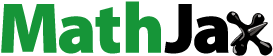
ABSTRACT
Background
This study aimed to evaluate VE of primary, first, and second booster ancestral-strain monovalent mRNA COVID-19 vaccination against symptomatic infections and severe diseases in Japan.
Methods
We conducted a test-negative case-control study. We included medically attended episodes and hospitalizations involving individuals aged 16 with signs and symptoms from July to November 2022, when Omicron BA.5 was dominant nationwide. To evaluate VE, we calculated adjusted ORs of vaccination among test-positive versus test-negative individuals using a mixed-effects logistic regression.
Results
For VE against symptomatic infections among individuals aged 16 to 59, VE of primary vaccination at > 180 days was 26.1% (95% CI: 10.6–38.8%); VE of the first booster was 58.5% (48.4–66.7%) at 90 days, decreasing to 41.1% (29.5–50.8%) at 91 to 180 days. For individuals aged
60, VE of the first booster was 42.8% (1.7–66.7%) at
90 days, dropping to 15.4% (−25.9–43.2%) at 91 to 180 days, and then increasing to 44.0% (16.4–62.5%) after the second booster. For VE against severe diseases, VE of the first and second booster was 77.3% (61.2–86.7%) at
90 days and 55.9% (23.4–74.6%) afterward.
Conclusion
mRNA booster vaccination provided moderate protection against symptomatic infections and high-level protection against severe diseases during the BA.5 epidemic in Japan.
1. Introduction
The first infection with severe acute respiratory syndrome coronavirus 2 (SARS-CoV-2) Omicron was identified in Japan at the end of November 2021 [Citation1]. From the beginning of January 2022, the Omicron variant started spreading with marked transmissibility and high-level evasion of vaccine-induced immunity against it [Citation2]. Following BA.1- and BA.2- predominant periods between January and June 2022, BA.5 became dominant from July 2022 in Japan (Supplementary Figures S1 and S2) [Citation3]. Before the BA.5 epidemic, the fourth dose (second booster) of the ancestral-strain monovalent coronavirus 2019 (COVID-19) messenger RNA (mRNA) vaccine was introduced for individuals aged ≥60 years and those aged 18 to 59 years with the following underlying medical conditions: chronic heart disease, chronic respiratory disease, chronic kidney diseases, chronic liver disease, immunosuppressive status including malignancy, use of immunosuppressive medicines, neurological diseases, chromosomal abnormality, hematological disease (except for iron-deficiency anemia), diabetes mellitus, sleep apnea syndrome, severe mental disorder, mental retardation, and obesity (body mass index ≥30 kg/m2) [Citation4].
The mRNA in the mRNA vaccine is encapsulated in lipid-nanoparticles, enabling mRNA to be taken into host cells to facilitate expression of the SARS-CoV-2 spike (S) antigen. The vaccine induces both neutralizing antibody and cellular immune responses to the antigen, which provides protection against SARS-CoV-2 [Citation5–7]. Primary vaccination (two doses) of mRNA COVID-19 vaccine proved to be highly effective against symptomatic infections and severe outcomes caused by the Delta variant [Citation8]. On the other hand, primary vaccination of mRNA COVID-19 vaccines was reported to have provided insufficient protection against symptomatic infection caused by the Omicron variant, while the first booster dose of mRNA COVID-19 vaccines increased effectiveness against symptomatic infection. For severe outcomes caused by the Omicron variant, primary vaccination of mRNA COVID-19 vaccines provided modest protection, and the first booster provided additional protection [Citation9]. Furthermore, COVID-19 vaccination provided protection against prolonged hospitalization and work absenteeism, although the reporting study was not limited to mRNA COVID-19 vaccines [Citation10].
Ongoing vaccine effectiveness (VE) monitoring is imperative for evaluating public health strategies. Up to the present in Japan, COVID-19 vaccination has been publicly funded. However, with changes in the epidemic situation and disease severity, it may be difficult to publicly fund all future COVID-19 vaccinations. To discuss and determine the optimal COVID-19 vaccination policy in Japan, several questions have been raised, such as which population groups should be included into the national immunization program and how often vaccination should be administered. Additionally, transmissibility, vaccine effectiveness, and the disease severity could change depending on the SARS-CoV-2 variants, and new COVID-19 vaccinations targeting specific variants have been introduced and develop; thus, it is essential to provide up-to-date knowledge of VE via a longitudinal framework.
Estimating VE is challenging and complex due to the following factors: proportion of individuals with vaccine- and infection-induced immunity, additional booster vaccination, various kinds of vaccine products, changes in health care policy for COVID-19, and transition of circulating variants [Citation11,Citation12]. These factors differ depending on countries or geographic areas. However, limited evidence was reported regarding VE of monovalent mRNA COVID-19 booster vaccines against both mild and severe disease during the BA.5 epidemic period, especially in Asian countries.
The objectives of this study were to evaluate VE of primary series, first (third dose), and second (fourth dose) booster vaccination of ancestral-strain monovalent mRNA COVID-19 vaccines against symptomatic SARS-CoV-2 infections and severe COVID-19 during the SARS-CoV-2 Omicron BA.5 epidemic in Japan. Additionally, relative VE of the first booster compared with primary vaccination (two doses) and relative VE of the second booster compared with the first booster vaccination against symptomatic infections were assessed.
2. Methods
2.1. COVID-19 vaccination strategy in Japan
Following two primary doses of BNT162b2, mRNA-1273, or AZD1222, the Japanese government introduced the first booster ancestral-strain monovalent mRNA COVID-19 vaccine in December 2021 for adults ≥18 years [Citation13]. Several studies revealed that the effectiveness of the first monovalent booster waned over time [Citation14–16]. The second monovalent mRNA COVID-19 booster vaccination was introduced for individuals aged ≥60 years and those aged ≥18 years with underlying medical conditions on 25 May 2022 [Citation4]. In response to the increased number of new SARS-COV-2 cases from July 2022, the second booster became available for healthcare professionals on 22 July 2022 [Citation17]. For the primary vaccination series, BNT162b2, mRNA-1273, and AZD1222 were employed. AZD1222 was used until September 2022, and NVX-CoV2373 began to be used in May 2022. For the booster dose, BNT162b2, mRNA-1273, and NVX-CoV2373 were administered. Bivalent mRNA COVID-19 vaccines started being used on 20 September 2022, for those aged ≥12 years with primary vaccination [Citation18]. Until the introduction of bivalent mRNA COVID-19 vaccines, only monovalent mRNA COVID-19 vaccines were available in Japan.
