pathogen. Likewise, the type of T cells that develop after activation by APCs influences the inflammatory mediators released by the activated cluster of differentiation 4 positive (CD4+) T helper (Th) cell sub-populations (Th1, Th2, Th17, Tfh), which in turn influence how effectively the pathogen can be removed or contained [Citation17]. CD8+ cytotoxic T cells activated by the innate response can also activate a range of different behaviors, such as killing cells directly or via the release of cytotoxins.
This series of events is conventionally presented as a linear process: first the activation of innate immune cells, then that of adaptive immune cells, then the development of long-term immune memory. However, it is better understood as a series of feedback loops that can either accelerate or inhibit the development of different types of immune response. Thus, the recruitment and activation of different subsets of innate and adaptive immune cells, and the microenvironment in which interactions occur, shape the evolving adaptive immune responses, and feedback from these cells can in turn alter the ongoing innate immune response [Citation18]. This interaction means that even relatively small differences in the early stages of the immune response can lead to quite divergent final outcomes.
Since the type of immune stimulus that activates the innate immune response can influence the quality and extent of an APC’s activation, directly translating into the quality and extent of antigen-specific adaptive responses, the possibility exists to directly manipulate these innate responses to improve the effectiveness of the subsequent memory response. Vaccine adjuvants, which are immune modulators added to vaccines to direct and optimize the immune response, exploit this possibility. Initially, adjuvants were selected empirically to stimulate broader, stronger responses than when the antigen was given alone. As our understanding of the underlying immune networks has improved, better understanding of the modes of action of adjuvants has allowed a rational selection of adjuvant components to better guide the immune response [Citation19].
3. How the innate immune system learns
Classical immunology suggests that the innate immune system is a primitive, undifferentiated response to a threat and that the flexibility and plasticity of the immune system as a whole rests solely with the adaptive response. This paradigm has evolved, in light of the increasingly strong knowledge that the innate immune system is capable of ‘learning,’ by a process known as ‘trained immunity,’ and of maintaining a kind of immune memory that is different from that of B cells and T cells [Citation11]. Unlike classical memory, ‘trained immunity’ is not based primarily on clonal expansion but on a relatively stable functional reprogramming that makes cells more prone to react to specific immune stimuli, including feedback from activated adaptive immune cells; reprogramming brings about fundamental and persistent changes in the metabolism of innate effector cells and their stem cells [Citation20,Citation21]. These changes occur at the level of the genome and gene transcription machinery (epigenetic modifications). The result is that the innate effector cells may respond either more or less strongly to subsequent stimuli. The metabolic alterations that trigger these events occur via numerous pathways, such as a switch from oxidative phosphorylation to aerobic glycolysis, and changes in glutamine metabolism and cholesterol synthesis [Citation22]. These changes can impact histones, change the degree of DNA methylation, lead to chromatin re-configuration and modify which (or how much) PRRs are expressed on cell surfaces [Citation22,Citation23]. These effects are known as epigenetic reprogramming and while they have no known impact on the DNA code itself, they alter how the code is exposed, read, and transcribed. ()
Figure 2. Trained immunity concept: Innate immune cells are epigenetically reprogrammed during a first infection or vaccination, allowing an enhanced response upon a secondary exposure.
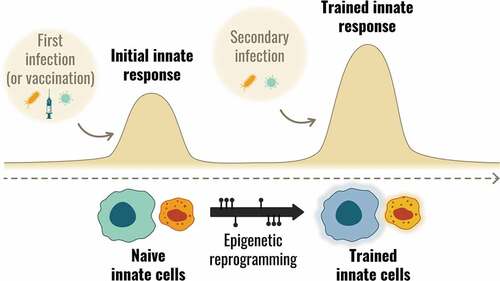
Modification of histones is an important mechanism underlying ‘trained immunity’ [Citation24]. Histones are structural proteins that wrap DNA into a condensed form (nucleosomes) in the nucleus. Modifications to the histone tail by acetylation of lysine or methylation of lysine or arginine have opposing actions on the architecture of the surrounding chromatin, making it easier or harder for the protein complexes involved in transcription to access gene promoter regions, respectively, thus leading to increased or decreased transcription [Citation23]. Acetylation (generally linked to increased transcription) and methylation (generally linked to decreased transcription), at the extremes can promote a state of hyper-inflammation or immune tolerance, respectively [Citation23,Citation25]. Long-term changes to myeloid cell populations can be brought about by epigenetic, transcriptomic, and functional reprogramming of myeloid stem cells in the bone marrow.
The clinical implications of ‘trained immunity’ are profound, with potentially far-reaching impacts for disease prevention and treatment. ‘Trained immunity,’ and the modification of initial innate immune responses by preexisting immune responses, could help explain some of the variability observed in the severity of infectious diseases experienced by different individuals. From a clinical perspective, targeted induction of ‘trained immunity’ could be used in to help prevent infections, prevent and treat cancers, and prevent autoimmune or other inflammatory diseases [Citation25–28]. As yet, these opportunities remain largely theoretical, although immune activation using bacteria or bacterial toxins have been used to treat cancer (with variable degrees of success) for over a century [Citation29,Citation30]. The most successful of these therapies uses BCG vaccine to treat urothelial cell cancers and remains in widespread use. The mechanism was initially unknown [Citation5], but is now thought to be due to ‘trained immunity’ rather than BCG antigen-specific immunity [Citation27,Citation31]. On the other hand, caution remains about inducing nonspecific responses by immunotherapy due to the concern that inappropriate activation of ‘trained immunity’ could have adverse effects, potentially contributing to the onset or exacerbation of autoimmune diseases [Citation32].
4. Why is ‘trained immunity’ relevant to vaccines?
Vaccination induces an immunological stimulus and there is growing evidence that some vaccines (BCG is only one of these, albeit the most studied), can induce ‘trained immunity’ alongside specific immune responses. If true, this could partly explain the observations of the broader protective effects of some vaccinations (i.e. beyond reduction of the burden of the targeted disease).
