ABSTRACT
Background
Wilson’s disease (WD) is a rare hepatic and neurological disorder, which can dramatically worsen by traumatic injuries, surgeries, and infections. No studies have reported safety data of severe acute respiratory syndrome coronavirus 2 (SARS-CoV-2) vaccination in WD patients. We aimed to investigate the SARS-CoV-2 vaccination status and post-vaccination adverse events in WD patients.
Methods
This is a multicenter, retrospective, observational study. We investigated the vaccination rates, the type of vaccine, subjective reasons for non-vaccination, and the adverse events following vaccination. Logistic regression analysis was used to assess the correlation between vaccination status and increased Unified Wilson’s Disease Rating Scale (UWDRS) scores.
Results
A total of 554 WD patients with a mean (SD) age of 25.3 (10.85) years were included in this study, of whom 336 (60.6%) were males and 218 (39.4%) were females. 368 (66.4%) patients received at least one dose of the SARS-CoV-2 vaccine.186 (33.6%) patients were unvaccinated. Logistic regression analysis showed that vaccination against SARS-CoV-2 was not significantly associated with increased UWDRS scores. The safety analysis demonstrated that 21.2% had post-vaccination adverse events.
Conclusions
In this study, vaccination against SARS-CoV-2 was safe in WD patients, providing evidence for the safety of vaccination in WD patients.
1. Introduction
In 2019, Coronavirus disease 2019 (COVID-19) started as an epidemic and spread around the world [Citation1]. As of 30 August 2022, more than 601 million global confirmed cases have occurred [Citation2]. Severe acute respiratory syndrome coronavirus 2 (SARS-CoV-2) was identified as the causative agent of COVID-19 [Citation3]. In addition to respiratory injuries, SARS-CoV-2 infection may also present with myocarditis, neurological diseases, liver damage, and skin changes [Citation4–6]. The most common neurological complaints in COVID-19 are headaches, malaise, myalgia, anosmia, and ageusia, followed by stroke, seizure, and encephalopathy [Citation7,Citation8]. Published case reports suggest that liver dysfunction may occur in patients with COVID-19, with an incidence ranging from 14.8% to 78% [Citation9]. Since December 2022, China has relaxed the control of COVID-19, and vaccination is the most scientific, economical, and effective means of epidemic prevention and control. Therefore, it is necessary to strengthen research on the SARS-CoV-2 vaccine.
Wilson’s disease (WD) is an autosomal recessive disorder caused by mutations in the ATP7b gene, with a worldwide clinical prevalence of 3.3/100,000 and a genetic prevalence of 14.3/100,000 [Citation10]. The clinical prevalence in China is 5.87/100,000 [Citation11]. There are approximately 40,000–80,000 patients with WD in China. Every year, patients from all over the country come to Anhui for treatment, so we have the opportunity to collect enough samples in a short period of time.
The disease is characterized by hepatic and neurological deficits as the main clinical manifestations [Citation12,Citation13]. As COVID-19 can also present with hepatic and neurological damage [Citation7–9], it is necessary for patients with WD to pay attention to the prevention of SARS-CoV-2 infection to avoid the risk of possible exacerbation after infection.
Administering COVID-19 vaccines provided limited protection against SARS-CoV-2 infection across all age groups but high protection against severe/critical illness and COVID-19-related death [Citation14]. Several SARS-CoV-2 vaccines have been evaluated for their efficacy and safety in phase III clinical trials [Citation15]. However, while effective in preventing infection with the original strain and variants of SARS-CoV-2, there is a risk of adverse events associated with vaccination [Citation16–20]. Previous studies have shown that liver disease (portal vein thrombosis, autoimmune hepatitis, liver injury), kidney injury (IgA glomerulonephritis, vasculitis), and immune thrombocytopenia may occur after vaccination [Citation21–26]. SARS-CoV-2 vaccination campaigns in most countries worldwide, including China, follow the principle of mass voluntarism: vaccination is encouraged and not mandated. People with neurological disorders such as epilepsy, transverse myelitis, Guillain-Barre syndrome (GBS), demyelinating disease, and those at high risk of severe illness or death after SARS-CoV-2 infection, such as those with acute exacerbations of chronic diseases, uncontrolled severe chronic diseases, and impaired immune function, have been advised to contraindicate use the SARS-CoV-2 vaccine [Citation27]. Most patients with WD have an insidious onset and slow exacerbation, while some patients have a dramatic exacerbation due to traumatic injuries, surgeries, and severe infections [Citation28,Citation29]. No studies have been reported on whether SARS-CoV-2 vaccination affects disease progression in patients with WD. Patients are concerned about the safety of vaccination and its potential for adverse events. Therefore, it is necessary to evaluate vaccination coverage and safety in patients with WD. To provide a clinical basis and recommendations for SARS-CoV-2 vaccination decisions for WD patients, we observed vaccination status and post-vaccination adverse events in WD patients, analyzed subjective reasons for non-vaccinated patients, and assessed the correlation between vaccination status and increased Unified Wilson’s Disease Rating Scale (UWDRS) scores.