2.2. Study design and settings
This multicenter prospective observational study has been conducted by the Vaccine Effectiveness Real-time Surveillance for SARS-CoV-2 (VERSUS) Group since July 2021. A test-negative design case-control study was applied [Citation19,Citation20]. In the present study, we evaluated VE against symptomatic SARS-CoV-2 infections and severe diseases between 1 July 2022, and 30 November 2022, when most of the circulating variants were SARS-CoV-2 Omicron BA.5 (Supplementary Figure S2). We defined severe disease as severe laboratory-confirmed COVID-19. To evaluate VE against symptomatic infections, we assessed outpatient clinic and emergency department encounters in 11 hospitals and five clinics. To evaluate VE against severe diseases, we assessed hospital admissions in 12 hospitals (Supplementary Figure S3). SARS-CoV-2 test results generated by nucleic acid amplification tests and antigen quantification tests were included in this study. We excluded results generated by the rapid antigen test and did not perform SARS-CoV-2 genome sequencing.
2.3. Study population
This study included individuals aged ≥16 years who medically attended or were hospitalized at participating medical facilities with signs and symptoms and had evidence of SARS-CoV-2 tests. Episodes involving individuals with signs or symptoms during hospitalization were not included. In the analysis of VE against symptomatic infections, cases were episodes involving individuals who medically attended outpatient clinics and emergency departments in the participating hospitals and clinics with one or more COVID-19-related signs and symptoms and test-positive for SARS-CoV-2; controls were episodes involving individuals who medically attended outpatient clinics and emergency departments in participating hospitals and clinics with one or more COVID-19-related signs and symptoms and test-negative for SARS-CoV-2. We defined COVID-19-related signs and symptoms as follow: fever (≥37·5°C), cough, fatigue, shortness of breath, myalgia, sore throat, nasal congestion, headache, diarrhea, taste disorder, and olfactory dysfunction.
In the analysis of VE against severe diseases, cases were hospitalizations at participating hospitals due to acute respiratory illness and/or radiologically confirmed pneumonia diagnosed by attending physicians and test-positive for SARS-CoV-2; controls were hospitalizations at participating hospitals due to acute respiratory illness and/or radiologically confirmed pneumonia diagnosed by attending physicians and test-negative for SARS-CoV-2. Some older patients with respiratory infections present only with nonspecific symptoms such as appetite loss [Citation21], and to incorporate such patients with respiratory infections, our study incorporated patients with radiologically confirmed pneumonia only. Acute respiratory illness was defined as having two or more signs and symptoms as follows: fever (≥37·5°C), cough, sputum, pleuritic chest pain, shortness of breath, tachypnea, or newly requiring oxygen administration. Hospitalizations tested >3 days after admission were excluded. All eligible individuals who visited or were hospitalized at the participating medical facilities were included.
2.4. Data collection and management
Researchers in each medical facility identified eligible episodes and hospitalizations from the medical charts. Patients’ demographics and clinical information were extracted from medical charts and recorded on an electronic form using REDCap [Citation22]. Stored data in REDCap were analyzed by researchers not involved in data collection. In the analysis of VE against symptomatic infections, we collected data on age, sex, place of residence, underlying medical conditions (i.e. chronic heart disease, chronic respiratory disease, obesity [body mass index ≥30 kg/m2], malignancy [including solid or hematological malignancy], diabetes mellitus, chronic kidney disease, receiving dialysis, liver cirrhosis, use of immunosuppressive medicines, and pregnancy), smoking history, history of contact with COVID-19 patients within the last 14 days, healthcare employment status, prior SARS-CoV-2 infection history, clinical symptoms, and COVID-19 vaccination history. We obtained the date of vaccination, types of vaccine product, and vaccination frequency from vaccination cards, medical charts, or interviews with the patients or family members.
In the analysis of VE against hospitalization, we collected data on vital signs and the blood urea nitrogen level at the time of admission, and the presence of radiologically confirmed pneumonia in addition to information collected in the analysis of VE against symptomatic infections. Criteria for hospitalization could vary by the stage of a COVID-19 wave, risk factors of patients, and geographic areas [Citation23]. Therefore, we assessed the CURB-65 score [Citation24] at the time of admission to compare the severity of test-positive cases and that of test-negative controls.
2.5. Definition of vaccination status
The COVID-19 vaccination status was defined based on doses received prior to the symptom onset date and number of days between the receipt of the last vaccination and symptom onset date: (1) unvaccinated, if no vaccine dose was received before symptom onset; (2) one dose within 0–13 days, if one dose was received <14 days before symptom onset; (3) one dose after 14 days if one dose was received ≥14 days before symptom onset; (4) two doses within 0–13 days, if two doses were received <14 days before symptom onset; (5) primary vaccination, if two doses were received ≥14 days before symptom onset; (6) three doses within 0–13 days, if the first monovalent booster (third dose) was received <14 days before symptom onset; (7) the first booster vaccination, if the first monovalent booster (third dose) was received ≥14 days before symptom onset; (8) four doses within 0–13 days, if the second monovalent booster (forth dose) was received <14 days before symptom onset; and (9) the second booster vaccination, if the second monovalent booster (fourth dose) was received ≥14 days before symptom onset. For episodes with an unknown onset date, we defined the vaccination status based on the number of days between receiving the last vaccination and test date. Completion of vaccination was defined as 14 days after the receipt of the last vaccination. For the evaluation of VE separated by the duration after the last receipt of vaccines, the number of days is shown starting from the completion of vaccination.
2.6. Statistical analysis
Characteristics of test-positive cases and test-negative controls were compared using rank-sum tests for continuous variables, Chi-square tests, and Fisher’s exact tests for categorical variables.VE was estimated as one minus an adjusted odds ratio (aOR), expressed as a percentage. Adjusted ORs were calculated by comparing the odds of antecedent COVID-19 vaccination versus unvaccinated status (reference group) among test-positive versus test-negative episodes using a mixed-effects logistic regression model. In the analysis of VE against symptomatic infections, we applied age, sex, presence of underlying medical conditions, calendar week of test, healthcare employment status, and prior SARS-CoV-2 infection history as fixed effects, and medical facilities as a random effect. Since the vaccination strategy for the second booster was different for each age group, VE of the second booster was evaluated solely for individuals aged ≥60 years. The time between symptom onset and completion of the last vaccination (i.e. 14 days after the last receipt of vaccine does) was separated into: 90 days, 91 to 180 days, and >180 days. Relative VE of the first booster compared with primary vaccination at >180 days for individuals aged ≥16 years and relative VE of the second booster compared with first booster vaccination at >180 days for individuals aged ≥60 years against symptomatic infections were assessed.
