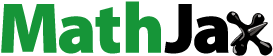
ABSTRACT
Background
Evaluating COVID-19 vaccine effectiveness (VE) domestically is crucial for assessing and determining national vaccination policy. This study aimed to evaluate VE of mRNA COVID-19 vaccines in Japan.
Methods
We conducted a multicenter test-negative case-control study. The study comprised individuals aged ≥16 visiting medical facilities with COVID-19-related signs or symptoms from 1 January to 26 June 2022, when Omicron BA.1 and BA.2 were dominant nationwide. We evaluated VE of primary and booster vaccination against symptomatic SARS-CoV-2 infections and relative VE of booster compared with primary.
Results
We enrolled 7,931 episodes, including 3,055 test positive. The median age was 39, 48.0% were male, and 20.5% had underlying medical conditions. In individuals aged 16 to 64, VE of primary vaccination within 90 days was 35.6% (95% CI, 19.0–48.8%). After booster, VE increased to 68.7% (60.6–75.1%). In individuals aged ≥65, VE of primary and booster was 31.2% (−44.0–67.1%) and 76.5% (46.7–89.7%), respectively. Relative VE of booster compared with primary vaccination was 52.9% (41.0–62.5%) in individuals aged 16 to 64 and 65.9% (35.7–81.9%) in individuals aged ≥65.
Conclusions
During BA.1 and BA.2 epidemic in Japan, mRNA COVID-19 primary vaccination provided modest protection. Booster vaccination was necessary to protect against symptomatic infections.
1. Introduction
In February 2021, the Japanese government approved and introduced coronavirus disease 2019 (COVID-19) vaccines [Citation1]. The effectiveness of messenger RNA (mRNA) COVID-19 vaccines was estimated to be high against symptomatic severe acute respiratory syndrome coronavirus 2 (SARS-CoV-2) infections in Japan during the Delta (B.1617.2) variant epidemic [Citation2]. However, the waning of immunity has been observed over time since the last vaccination in many countries [Citation3–5]. The Japanese government introduced a third (booster) dose in December 2021, following the primary series of two doses [Citation6].
The Omicron (B.1.1.529) variant was reported to exhibit marked transmissibility and immune evasion after two-dose-vaccination and infection [Citation7]. A reduced neutralizing antibody response to the Omicron variant in two-dose-vaccinated individuals compared with the previous variants was observed [Citation8]. Real-world data also showed that the effectiveness of two doses of mRNA COVID-19 vaccines against symptomatic SARS-CoV-2 infections due to the Omicron variant was estimated to decline compared with that of the Delta variant [Citation9,Citation10]. However, the first booster dose (third dose) was reported to strengthen protection against symptomatic SARS-CoV-2 infections of the Omicron variant [Citation9–12].
In Japan, the first infection with the Omicron variant was identified at the end of November 2021 [Citation13]. Initially, Omicron cases involved travelers from abroad or their close contacts. Thereafter, community transmission occurred, and infections of the Omicron variant increased rapidly from the beginning of January 2022, leading to the sixth epidemic wave (Supplementary Figure 1) [Citation14]. Finally, during the sixth wave, from January to June 2022, most of the circulating variants were SARS-CoV-2 Omicron BA.1 and BA.2 (Supplementary Figure 2) [Citation15].
Assessing the effectiveness of COVID-19 vaccines domestically is essential when evaluating the national vaccination policy and determining future optimal vaccination policy. Accordingly, from 1 July 2021, we started surveillance activity to monitor the vaccine effectiveness of COVID-19 vaccines against symptomatic SARS-CoV-2 infections in Japan, named Vaccine Effectiveness Real-time Surveillance for SARS-CoV-2 (VERSUS) [Citation2,Citation16]. In this study, we aimed to evaluate the vaccine effectiveness of two doses (primary vaccination) and the first booster vaccination (third dose) of mRNA COVID-19 vaccines (BNT162b2 and mRNA-1273) as well as the relative vaccine effectiveness of booster compared with the primary vaccination vaccines against symptomatic SARS-CoV-2 infections during the SARS-CoV-2 Omicron BA.1 and BA.2 epidemic in Japan.
2. Methods
2.1. Study design
We used the data from our surveillance to monitor the vaccine effectiveness of COVID-19 vaccines against symptomatic SARS-CoV-2 infections in Japan [Citation16]. This surveillance included episodes involving individuals aged ≥16 years visiting participating hospitals or clinics with one or more COVID-19-related signs or symptoms and tested for SARS-CoV-2. We defined the following signs or symptoms as COVID-19-related signs or symptoms: fever (≥37 · 5°C), cough, fatigue, shortness of breath, myalgia, sore throat, nasal congestion, headache, diarrhea, taste disorder, and olfactory dysfunction. Episodes involving individuals tested from 1 January to 26 June 2022, when most of circulation variants were SARS-CoV-2 Omicron BA.1 and BA.2 (Supplementary Figure 2) [Citation15], were included in this present study.