2. Methods
2.1. Study population
The cohort of this study was obtained from patients with WD who were hospitalized in the departments of neurology of three hospitals (The First Affiliated Hospital of Anhui University of Traditional Chinese Medicine, Institute of Neurology of Anhui University of Traditional Chinese Medicine, and Anhui Integrated Traditional Chinese and Western Medicine Hospital). These patients were admitted to the hospital with WD between 2020–2022.
2.2. Inclusion criteria
(1) Patients who met the diagnostic criteria for WD and were receiving routine anti-copper therapy; (2) patients who could cooperate with the UWDRS survey; (3) patients who were willing to participate in the survey and sign an informed consent form; (4) WD patients diagnosed before 29 March 2021.
2.3. Exclusion criteria
(1) Patients with severe dysarthria, cognitive dysfunction, or mental disorders who could not cooperate; (2) pregnant and lactating patients; (3) refusal to participate for various reasons; (4) patients with fever, cough, sore throat, diarrhea, and dyspnea within 14 days before vaccination.
2.4. Research data collection
Between 1 April 2022, and 20 August 2022, clinical data of patients with WD were collected through the Hospital Information System (HIS), including patient characteristics (age, sex, marital status, region, etc.), past medical history, abdominal ultrasound, and bone examination. In addition, we collected the patients’ vaccination status and UWDRS scores using a questionnaire and telephone follow-up. The questionnaire was designed as follows: (1) vaccination status: whether to receive the SARS-CoV-2 vaccine, number of vaccination shots, vaccine type, and time of the first vaccination; (2) subjective reasons for not receiving the vaccine; (3) whether adverse events occurred after the participants received the vaccine. The study was monitored by the Information Center of the First Affiliated Hospital of Anhui University of Traditional Chinese Medicine to improve the accuracy of the data.
2.5. SARS-CoV-2 vaccination
On 29 March 2021, China released the technical guidelines for vaccination against SARS-CoV-2 (first edition). SARS-CoV-2 vaccines approved for conditional marketing or emergency use mainly include inactivated vaccines, Adenovirus-vectored vaccines, and recombinant subunit vaccines [Citation27]. China advocates the basic principles of “informed, consent, and voluntariness” for the public.
2.6. Clinical evaluation of the UWDRS
WD is an autosomal recessive disorder of copper metabolism with liver, neurological, and psychiatric symptoms as the main clinical manifestations. The UWDRS can comprehensively assess patients’ signs and symptoms in the liver, nerves, and psychiatric systems. The full UWDRS (maximum score of 320 points) consists of three subscales representing three main features of clinical manifestation in WD: a hepatic subscale (9 items, 36 points), a neurological subscale (27 items, 208 points), and a psychiatric subscale (19 items, 76 points) [Citation30]. The date of the first vaccination was used as the time point for patients in the vaccinated group, and 29 March 2021, when the mass vaccination program began in China, was used as the time point for the unvaccinated group. The UWDRS scores of patients before and after the time point were recorded according to their medical history and current condition. Two separate UWDRS scores were performed by two neurologists, completed on the first and second days of patient inclusion to ensure consistency of scoring. The mean values of the two scores were calculated and expressed as the mean (SD).
2.7. Adverse events after SARS-CoV-2 vaccination
Patients with WD were evaluated for adverse events following SARS-CoV-2 vaccination, including exacerbation of extrapyramidal damage symptoms and vaccine-related adverse reactions. Symptoms of extrapyramidal damage in WD manifest as limb shaking, increased muscle tone, slurred speech, salivation, and unsteady standing. Adverse reactions to the SARS-CoV-2 vaccine were mainly local injection site reactions (injection site pain, redness, swelling, rash, and itching) and systemic reactions (headache, fever, fatigue, malaise, muscle pain, arthralgia, cough, dyspnea, nausea, diarrhea, anorexia, and vomiting) within 14 days of vaccination.
2.8. Statistical analysis
The measurement data conforming to a normal distribution were expressed as mean (SD) and analyzed using the t-test for comparison. Measurement data that did not conform to a normal distribution were used in the Wilcoxon Signed-Rank Test. Count data were expressed as composition ratios or rates (%), and chi-square (χ2) test was used for analysis. To compare the baseline data between the two groups, age, male, liver cirrhosis, hypersplenism, post-splenectomy, kidney damage, bone damage, and cholecystitis were used as confounding factors to compare the differences between the vaccinated and unvaccinated groups. Differences in UWDRS scores between vaccinated and unvaccinated patients were analyzed using Independent-Sample t-test. Paired Sample t-test were used to analyze whether there were differences in UWDRS scores before and after vaccination in vaccinated patients. Logistic regression analysis was used to assess the correlation between vaccination status and increased UWDRS score. A significance level of α = 0.05 and P < 0.05 was considered statistically significant in all statistical analyses. Data analysis and processing were performed using IBM SPSS Statistics 27.0 (International Business Machines Corporation).