‘Trained immunity’ induced by BCG vaccination is now well described [Citation33,Citation34]. BCG vaccine given to healthy persons causes metabolic, epigenetic, and transcriptional reprogramming of myeloid cells. This results in sustained changes in the expression of specific cell surface activation markers such as toll-like receptor 4, known to recognize many PAMPs including bacterial and viral antigens, and CD14 and CD11b, which recognize bacterial lipopolysaccharide and have possible roles in cell-mediated cytotoxicity, phagocytosis, and viral clearance [Citation35,Citation36] As a result, when exposed to unrelated pathogens, these myeloid cells are primed for enhanced production of pro-inflammatory cytokines [Citation37]. The shared nature of many innate immune triggers means that this immunity is far broader in scope than that dependent on adaptive immunity. BCG vaccine is known to improve the protective capabilities of the immune system against unrelated pathogens for up to 1 year after vaccination [Citation38,Citation39], and a few studies suggest that beneficial effects for some conditions may last significantly longer [Citation2]. Epigenetic modulation after BCG vaccination is also associated with long-term, durable induction of regulatory T cells, potentially extending the effect of therapy over substantial periods – up to three years in one study [Citation40].
Nonetheless, some basic questions remain. Do other existing vaccines have the potential to induce ‘trained immunity’? Can ‘trained immunity’ induced by BCG or other vaccines be harnessed to reduce the risk of diseases – infectious and potentially non-communicable ones – for which vaccines are not currently available?
4.1. Can vaccine-induced ‘trained immunity’ prevent COVID-19?
In May 2019, Moolag et al. [Citation41] presciently opined that BCG vaccination could be used as a novel strategy to protect against viral infections that cause global pandemics, and for which an effective vaccine was not yet available. Within less than a year, the world was grappling with the early stages of the SARS-CoV-2 pandemic and the question of a potential role for BCG vaccination until specific vaccines became available was raised [Citation42]. Attempts were made to see if the incidence and severity of COVID-19 was different in countries that practiced infant BCG vaccination, even though, given that ‘trained immunity’ is relatively short-lived, it seemed unlikely that BCG vaccination during infancy would still be exerting a measurable impact through adulthood. One epidemiological study suggested an association between BCG vaccination and reduced COVID-19 mortality [Citation43], but subsequent studies have produced very different outcomes – perhaps in part because the degree of confounding inherent to these retrospective analyses hindered interpretation of the data [Citation43–45].
Another approach has been to try and evaluate population levels of ‘trained immunity’ using tuberculin immunoreactivity as a proxy. A positive tuberculin test using the Mantoux test, or an interferon gamma release assay is used to identify latent tuberculosis (TB), suggestive of immunity in the absence of active TB disease. A positive Mantoux test can also indicate environmental exposure to Mycobacterium species – including antigens present in the BCG vaccine. Persistence of tuberculin immunoreactivity is associated with reduced mortality from non-TB diseases and has been proposed to estimate the level of ‘trained immunity’ in the population [Citation44]. Population-based data for Europe report the prevalence of latent TB in different countries. An analysis of COVID-19 and the prevalence of latent TB in individual European countries showed a strong negative correlation, with lower rates of COVID-19 cases and mortality in countries where latent TB was more prevalent [Citation44]. These results can only be considered suggestive – the observational study in question highlights the need for careful selection of controls in studies of ‘trained immunity’ given the many types of immune stimuli that can influence the epigenome.
Clinical trials are underway to address, and hopefully definitively answer, the specific question as to whether ‘trained immunity’ induced by BCG vaccination can prevent or modulate infection caused by SARS-CoV-2 [Citation46]. Fortunately, effective vaccines for the SARS-CoV-2 pandemic became rapidly available, but the question of whether vaccines such as BCG could help to reduce the risk or severity of viral infections remains relevant as new viral threats emerge.
For other vaccines available, the evidence is preliminary but intriguing. The probability of a positive SARS-CoV-2 test was decreased in adults <65 years of age who had prior vaccination with a pneumococcal vaccine or an influenza vaccine, and in adults aged ≥65 years of age who had received a pneumococcal vaccine previously [Citation47]. SARS-CoV-2 infection was also significantly less common in hospital employees who had received an influenza vaccination [Citation48]. The authors found that immune cells collected after influenza vaccination of healthy individuals showed improved responsiveness to a range of viral stimuli, as well as an impact on the specific immune response to SARS-CoV-2 [Citation48]. All these observations are suggestive of a trained immune response.
Other data have emerged that suggest a protective effect of the recombinant herpes zoster vaccine (RZV, GSK) on SARS-CoV-2 infection. RZV contains AS01, a liposome-based adjuvant comprising 3-O-desacyl-4′-monophosphoryl lipid A (MPL), a Toll-like receptor 4 ligand and QS-21, a saponin extracted from the bark of the Quillaja saponaria Molina tree. AS01 interacts effectively with the innate immune system and activates specific pathways that lead to enhanced adaptive responses and improved antigen-specific responses when combined into a vaccine [Citation19]. RZV has been shown to cause expansion of the T cell repertoire in older adults [Citation49]. A case-control study in the United States found that adults ≥50 years of age who had been vaccinated with RZV had a 16% lower risk of having a COVID-19 diagnosis, and a 32% lower risk of hospitalization compared to unvaccinated adults. This suggests that RZV elicits heterologous protection, possibly through ‘trained immunity’ [Citation50]. This possibility triggered further investigations into the implications of vaccine adjuvants in ‘trained immunity,’ and the potential for such effects to confer protection of individuals against unrelated diseases.
4.2. ‘Trained immunity’ and aging
As individuals age, their immune system also undergoes changes that result in a state of chronic low-grade inflammation with associated reduction in their ability to respond to new threats and an increased risk of developing autoimmune diseases and cancer [Citation51–53]. This process is often referred to as ‘inflammaging.’ However, the speed at which ‘inflammaging’ and functional decline of the immune system occurs in aging individuals is highly variable: the functional age of the immune system (often called the ‘bioage’) cannot be predicted simply from the chronological age of an individual. ‘Bioage’ is influenced by numerous intrinsic and extrinsic factors, including genetic makeup, diet, exercise, sex, exposure to toxins such as smoking and alcohol, the health of the microbiome, psychological stress and other environmental exposures [Citation54]. These factors can alter the epigenome and directly influence the functioning of the immune system, affecting the overall state of wellness or illness. The changes to the epigenome typical of aging is visible at the level of histones, by a progressive reduction in DNA methylation that causes expression of DNA sequences that are normally silenced, also by hypermethylation of some regions with suppression of promoter regions that are normally active, and by a general loss of heterochromatin. In effect, these changes alter the levels of gene transcription and can lead to immune system dysregulation [Citation52,Citation55].