A test-negative case-control study was applied to estimate vaccine effectiveness. This study design has been widely applied when evaluating vaccine effectiveness of COVID-19 vaccines [Citation17]. A case was defined as an episode with an individual with signs or symptoms and a positive test result for SARS-CoV-2, and a control was defined as an episode with an individual with signs or symptoms but a negative result for SARS-CoV-2. All SARS-CoV-2 tests were conducted in participating hospitals or clinics as standard clinical practice. We included the following test methods, which are commonly employed for the diagnosis of SARS-CoV-2 infection in Japan [Citation18]: nucleic acid amplification test, including polymerase chain reaction (PCR), loop-medical isothermal amplification (LAMP) [Citation19,Citation20], nicking endonuclease amplification reaction (NEAR) [Citation21], and transcription-mediated amplification (TMA) [Citation22]; and antigen quantification test [Citation23,Citation24]. We excluded results generated by the rapid antigen test. Test methods used as clinical practice in each medical facility were employed, and SARS-CoV-2 genome sequencing was not conducted for test-positive specimens in this study.
2.2. COVID-19 vaccination strategy in Japan
The Japanese government started the national COVID-19 vaccination campaign in February 2021 with primary courses of two doses of BNT162b2, mRNA-1273, or AZD1222 (Supplementary Figure 3) (1). AZD1222 was optional for those aged ≥40 years. Booster vaccination with either BNT162b2 or mRNA-1273 was introduced in December 2021 for adults ≥18 years (Supplementary Figure 3) (6). NVX-CoV2373 was also approved and used from 27 May 2022, as a booster dose [Citation25]. By the end of June 2022, approximately 90 and 70% of the Japanese population aged ≥65 years and ≥12 years had received booster vaccination, respectively (Supplementary Figure 1) [Citation26]. Uses of AZD1222 and NVX-CoV2373 were limited to less than 0.1% of COVID-19 vaccines administered in Japan (Supplementary Table 3) [Citation26].
2.3. Data collection
Demographic and clinical information included age, sex, place of residence, underlying medical conditions (i.e. chronic heart disease, chronic respiratory disease, obesity [body mass index ≥30 kg/m2], malignancy [including solid or hematological malignancy], diabetes mellitus, chronic kidney disease, receiving dialysis, liver cirrhosis, use of immunosuppressive medicines, and pregnancy), smoking history, history of contact with COVID-19 patients within the last 14 days, healthcare employment status, past history of COVID-19, clinical symptoms, and COVID-19 vaccination history. Date of vaccination, types of vaccine product, and vaccination frequency were obtained from medical charts, vaccination cards, or interviews with the patients or family members.
2.4. Definition of vaccination status
We defined the vaccination status as follows, based on the number of vaccine doses received before the symptom onset date and number of days between the receipt of the last vaccination and symptom onset date: (1) no vaccination, individuals had received no vaccine dose before symptom onsets; (2) primary vaccination, individuals had received two doses ≥14 days before symptom onset; (3) booster vaccination, individuals had received the booster dose ≥14 days before symptom onset. For episodes with an unknown onset date, the vaccination status was defined based on number of days between the receipt of the last vaccination and test date. Completion of vaccination was defined as 14 days after the receipt of the last vaccination. When we evaluated vaccine effectiveness separated by the days after vaccination, the number of days is shown starting from the completion of vaccination.
2.5. Statistical analysis
Vaccine effectiveness was defined as one minus an adjusted odds ratio, expressed as a percentage. A mixed-effects logistic regression model was used to calculate the adjusted OR. Adjusted ORs were calculated by comparing the odds of antecedent COVID-19 vaccination in test-positive versus test-negative episodes. We applied age, sex, underlying medical conditions, calendar week of test, history of contact with COVID-19 patients within the last 14 days, and healthcare employment status as fixed effects, and medical facilities as a random effect. Since the possibility of confounding was present due to the difference in COVID-19 vaccination strategy by age group, we evaluated vaccine effectiveness separately in individuals aged 16 to 64 and those aged ≥65. For the analysis of individuals aged 16 to 64 years, vaccine effectiveness was assessed separately within 90 days, at 91 to 180 days, and more than 180 days after the completion of vaccination. For analysis of individuals aged ≥65 years, vaccine effectiveness was evaluated regardless of the days after vaccine receipt due to the small sample size.
We evaluated vaccine effectiveness of either BNT162b2 or mRNA-1273, and the effectiveness of BNT162b2 and mRNA-1273 was assessed separately as well. Bivalent mRNA COVID-19 vaccines had not been approved in Japan by the end of the study period, and so monovalent vaccines with the ancestral strain were solely evaluated in this study. We excluded episodes involving individuals receiving COVID-19 vaccines other than BNA162b2 and mRNA-1273. Episodes involving an unknown type of vaccine product were considered to be BNT162b2 or mRNA-1273 and included in BNT162b2 or mRNA-1273 analysis, referring to the COVID-19 vaccination situation in Japan (Supplementary Table 3) [Citation26]. We analyzed relative vaccine effectiveness of booster vaccination: for individuals aged 16 to 64 years, the comparator was primary vaccination at more than 180 days; for individuals aged ≥65 years, the comparator was primary vaccination regardless of days after the receipt of vaccines due to the small sample size. Only for individuals aged 16 to 64, we conducted subgroup analyses stratified by sex, presence of underlying medical conditions, or test period. To evaluate vaccine effectiveness during SARS-CoV-2 Omicron BA.1- or BA.2-dominant period separately, we assessed vaccine effectiveness in two separate periods, as follows: (1) between 1 January 2022, and 13 March 2022, when more than 78% of the circulating variant was BA.1; and (2) between 11 April 2022, and 26 June 2022, when more than 80% was BA.2.