3. Results
3.1. Study population characteristics and vaccination status
In this study, 23 patients refused to participate, and 28 were excluded due to severe dysarthria and cognitive impairment, which prevented them from cooperating. A total of 554 WD patients were included in this study (). The age range was 6–67 years, with a mean (SD) age of 25.3 (10.85) years. The study included 336 males (60.6%) and 218 females (39.4%). A total of 368 WD patients (66.4%) received at least one dose of the vaccine (1 dose: n = 10, 2 doses: n = 161, 3 doses: n = 197). Vaccine types included inactivated vaccine (n = 350, 95.1%), adenovirus-vectored vaccine (n = 25, 6.8%), and recombinant novel coronavirus vaccine (n = 2, 0.5%), among which nine patients were vaccinated with inactivated vaccine and adenovirus vector vaccine. 186 WD patients (33.6%) were unvaccinated. In this study, none of the patients had been infected with SARS-CoV-2 as of the survey date ().
Figure 1. Cohort development in Wilson’s disease study with and without the SARS-CoV-2 vaccination.
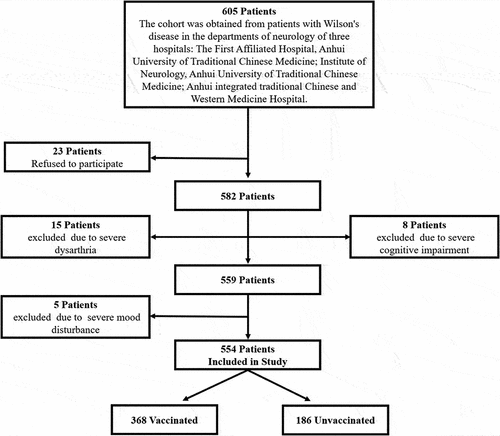
Table 1. Patients’ characteristics and vaccination status.
This study specifically analyzed the regional distribution of the participants. The results showed that the participants covered 4 municipalities, 22 provinces, and 5 autonomous regions in China (). Due to the geographical location of the study site and the epidemic, the most distributed regions were Anhui (n = 164, 29.6%), Jiangsu (n = 68, 12.3%), and Shandong (n = 56, 10.1%). The vaccination rates for WD patients varied by region, with Anhui (66.46%, 109/164) having the highest vaccination rate ().
Figure 2. Population distribution and regional vaccination rate of WD patients (color).
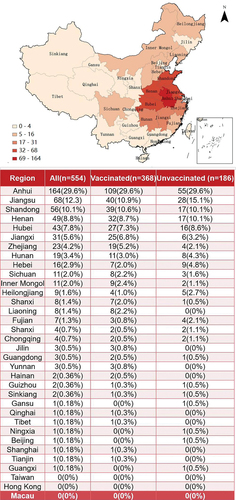
3.2. Subjective reasons related to the unvaccinated status
We obtained the reason why patients with WD did not receive the vaccine using a questionnaire. 83 patients (44.6%) followed the advice of their doctors or vaccination centers, 63 (33.9%) were more concerned about whether the vaccine would aggravate WD symptoms and the remaining 40 (21.5%) doubted the efficacy and safety of the vaccine (). Few clinical studies have been conducted on the safety of SARS-CoV-2 vaccination in patients with WD. The lack of a clinical basis for medical centers to provide consistent advice is a major factor influencing the vaccination of WD patients.
Figure 3. The subjective reason for not vaccinating (color).
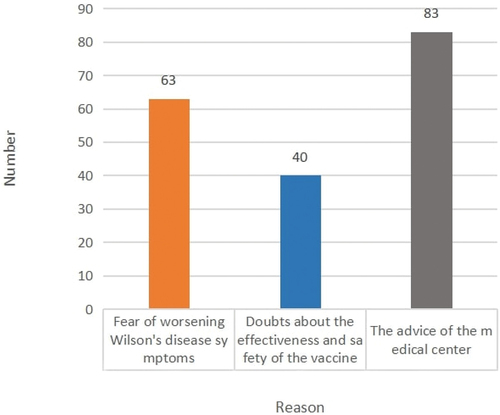
3.3. Safety assessment
A total of 368 and 186 patients were included in the vaccinated and unvaccinated groups, respectively. The two groups were compared based on confounding factors, such as age, male sex, liver cirrhosis, hypersplenism, post-splenectomy, kidney damage, bone damage, and cholecystitis. The results showed no significant difference in the confounding factors between the two groups (P > 0.05) ().
Table 2. Analysis of confounding factors and multi-factors in vaccination combination group without vaccination.
This indicates that the baseline data maintained the balance of the two groups. Multifactorial regression analysis showed that age (OR = 0.996, 95% CI: 0.979–1.014, P = 0.688), male (OR = 0.870, 95% CI: 0.602–1.256, P = 0.457), liver cirrhosis (OR = 0.834, 95% CI: 0.549–1.267, P = 0.395), hypersplenism (OR = 0.811, 95% CI: 0.535–1.228, P = 0.322), post-splenectomy (OR = 0.565, 95% CI: 0.296–1.080, P = 0.084), kidney damage (OR = 0.938, 95% CI: 0.590–1.492, P = 0.788), bone damage (OR = 0.928, 95% CI: 0.428–2.015, P = 0.851), and cholecystitis (OR = 0.951, 95% CI: 0.531–1.703, P = 0.866) were not associated with vaccination status ().