These DNA methylation levels can be used as markers of ‘bioaging’ and are sometimes referred to as an ‘epigenetic clock.’ The accumulation of such markers can be accelerated, or slowed, or even possibly reversed by given factors, although research is only beginning in this field. However, in general, the impact of external or environmental factors on the ‘epigenetic clock’ increases as individuals age. The outcome of these external factors may not always be negative, theoretically making the ‘epigenetic clock’ amenable to positive intervention to maintain immune health for longer. It appears that the ‘epigenetic clock’ can be accelerated by some chronic infections such as cytomegalovirus (CMV) and human immunodeficiency virus (HIV) infections [Citation56]. The ‘epigenetic clock’ can be slowed down, perhaps predictably, by a healthy diet and physical exercise [Citation57–59].
But what about vaccination? We now know that some vaccines can bring about changes to the epigenome, expressed as ‘trained immunity’ (discussed in more detail in Section 4.0, above). Could the vaccine effects on the epigenome slow down epigenetic aging? As yet, there is no definitive answer to this question but observations that vaccination is associated with reductions in the overall death rate and improvements in longevity [Citation60] strongly suggest that vaccines contribute positively to immune health, helping to promote immune fitness which in itself can contribute to a longer and healthier life. Additionally, good diet, physical exercise, avoidance of toxins, screening for common diseases to promote early diagnosis and treatment, and vaccination to prevent infection are also key contributors to maintaining good health [Citation61,Citation62].
5. The role of vaccines in maintaining a ‘fighting fit’ immune system
A ‘fit’ immune system is one that can respond appropriately to any immune challenge, resulting in disease prevention and ongoing health, and then when the need has passed, reverts to baseline, or near-baseline levels of response [Citation61], a process referred to as immune homeostasis. Immune homeostasis is maintained by several systems and the ability to switch from inflammation to tissue regeneration and repair is a fundamental characteristic of a ‘fit’ immune system [Citation63–65]. At sites of injury, macrophages and other immune cells promote wound healing and prevent a fibrotic cascade [Citation66], and regulatory T cells are maintained in sufficient numbers in the peripheral pool to prevent autoimmunity and excessive immune responses [Citation67]. Attempts are being made to exploit the regenerative capacity of the immune system to improve clinical outcomes [Citation68]. The failure to maintain homeostasis results in persistent immune activation, which, in T cells, resembles the epigenetic changes associated with aging [Citation55] ().
Figure 3. The concept of immune fitness: (a) Resilience of the immune system and immune fitness (b) Effect of extrinsic modulators of immune fitness.
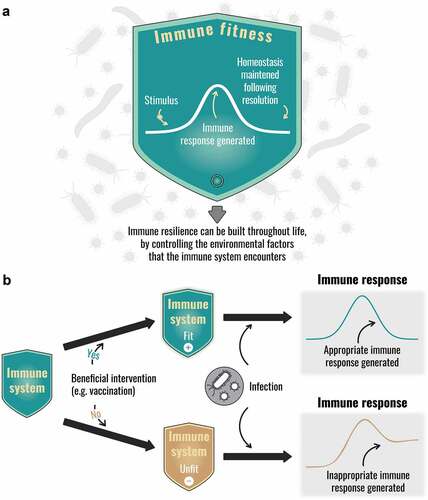
Because of its plasticity, the immune system is shaped by all of the immune encounters it experiences throughout lifetime. Such challenges not only include infections but also all of the stimuli encountered from (for example) food, contact with animals, and other environmental vectors. This means that every person is immunologically unique, and their collected immune responses are sometimes described as their ‘immunobiography.’ Some encounters with infectious diseases, for example, infection with CMV or HIV are known to have long-term deleterious immune effects. Chronic CMV infection induces a state of constant immune system activation, as the infection causes immune exhaustion of CD8+ memory T cells and reduces ability of the latter to respond to subsequent threats [Citation69]. Chronic CMV infection is thought to accelerate the epigenic changes associated with aging [Citation56]. HIV infection also results in immune dysregulation characterized by T cell activation, inflammation, and coagulopathy that results in morbidities typical of older populations, such as cardiovascular disease, osteoporosis, diabetes, cancer, and neurocognitive dysfunction [Citation70].
Vaccines can make multi-faceted contributions to health. In the first instance, vaccines reduce the risk of diseases caused by vaccine-targeted infectious agents, thus preventing periods of ill-health, the potential short- and long-term complications of those diseases, and any far-reaching effects of these complications on long-term health. The example of BCG vaccine was discussed above, but it is not unique in having broad-ranging, off-target beneficial protective effects. For example, measles vaccination reduces all-cause mortality by a greater degree than can be accounted for by the direct impact of measles mortality [Citation71]. Measles infection induces life-long immunity but results in a prolonged state of immune paralysis through restricted innate anti-viral immune signaling, impaired dendritic cell functioning, restricted secretion of pro-inflammatory cytokines, a loss of up to 40% of the diversity of antibody repertoire, and a decreased capacity of the immune system to identify pathogenic epitopes and bind to them [Citation71,Citation72]. Children who develop measles are therefore more likely to sustain other infections and become unwell (or die), with long-term implications for their growth as well as physical and cognitive development. Measles vaccination, on the other hand, also induces life-long protection against disease but is not associated with immune paralysis or loss of immune memory to other infections [Citation73].
Measles vaccination has also been associated with reduced long-term, negative impacts on development and improved educational and economic outcomes. It has been associated with cognitive gains compared to unvaccinated children in India, Ethiopia, and Vietnam [Citation74], while childhood vaccination more generally has been associated with increased cognitive ability in young teenagers in the Philippines [Citation75]. Thus, through primary disease prevention with consequent downstream benefits on childhood health [Citation74], vaccines appear to contribute to long-term health and well-being, with profound implications for life expectancy, life-time productivity, and economic development.