Episodes tested 15 days or more after symptom onset were excluded because of the potential inaccuracy of test results [Citation27]. We applied criteria to exclude episodes involving individuals with multiple episodes: (1) episodes with multiple negative test results and identical symptom onset date; (2) episodes with negative test results within seven days after a previous negative result; (3) episodes with negative test results within three weeks before or after a positive test result; (4) for multiple positive episodes during the study period, only the first episode was included; and (5) we included a maximum of three negative test results for each individual.
This study included individuals whose precise vaccination date was not documented. In the primary analysis, we used the following criteria: for individuals whose precise vaccination date was not documented (for example, only the vaccination month was reported), the midpoint between the two possible dates was set as the vaccination date; and individuals for whom only the number of vaccine doses was recorded were included in the primary- or booster-vaccinated group, depending on the number of vaccine doses.
Since some episodes involved unclear vaccination dates or unknown vaccination history, we conducted sensitivity analyses of vaccine effectiveness and relative vaccine effectiveness analysis using four scenarios, as follows: (1) we set the vaccination date as the most recent possible date from symptom onset when the precise vaccination date was uncertain; (2) we set the vaccination date as the earliest possible date from symptom onset when the precise vaccination date was uncertain; (3) we excluded episodes involving an unknown vaccination status; and (4) we excluded episodes with no information on the vaccination date. Additionally, we employed two other scenarios for sensitivity analyses, as follows: (5) we included episodes restricted to respiratory symptoms, including fever, cough, shortness of breath, or sore throat; and (6) we excluded episodes involving individuals with previous COVID-19 histories (Supplementary Section 1). All analyses were performed using Stata version 16.0 (Stata Corp., College Station, Texas, USA)
2.6. Ethical consideration
This study was conducted following the principles of the Declaration of Helsinki, the Ethical Guidelines for Medical Research Involving Human Subjects of Japan, and the Act on the Protection of Personal Information of Japan. This study was approved by the Institutional Review Board (IRB) of the Institute of Tropical Medicine, Nagasaki University (approval no. : 210225257) and the participating hospital and clinics. A centralized IRB review process was applied to the medical facilities without IRBs, and the IRB of the Institute of Tropical Medicine approved the study at those medical facilities. Since this study did not involve intervention and did not use specimens collected from the human body, the requirement for obtaining consent from individual patients was waived by the IRBs with reference to the Ethical Guidelines for Medical Research Involving Human Subjects of Japan.
3. Results
3.1. Patient characteristics
Between 1 January 2022, and 26 June 2022, 8,283 episodes were registered in our surveillance. We excluded 143 episodes with tests ≥15 days after symptom onset, 191 with multiple test occasions, and 18 involving individuals receiving vaccines other than BNT162b2 or mRNA-1273. Subsequently, 7,931 episodes (3,055 test-positive, 4,876 test-negative) were included in the analysis () from 14 medical facilities in 11 prefectures on three islands of Japan (Supplementary Figure 4). Three medical facilities registered only episodes involving patients who visited medical facilities between January and March, and one medical facility between April and May. Overall, the median age was 39 years (interquartile range, 27–53); 3,810 (48.0%) were male; 1,628 (20.5%) had one or more underlying medical conditions; and 142 (1.8%) had a past history of COVID-19 (). Among the study population, 13.8% were not vaccinated, 60.1% were primary-vaccinated, and 20.1% were booster-vaccinated. Among individuals aged ≥65 years, 5.8% were not vaccinated, 49.7% were primary-vaccinated, and 34.8% were booster-vaccinated. The percentages of test-positive cases were 52.7, 42.2, and 20.3% in the non-, primary-, and booster- vaccinated individuals, respectively.
Figure 1. This study included individuals aged ≥16 years visiting participating hospitals or clinics with one or more of the following signs or symptoms: fever (≥37.5°C), cough, fatigue, shortness of breath, myalgia, sore throat, nasal congestion, headache, diarrhea, taste disorder, or olfactory dysfunction, and tested for SARS-CoV-2 in Japan between 1 January 2022, and 26 June 2022. Abbreviations: SARS-CoV-2, severe acute respiratory syndrome coronavirus 2; COVID-19, coronavirus disease 2019.

Table 1. Demographics and characteristics of test-positive cases and test-negative controls: VERSUS study, Japan, 1 January to 26 June 2022.
3.2. Vaccine effectiveness of mRNA COVID-19 vaccines
shows vaccine effectiveness of primary and booster vaccinations of mRNA COVID-19 vaccines against symptomatic SARS-CoV-2 infections during the study period. For BNT162b2 or mRNA-1273 analysis among individuals aged 16 to 64 years, vaccine effectiveness of primary vaccination was 35.6% (95% confidence interval [CI], 19.0–48.8%) within 90 days, 32.3% (95% CI, 20.7–42.2%) at 91 to 180 days, and 33.6% (95% CI, 18.5–45.8%) at more than 180 days after the completion of primary vaccination, respectively. After booster vaccination, vaccine effectiveness increased to 68.7% (95% CI, 60.6–75.1%) within 90 days. At 91 to 180 days, the point estimate of vaccine effectiveness of booster vaccination declined to 59.1% (95% CI, 37.5–73.3%). Point estimates were higher for mRNA-1273 than for BNT162b2 for both primary and booster vaccination; however, there was no significant difference. For BNT162b2 or mRNA-1273 analysis among individuals aged ≥65 years, vaccine effectiveness of primary vaccination was 31.2% (95% CI, −44.0–67.1%). After booster vaccination, vaccine effectiveness increased to 76.5% (95% CI, 46.7–89.7%).