The UWDRS scores of the two groups were compared using the t-test. As shown in , there were no significant differences in the UWDRS scores between the vaccinated and unvaccinated patients (P > 0.05). There were also no differences in the UWDRS scores of the vaccinated patients before and after vaccination (P > 0.05) (). Logistic regression analysis showed that vaccination against SARS-CoV-2 was not significantly associated with increased scores on the UWDRS (OR = 0.924, 95% CI: 0.336–2.540, P = 0.879), hepatic subscale (OR = 0.170, 95% CI: 0.148–3.027, P = 0.603), neurological subscale (OR = 1.322, 95% CI: 0.219–7.984, P = 0.761), or psychiatric subscale (OR = 1.011, 95% CI: 0.183–5.571, P = 0.990) ().
Figure 4. Association of vaccination status with increased UWDRS score in patients with Wilson’s disease (color).
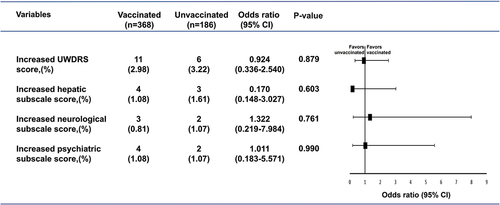
Table 3. Impact of vaccination on UWDRS score.
3.4. Adverse events after vaccination
Among the 368 WD patients who received at least 1 dose of vaccine, a total of 78 patients (21.2%) had adverse events, including 34 (15.5%) males and 44 (29.5%) females. The type of vaccine given to these 78 patients was inactivated SARS-CoV-2 vaccine. 8 patients (2.2%) showed worsening symptoms of extrapyramidal damage, 4 (1.1%) had increased limb shaking, 1 (0.3%) had slurred speech, 1 (0.3%) had salivation, and 2 (0.5%) had unsteady standing. Local and systemic adverse reactions occurred in 70 (19.0%) patients, 48 (13.0%) had local pain at the injection site, 6 (1.6%) had muscle swelling of the vaccination arm, 6 (1.6%) had fever, 4 (1.1%) had headache, 3 (0.8%) had fatigue, 2 (0.5%) had stomachache, and 1 (0.3%) had palpitation. Overall, there was a low incidence of adverse events in WD patients after vaccination (). In our study, no similar adverse events were observed in 186 unvaccinated patients with WD.
Table 4. Adverse events following COVID-19 vaccination in WD patients.
4. Discussion
In 2020, with the rapid worldwide spread of the pandemic disease COVID-19, a massive COVID-19 vaccination campaign is underway worldwide [Citation31]. COVID-19 is highly contagious, and it is mainly transmitted by respiratory droplets and close contact with an infected individual [Citation32]. Several studies have shown that COVID-19 causes neurological and hepatic damage [Citation7–9]. The impaired copper metabolism characteristic of WD makes it possible to develop pathologic copper deposition in various organs and tissues of the body, which can lead to multisystem damage [Citation33]. Compared with the general population, patients with WD, in which neurological and liver damage are the main clinical symptoms, have a greater need for the prevention of SARS-CoV-2 infection. A recent study observed the clinical characteristics of 68 WD patients during the COVID-19 pandemic and found no deterioration in hepatic and extrahepatic conditions but an increased incidence of complications, such as respiratory infections, in patients treated with anti-copper [Citation34]. Therefore, adherence to regular anti-copper therapy and prophylaxis of SARS-CoV-2 infection should be clinically recommended for WD patients.
Current clinical evidence shows that COVID-19 vaccination protects against severe symptoms of the disease but is also an important tool to decrease the spread of the virus and the infection rate [Citation31]. As of 23 August 2022, 12.4 billion doses of the vaccine have been administered worldwide [Citation35]. Clinical trials have confirmed that all COVID-19 vaccines have high efficacy against the original strain and the variants of concern, and were well tolerated [Citation14]. Serious adverse events, including liver injury, myocarditis, immune thrombotic thrombocytopenia, and nephrotic syndrome have been reported to occur after vaccination [Citation23,Citation36–38]. The safety of the SARS-CoV-2 vaccine in patients with WD has not been studied, and there is confusion about the availability of the vaccine in such patients.
In this population-based cohort study, we focused on the current status of SARS-CoV-2 vaccination among Chinese WD patients. The 554 patients with WD included 4 municipalities, 22 provinces, and 5 autonomous regions in China. Anhui, where the research team is located at a clinical research center of the State Administration of Traditional Chinese Medicine of the People’s Republic of China for major and difficult diseases – Wilson’s disease [Citation39]. Patients with WD in Anhui and its border areas are more convenient to seek medical treatment. Therefore, the number of patients in Anhui, Jiangsu and Shandong is relatively high. In contrast, the number of patients in remote areas was relatively low, such as Beijing, Guangxi, and Tianjin. In April 2022, the COVID-19 outbreak was centered in Shanghai, China. The number of patients in Shanghai has also decreased due to the epidemic and social control measures. Patients in Hong Kong, Macau, and Taiwan were missing because of the dual impact of regional distance and the epidemic. In addition, due to better medical resources in Beijing, Shanghai, Hong Kong, Macao, and Taiwan, patients may choose to be seen locally, which is the reason why there were fewer patients from these regions in our study. None of the patients had been infected with SARS-CoV-2. This may be related to the lower prevalence of SARS-CoV-2 infection in China and the higher awareness of self-protection against the disease among WD patients [Citation40].