In the analysis of VE against severe diseases, we restricted individuals aged ≥60 years due to the small sample size of hospitalizations of individuals aged 16 to 59 years. Age, sex, presence of underlying medical conditions, calendar week of hospital admission, and prior SARS-CoV-2 infection history were applied to the model as fixed effects, and medical facilities as a random effect. We conducted the following subgroup analyses: (1) VE for the prevention of hospitalizations with respiratory distress at the time of admission by limiting analysis to individuals with hypoxia (oxygen saturation [SpO2] <90%), oxygen administration, or tachypnea (respiratory rate ≥ 30/minute); (2) VE for the prevention of hospitalizations with moderate or severe disease at the time of admission defined as CURB-65 scores ≥ 2; and (3) VE for the prevention of hospitalizations with radiologically confirmed pneumonia at the time of admission. We did not evaluate relative VE against severe diseases due to the small sample size of episodes involving individuals with primary vaccination. VE of primary series, first, and second booster vaccination and relative VE of the first and second booster vaccination were shown solely in the results.
We excluded the followings: (1) episodes involving individuals vaccinated with COVID-19 vaccines other than BNA162b2 and mRNA-1273 for both primary and booster vaccination; (2) episodes involving individuals vaccinated with bivalent mRNA vaccines since the present study aimed to evaluate the monovalent COVID-19 vaccine; (3) episodes involving individuals who possibly received bivalent COVID-19 vaccines with an unknown precise vaccine product or vaccination date; (4) episodes involving individuals with an unknown vaccination history or vaccinated with an unknown dose; (5) episodes tested >14 days after symptom onset; and (6) for hospitalizations, episodes tested >3 days after admission. In the analysis of VE against symptomatic infection, we applied the following criteria to exclude episodes involving individuals with multiple episodes [Citation1]: episodes with multiple negative test results and identical symptom onset dates [Citation2]; episodes with negative test results within seven days after a previous negative result [Citation3]; episodes with negative test results within three weeks before or after a positive test result [Citation4]; for multiple positive episodes during the study period, only the first episode was included; and [Citation5] we included a maximum of three negative test results for each individual.
This study included individuals whose precise vaccination date was not documented. In the primary analysis, we used the following criteria: for individuals whose precise vaccination date was not documented (for example, only the vaccination month was reported), the midpoint between the two possible dates was set as the vaccination date; and individuals for whom only the number of vaccine doses was recorded were included in the primary-, first booster-, or second booster-vaccinated group, depending on the number of vaccine doses, and we did not include these episodes in the analysis for VE separated by the duration after the completion of vaccination. Sensitivity analysis was conducted due to the above uncertainty using the following scenarios: (1) we set the vaccination date as the most recent possible date from symptom onset when the precise vaccination date was uncertain; and (2) we set the vaccination date as the earliest possible date from symptom onset when the precise vaccination date was uncertain. Additionally, we considered episodes involving an unknown type of vaccine product to be BNT162b2 or mRNA-1273 and included them in the analysis because more than 99.8% of administered COVID-19 vaccines in Japan comprised mRNA vaccines (Supplementary Table S1) [Citation25]. Considering potential differences in characteristics between individuals with clear and unclear vaccine histories, we compared their characteristics. Regarding missing data, we excluded episodes involving individuals with an unknown COVID-19 vaccination status. For other variables, missing data are shown as ‘unknown,’ and frequency and percentages include ‘unknown’ as a category. Sample size calculation was not performed since we included all eligible episodes and hospitalizations. Where p-values were used, a two-sided p-value of < 0.05 was considered significant. All analyses were performed using Stata version 16.0 (Stata Corp., College Station, Texas, U.S.A.).
2.7. Ethical consideration
This study was approved by the Institutional Review Board (IRB) of the Institute of Tropical Medicine, Nagasaki University (approval no.: 210225257) and participating hospitals and clinics. A centralized IRB review process was applied to the medical facilities without IRBs, and the IRB of the Institute of Tropical Medicine approved the study at those medical facilities. As this study did not involve intervention and did not use specimens collected from the human body, the requirement for obtaining consent from individual patients was waived by the IRBs regarding the Ethical Guidelines for Medical Research Involving Human Subjects of Japan.
3. Results
3.1. Analysis of VE against symptomatic infections
3.1.1. Patient characteristics
Among 12,429 episodes involving individuals with COVID-19-related signs or symptoms 10,746 episodes (6,014 test-positive and 4,732 test-negative for SARS-CoV-2) were eligible for analysis (). shows the distribution of the weekly number of test-positive and test-negative episodes by vaccination status included in the analysis. The 463 episodes excluded from the analysis due to an unknown vaccination history were more likely to involve individuals who were test-negative (p < 0.001), older (p < 0.001), and have one or more underlying medical conditions (p < 0.001) (Supplementary Table S2). Regarding episodes included in the analysis, the median age was 41 years (interquartile range [IQR]: 28–57 years); 4,887 (45.5%) were male; 2,407 (22.4%) had one or more underlying medical conditions; 140 (1.3%) were residents of long-term care facilities; 3,036 (28.3%) had a current or past smoking history; 1,914 (17.8%) were healthcare workers; 3,291 (30.6%) had a history of contact with a COVID-19 patient within the last 14 days; and 487 (4.5%) had a prior SARS-CoV-2 infection history (). As the characteristics of individuals, test-positive individuals were more likely to be younger (p < 0.001), and less likely to have one or more underlying medical conditions (p < 0.001). Regarding the vaccination status, test-positive individuals were more likely to be unvaccinated (914 test-positive [15.2%] vs. 425 test-negative [9.0%], p < 0.001) and less likely to have received at least one booster dose compared with test-negative individuals (3,687 test-positive [61.3%] vs. 3,370 test-negative [71.2%], p < 0.001). Among episodes involving individuals aged 60 years, 291 (25.1%) test-positive cases involved patients who had received a second booster, while 497 (38.8%) test-negative controls had received a second booster (p < 0.001).
Figure 1. (a) Sample selection flowchart in the analysis of vaccine effectiveness against symptomatic SARS-CoV-2 infections. (b) Sample selection flowchart in the analysis of vaccine effectiveness against severe diseases.