Figure 2. Vaccine effectiveness of messenger RNA COVID-19 vaccines against symptomatic SARS-CoV-2 infections among individuals aged 16 to 64 years and aged ≥65 years, VERSUS study, Japan, 1 January–26 June 2022. The analysis included test-positive cases with signs or symptoms that tested positive for SARS-CoV-2, and test-negative controls with signs or symptoms that tested negative for SARS-CoV-2. Vaccine effectiveness was adjusted for age, sex, underlying medical conditions, calendar week of test, history of contact with COVID-19 patients within 14 days, healthcare professional status, and medical facilities. The vaccination status was classified into three statuses based on the number of vaccine doses received before symptom onset and the number of days between the last vaccination date and symptom onset: no vaccination, individuals had received no vaccine dose before symptom onset; primary vaccination, individuals had received the second dose ≥14 days before symptom onset; and booster vaccination, individuals had received the third dose ≥14 days before symptom onset. Completion of vaccination was defined as 14 days after receiving the last vaccine. When evaluating vaccine effectiveness separated by days after vaccination, the number of days is shown from completion of vaccination. Abbreviation: VE, vaccine effectiveness.

shows the relative vaccine effectiveness of booster vaccination compared with primary vaccination against symptomatic SAR-CoV-2 infections. Among individuals aged 16 to 64 years, compared with primary vaccination at more than 180 days, relative vaccine effectiveness of booster vaccination was 52.9% (95% CI, 41.0–62.5%) and 38.5% (95% CI, 6.9–59.3%) within 90 days and at 91 to 180 days, respectively; for individuals aged ≥65 years, relative vaccine effectiveness of booster vaccination was 65.9% (95% CI, 35.7–81.9%) compared with primary vaccination.
Figure 3. Relative vaccine effectiveness of messenger RNA COVID-19 booster vaccination, compared with the primary vaccination against symptomatic SARS-CoV-2 infections among individuals aged 16 to 64 years and aged ≥65 years, VERSUS study, Japan, 1 January–26 June 2022. The analysis included test-positive cases with signs or symptoms and tested positive for SARS-CoV-2 and test-negative controls with signs or symptoms and tested negative for SARS-CoV-2. Vaccine effectiveness was adjusted for age, sex, underlying medical conditions, calendar week of test, history of contact with COVID-19 patients within the last 14 days, healthcare professional status, and medical facilities. The vaccination status was classified into two statuses based on the number of vaccine doses received before symptom onset and number of days between the last vaccination date and symptom onset: primary vaccination, individuals had received the second dose ≥14 days before symptom onset; and booster vaccination, individuals had received the third dose ≥14 days before symptom onset. Completion of vaccination was defined as 14 days after receiving the last vaccine. When evaluating vaccine effectiveness separated by days after vaccination, the number of days is shown from completion of vaccination. Abbreviation: VE, vaccine effectiveness.

In subgroup analyses, there was no difference by sex in vaccine effectiveness of either primary or booster vaccination (). On comparing BA.1- and BA.2-dominant periods, vaccine effectiveness of booster vaccination were similar, with overlapping confidence intervals.
Figure 4. Vaccine effectiveness of messenger RNA COVID-19 vaccines against symptomatic SARS-CoV-2 infections stratified by subgroups among individuals aged 16 to 64 years, VERSUS study, Japan, 1 January–26 June 2022. The analysis included test-positive cases with signs or symptoms and tested positive for SARS-CoV-2 and test-negative controls with signs or symptoms and tested negative for SARS-CoV-2. Vaccine effectiveness was adjusted for age, sex, underlying medical conditions, calendar week of test, history of contact with COVID-19 patients within the last 14 days, and medical facilities. The vaccination status was classified into three statuses based on the number of vaccine doses received before symptom onset and number of days between the last vaccination date and symptom onset: no vaccination, individuals had received no vaccine dose before symptom onset; primary vaccination, individuals had received one dose ≥14 days before symptom onset; and booster vaccination, individuals had received two doses ≥14 days before symptom onset. Completion of vaccination was defined as 14 days after receiving the last vaccine. When evaluating vaccine effectiveness separated by days after vaccination, the number of days is shown from completion of vaccination. Underlying medical conditions included chronic heart disease, chronic respiratory disease, obesity (body mass index ≥30 kg/m2), malignancy (including solid or hematological malignancy), diabetes, chronic kidney disease, receiving dialysis, liver cirrhosis, use of immunosuppressive medicines, and pregnancy. We assessed VE in two separate periods, as follows: (1) BA.1-dominant period defined as between 1 January 2022, and 13 March 2022, when more than 78% of the circulating variant was BA.1; and (2) BA.2-dominant period defined as between 11 April 2022, and 26 June 2022, when more than 80% was BA.2. Abbreviation: VE, vaccine effectiveness.

The sensitivity analysis results are shown in Supplementary Tables 1 and 2. The results are similar to those of primary analysis (Supplementary Section 1).