Influenza disease has similarly profound implications for long-term health. Respiratory infections, myocardial infarction, and deaths due to cardiovascular disease all increase in older adults during influenza seasons [Citation76,Citation77], a disease burden that is reduced in vaccinated individuals [Citation78]. Immune changes following influenza infection have been proposed to directly contribute to the development of atherosclerosis, something that influenza vaccination may reduce, and vaccination is now generally recognized as a preventative therapy for coronary artery disease [Citation79]. Other infections also apparently increase the risks for non-communicable disease: for example, infection has long been associated with elevated risk for stroke, with acute infection or reactivation of chronic varicella zoster virus (VZV) infection the commonest [Citation80–82]. Perhaps not surprisingly, given this association, vaccination against VZV appears to significantly reduce the risk of both ischemic and hemorrhagic stroke [Citation81]. The postulated mechanism appears similar to that mentioned above for influenza i.e. inflammation driven tissue-remodeling and thrombosis, triggered by the infection. However, recent studies indicate that vaccination against VZV also significantly reduces the risk of subsequently developing dementia [Citation83,Citation84], as does antiviral treatment [Citation85]. While the simplest hypothesis is that similar mechanisms to those involved in elevated stroke risk after influenza infection may also cause neurological damage after VZV infection, thus raising the risk of dementia, a broader analysis of the data suggests that something different is going on. Unlike the situation for stroke, zoster incidence is weakly, if at all, associated with risk of dementia [Citation86–88]. Moreover, adult vaccination in general appears broadly associated with a decreased risk of dementia [Citation89,Citation90]. These observations are more consistent with a generalized immune benefit leading to reduced risk of dementia, rather than an effect driven by control of a specific pathogen.
6. Conclusion
It has been postulated for over a century that vaccination can have, in some cases, health benefits significantly beyond those attributable to the prevention of acute illness from the vaccine-targeted infection. Such benefits have been attributed to induction of nonspecific immune responses, although the mechanisms were unknown [Citation4]. Recent advances in immunology have paved the way for understanding how vaccines exert these broader effects [Citation27,Citation31,Citation71,Citation72]. At the same time, large-scale data analyses of public health databases suggest these beneficial effects may be more frequent and substantial than previously appreciated [Citation2,Citation3,Citation6,Citation7,Citation89,Citation90]. We have reached the point where the benefits of vaccination could be deliberately harnessed to maintain a fit immune system, leading to health benefits than can potentially eclipse the benefits due to prevention only of acute illness.
Current data suggest that vaccines contribute to a fit immune system in two ways (1) by preventing targeted infectious diseases and the whole spectra of long-term adverse health consequences following such infections; (2) by driving long-term changes in metabolism or ‘trained immunity,’ thus leading to broader protective effects to diverse pathogens, or even reducing the risks for certain chronic, non-communicable diseases such as cardiovascular disease, stroke, dementia, and diabetes. As yet, the use of targeted vaccine-induced ‘trained immunity’ for wider disease prevention remains theoretical but given that the non-communicable diseases named above are among the leading causes of premature mortality, even minor reductions in incidence could provide very large savings in life, quality of life and healthcare costs. What is not theoretical is that, along with lifestyle factors such as diet and exercise, vaccination has an unambiguous role in promoting good health in older adults.
7. Expert Opinion
Vaccines have contributed more to human health than any other intervention, aside from provision of clean water [Citation91]. However, we are only beginning to understand the broader benefits of vaccines to immune homeostasis and health. The role of vaccines in preventing primary disease and the resulting benefits to society in terms of health, wellbeing and productivity of individuals is undisputed, but the precise mechanisms of vaccination as a tool to contribute to the maintenance of good health over a lifetime are poorly described. The prevention of any infection, whether parasitic, bacterial, or viral, and whether acute or chronic, can potentially have long-term, positive impacts on health at all ages. When an infection occurs, its long-term effects can be immunological, physical, emotional, and cognitive, with individual, familial, and economical consequences. The prevention of infection i.e. maintaining homeostasis by preventing the primary infection and secondary illnesses that arise a result of the infection, is most probably going to stay as a pivotal public health strategy, and in the future, it may involve novel vaccine design strategies directed to -promote immune homeostasis. In theory, this can go beyond preventing secondary effects of infection, important as that is. In the future, we indeed anticipate that vaccine design will be applied to generate vaccines with the ability to confer broad, positive modifications to the immune response or metabolism in a way to prevent a wider range of illnesses, in much the same way as the presumed improving effect of BCG vaccination on glycemic control [Citation92]. Some researchers are even speculating that producing anti-aging vaccines might be an achievable task, by targeting host cell processes involved in aging-related physiological defects [Citation93,Citation94], thus reducing or slowing the loss-of-function associated with the process of aging.
This may sound highly hypothetical, but it is worth remembering that the cells of the immune system are not purely devoted to immune surveillance and response. Immune cells also play critical roles in tissue homeostasis and tissue remodeling that underlies regenerative processes, and these processes are also potential therapeutic targets [Citation95]. Further, the accumulation of senescent endothelial cells in vascular tissue is associated with risk of development of atherosclerosis and diabetes [Citation93] – two conditions where vaccination is known to have potentially beneficial off-target effects [Citation6,Citation7,Citation79].
The observations of nonspecific vaccine effects, though broadly reported, remain controversial. Some studies suggest that inactivated vaccines may cause immune interference with other vaccines, leading to adverse nonspecific impacts on all-cause mortality [Citation96]. Others studies of BCG conducted in a high-income country did not replicate the findings observed in low-income countries [Citation97–99]. A significant issue with data interpretation is that findings from small observational studies have not always been confirmed in clinical trials. Plus, there is the possibility that unaddressed confounders might explain some of the results in observational studies. For example, healthy vaccinee bias may impact outcomes when seeking vaccination acts as a proxy for a healthier lifestyle and behaviors that limit exposure to infectious agents. Data from randomized, blinded clinical trials, however, provide compelling evidence that these kinds of bias cannot explain all of the effects observed. Randomized, blinded clinical trials have directly evaluated the protective effect of influenza vaccination on cardiovascular or cerebrovascular outcomes and mortality [Citation100–102]. While these have been largely small to medium-sized studies and conducted in high-risk populations, which limits their generalizability, the reduction in mortality in these trials has replicated the protective effects seen in observational studies. Unlike observational studies, the reduction in mortality in randomized controlled trials cannot be due to confounding arising from health seeking behavior or healthy vaccinee bias [Citation100–102]. These data suggest that considerable health benefits in the form of reduced risk for cardiac disease, strokes and possibly even cognitive decline can be obtained with improved vaccination coverage.