Vaccine hesitancy is defined by the World Health Organization (WHO) as a behavior in which a group of people holds varying degrees of hesitancy about specific vaccines or vaccination in general [Citation41]. As was the case during the COVID-19 pandemic, concerns about the safety of the SARS-CoV-2 vaccine may have led to vaccine hesitancy. Shroff et al. reported a series of patients who developed liver injury after COVID-19 vaccination [Citation36]. Previous studies have shown that vaccine-induced neurological adverse reactions exist but are rare. Epilepsy and demyelinating diseases have been clinically reported [Citation42]. More than 92% of the Chinese population received at least one dose of COVID-19 vaccine till now [Citation43], while only 66.4% of WD patients in this study were vaccinated. The vaccination rate of patients with WD was significantly lower than that in the whole of China. Concerns regarding the safety of the SARS-CoV-2 vaccine may lead to vaccine hesitancy in patients with WD. The recommendations of medical centers and doubts about the effectiveness of vaccines are the main reason why some patients with WD are not vaccinated.
The impact of the COVID-19 pandemic on neurological and psychiatric symptoms of WD has attracted the attention of researchers. Some experts suggested that it is necessary to observe the impact of COVID-19 pandemic on the neurological and psychiatric symptoms of WD patients during and after the pandemic [Citation44]. Our study observed the impact of the COVID-19 vaccine on the neurological and psychiatric systems of WD patients, which is also of interest. WD has a highly complex clinical manifestation that includes liver, neurological, and psychiatric disorders [Citation12]. The UWDRS is a uniform clinical rating scale for WD that can be used to assess the extent of liver, neurological, and psychiatric impairment in patients [Citation30]. Our study is the first to evaluate the safety of SARS-CoV-2 vaccination in patients with WD. After excluding the effect of confounding factors, the study showed that vaccination did not lead to increased liver, neurological, or psychiatric damage, which is common in WD. There was no correlation between vaccination status and increased UWDRS score. This provides a clinical basis for the safety of SARS-CoV-2 vaccination in WD patients.
Statistically, common adverse events following COVID-19 vaccination include injection site pain, fever, fatigue, and headache [Citation45]. The incidence of adverse events was as follows: fever (2.85%), headache (1.34%), fatigue (3.9%), and pain (14.6%). The most common neurological side effects of the SARS-CoV-2 vaccine are headache, Guillain-Barre syndrome, and transverse myelitis, but they occur infrequently [Citation20]. In a study of the safety of vaccination in 704,003 subjects who received the first dose of the mRNA vaccine, 0.6% had neurological manifestations such as headache and transient sensory symptoms, and there were only 17 serious adverse events, seizures (7), functional syndromes (4), Guillain-Barré syndrome (3), and transverse myelitis (2) [Citation46]. A clinical trial of the safety of the inactivated SARS-CoV-2 vaccine showed that local injection site adverse reactions in 336 vaccine recipients were 53 (16%) pain, 6 (2%) swelling, and systemic adverse reactions were 7 (2%) fever, 9 (3%) fatigue, and 4 (1%) headaches [Citation47]. In our study, a total of 78 (21.2%) of the 368 patients who received at least 1 dose of vaccine had adverse events. 8 patients (2.2%) had worsening symptoms of extrapyramidal damage. Local and systemic adverse reactions occurred in 70 (19.0%) patients, 48 (13.0%) patients had local pain at the injection site, and 6 (1.6%) had swelling in the vaccination arm. Systemic adverse reactions were 6 (1.6%) fevers, 4 (1.1%) headaches, and 3 (0.8%) fatigue. Compared with data reported in the literature, there was no apparent increase in the incidence of adverse reactions to vaccination in patients with WD.
Previous studies have reported that sex and gender differences affect adverse reactions following vaccination [Citation48]. Females typically develop a more sensitive antibody response and experience more adverse events after vaccination compared to males [Citation49]. A study of gender differences in adverse reactions to the inactivated SARS-CoV-2 vaccine in 1397 Chinese medical personnel showed a higher incidence of common adverse reactions such as muscle pain, fatigue, and headache in women than in men after vaccination [Citation50]. However, studies based on Pfizer-BioNTech and Moderna COVID-19 vaccination in the United States have shown that males are more likely to experience serious adverse events or death than females [Citation51]. The presence of adverse reactions to the SARS-CoV-2 vaccine gender differences may be related to the type of vaccine. In this study, 34 (15.5%) male and 44 (29.5%) female patients who received SARS-CoV-2 inactivated vaccine experienced adverse reactions. Of these, 7 males (3.2%) and 1 female (0.7%) showed worsening symptoms of extrapyramidal damage. About local and systemic adverse reactions to the vaccine, the incidence of muscle pain (20.1%), muscle swelling (2.7%), and fever (2.7%) was apparently higher in females than in males for muscle pain (8.2%), muscle swelling (0.9%), and fever (0.9%).
Before this study, there was a gap in the assessment of vaccination safety in patients with WD. Medical professionals lacked a clinical basis to answer WD patients’ vaccination questions. Our study resolves patient confusion and provides a basis for multi-system disorders such as liver injury and other neurologic disorders. We recommend that neurologists and hepatologists when confronted with the issue of vaccination in WD patients, choose to make patients fully aware of the pros and cons of SARS-CoV-2 infection and vaccination before making a choice. This study is limited to the population in China, but WD patients in different regions may have different responses to the vaccine due to different races. We look forward to the research about the COVID-19 vaccination effects on WD patients from other parts of the world.