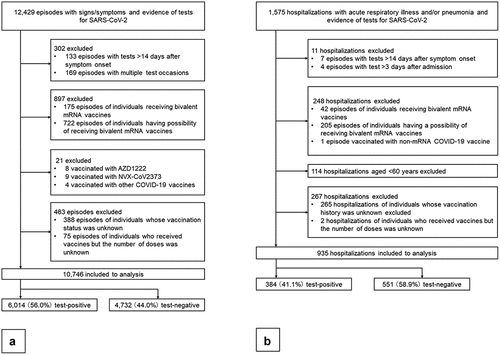
Figure 2. (a) The weekly number of test-positive episodes for SARS-CoV-2 and test-negative episodes for SARS-CoV-2 by vaccination status included in the analysis of vaccine effectiveness against symptomatic SARS-CoV-2 infection. (b) The weekly number of test-positive hospital admissions for SARS-CoV-2 and test-negative hospital admissions for SARS-CoV-2 by vaccination status included in the analysis of vaccine effectiveness against severe diseases.
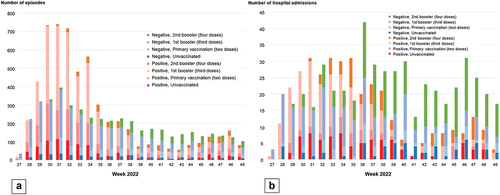
Table 1. Characteristics of individuals included in vaccine effectiveness analysis against symptomatic SARS-CoV-2 infections: VERSUS study, Japan, July 1 to November 30, 2022.
3.1.2. Vaccine effectiveness against symptomatic SARS-CoV-2 infections
For individuals aged 16 to 59 years, VE against symptomatic infections of primary vaccination at >180 days after the completion of vaccination was 26.1% (95% confidence interval [CI]: 10.6–38.8%); VE of the first booster vaccination was 58.5% (95% CI: 48.4–66.7%) at 90 days, 41.1% (95% CI: 29.5–50.8%) at 91 to 180 days, and 31.1% (95% CI: 13.8–44.9%) at >180 days after the completion of vaccination (). Among individuals with the first booster vaccination of BNT162b2, VE was 53.7% (95% CI: 38.6–65.1%) at
90 days, 42.9% (95% CI: 29.1–54.0%) at 91 to 180 days, and 25.8% (95% CI: 2.8–43.4%) at >180 days after the completion of vaccination. Among individuals with the first booster vaccination of mRNA-1273, VE was 52.0% (95% CI: 30.8–66.7%) at
90 days, 37.4% (95% CI: 20.4–50.8%) at 91 to 180 days, and 27.5% (95% CI: −8.9–51.8%) at >180 days after the completion of vaccination. For individuals aged
60 years, VE against symptomatic infections of the first booster vaccination was 42.8% (95% CI: 1.7–66.7%) at
90 days, 15.4% (95% CI: −25.9–43.2%) at 91 to 180 days, and 16.5% (95% CI: −46.1–52.3%) at 180 days after the completion of vaccination; VE against symptomatic infections of the second booster vaccination was 44.0% (95% CI: 16.4–62.5%) at
90 days. We did not evaluate VE stratified by types of vaccine product among individuals aged
60 years due to the small sample size.
Figure 3. Vaccine effectiveness of monovalent mRNA COVID-19 vaccines against symptomatic SARS-CoV-2 infections among individuals aged 16 to 59 years and aged 60 years, VERSUS study, Japan, between July 1 and November 30, 2022. The analysis included test-positive cases with signs or symptoms that tested positive for SARS-CoV-2 and test-negative controls with signs or symptoms that tested negative for SARS-CoV-2. Vaccine effectiveness was adjusted for age, sex, underlying medical conditions, calendar week of test, healthcare professional status, prior SARS-CoV-2 infection history, and medical facilities. The vaccination status was classified into four based on the number of vaccine doses received before symptom onset and number of days between the last vaccination date and symptom onset: unvaccinated, individuals had received no vaccine dose before symptom onset; primary vaccination, individuals had received the second dose
14 days before symptom onset; 1st booster vaccination, individuals had received the third dose
14 days before symptom onset; and 2nd booster vaccination, individuals had received the fourth dose
14 days before symptom onset. Completion of vaccination was defined as 14 days after receiving the last vaccine. When evaluating vaccine effectiveness separated by days after vaccination, the number of days is shown from completion of vaccination.
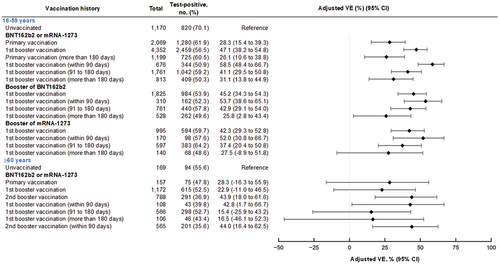
3.1.3. Relative vaccine effectiveness against symptomatic infections
For individuals aged 16 to 59 years, relative VE against symptomatic infection of the first booster vaccination compared with primary vaccination at >180 days was 43.9% (95% CI: 30.9–54.5%) at 90 days, 20.4% (95% CI: 5.9–32.7%) at 91 to 180 days, and 6.8% (95% CI: −15.6–24.9%) at >180 days after the completion of vaccination (). For individuals aged
60 years, relative VE against symptomatic infection of the first booster vaccination compared with primary vaccination at >180 days was 32.4% (95% CI: −31.8–65.3%) at
90 days, −0.02% (95% CI: −74.9–42.8%) at 91 to 180 days, and 1.3% (95% CI: −96.4–50.4%) at >180 days after the completion of vaccination; VE against symptomatic infection of the second booster vaccination compared with first booster vaccination at >180 days was 32.9% (95% CI: −7.8–58.2%) at
90 days.
Figure 4. Relative vaccine effectiveness of monovalent mRNA COVID-19 booster vaccination, compared with the primary vaccination at more than 180 days after the completion of vaccination against symptomatic SARS-CoV-2 infections among individuals aged 16 to 59 years and 60 years, VERSUS study, Japan, between July 1 and November 30, 2022. The analysis included test-positive cases with signs or symptoms that tested positive for SARS-CoV-2 and test-negative controls with signs or symptoms that tested negative for SARS-CoV-2. Relative vaccine effectiveness was adjusted for age, sex, underlying medical conditions, calendar week of test, healthcare professional status, prior SARS-CoV-2 infection history, and medical facilities. The vaccination status was classified into four based on the number of vaccine doses received before symptom onset and number of days between the last vaccination date and symptom onset: unvaccinated, individuals had received no vaccine dose before symptom onset; primary vaccination, individuals had received the second dose
14 days before symptom onset; 1st booster vaccination, individuals had received the third dose
14 days before symptom onset; and 2nd booster vaccination, individuals had received the fourth dose
14 days before symptom onset. Completion of vaccination was defined as 14 days after receiving the last vaccine. When evaluating vaccine effectiveness separated by days after vaccination, the number of days is shown from completion of vaccination. Abbreviation: VE, vaccine effectiveness.