Despite the proven and theoretical benefits of vaccination, these can only be obtained if vaccines are actually administered. The first vaccines were originally designed for infants and children. Most vaccines become less effective during later adulthood or old age. Development of vaccines that are equally effective in older adults has been limited until relatively recently by empirical vaccine design approaches. The toolbox of available technologies for developing vaccines that are highly effective in older adults continues to expand [Citation103], and construction of a vaccine portfolio developed specifically for prevention of disease in older adults is emerging as a public health goal [Citation104]. In addition, vaccination of adults has historically been plagued by problems of access, complacency, and indifference, leading to low coverage rates. In 2022, we are seeing unprecedented vaccine uptake by adults of vaccines targeting COVID-19, brought about by a combination of high public awareness for the disease, and media and government buy-in supporting adult vaccination. This experience has illustrated what can be achieved when the priorities of industry, public health and governments are aligned. Vaccine delivery to adults has been streamlined through investments in infrastructure and an expanded vaccination workforce, including provision of vaccination by pharmacists outside of the clinic setting. Relentless media coverage and targeted messaging by governments and public health bodies have raised health literacy and made vaccines/vaccination topics of dinner-time conversation. The SARS-CoV-2 pandemic will pass. The burden of disease due to infections such as influenza and VZV will not. In fact, with aging populations, this burden is expected to increase [Citation105]. The SARS-CoV-2 pandemic has demonstrated that adult vaccination can flourish given the right circumstances. However, the investments made under pandemic conditions need to be maintained to permanently increase access to all adult routine vaccinations. Lessons learned during the pandemic should not be lost or squandered, but long-term sustainable solutions such as continued access to vaccination at pharmacies are needed if we wish to ensure that the potential benefits of life-course vaccination are achievable by all.
Article highlights
Non-specific effects of vaccines on unrelated diseases, including other infections and cancer, have been postulated since at least the early 20th century.
These non-specific effects may be due in part to epigenetic reprogramming of innate immune cells, leading to ‘“trained immunity”’.
Vaccination induces an immunological stimulus and some vaccines (eg BCG) induce ‘trained immunity’ alongside specific immune responses. As a result, myeloid cells show enhanced production of pro-inflammatory cytokines and provide protection against unrelated pathogens that can last for a year or longer after vaccination.
By preventing infection, vaccines may contribute to good health and immune fitness by helping to maintain immune homeostasis.
As yet, the deliberate use of targeted vaccine-induced ‘trained immunity’ for wider disease prevention remains speculative. Nonetheless, along with lifestyle factors such as diet and exercise, vaccination has an unambiguous role in promoting good health and immune fitness.
Box: glossary
Bioage: biological age measured by several available tools that aim to predict health and remaining healthy lifespan and life expectancy
Epigenetics: modifications to gene expression brought about by altered access to transcription factors, promoter regions, silencer regions, through acetylation, methylation, or phosphorylation of histones.
Histones: structural proteins that wrap DNA within the nucleus and play a role in regulating gene expression by ‘opening’ or ‘closing’ wound DNA structures, allowing increased/decreased access to transcription factors.
Immune biography: the overall immune responsiveness that arises as the sum of all of the immune encounters experienced throughout a lifetime
Immune fitness: a resilient immune system capable of adapting to external challenges by employing an appropriate immune response and returning to baseline or near baseline levels of activation.
Immunosenesence: age-related, progressive modulation of immune system functioning, often associated with gradually developing chronic inflammation.
‘Trained immunity’: re-programming of innate immune cells that causes them to respond differently to immune activation by acquiring a form of immune memory
Declaration of interest
Béatrice Laupèze and T. Mark Doherty are employed by and hold shares in GSK. GSK have no other relevant affiliations or financial involvement with any organization or entity with a financial interest in or financial conflict with the subject matter or material discussed in the manuscript apart from those disclosed.
Reviewer disclosures
Peer reviewers on this manuscript have no relevant financial or other relationships to disclose.
Author contributions
All authors participated in the discussion and the development of this manuscript, reviewed and approved the final manuscript.
Acknowledgments
The authors thank Business & Decision Life Sciences platform for editorial assistance and publication coordination, on behalf of GSK. Joanne Wolter (independent medical writer on behalf of GSK) for providing medical writing support.
Additional information
Funding
References
- Mayr A. Taking advantage of the positive side-effects of smallpox vaccination. J Vet MedInfect Dis Vet Public Health. 2004;51(5):199–201.
- Usher NT, Chang S, Howard RS, et al. Association of BCG vaccination in childhood with subsequent cancer diagnoses: a 60-year follow-up of a clinical trial. JAMA network open. 2019;2(9):e1912014.
- Higgins JP, Soares-Weiser K, Lopez-Lopez JA, et al. Association of BCG, DTP, and measles containing vaccines with childhood mortality: systematic review. BMJ. 2016;355:i5170.
- Schaltz-Buchholzer F, Kjaer Sorensen M, Benn CS, et al. The introduction of BCG vaccination to neonates in Northern Sweden, 1927–1931: re-analysis of historical data to understand the lower mortality among BCG-vaccinated children. Vaccine. 2022;40(11):1516–1524.
- Redelman-Sidi G, Glickman MS, Bochner BH. The mechanism of action of BCG therapy for bladder cancer–a current perspective. Nat Rev Urol. 2014;11(3):153–162.
- Doupis J, Kolokathis K, Markopoulou E, et al. The role of pediatric BCG vaccine in type 1 diabetes onset. Diabetes Ther. 2021;12(11):2971–2976.
- Corsenac P, Parent ME, Mansaray H, et al. Early life bacillus calmette-guerin vaccination and incidence of type 1, type 2, and latent autoimmune diabetes in adulthood. Diabetes Metab. 2022;48(3):101337.