5. Advantages and limitations
The strength of this study is that it was a multicenter study of a large cohort of WD patients that investigated vaccination in WD patients and performed a safety analysis of the adverse events after vaccination. No previous safety studies have been conducted on vaccination in patients with WD. The results of our study provide a clinical basis and recommendations for patients and healthcare professionals. The limitations of this study are as follows: Our investigation and follow-up focused on the period from 3 months to 12 months after vaccination, and a longer follow-up is needed to determine whether vaccination has a more distant effect on WD patients.
6. Conclusion
In this cohort study, vaccination against SARS-CoV-2 was safe in patients with WD, providing evidence for the safety of vaccination in patients with WD. It provides a clinical rationale and recommendations for SARS-CoV-2 vaccination decisions for WD patients. It also provides a reference for whether patients with other heavy metal metabolic disorders, liver damage, brain damage, and similar diseases can receive the SARS-CoV-2 vaccine. More safety studies are needed to increase vaccination intentions among patients with WD and to help them cope with the COVID-19 pandemic.
Declaration of interests
The authors have no relevant affiliations or financial involvement with any organization or entity with a financial interest in or financial conflict with the subject matter or materials discussed in the manuscript. This includes employment, consultancies, honoraria, stock ownership or options, expert testimony, grants or patents received or pending, or royalties.
Authors’ contributions
Hui Han and Limin Wu have full access to all data in the study and are responsible for the data’s integrity and the data analysis’s accuracy; Hui Han, Dan Zhao, and Xinru Fang contributed equally to this work. Hui Han, Dan Zhao, and Xinru Fang are the co-first authors; Conception and design: Hui Han, Limin Wu; Case collection: Dan Zhao, Xinru Fang, Mengli Wang, Zhihui Ji, Qianzhuo Liu, Luyao Wang, Juan Zhang, Zhifeng Hou, Lei Hua, Yu Wang; Data collection, data analysis, or interpretation: Dan Zhao, Xinru Fang, Wenming Yang, Mengli Wang, Zhihui Ji, Juan Zhang, Zhifeng Hou, Lei Hua, Yu Wang; Manuscript drafting: Dan Zhao, Xinru Fang; Critical revision of the manuscript for important intellectual content: Hui Han,Limin Wu; Statistical analysis: Dan Zhao, Xinru Fang; Obtaining funding: Hui Han,Limin Wu; Administrative, technical, or material support: Hui Han,Limin Wu; Supervision: Limin Wu. All authors read and approved the final manuscript.
Ethics approval and consent to participate
All patient identifiers were removed from the database, and no personal identifiers were used during the study. This study was conducted in accordance with the guidelines of the Declaration of Helsinki. This study was approved by the Medical Ethics Committee of the First Affiliated Hospital of Anhui University of Traditional Chinese Medicine (No. 2021MCZQ07).
Availability of data and materials
The datasets used or analyzed during the current study are available from the corresponding author on reasonable request.
Acknowledgments
This manuscript has been stored on the preprint server and is available at the website (https://www.researchsquare.com/article/rs-2152063/latest).
Additional information
Funding
References
- Asselah T, Durantel D, Pasmant E, et al. COVID-19: discovery, diagnostics and drug development. J Hepatol. 2021;74(1):168–184. doi: 10.1016/j.jhep.2020.09.031
- Johns Hopkins University and Medicine Coronavirus Resource Center. (accessed Aug 30, 2022). https://coronavirus.jhu.edu
- Zhou P, Yang XL, Wang XG, et al. A pneumonia outbreak associated with a new coronavirus of probable bat origin [published correction appears in nature. Nature. 2020;579(7798):270–273. doi: 10.1038/s41586-020-2012-7
- Majumder J, Minko T. Recent developments on therapeutic and diagnostic approaches for COVID-19. AAPS J. 2021;23(1):14 [Published 2021 Jan 5]. doi: 10.1208/s12248-020-00532-2
- Nardo AD, Schneeweiss-Gleixner M, Bakail M, et al. Pathophysiological mechanisms of liver injury in COVID-19. Liver Int. 2021;41(1):20–32. doi: 10.1111/liv.14730
- Abboud H, Abboud FZ, Kharbouch H, et al. COVID-19 and SARS-Cov-2 infection: pathophysiology and clinical effects on the Nervous System. World Neurosurg. 2020;140:49–53. doi: 10.1016/j.wneu.2020.05.193
- Fisicaro F, Di Napoli M, Liberto A, et al. Neurological sequelae in patients with COVID-19: a histopathological perspective. Int J Environ Res Public Health. 2021;18(4):1415. doi: 10.3390/ijerph18041415
- Zubair AS, McAlpine LS, Gardin T, et al. Neuropathogenesis and neurologic manifestations of the Coronaviruses in the age of coronavirus disease 2019: a review. JAMA Neurol. 2020;77(8):1018–1027. doi: 10.1001/jamaneurol.2020.2065IF:29.0Q1
- Musa S. Hepatic and gastrointestinal involvement in coronavirus disease 2019 (COVID-19): what do we know till now? Arab J Gastroenterol. 2020;21(1):3–8. doi: 10.1016/j.ajg.2020.03.002
- Leung M, Aronowitz PB, Medici V. The present and future challenges of Wilson’s disease diagnosis and treatment. Clin Liver Dis. 2021;17(4):267–270 [Published 2021 May 1]. doi: 10.1002/cld.1041
- Xie JJ, Wu ZY. Wilson's Disease in China. Neurosci Bull. 2017;33(3):323–370. doi: 10.1007/s12264-017-0107-4
- Bandmann O, Weiss KH, Kaler SG. Wilson's disease and other neurological copper disorders. Lancet Neurol. 2015;14(1):103–113. doi: 10.1016/S1474-4422(14)70190-5
- Wang T, Wu L, Chen Q, et al. Copper deposition in Wilson’s disease causes male fertility decline by impairing reproductive hormone release through inducing apoptosis and inhibiting ERK signal in hypothalamic-pituitary of mice. Front Endocrinol. 2022 [Published 2022 Aug 5];13:961748.