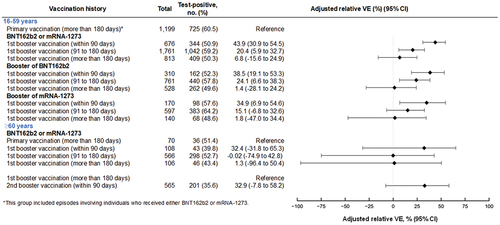
3.2. Analysis of VE against severe diseases
3.2.1. Patient characteristics
Among 1,575 patients hospitalized with acute respiratory illness and/or radiologically confirmed pneumonia, 935 patients aged 60 years (384 test-positive and 551 test-negative for SARS-CoV-2) were eligible for the analysis (). shows the distribution of the weekly number of test-positive and test-negative hospital admissions by vaccination status included in the analysis. The 267 patients excluded from the analysis due to an unknown vaccination history were more likely to be test-negative (p < 0.001, Supplementary Table S3). Concerning hospitalizations included in the analysis, the median age was 82 years (IQR: 75–88 years); 584 (62.5%) were male; 667 (71.3%) had one or more underlying medical conditions; 244 (26.1%) were residents of long-term care facilities; 406 (43.4%) had a current or past smoking history; 177 (18.9%) had a history of contact with a COVID-19 patient within the last 14 days; and 32 (3.4%) had a prior SARS-CoV-2 infection history (). Regarding for clinical information at the time of hospital admission, 525 (56.1%) had respiratory distress, 722 (77.2%) had moderate to severe diseases (CURB-65
2), and 705 (75.4%) had radiologically confirmed pneumonia. The proportion of moderate to severe diseases among test-negative individuals (79.1%) was higher than that among test-positive individuals (74.5%) (p = 0.02). Test-positive individuals were more likely to be unvaccinated (83 test-positive [21.6%] vs. 60 test-negative [10.9%], p < 0.001) and less likely to have received at least one booster dose compared with test-negative individuals (253 test-positive [65.9%] vs. 424 test-negative [77.0%], p < 0.001).
Table 2. Characteristics of individuals aged 60 years included in vaccine effectiveness analysis against severe COVID-19: VERSUS study, Japan, July 1 to November 30, 2022.
3.2.2. Vaccine effectiveness against severe diseases
Among hospitalized patients aged 60 years, VE of the first or second booster was 77.3% (95% CI: 61.2–86.7%) at
90 days, and 55.9% (95% CI: 23.4–74.6%) at 91 to 180 days (). In the subgroup analysis, VE of the first or second booster against hospitalizations with respiratory distress was 76.7% (95% CI: 51.4–88.8%) at
90 days, and 59.6% (95% CI: 16.2–80.5%) at 91 to 180 days; VE of the first or second booster against hospitalizations with moderate to severe diseases was 80.6% (95% CI: 64.0–89.6%) at
90 days, and 58.7% (95% CI: 23.1–77.8%) at 91 to 180 days; and VE of the first or second booster against hospitalizations with pneumonia was 85.3% (95% CI: 71.2–92.5%) at
90 days, and 70.5% (95% CI: 41.4–85.1%) at 91 to 180 days. The results for VE on subgroup analysis exhibited similar trends across the different types of vaccination status, and point estimates of VEs were slightly higher than VE against overall hospitalizations.
Figure 5. Vaccine effectiveness of monovalent mRNA COVID-19 vaccines against severe diseases among individuals aged 60 years, VERSUS study, Japan, between July 1 and November 30, 2022. The analysis included test-positive cases with signs or symptoms that tested positive for SARS-CoV-2, and test-negative controls with signs or symptoms that tested negative for SARS-CoV-2. Vaccine effectiveness was adjusted for age, sex, underlying medical conditions, calendar week of admission, prior SARS-CoV-2 infection history, and medical facilities. The vaccination status was classified into four based on the number of vaccine doses received before symptom onset and number of days between the last vaccination date and symptom onset: unvaccinated, individuals had received no vaccine dose before symptom onset; primary vaccination, individuals had received the second dose
14 days before symptom onset; 1st booster vaccination, individuals had received the third dose
14 days before symptom onset; and 2nd booster vaccination, individuals had received the fourth dose
14 days before symptom onset. Completion of vaccination was defined as 14 days after receiving the last vaccine. When evaluating vaccine effectiveness separated by days after vaccination, the number of days is shown from completion of vaccination. The following subgroup analyses were conducted: a subgroup with respiratory distress at the time of admission by limiting analysis to individuals with hypoxia (oxygen saturation<90%), oxygen administration, or tachypnea (respiratory rate≥30/minute); a subgroup with moderate or severe diseases at the time of admission defined CURB-65≥2; and a subgroup with radiologically confirmed pneumonia at the time of admission.
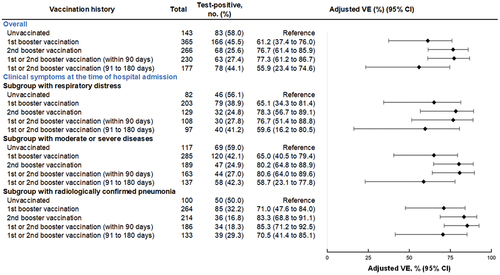
3.3. Sensitivity analysis for vaccine effectiveness and relative vaccine effectiveness
The sensitivity analysis results for VE and relative VE against symptomatic infections and VE against severe diseases are shown in Supplementary Figures S5, S6, and S7, respectively. The results are similar to those of the primary analysis.