4. Discussion
Here, we evaluated primary and booster vaccine effectiveness of mRNA COVID-19 vaccines against symptomatic SARS-CoV-2 infections in Japan between 1 January 2022, and 26 June 2022, when the SARS-CoV-2 Omicron BA.1 and BA.2 were dominant (Supplementary Figure 2) [Citation15]. For individuals aged 16 to 64 years, vaccine effectiveness of primary vaccination provided only modest protection of 35.6% (95% CI, 19.0–48.8%) even within 90 days. After booster vaccination, vaccine effectiveness increased to 68.7% (95% CI, 60.6–75.1%) within 90 days and then declined to 59.1% (95% CI, 37.5–73.3%), although confidence intervals were wide around the point estimates. Our findings support the effectiveness of booster vaccination to protect against symptomatic SARS-CoV-2 infections during the SARS-CoV-2 Omicron BA.1 and BA.2 epidemic in Japan. The Japanese government decided to introduce booster (third dose) vaccination before SARS-CoV-2 Omicron BA.1 and BA.2 started spreading nationwide. This study suggested that the implementation of booster vaccination was appropriate, and justified the introduction of booster vaccination as a national COVID-19 vaccination policy, including for younger populations, to prevent symptomatic SARS-CoV-2 infections. In the future, an additional booster will be required to protect against symptomatic infections if a new SARS-CoV-2 variant showing marked immune evasion emerges.
During the Delta variant epidemic, vaccine effectiveness of primary vaccination was high against symptomatic SARS-CoV-2 infections in Japan: 91.8% (95% CI, 80.3–96.6%) within 90 days, 86.4% (95% CI, 56.9–95.7%) at 91 to 180 days in individuals aged 16 to 64 years, and 90.3% (95% CI, 73.6–96.4%) in individuals aged ≥65 years (Supplementary Figure 5) [Citation2]. During the Omicron variant epidemic, however, primary vaccination provided only modest protection, being lower compared with vaccine effectiveness of primary vaccination during the Delta variant epidemic (Supplementary Figure 5) [Citation2]. These findings are consistent with previous studies from other countries [Citation9–12,Citation28,Citation29]. Unlike during the Delta variant epidemic, primary vaccination was inadequate to protect against symptomatic SARS-CoV-2 infections, and booster vaccination was needed.
Our estimates of booster vaccination within 90 days were similar to those reported from the UK (45.7–67.2% for BNT162b; 66.3% for mRNA-1273) [Citation9], US (64% for BNT162b2; 72.1% for mRNA-1273) [Citation10,Citation29], and Canada (60% for BNT162b2; 65% for mRNA-1273) [Citation28]. Also, we detected early signs of the waning effectiveness of booster vaccination against symptomatic SARS-CoV-2 infections, which were less marked than those seen in the UK and US [Citation9,Citation29]. On the other hand, our estimates of primary vaccination within 90 days were lower or similar compared with those in the UK (48.7–65.5% for BNT162b2; 52.8–75.1% for mRNA1273) [Citation9], US (64% for BNT162b2; 44.0% for mRNA127) [Citation10,Citation29], and Canada (36% for either BNT162b2 or mRNA-1273) [Citation28]. One of the possible reasons for the differences in vaccine effectiveness was the differential percentage of individuals with infection-induced SARS-CoV-2 antibodies in the population. Less than 5% of individuals had infection-induced antibodies in Japan [Citation30], being lower than in the UK [Citation31] and US [Citation32]. Infection-induced protection is also effective against SARS-CoV-2 infections [Citation33,Citation34]. There is a possibility that protection induced by both infection and vaccination was evaluated solely as vaccine-induced protection in the UK and US. Similarly, many unvaccinated individuals, who are used as a comparator group when estimating vaccine effectiveness, could have infection-induced antibodies in the UK and US, and this fact could also affect vaccine effectiveness. The percentage of individuals with infection-induced antibodies, vaccinated individuals, and individuals with protection induced by both infection and vaccination in the general population varies among countries, and this difference can affect vaccine effectiveness assessed in those countries. Therefore, it is essential to evaluate domestic vaccine effectiveness.
Comparing with studies in the UK [Citation9] or US [Citation10,Citation29], vaccine effectiveness of primary vaccination did not wane over time in this study. Another study from Japan showed a similar trend: vaccine effectiveness was maintained at a similar level, even at six months after vaccination, and did not decline over time [Citation35]. One possible reason for the regional difference could be the difference in the percentage of individuals with infection-induced immunity between Japan and other countries. More people without vaccination in the UK or US achieved infection-induced immunity during the SARS-CoV-2 Omicron wave. Therefore, when evaluating vaccine effectiveness at six months or longer after the last receipt of vaccination, many non-vaccinated individuals already had infection-induced immunity, which would have had a negative impact on vaccine effectiveness, as it was calculated by comparison of vaccinated and non-vaccinated populations. On the other hand, in Japan, the number of infections was lower than that in other countries, and the percentage of individuals with infection-induced immunity among non-vaccinated individuals could have been low even after the spread of Omicron BA.1 and BA.2. Therefore, no decline in vaccine effectiveness over time was seen.
In our vaccine effectiveness analysis, the comparator group was an unvaccinated population, which comprised 14% of the total study population. This unvaccinated population could potentially have differed from the vaccinated population by showing characteristics that could be unknown confounders. Therefore, we also assessed relative vaccine effectiveness against symptomatic SARS-CoV-2 infections. Our estimates were consistent with previous studies [Citation11,Citation36,Citation37].