- Huang Z, Xu S, Liu J, et al. Effectiveness of inactivated and Ad5-nCov COVID-19 vaccines against SARS-CoV-2 omicron BA. 2 variant infection, severe illness, and death. BMC Med. 2022;20(1):400 [Published 2022 Oct 20]. doi: 10.1186/s12916-022-02606-8
- Fiolet T, Kherabi Y, MacDonald CJ, et al. Comparing COVID-19 vaccines for their characteristics, efficacy and effectiveness against SARS-CoV-2 and variants of concern: a narrative review. Clin Microbiol Infect. 2022;28(2):202–221. doi: 10.1016/j.cmi.2021.10.005
- Husby A, Hansen JV, Fosbøl E, et al. SARS-CoV-2 vaccination and myocarditis or myopericarditis: population based cohort study. BMJ. 2021;375:e068665. Published 2021 Dec 16. doi: 10.1136/bmj-2021-068665.
- Marcucci R, Marietta M. Vaccine-induced thrombotic thrombocytopenia: the elusive link between thrombosis and adenovirus-based SARS-CoV-2 vaccines. Intern Emerg Med. 2021;16(5):1113–1119. doi: 10.1007/s11739-021-02793-x
- Avci E, Abasiyanik F. Autoimmune hepatitis after SARS-CoV-2 vaccine: new-onset or flare-up? J Autoimmun. 2021;125:102745. doi: 10.1016/j.jaut.2021.102745
- Cirillo N, Doan R. Bell’s palsy and SARS-CoV-2 vaccines-an unfolding story. Lancet Infect Dis. 2021;21(9):1210–1211. doi: 10.1016/S1473-3099(21)00273-5
- Finsterer J. Neurological side effects of SARS-CoV-2 vaccinations. Acta Neurol Scand. 2022;145(1):5–9. doi: 10.1111/ane.13550
- Alhumaid S, Al Mutair A, Rabaan AA, et al. New-onset and relapsed liver diseases following COVID-19 vaccination: a systematic review. BMC Gastroenterol. 2022;22(1):433 [Published 2022 Oct 13]. doi: 10.1186/s12876-022-02507-3
- Rocco A, Sgamato C, Compare D, et al. Autoimmune hepatitis following SARS-CoV-2 vaccine: May not be a casuality. J Hepatol. 2021;75(3):728–729. doi: 10.1016/j.jhep.2021.05.038
- Izzedine H, Bonilla M, Jhaveri KD. Nephrotic syndrome and vasculitis following SARS-CoV-2 vaccine: true association or circumstantial? Nephrol Dial Transplant. 2021;36(9):1565–1569. doi: 10.1093/ndt/gfab215
- Nagai K, Kageyama M, Iwase M, et al. A young adult with nephrotic syndrome following COVID-19 vaccination. CEN Case Rep. 2022;11(3):397–398. doi: 10.1007/s13730-021-00673-z
- Hummel A, Oniszczuk J, Kervella D, et al. Idiopathic nephrotic syndrome relapse following COVID-19 vaccination: a series of 25 cases. Clin Kidney J. 2022;15(8):1574–1582 [Published 2022 May 6]. doi: 10.1093/ckj/sfac134
- Candelli M, Rossi E, Valletta F, et al. Immune thrombocytopenic purpura after SARS-CoV-2 vaccine. Br J Haematol. 2021;194(3):547–549. doi: 10.1111/bjh.17508
- National Health Commission of the People’s Republic of China. (accessed Aug 30, 2022). http://www.nhc.gov.cn/
- Li LY, Zhu XQ, Tao WW, et al. Acute onset neurological symptoms in Wilson disease after traumatic, surgical or emotional events: a cross-sectional study. Medicine (Baltimore). 2019;98(26):e15917. doi: 10.1097/MD.0000000000015917
- Lubnow M, Schmidt B, Fleck M, et al. Secondary hemophagocytic lymphohistiocytosis and severe liver injury induced by hepatic SARS-CoV-2 infection unmasking Wilson’s disease: balancing immunosuppression. Int J Infect Dis. 2021;103:624–627. doi: 10.1016/j.ijid.2020.12.047
- Volpert HM, Pfeiffenberger J, Gröner JB, et al. Comparative assessment of clinical rating scales in Wilson’s disease. BMC neurol. 2017;17(1):140 [Published 2017 Jul 21]. doi: 10.1186/s12883-017-0921-3
- Vitiello A, Ferrara F, Troiano V, et al. COVID-19 vaccines and decreased transmission of SARS-CoV-2. Inflammopharmacology. 2021;29(5):1357–1360. doi: 10.1007/s10787-021-00847-2
- Du P, Li D, Wang A, et al. A systematic review and meta-analysis of risk factors associated with severity and death in COVID-19 patients. Can J Infect Dis Med Microbiol. 2021 [Published 2021 Apr 10];2021:6660930.