- Gómez-Rial J, Sánchez-Bátan S, Rivero-Calle I, et al. Rotavirus infection beyond the gut. Infect Drug Resist. 2019;12:55–64.
- Rogers MAM, Basu T, Kim C. Lower incidence rate of type 1 diabetes after receipt of the rotavirus vaccine in the United States, 2001–2017. Sci Rep. 2019;9(1):7727.
- Messina NL, Zimmermann P, Curtis N. The impact of vaccines on heterologous adaptive immunity. Clin Microbiol Infect. 2019;25(12):1484–1493.
- Netea MG, Dominguez-Andres J, Barreiro LB, et al. Defining trained immunity and its role in health and disease. Nat Rev Immunol. 2020;20(6):375–388.
- Moser M, Leo O. Key concepts in immunology. Vaccine. 2010;28(Suppl 3):C2–13.
- Ishii KJ, Koyama S, Nakagawa A, et al. Host innate immune receptors and beyond: making sense of microbial infections. Cell Host Microbe. 2008;3(6):352–363.
- Leo O, Cunningham AL, Stern P. Vaccine immunology. Perspectives Vaccinol. 2011;1(1)25–59.
- Imler JL, Hoffmann JA. Toll receptors in innate immunity. Trends Cell Biol. 2001;11(7):304–311.
- Reinhardt RL, Liang HE, Locksley RM. Cytokine-secreting follicular T cells shape the antibody repertoire. Nat Immunol. 2009;10(4):385–393.
- Luckheeram RV, Zhou R, Verma AD, et al. CD4⁺T cells: differentiation and functions. Clin Dev Immunol. 2012;925135:2012.
- Greene JT, Brian BF, Senevirathne SE, et al. Regulation of myeloid-cell activation. Curr Opin Immunol. 2021;73:34–42
- Didierlaurent AM, Laupeze B, Di Pasquale A, et al. Adjuvant system AS01: helping to overcome the challenges of modern vaccines. Expert Rev Vaccines. 2017;16(1):55–63.
- Dominguez-Andres J, Netea MG. Long-term reprogramming of the innate immune system. J Leukoc Biol. 2019;105(2):329–338.
- Sanchez-Ramon S, Conejero L, Netea MG, et al. Trained immunity-based vaccines: a new paradigm for the development of broad-spectrum anti-infectious formulations. Front Immunol. 2018;9:2936
- Sohrabi Y, Godfrey R, Findeisen HM. Altered cellular metabolism drives trained immunity. Trends Endocrinol Metab. 2018;29(9):602–605.
- van der Heijden C, Noz MP, Joosten LAB, et al. Epigenetics and trained immunity. Antioxid Redox Signal. 2018;29(11):1023–1040.
- Rusek P, Wala M, Druszczynska M, et al. Infectious agents as stimuli of trained innate immunity. Int J Mol Sci. 2018;19(2):465.
- Mourits VP, Wijkmans JC, Joosten LA, et al. Trained immunity as a novel therapeutic strategy. Curr Opin Pharmacol. 2018;41:52–58
- Stevens WB, Netea MG, Kater AP et al. ‘Trained immunity’: consequences for lymphoid malignancies. Haematologica. 2016;101(12):1460–1468.
- van Puffelen JH, Keating ST, Oosterwijk E, et al. Trained immunity as a molecular mechanism for BCG immunotherapy in bladder cancer. Nat Rev Urol. 2020;17(9):513–525.
- Ter Steeg L, Dominguez-Andres J, Netea MG, et al. Trained immunity as a preventive measure for surgical site infections. Clin Microbiol Rev. 2021;34(4):e0004921.
- Coley WB. The treatment of malignant tumors by repeated inoculations of erysipelas. With a report of ten original cases. 1893. Clin Orthop Relat Res. 1991;105(6):487.
- Coley WB. The treatment of inoperable sarcoma by bacterial toxins (the Mixed Toxins of the Streptococcus erysipelas and the Bacillus prodigiosus). Proc R Soc Med. 1910;3(Surg Sect):1–48.
- Antonelli AC, Binyamin A, Hohl TM, et al. Bacterial immunotherapy for cancer induces CD4-dependent tumor-specific immunity through tumor-intrinsic interferon-gamma signaling. Proc Natl Acad Sci U S A. 2020;117(31):18627–18637.
- Arts RJW, Joosten LAB, Netea MG. The potential role of trained immunity in autoimmune and autoinflammatory disorders. Front Immunol. 2018;9:298
- Arts RJW, Moorlag S, Novakovic B, et al. BCG vaccination protects against experimental viral infection in humans through the induction of cytokines associated with trained immunity. Cell Host Microbe. 2018;23(1):89–100 e105.
- Kaufmann E, Sanz J, Dunn JL, et al. BCG educates hematopoietic stem cells to generate protective innate immunity against tuberculosis. Cell. 2018;172(1–2):176–190 e119.
- Wang Q, Zhang H, Chen Z, et al. Proliferation of CD11b+ myeloid cells induced by TLR4 signaling promotes hepatitis B virus clearance. Cytokine. 2022;153:155867
- Zanoni I, Granucci F. Role of CD14 in host protection against infections and in metabolism regulation. Front Cell Infect Microbiol. 2013;3:32
- Kleinnijenhuis J, Quintin J, Preijers F, et al. Bacille Calmette-Guerin induces NOD2-dependent nonspecific protection from reinfection via epigenetic reprogramming of monocytes. Proc Natl Acad Sci U S A. 2012;109(43):17537–17542.
- Kleinnijenhuis J, Quintin J, Preijers F, et al. Long-lasting effects of BCG vaccination on both heterologous Th1/Th17 responses and innate trained immunity. J Innate Immun. 2014;6(2):152–158.
- Kleinnijenhuis J, van Crevel R, Netea MG. Trained immunity: consequences for the heterologous effects of BCG vaccination. Trans R Soc Trop Med Hyg. 2015;109(1):29–35.
- Keefe RC, Takahashi H, Tran L, et al. BCG therapy is associated with long-term, durable induction of Treg signature genes by epigenetic modulation. Sci Rep. 2021;11(1):14933.