There is limited knowledge about the difference between vaccine effectiveness against BA.1 and that against BA.2. Neutralizing antibody titers induced by vaccines were almost the same against BA.1 and BA.2 [Citation38]. Additionally, real-world data also showed that vaccine effectiveness of mRNA COVID-19 vaccines against BA.1 and BA.2 was almost the same [Citation39,Citation40], while vaccine effectiveness against BA.2.12.1 was estimated to be lower than that against BA.1 or BA.2 [Citation41]. In the present study, we assessed vaccine effectiveness stratified by the study period to evaluate the difference for each variant. The results were almost the same in the BA.1 and BA.2 epidemic period.
The sample size of individuals aged ≥65 years was limited in this study. One of the possible reasons was the way the study population was collected. The characteristics of the study population were dictated by the patients who visited medical facilities included in this study, even though the facilities were community-based hospitals or clinics, and the same inclusion criteria were applied in each of them. Another possible reason was that the number of SARS-CoV-2 tests for individuals aged ≥65 years with signs or symptoms could have been originally lower compared with that for individuals aged 16 to 64 years nationwide. From the national data, the number of COVID-19 patients aged 20 to 59 years was about five times higher than that of those aged ≥60 years during the study period [Citation14]. Therefore, it is possible that the number of SARS-CoV-2 tests for those aged ≥65 years was originally lower compared with that for those aged 16 to 64 years, and the study population simply reflected this, and not a collection-based problem.
This study had several limitations. First, since our study was observational, there could be another confounder that has yet to be identified. Second, recall bias may be present regarding vaccination histories. In Japan, medical professionals and researchers are generally not allowed to access governmental vaccination records. Additionally, medical services and vaccination are covered by different systems, and no system integrates both records at present. Therefore, studies conducted in clinical fields must partially rely on vaccination histories disclosed by patients or their family members. Some vaccination histories included in this study were obtained through interviews with patients or family members. To strengthen our results, we conducted several sensitivity analyses (Supplementary Section 2, Supplementary Tables 1 and 2) and the results were similar to those of the primary analysis, meaning that our results were robust. Third, since SARS-CoV-2 genome sequencing was not conducted for test-positive patients, it was impossible to obtain an accurate vaccine effectiveness of mRNA COVID-19 vaccines against BA.1 and BA.2. Even though most of the circulating SARS-CoV-2 variants were Omicron BA.1 and BA.2, a possibility exists that some of the episodes were caused by other SARS-CoV-2 variants. Fourth, the possibility of misclassification of outcomes cannot be ruled out. Since SARS-CoV-2 testing methods do not show 100% sensitivity or specificity, there could be false-positive and false-negative test results, possibly leading to the misclassification of outcomes. Fifth, there is a possibility that some patients may have had protection induced by infections without realizing it because SARS-CoV-2 infections caused by the Omicron variant tended to be asymptomatic or cause very mild disease. However, as mentioned above, the percentage of individuals with infection-induced antibodies was less than 5% in Japan [Citation30], being lower than those in the UK [Citation31] and US [Citation32]. Therefore, our analysis identified vaccine effectiveness among a mostly COVID-19-naïve population. Sixth, the sample size of individuals aged ≥65 years was limited as we have already discussed. Seventh, this study did not assess vaccine effectiveness against Omicron BA.5, which has been dominant worldwide, including Japan. Also, this study did not evaluate vaccine effectiveness against hospitalization or severe diseases. Thus, further studies are warranted.
5. Conclusions
During the SARS-CoV-2 Omicron BA.1 and BA.2 epidemic, primary vaccination with mRNA COVID-19 vaccines provided only modest protection against symptomatic SARS-CoV-2 infections. After booster vaccination, vaccine effectiveness increased to more than 65% effectiveness against symptomatic SARS-CoV-2 infection. Our findings support that booster vaccination was effective to protect against symptomatic SARS-CoV-2 infections during the SARS-CoV-2 Omicron variant BA.1 and BA.2 epidemic in Japan.
Declaration of interest
H Maeda reports lecture and consulting fees from Moderna Inc and participating in Pfizer Inc-funded research outside this work. A Igarashi reports grants from Takeda Pharmaceutical Inc, consulting fees from Pfizer Inc and Astra Zeneca Inc, and lecture fees from Takeda Pharmaceutical Inc, Shionogi Inc, Janssen Pharma Inc, and Modera Inc outside this work. M Terada reports lecture fees from Astra Zeneca outside this work. T Ito reports stock ownership of Takeda Pharmaceutical Inc. H Imura reports participating in research funded by Pfizer Inc, research funded by GENOVA Inc, research funded by Astra Zeneca Inc, and research funded by FUJIFILM Toyama Chemical CO., Ltd. outside this work. T Hayakawa reports stock ownership of Takeda Pharmaceutical Inc. and Daiichi Sankyo Healthcare Co., Ltd. K Morimoto reports grants from Pfizer Inc, consulting fees from Moderna Inc, and lecture fees from MSD outside this work. The authors have no other relevant affiliations or financial involvement with any organization or entity with a financial interest in or financial conflict with the subject matter or material discussed in the manuscript apart from those disclosed.