- Członkowska A, Litwin T, Dusek P, et al. Wilson disease. Nat Rev Dis Primers. 2018;4(1):21. doi: 10.1038/s41572-018-0018-3
- Zhuang YP, Zhong HJ. Impact of COVID-19 on the clinical status of patients with Wilson disease. World J Gastroenterol. 2021;27(26):4248–4251. doi: 10.3748/wjg.v27.i26.4248
- WHO Coronavirus (COVID-19) Dashboard. Geneva: World Health Organization. https://covid19.who.int/
- Shroff H, Satapathy SK, Crawford JM, et al. Liver injury following SARS-CoV-2 vaccination: a multicenter case series. J Hepatol. 2022;76(1):211–214. doi: 10.1016/j.jhep.2021.07.024
- Gargano JW, Wallace M, Hadler SC, et al. Use of mRNA COVID-19 vaccine after reports of myocarditis among vaccine recipients: update from the Advisory Committee on Immunization Practices - United States, June 2021. MMWR Morb Mortal Wkly Rep. 2021 [Published 2021 Jul 9];70(27):977–982. doi: 10.15585/mmwr.mm7027e2
- Cines DB, Bussel JB. SARS-CoV-2 vaccine-induced immune thrombotic thrombocytopenia [published correction appears in. N Engl J Med.2021;384(23):2254–2256. doi: 10.1056/NEJMe2106315
- Zhang S, Yang W, Li X, et al. Clinical and genetic characterization of a large cohort of patients with Wilson’s disease in China. Transl Neurodegener. 2022 [Published 2022 Feb 28];11(1):13 . doi: 10.1186/s40035-022-00287-0
- The epidemic distribution of COVID-19. (accessed Aug 30, 2022).https://2019ncov.chinacdc.cn/2019-nCoV/
- Troiano G, Nardi A. Vaccine hesitancy in the era of COVID-19. Public Health. 2021;194:245–251. doi: 10.1016/j.puhe.2021.02.025
- Lu L, Xiong W, Mu J, et al. The potential neurological effect of the COVID-19 vaccines: a review. Acta Neurol Scand. 2021;144(1):3–12. doi: 10.1111/ane.13417
- National Health Commission of the People’s Republic of China. (accessed Aug 30, 2022). http://www.nhc.gov.cn
- Lanza G, Godani M, Ferri R, et al. Impact of COVID-19 pandemic on the neuropsychiatric status of Wilson’s disease. World J Gastroenterol. 2021;27(39):6733–6736. doi: 10.3748/wjg.v27.i39.6733
- Chen M, Yuan Y, Zhou Y, et al. Safety of SARS-CoV-2 vaccines: a systematic review and meta-analysis of randomized controlled trials. Infect Dis Poverty. 2021;10(1):94 [Published 2021 Jul 5]. doi: 10.1186/s40249-021-00878-5
- García-Grimshaw M, Ceballos-Liceaga SE, Hernández-Vanegas LE, et al. Neurologic adverse events among 704,003 first-dose recipients of the BNT162b2 mRNA COVID-19 vaccine in Mexico: a nationwide descriptive study. Clin Immunol. 2021;229:108786. doi: 10.1016/j.clim.2021.108786
- Xia S, Zhang Y, Wang Y, et al. Safety and immunogenicity of an inactivated SARS-CoV-2 vaccine, BBIBP-CorV: a randomised, double-blind, placebo-controlled, phase 1/2 trial. Lancet Infect Dis. 2021;21(1):39–51. doi: 10.1016/S1473-3099(20)30831-8
- Klein SL, Jedlicka A, Pekosz A. The xs and Y of immune responses to viral vaccines [published correction appears in Lancet Infect Dis. Lancet Infect Dis. 2010 Nov;10(5):338–349. doi: 10.1016/S1473-3099(10)70049-9
- Klein SL, Marriott I, Fish EN. Sex-based differences in immune function and responses to vaccination. Trans R Soc Trop Med Hyg. 2015;109(1):9–15. doi: 10.1093/trstmh/tru167
- Zhu JS, Zhang MX, Chien CW, et al. Sex differences in adverse reactions to an inactivated SARS-CoV-2 vaccine among medical staff in China. Front Med. 2021 [Published 2021 Sep 8];8:731593.
- Xiong X, Yuan J, Li M, et al. Age and gender disparities in adverse events following COVID-19 vaccination: Real-World evidence based on big data for risk management. Front Med. 2021 [Published 2021 Jul 19];8:700014.