- Moorlag S, Arts RJW, van Crevel R, et al. Non-specific effects of BCG vaccine on viral infections. Clin Microbiol Infect. 2019;25(12):1473–1478.
- LAJ O'Neill, Netea MG. BCG-induced trained immunity: can it offer protection against COVID-19? Nat Rev Immunol. 2020;20(6):335–337.
- Escobar LE, Molina-Cruz A, Barillas-Mury C. BCG vaccine protection from severe coronavirus disease 2019 (COVID-19). Proc Natl Acad Sci U S A. 2020;117(30):17720–17726.
- Singh S, Maurya RP, Singh RK. Trained immunity” from Mycobacterium spp. exposure or BCG vaccination and COVID-19 outcomes. PLoS Pathog. 2020;16(10):e1008969.
- Hamiel U, Kozer E, Youngster I. SARS-CoV-2 rates in BCG-vaccinated and unvaccinated young adults. JAMA. 2020;323(22):2340–2341.
- Belizario JE. Trained innate immunity, COVID-19 therapeutic dilemma, and fake science. Clinics (Sao Paulo). 2020;75:e2124.
- Noale M, Trevisan C, Maggi S, et al. The association between influenza and pneumococcal vaccinations and SARS-Cov-2 infection: data from the EPICOVID19 web-based survey. Vaccines (Basel). 2020;8(3):471
- Debisarun PA, Gossling KL, Bulut O, et al. Induction of trained immunity by influenza vaccination - impact on COVID-19. PLoS Pathog. 2021;17(10):e1009928.
- Qi Q, Cavanagh MM, Le Saux S, et al. Diversification of the antigen-specific T cell receptor repertoire after varicella zoster vaccination. Sci Transl Med. 2016;8(332):332ra346.
- Bruxvoort KJ, Ackerson B, Sy LS, et al. Recombinant adjuvanted zoster vaccine and reduced risk of COVID-19 diagnosis and hospitalization in older adults. J Infect Dis. 2021;225(11):1915–1922.
- Del Giudice G, Goronzy JJ, Grubeck-Loebenstein B, et al. Fighting against a protean enemy: immunosenescence, vaccines, and healthy aging. NPJ Aging Mech Dis. 2018;4:1.
- Grolleau-Julius A, Ray D, Yung RL. The role of epigenetics in aging and autoimmunity. Clin Rev Allergy Immunol. 2010;39(1):42–50.
- Franceschi C, Salvioli S, Garagnani P, et al. Immunobiography and the heterogeneity of immune responses in the elderly: a focus on inflammaging and trained immunity. Front Immunol. 2017;8:982
- Jasiulionis MG. Abnormal epigenetic regulation of immune system during aging. Front Immunol. 2018;9:197
- Goronzy JJ, Hu B, Kim C, et al. Epigenetics of T cell aging. J Leukoc Biol. 2018;104(4):691–699.
- Kananen L, Nevalainen T, Jylhava J, et al. Cytomegalovirus infection accelerates epigenetic aging. Exp Gerontol. 2015;72:227–229
- Xu M, Zhu J, Liu XD, et al. Roles of physical exercise in neurodegeneration: reversal of epigenetic clock. Transl Neurodegener. 2021;10(1):30.
- Kresovich JK, Park YM, Keller JA, et al. Healthy eating patterns and epigenetic measures of biological age. Am J Clin Nutr. 2021;115(1):171–179.
- Quach A, Levine ME, Tanaka T, et al. Epigenetic clock analysis of diet, exercise, education, and lifestyle factors. Aging (Albany NY). 2017;9(2):419–446.
- Bonanni P. Demographic impact of vaccination: a review. Vaccine. 1999;17(Suppl 3):S120–125.
- Laupèze B, Del Giudice G, Doherty M, et al. Vaccination as a preventative measure contributing to immune fitness. NPJ Vaccines. 2021;6(1):93.
- Philip RK, Attwell K, Breuer T, et al. Life-course immunization as a gateway to health. Expert Rev Vaccines. 2018;17(10):851–864.
- Karin M, Clevers H. Reparative inflammation takes charge of tissue regeneration. Nature. 2016;529(7586):307–315.
- Caballero-Sanchez N, Alonso-Alonso S, Nagy L. Regenerative inflammation: when immune cells help to re-build tissues. FEBS J. 2022;20:335–337.
- Tappenden KA, Deutsch AS. The physiological relevance of the intestinal microbiota–contributions to human health. J Am Coll Nutr. 2007;26(6):679S–683S.
- Eming SA, Hammerschmidt M, Krieg T, et al. Interrelation of immunity and tissue repair or regeneration. Semin Cell Dev Biol. 2009;20(5):517–527.
- Liston A, Gray DH. Homeostatic control of regulatory T cell diversity. Nat Rev Immunol. 2014;14(3):154–165.
- Wang M, Thomson AW, Yu F, et al. T lymphocytes as a therapy for ischemic stroke. Seminars in Immunopathology, 2022. Berlin (Heidelberg): Springer Berlin Heidelberg.
- Souquette A, Frere J, Smithey M, et al. A constant companion: immune recognition and response to cytomegalovirus with aging and implications for immune fitness. GeroScience. 2017;39(3):293–303.
- Lederman MM, Funderburg NT, Sekaly RP, et al. Residual immune dysregulation syndrome in treated HIV infection. Adv Immunol. 2013;119;51–83
- Mina MJ. Measles, immune suppression and vaccination: direct and indirect nonspecific vaccine benefits. J Infect. 2017;74(Suppl 1):S10–S17.
- Mina MJ, Kula T, Leng Y, et al. Measles virus infection diminishes preexisting antibodies that offer protection from other pathogens. Science. 2019;366(6465):599–606.
- Mina MJ, Metcalf CJ, de Swart RL, et al. Long-term measles-induced immunomodulation increases overall childhood infectious disease mortality. Science. 2015;348(6235):694–699.
- Nandi A, Shet A, Behrman JR, et al. Anthropometric, cognitive, and schooling benefits of measles vaccination: longitudinal cohort analysis in Ethiopia, India, and Vietnam. Vaccine. 2019;37(31):4336–4343.
- Bloom DE, Canning D, Shenoy ES. The effect of vaccination on children’s physical and cognitive development in the Philippines. Appl Econ. 2012;44(21):2777–2783.