Author contributions
HM, NS, AI, MI, MT, MS, and KM conceived and designed the study. MI, MT, TI, HI, HK, IM, YK, MK, HA, OK, NA, ES, TM, HI, HI, TH, OT, and YO collected and verified the data. HM and NS did the analysis and MS supervised the analysis. AI performed the analysis separately from HM and NS. HM, NS, AI, MI, MT, MS, and KM had full access to the data. HM and KM wrote the first draft of the manuscript, and all authors provided critical review and revision of the text and approved the final version for publication.
Reviewer disclosures
Peer reviewers on this manuscript have received an honorarium for their review work. Peer reviewers on this manuscript have no other relevant financial or other relationships to disclose.
Supplemental Material
Download MS Word (680.2 KB)Acknowledgments
We are grateful to medical staff of the participating hospitals and clinics. We thank Rina Kubo, Yumi Araki, Kyoko Uchibori, Rina Shiramizu, Fumiyo Tsujita (Institute of Tropical Medicine, Nagasaki University), and Daichi Hatahara (Nagasaki University) for their assistance
Supplemental data
Supplemental data for this article can be accessed online at https://doi.org/10.1080/14760584.2023.2188950.
Additional information
Funding
References
- National Institute of Infectious Diseases. COVID-19 vaccine (as of May 10, 2021). Availabel at: https://www.niid.go.jp/niid/images/vaccine/covid19_vaccine_20210510.pdf. Accessed 2022 Dec 4
- Maeda H, Saito N, Igarashi A, et al. Effectiveness of mRNA COVID-19 vaccines against symptomatic SARS-CoV-2 infections during the delta variant epidemic in Japan: vaccine effectiveness real-time surveillance for SARS-CoV-2 (VERSUS). Clin Infect Dis. 2022;75:1971–1979.
- Tartof SY, Slezak JM, Fischer H, et al. Effectiveness of mRNA BNT162b2 COVID-19 vaccine up to 6 months in a large integrated health system in the USA: a retrospective cohort study. Lancet. 2021;398:1407–1416.
- Andrews N, Tessier E, Stowe J, et al. Duration of protection against mild and severe disease by covid-19 vaccines. N Engl J Med. 2022;386:340–350.
- Goldberg Y, Mandel M, Bar-On YM, et al. Waning Immunity after the BNT162b2 Vaccine in Israel. N Engl J Med. 2021;385:e85.
- National Institute of Infectious Diseases. COVID-19 vaccine (as of December 16, 2021). Availabel at: https://www.niid.go.jp/niid/images/vaccine/covid19_vaccine_20211216.pdf. Accessed 2022 Dec 4
- Viana R, Moyo S, Amoako DG, et al. Rapid epidemic expansion of the SARS-CoV-2 Omicron variant in Southern Africa. Nature. 2022;603:679–686.
- Dejnirattisai W, Shaw RH, Supasa P, et al. Reduced neutralisation of SARS-CoV-2 omicron B.1.1.529 variant by post-immunisation serum. Lancet. 2022;399:234–236.
- Andrews N, Stowe J, Kirsebom F, et al. Covid-19 Vaccine Effectiveness against the Omicron (B.1.1.529) Variant. N Engl J Med. 2022;386:1532–1546.
- Tseng HF, Ackerson BK, Luo Y, et al. Effectiveness of mRNA-1273 against SARS-CoV-2 Omicron and Delta variants. Nat Med. 2022;28:1063–1071.
- Abu-Raddad LJ, Chemaitelly H, Ayoub HH, et al. Effect of mRNA Vaccine Boosters against SARS-CoV-2 Omicron Infection in Qatar. N Engl J Med. 2022;386:1804–1816.
- Accorsi EK, Britton A, Fleming-Dutra KE, et al. Association between 3 doses of mRNA COVID-19 vaccine and symptomatic infection caused by the SARS-CoV-2 Omicron and delta variants. JAMA. 2022;327:639–651.
- Ministry of Health, Lbour and Welfare. Asymptomatic carriers of SARS-COV-2 in airport quarantine, November 30, 2021 2021. Availabel at: https://www.mhlw.go.jp/stf/newpage_22507.html. Accessed 2022 Dec 4
- Ministry of Health, Lbour and Welfare. Visualizing the data: information on COVID-19 infections. Available at: https://covid19.mhlw.go.jp/en/. Accessed 2022 Dec 4
- Ministry of Health, Lbour and Welfare. Materials of advisory board on countermeasures to combat novel coronavirus infections 2022 Availabel at: https://www.mhlw.go.jp/stf/seisakunitsuite/bunya/0000121431_00333.html. Accessed 2022 Dec 4
- VERSUS Group. Vaccine effectiveness real-time surveillance for SARS-CoV-2 Availabel at: http://www.tm.nagasaki-u.ac.jp/versus/about.html. Accessed 2022 Dec 4
- World Health Organization. Evaluation of COVID-19 vaccine effectiveness. Available at: https://www.who.int/publications/i/item/WHO-2019-nCoV-vaccine_effectiveness-measurement-2021.1. Accessed 2023 Feb 24
- Ministry of Health, Lbour and Welfare. Approval information for diagnostic tests for SARS-CoV-2 Availabel at: https://www.mhlw.go.jp/stf/newpage_11331.html#Nucleic. Accessed 2022 Dec 4
- Lai MY, Bukhari FDM, Zulkefli NZ, et al. Clinical testing on SARS-CoV-2 swab samples using reverse-transcription loop-mediated isothermal amplification (RT-LAMP). BMC Infect Dis. 2022;22:697.