4. Discussion
In this analysis, the effectiveness of primary vaccination of monovalent COVID-19 vaccines was low, and receipt of the first or second monovalent booster COVID-19 vaccination provided additional protection against symptomatic infections and high-level protection against severe diseases. The key study findings were as follows [Citation1]: for VE against symptomatic infections in individuals aged 16 to 59 years, VE of the primary vaccination at >180 days was 26.1% (95% CI: 10.6–38.8%) and VE of the first booster was 58.5% (95% CI: 48.4–66.7%) at 90 days, dropping to 41.1% (95% CI: 29.5–50.8%) at 91 to 180 days, and 31.1% (95% CI: 13.8–44.9%) at >180 days [Citation2]; for VE against symptomatic infections in individuals aged
60 years, VE of the first booster was 42.8% (95% CI: 1.7–66.7%) at
90 days, decreasing to 15.4% (95% CI: −25.9–43.2%) at 91 to 180 days, and then increasing to 44.0% (95% CI: 16.4–62.5%) after the second booster vaccination [Citation3]; for VE against hospitalizations in individuals aged
60 years, VE of the first or second booster was high at 77.3% (95% CI: 61.2–86.7%) at
90 days and 55.9% (95% CI: 23.4–74.6%) at 91 to 180 days [Citation4]; VE against hospitalizations was consistent with VE against hospitalizations on restricting to more severe conditions. Our results showed that receipt of a booster dose increased VE against symptomatic infections and provided high-level protection against severe diseases, but we detected signs of waning effectiveness of booster vaccination within several months of the last vaccination. These results show the importance of booster vaccination to protect against both mild and severe COVID-19, especially for older people at risk of severe COVID-19. They also indicate the limitations of monovalent vaccines, and highlight the need for additional booster vaccinations, including bivalent vaccines. Our study provides real-world evidence of the effectiveness of booster monovalent COVID-19 vaccination during the SARS-CoV-2 Omicron BA.5 epidemic period in Japan. Furthermore, our estimates of VE preventing hospitalizations are similar to those restricted to severe conditions, such as hospitalizations with respiratory distress. Therefore, we believe that we succeeded in evaluating how COVID-19 vaccines reduces hospitalization for patients who would otherwise medically require it.
Our VE estimates of the first or second booster dose against hospitalization were higher than those in the US but similar to those in Canada; IVY Network from the US reported that estimated VEs of boosters at 7 to 120 days were 60% (95% CI: 12 to 81%) for the first booster and 60% (36 to 75%) for the second booster among immunocompetent adults [Citation26]; a study from Canada found that VEs of the first and second boosters at 7 to 119 days were 74 to 94% and 80 to 88%, respectively, among adults aged 60 years [Citation27]. One of the possible reasons for the variation could be differences in definitions of outcomes. A study from the US defined COVID-19-associated hospitalization as hospitalization with one or more COVID-19-like illnesses; on the other hand, a study from Canada defined it as patients who received treatment for COVID-19 and/or if the length of hospital stay was extended due to COVID-19. The definitions of COVID-19-associated hospitalization used by the study in Canada and our study could be more severe than those in the US studies, leading to higher estimates [Citation23]. Regarding the waning effectiveness of booster vaccination against severe diseases, all four studies and a study from South Africa [Citation15] showed similar trends, revealing that the protection declined within several months after the last vaccination.
Evidence for VE of monovalent COVID-19 vaccines against symptomatic infections during the SARS-CoV-2 Omicron BA.5- predominant period has been limited. The protection afforded by vaccines against severe disease has tended to be a major target for the national COVID-19 vaccination policy. However, in Japan, many people with mild COVID-19-like symptoms have attended medical facilities to be tested or received medication. This has been a burden on the medical system and caused medical shortages, prompting a joint statement to the public on the effective use of limited medical resources by the four organizations of the Japanese Society of Medicines [Citation28]. Under such circumstances, it is still considered important for vaccination policy to evaluate VE against mild diseases and understand whether VE against a new emerging variant would be reduced or equivalent to VE against an existing strain. Studies showed that BA.5 increased evasion of vaccine-induced antibodies compared with BA.1 or BA.2 [Citation29,Citation30]. On the other hand, regarding real-world evidence of VE, a study from Denmark showed that vaccine protection against infection caused by BA.5 was similar to, or slightly weaker than, that against infection caused by BA.2 [Citation31], and a study from Portugal found that there was no reduced VE for primary or booster vaccination against infection caused by BA.5 compared with that against infection caused by BA.2 [Citation32]. In our study, compared with VE against symptomatic infections during the BA.1 or BA.2 epidemic period in Japan [Citation20], VE during the BA.5 period tended to be lower across the same time intervals after vaccination among individuals aged 16 to 64 years, although the 95% CIs overlapped (Supplementary Figure S4). A potential explanation for the differences from the previous two studies is that we may not have fully incorporated prior SARS-COV-2 infection history into the analysis, although prior SARS-COV-2 infection history and calendar time were included in the regression model. Previous infection caused by Omicron was reported to provide protection against infection caused by BA.5 [Citation33,Citation34]. VE would be underestimated if unvaccinated individuals were more likely to have infection-induced immunity, and estimates of VE could change depending on the percentage of individuals with infection-induced immunity in each country. A national survey in Japan found that the seroprevalence of prior SARS-CoV-2 infection among the younger population in Japan increased during the Omicron predominant period, from 2% in December 2021 (i.e. before Omicron predominance) to 4% in February 2022 (i.e. during BA.1 predominance), and 30 to 40% in November 2022. In our study, 4.5% of the population reported having a prior SARS-COV-2 infection history, which could be underreported judging from the national survey and possibly lead to lower estimation of VE against symptomatic infections during the BA.5 epidemic period.
Our VE against hospitalizations was similar to, or slightly lower than, VE against more severe outcomes. Evaluating VE against hospitalizations due to infection caused by Omicron using hospitalization as a measurement can be challenging because of the high prevalence of infection caused by Omicron and attenuated disease severity. SARS-CoV-2 infection can occur incidentally among patients admitted due to another disease [Citation11,Citation23]. To tackle this problem, we limited the study population who could be identified from medical charts to patients admitted with two or more symptoms of acute respiratory illness and/or radiologically confirmed pneumonia. VE against hospitalizations was similar to VE against hospitalization with severe conditions, and we believe that our study design was appropriate to successfully evaluate VE against COVID-19-associated hospitalizations.