- Warren-Gash C, Smeeth L, Hayward AC. Influenza as a trigger for acute myocardial infarction or death from cardiovascular disease: a systematic review. Lancet Infect Dis. 2009;9(10):601–610.
- Nguyen JL, Yang W, Ito K, et al. Seasonal influenza infections and cardiovascular disease mortality. JAMA Cardiol. 2016;1(3):274–281.
- Nichol KL. Influenza vaccination in the elderly: impact on hospitalisation and mortality. Drugs Aging. 2005;22(6):495–515.
- Aidoud A, Marlet J, Angoulvant D, et al. Influenza vaccination as a novel means of preventing coronary heart disease: effectiveness in older adults. Vaccine. 2020;38(32):4944–4955.
- Saberi A, Akhondzadeh S, Kazemi S, et al. Stroke: a systematic review. Basic Clin Neurosci. 2021;12(4):427–440.
- Murala S, Nagarajan E, Bollu PC. Infectious causes of stroke. J Stroke Cerebrovasc Dis. 2022;31(4):106274.
- Amlie-Lefond C, Gilden D. Varicella zoster virus: a common cause of stroke in children and adults. J Stroke Cerebrovasc Dis. 2016;25(7):1561–1569.
- Schnier C, Janbek J, Lathe R, et al. Reduced dementia incidence after varicella zoster vaccination in Wales 2013–2020. Alzheimers Dement (N Y). 2022;8(1):e12293.
- Scherrer JF, Salas J, Wiemken TL, et al. Impact of herpes zoster vaccination on incident dementia: a retrospective study in two patient cohorts. PLoS One. 2021;16(11):e0257405.
- Bae S, Yun SC, Kim MC, et al. Association of herpes zoster with dementia and effect of antiviral therapy on dementia: a population-based cohort study. Eur Arch Psychiatry Clin Neurosci. 2021;271(5):987–997.
- Lophatananon A, Mekli K, Cant R, et al. Shingles, Zostavax vaccination and risk of developing dementia: a nested case-control study-results from the UK Biobank cohort. BMJ Open. 2021;11(10):e045871.
- Warren-Gash C, Williamson E, Shiekh SI, et al. No evidence that herpes zoster is associated with increased risk of dementia diagnosis. Ann Clin Transl Neurol. 2022;9(3):363–374.
- Johannesdottir Schmidt SA, Veres K, Sorensen HT, et al. Incident herpes zoster and risk of dementia: a population-based danish cohort study. Neurology. 2022;99(7):e660–e668.
- Wu X, Yang H, He S, et al. Adult vaccination as a protective factor for dementia: a meta-analysis and systematic review of population-based observational studies. Front Immunol. 2022;13:872542
- Wiemken TL, Salas J, Morley JE, et al. Comparison of rates of dementia among older adult recipients of two, one, or no vaccinations. J Am Geriatr Soc. 2022;70(4):1157–1168.
- Greenwood B. The contribution of vaccination to global health: past, present and future. Philos Trans R Soc Lond B Biol Sci. 2014;369:1645.
- Shpilsky GF, Takahashi H, Aristarkhova A, et al. Bacillus Calmette-Guerin ‘s beneficial impact on glucose metabolism: evidence for broad based applications. iScience. 2021;24(10):103150.
- Suda M, Shimizu I, Katsuumi G. al. e. Senolytic vaccination improves normal and pathological age-related phenotypes and increases lifespan in progeroid mice. Nat Aging. 2021;1:1117–1126.
- Mendelsohn AR, Larrick JW. Antiaging Vaccines Targeting Senescent Cells. Rejuvenation Res. 2022;25(1):39–45.
- Piotto C, Julier Z, Martino MM. Immune regulation of tissue repair and regeneration via mirnas-new therapeutic target. Front Bioeng Biotechnol. 2018;6:98
- Aaby P, Benn C, Nielsen J, et al. Testing the hypothesis that diphtheria-tetanus-pertussis vaccine has negative non-specific and sex-differential effects on child survival in high-mortality countries. BMJ Open. 2012;2(3):e000707.
- Biering-Sorensen S, Aaby P, Lund N, et al. Early BCG-Denmark and neonatal mortality among infants weighing <2500 g: a randomized controlled trial. Clin Infect Dis. 2017;65(7):1183–1190.
- Stensballe LG, Ravn H, Birk NM, et al. BCG vaccination at birth and rate of hospitalization for infection until 15 months of age in danish children: a randomized clinical multicenter trial. J Pediatric Infect Dis Soc. 2019;8(3):213–220.
- Thostesen LM, Kjaer HF, Pihl GT, et al. Neonatal BCG has no effect on allergic sensitization and suspected food allergy until 13 months. Pediatr Allergy Immunol. 2017;28(6):588–596.
- Gurfinkel EP, Leon de la Fuente R, Mendiz O, et al. Flu vaccination in acute coronary syndromes and planned percutaneous coronary interventions (FLUVACS) Study. Eur Heart J. 2004;25(1):25–31.
- Gurfinkel EP, de la Fuente RL, Mendiz O, et al. Influenza vaccine pilot study in acute coronary syndromes and planned percutaneous coronary interventions: the FLU Vaccination Acute Coronary Syndromes (FLUVACS) study. Circulation. 2002;105(18):2143–2147.
- Ciszewski A, Bilinska ZT, Brydak LB, et al. Influenza vaccination in secondary prevention from coronary ischaemic events in coronary artery disease: FLUCAD study. Eur Heart J. 2008;29(11):1350–1358.
- Laupèze B, van der Most R, Del Giudice G. Novel technologies to improve vaccines for older adults. In: Weinberger B, editor. Vaccines for older adults: current practices and future opportunities. Basel (Karger): Interdiscip Top Gerontol Geriatr; 2020. Vol. 43. p. 218–233.
- Poolman JT. Expanding the role of bacterial vaccines into life-course vaccination strategies and prevention of antimicrobial-resistant infections. NPJ Vaccines. 2020;5:84
- Doherty TM, Connolly MP, Del Giudice G, et al. Vaccination programs for older adults in an era of demographic change. Eur Geriatr Med. 2018;9(3):289–300.