- Subali AD, Wiyono L. Reverse transcriptase loop mediated isothermal amplification (RT-LAMP) for COVID-19 diagnosis: a systematic review and meta-analysis. Pathog Glob Health. 2021;115:281–291.
- Ulhaq ZS, Soraya GV. The diagnostic accuracy of seven commercial molecular in vitro SARS-CoV-2 detection tests: a rapid meta-analysis. Expert Rev Mol Diagn. 2021;21:733–740.
- Schneider M, Iftner T, Ganzenmueller T. Evaluation of the analytical performance and specificity of a SARS-CoV-2 transcription-mediated amplification assay. J Virol Methods. 2021;294:114182.
- Hirotsu Y, Maejima M, Shibusawa M, et al. Direct comparison of xpert xpress, filmarray respiratory panel, lumipulse antigen test, and RT-qPCR in 165 nasopharyngeal swabs. BMC Infect Dis. 2022;22:221.
- Hirotsu Y, Sugiura H, Maejima M, et al. Comparison of roche and lumipulse quantitative SARS-CoV-2 antigen test performance using automated systems for the diagnosis of COVID-19. Int J Infect Dis. 2021;108:263–269.
- National Institute of Infectious Diseases. List of vaccines available in Japan. Availabel at: https://www.niid.go.jp/niid/images/vaccine/corona_vaccine/covid19vac_list_20221013.pdf. Accessed 2022 Dec 4
- Prime Minister of Japan and His Cabinet. COVID-19 Vaccines. Availabel at: https://www.kantei.go.jp/jp/headline/kansensho/vaccine.html. Accessed 2022 Dec 4
- Lauer SA, Grantz KH, Bi Q, et al. The incubation period of coronavirus disease 2019 (COVID-19) from publicly reported confirmed cases: estimation and application. Ann Intern Med. 2020;172:577–582.
- Buchan SA, Chung H, Brown KA, et al. Estimated effectiveness of COVID-19 vaccines against omicron or delta symptomatic infection and severe outcomes. JAMA Network Open. 2022;5:e2232760.
- Tartof SY, Slezak JM, Puzniak L, et al. Durability of BNT162b2 vaccine against hospital and emergency department admissions due to the omicron and delta variants in a large health system in the USA: a test-negative case-control study. Lancet Respir Med. 2022;10:689–699.
- National Institute of Infectious Diseases. Report on seroepidemiological surveillance for SARS-CoV-2 infections in 2021 in Japan. Availabel at: https://www.niid.go.jp/niid/images/epi/corona/79/covid19-79.pdf. Accessed 2022 Dec 4
- UK Health Security Agency. COVID-19 vaccine surveillance report Week 17 Available at: https://assets.publishing.service.gov.uk/government/uploads/system/uploads/attachment_data/file/1072064/Vaccine-surveillance-report-week-17.pdf. Accessed 2022 Dec 4
- Clarke KEN, Jones JM, Deng Y, et al. Seroprevalence of infection-induced SARS-CoV-2 antibodies - United States, September 2021-February 2022. MMWR Morb Mortal Wkly Rep. 2022;71:606–608.
- Altarawneh HN, Chemaitelly H, Ayoub HH, et al. Effects of Previous Infection and Vaccination on Symptomatic Omicron Infections. N Engl J Med. 2022;387:21–34.
- Chin ET, Leidner D, Lamson L, et al. Protection against Omicron from vaccination and previous infection in a prison system. N Engl J Med. 2022;387:1770–1782.
- National Institute of Infectious Diseases. Test-negative case-control study to evaluate COVID-19 vaccines. Available at: https://www.niid.go.jp/niid/ja/2019-ncov/2484-idsc/10966-covid19-71.html. Accessed 2023 Feb 24
- Monge S, Rojas-Benedicto A, Olmedo C, et al. Effectiveness of mRNA vaccine boosters against infection with the SARS-CoV-2 omicron (B.1.1.529) variant in Spain: a nationwide cohort study. Lancet Infect Dis. 2022;22:1313–1320.
- Sheikh A, Kerr S, Woolhouse M, et al. Severity of omicron variant of concern and effectiveness of vaccine boosters against symptomatic disease in Scotland (EAVE II): a national cohort study with nested test-negative design. Lancet Infect Dis. 2022;22:959–966.
- Yu J, Collier AY, Rowe M, et al. Neutralization of the SARS-CoV-2 Omicron BA.1 and BA.2 Variants. N Engl J Med. 2022;386:1579–1580.
- Chemaitelly H, Ayoub HH, AlMukdad S, et al. Duration of mRNA vaccine protection against SARS-CoV-2 Omicron BA.1 and BA.2 subvariants in Qatar. Nat Commun. 2022;13:3082.
- Kirsebom FCM, Andrews N, Stowe J, et al. COVID-19 vaccine effectiveness against the omicron (BA.2) variant in England. Lancet Infect Dis. 2022;22:931–933.
- Link-Gelles R, Levy ME, Gaglani M, et al. Effectiveness of 2, 3, and 4 COVID-19 mRNA Vaccine Doses Among immunocompetent adults during periods when SARS-CoV-2 Omicron BA.1 and BA.2/BA.2.12.1 sublineages predominated - VISION network, 10 States, December 2021-June 2022. MMWR Morb Mortal Wkly Rep. 2022;71: 931-9.