This study had several limitations. First, recall bias may exist concerning COVID-19 vaccination histories. Neither medical professionals nor researchers have been permitted to access governmental vaccination records of patients in Japan. Therefore, studies conducted in the clinical fields in Japan must partly rely on vaccination histories disclosed by patients or their family members, while some vaccination histories could be confirmed by vaccination cards or records in medical charts. For these uncertainties about vaccination history, we conducted two sensitivity analyses (Supplementary Figures S5, S6, and S7), and the results were similar to those of the primary analysis, showing that our findings were robust. Second, since SARS-CoV-2 genome sequencing was not performed for test-positive patients, it was impossible to precisely assess VE against BA.5. Although most of the circulating SARS-CoV-2 variants were Omicron BA.5 during the study period, other SARS-CoV-2 variants could potentially have caused some of the episodes. Third, some patients have infection-induced immunity without recognizing it. Although we applied prior SARS-CoV-2 infection history and calendar week to the regression model as confounders, we may not have been able to control sufficiently for the immunity, which would have influenced the estimated VE. Fourth, we excluded episodes involving individuals with an unknown vaccination history. Due to an unknown COVID-19 vaccination history, 4% (463/11,209) and 22.3% (267/1,199) of the study population were excluded from the analysis of VE against symptomatic infections and severe diseases, respectively. In both analyses, individuals with an unknown vaccination history were more likely to be test-negative for SARS-CoV-2, which could affect the results. Fifth, the sample size for analysis of VE against symptomatic infections was limited for individuals aged 60 years compared with those aged 16 to 59 years. One of the possible reasons for the differences in the sample size by age group may be that the number of SARS-CoV-2 tests for individuals aged ≥60 years could have been initially lower than that for younger age groups nationwide. According to the national survey, the number of newly confirmed SARS-CoV-2 cases involving individuals aged 20 to 59 was 3.5 times higher than that ≥60 years during the study period [Citation35]. This number is similar to that obtained on the analysis of VE against symptomatic infections in our study, and we believe that the study population simply reflected this fact. Sixth, the possibility of misclassification of outcomes cannot be ruled out. Since SARS-CoV-2 testing methods do not show 100% sensitivity or specificity, there could be false-positive and false-negative test results. Seventh, we did not collect the date of prior SARS-CoV-2 infection. Even though we collected the information on the history of prior SARS-CoV-2 infection and included it in the regression model, we failed to take consider the time after the previous infection. Infection-induced immunity was reported to wane over time [Citation36,Citation37], and this would have affected the results. Eighth, the remaining unvaccinated population may potentially differ from the vaccinated population, possibly leading to residual confounding in the analysis of VE. Nineth, in the analysis of VE against symptomatic infections, test-positive individuals were more likely to be younger and less likely to one or more have underlying medical conditions (p < 0.001). Although we adjusted for patient characteristics in the regression model, there is a possibility that these differences affected VE estimations.
5. Conclusion
Our analyses indicated that the first or second mRNA booster provided moderate protection against symptomatic infections and high-level protection against severe diseases during the BA.5 epidemic period in Japan. However, the protection afforded may have declined within several months, and an additional booster may have been required. With the introduction of bivalent mRNA COVID-19 vaccines in Japan, it will be critical to continue to evaluate VE.
Declaration of interest
H Maeda reports lecture and consulting fees from Moderna Inc and participating in Pfizer Inc-funded research outside this work. AI reports grants from Takeda Pharmaceutical Inc, consulting fees from Pfizer Inc and Astra Zeneca Inc, and lecture fees from Takeda Pharmaceutical Inc, Shionogi Inc, Janssen Pharma Inc, and Moderna Inc outside this work. M Terada reports lecture fees from Astra Zeneca outside this work. H Inoue reports participating in research funded by Pfizer Inc, research funded by GENOVA Inc, research funded by Astra Zeneca Inc, and research funded by FUJIFILM Toyama Chemical CO., Ltd. outside this work. T Hayakawa reports stock ownership of Takeda Pharmaceutical Inc. and Daiichi Sankyo Healthcare Co., Ltd. K Morimoto reports grants from Pfizer Inc, consulting fees from Moderna Inc, and lecture fees from MSD outside this work. The authors have no other relevant affiliations or financial involvement with any organization or entity with a financial interest in or financial conflict with the subject matter or material discussed in the manuscript apart from those disclosed.
Reviewer disclosures
Peer reviewers on this manuscript have received honoraria for their review work. Peer reviewers on this manuscript have no other relevant financial or other relationships to disclose.
Abbreviations
Coronavirus disease 2019 (COVID-19); messenger RNA (mRNA); severe acute respiratory syndrome coronavirus 2 (SARS-CoV-2); polymerase chain reaction (PCR), loop-medical isothermal amplification (LAMP); nicking endonuclease amplification reaction (NEAR); transcription-mediated amplification (TMA); oxygen saturation (SpO2); Institutional Review Board (IRB); confidence interval (CI).
Author contributions
HM, NS, AI, MI, MT, SM, MS, and KM conceived and designed the study. MI, MT, RO, NH, KN, HK, HI, HI, SM, YS, OK, IM, TM, RO, YH, TM, OT, ES, SA, SK, NA, YM, TH, TK, and YO collected and verified the data. HM and NS did the analysis and MS and YK supervised the analysis. AI performed the analysis separately from HM and NS. HM, NS, AI, MI, MT, SM, MS, and KM had full access to the data. HM and KM wrote the first draft of the manuscript, and all authors provided critical review and revision of the text and approved the final version for publication.
Supplemental Material
Download PDF (1,008.5 KB)Acknowledgments
We are grateful to medical staff of the participating hospitals and clinics. We thank Yukie Kozone, Rina Kubo, Yumi Araki, Rina Shiramizu (Institute of Tropical Medicine, Nagasaki University), and Daichi Hatahara (Nagasaki University) for their assistance.
Supplementary material
Supplemental data for this article can be accessed online at https://doi.org/10.1080/14760584.2024.2310807.
Additional information
Funding
References
- Ministry of Health, Labour and Welfare. Asymptomatic carriers of SARS-COV-2 in airport quarantine. 2021 Nov 30 [cited 2023 Nov 20]. Available from: https://www.mhlw.go.jp/stf/newpage_22507.html
- Ministry of Health, Labour and Welfare. SARS-CoV-2 open data. [cited 2023 Nov 20]. Available from: https://www.mhlw.go.jp/stf/covid-19/open-data.html
- National Institute of Infectious Diseases. SARS-CoV-2 variants. [cited 2023 Nov 20]. Available from: https://www.niid.go.jp/niid/ja/2019-ncov/2624-flu/12054-flu2-1-2.html
- Ministry of Health, Labour and Welfare. Materials at the 32nd meeting of the subcommittee on immunization and vaccine of the health science council (held on April 27, 2022). [cited 2023 Nov 20.] Available from: https://www.mhlw.go.jp/stf/newpage_25379.html
- Polack FP, Thomas SJ, Kitchin N, et al. Safety and efficacy of the BNT162b2 mRNA COVID-19 vaccine. N Engl J Med. 2020;383(27):2603–2615. doi: 10.1056/NEJMoa2034577
- Corbett KS, Edwards DK, Leist SR, et al. SARS-CoV-2 mRNA vaccine design enabled by prototype pathogen preparedness. Nature. 2020;586(7830):567–571. doi: 10.1038/s41586-020-2622-0
- Jackson LA, Anderson EJ, Rouphael NG, et al. An mRNA Vaccine against SARS-CoV-2 — Preliminary Report. N Engl J Med. 2020;383(20):1920–1931. doi: 10.1056/NEJMoa2022483
- Pormohammad A, Zarei M, Ghorbani S, et al. Effectiveness of COVID-19 vaccines against Delta (B.1.617.2) variant: a systematic review and meta-analysis of clinical studies. Vaccines (Basel). 2021;10(1):23. doi: 10.3390/vaccines10010023
- Guo K, Ni P, Chang S, et al. Effectiveness of mRNA vaccine against omicron-related infections in the real world: a systematic review and meta-analysis. Am J Infect Control. 2023;51(9):1049–1055. doi: 10.1016/j.ajic.2023.02.005
- Maltezou HC, Basoulis D, Bonelis K, et al. Effectiveness of full (booster) COVID-19 vaccination against severe outcomes and work absenteeism in hospitalized patients with COVID-19 during the Delta and omicron waves in Greece. Vaccine. 2023;41(14):2343–2348. doi: 10.1016/j.vaccine.2023.01.067
- World Health Organization. Evaluation of COVID-19 vaccine effectiveness in a changing landscape of COVID-19 epidemiology and vaccination. [cited 2023 Nov 20]. Available from: https://www.who.int/publications/i/item/WHO-2019-nCoV-vaccine_effectiveness-VE_evaluations-2022.1
- Centers for Disease Control and Prevention. How CDC monitors vaccine effectiveness. [cited 2023 Dec 28]. Available from: https://www.cdc.gov/coronavirus/2019-ncov/vaccines/effectiveness/how-cdc-monitors.html
- National Institute of Infectious Diseases. COVID-19 vaccine (as of May 10, 2021). [cited 2023 Nov 20]. Availabel from: https://www.niid.go.jp/niid/images/vaccine/covid19_vaccine_20210510.pdf
- Tartof SY, Slezak JM, Puzniak L, et al. Durability of BNT162b2 vaccine against hospital and emergency department admissions due to the omicron and delta variants in a large health system in the USA: a test-negative case-control study. Lancet Respir Med. 2022;10:689–699. doi: 10.1016/S2213-2600(22)00101-1
- Collie S, Nayager J, Bamford L, et al. Effectiveness and durability of the BNT162b2 vaccine against omicron sublineages in South Africa. N Engl J Med. 2022;387(14):1332–1333. doi: 10.1056/NEJMc2210093
- Tseng HF, Ackerson BK, Luo Y, et al. Effectiveness of mRNA-1273 against SARS-CoV-2 omicron and Delta variants. Nat Med. 2022;28(5):1063–1071. doi: 10.1038/s41591-022-01753-y
- Ministry of Health, Labour and Welfare. Materials at the 33rd meeting of the subcommittee on immunization and vaccine of the health science council (held on July 22, 2022). [cited 2023 Nov 20]. Available from: https://www.mhlw.go.jp/content/10900000/000968057.pdf
- National Institute of Infectious Diseases. Information on COVID-19 vaccination. [cited 2023 Dec 27]. Availabel from: https://www.niid.go.jp/niid/images/vaccine/covid19_vaccine_20230123.pdf
- Maeda H, Saito N, Igarashi A, et al. Effectiveness of messenger RNA coronavirus disease 2019 vaccines against symptomatic severe acute respiratory syndrome coronavirus 2 infections during the delta variant epidemic in Japan: vaccine effectiveness real-time surveillance for SARS-CoV-2 (VERSUS). Clin Infect Dis. 2022;75(11):1971–1979. doi: 10.1093/cid/ciac292
- Maeda H, Saito N, Igarashi A, et al. Effectiveness of mRNA COVID-19 vaccines against symptomatic SARS-CoV-2 infections during the SARS-CoV-2 omicron BA.1 and BA.2 epidemic in Japan: vaccine effectiveness real-time surveillance for SARS-CoV-2 (VERSUS). Expert Rev Vaccines. 2023;22(1):288–298. doi: 10.1080/14760584.2023.2188950
- Takada T, Yamamoto Y, Terada K, et al. Diagnostic utility of appetite loss in addition to existing prediction models for community-acquired pneumonia in the elderly: a prospective diagnostic study in acute care hospitals in Japan. BMJ Open. 2017;7(11):e019155. doi: 10.1136/bmjopen-2017-019155
- Harris PA, Taylor R, Thielke R, et al. Research electronic data capture (REDCap)–a metadata-driven methodology and workflow process for providing translational research informatics support. J Biomed Inform. 2009;42:377–381. doi: 10.1016/j.jbi.2008.08.010
- Feikin DR, Abu-Raddad LJ, Andrews N, et al. Assessing vaccine effectiveness against severe COVID-19 disease caused by omicron variant. report from a meeting of the world health organization. Vaccine. 2022;40(26):3516–3527. doi: 10.1016/j.vaccine.2022.04.069
- Barlow G, Nathwani D, Davey P. The CURB65 pneumonia severity score outperforms generic sepsis and early warning scores in predicting mortality in community-acquired pneumonia. Thorax. 2007;62(3):253–259. doi: 10.1136/thx.2006.067371
- Government CIOS’ Portal, Japan. Vaccination status of COVID-19 vaccine (general population (including older adults)). [cited 2021 Dec 20]. Available from: https://cio.go.jp/c19vaccine_dashboard
- Surie D, Bonnell L, Adams K. Effectiveness of monovalent mRNA vaccines against COVID-19-Associated hospitalization among immunocompetent adults during BA.1/BA.2 and BA.4/BA.5 predominant periods of SARS-CoV-2 omicron variant in the United States - IVY network, 18 States, December 26, 2021-August 31, 2022. MMWR Morb Mortal Wkly Rep. 2022;71(42):1327–1334. doi: 10.15585/mmwr.mm7142a3
- Grewal R, Nguyen L, Buchan SA, et al. Effectiveness of mRNA COVID-19 vaccine booster doses against omicron severe outcomes. Nat Commun. 2023;14(1):1273. doi: 10.1038/s41467-023-36566-1
- The Japanese Assocition for Infectious Diseases, Japanese Association for Acute Medicine, Japan Primary Care Association, and Japanese Society for Emergency Medicine. Statement on attending medical facilities use of ambulances for effective utilization of limited medical resources. [cited 2023 Nov 20]. Available from: https://www.kansensho.or.jp/uploads/files/topics/2019ncov/covid19_4seimei_220803.pdf
- Hachmann NP, Miller J, Collier AY, et al. Neutralization Escape by SARS-CoV-2 Omicron Subvariants BA.2.12.1, BA.4, and BA.5. N Engl J Med. 2022;387(1):86–88. doi: 10.1056/NEJMc2206576
- Tuekprakhon A, Nutalai R, Dijokaite-Guraliuc A, et al. Antibody escape of SARS-CoV-2 omicron BA.4 and BA.5 from vaccine and BA.1 serum. Cell. 2022;185(14):2422–33.e13. doi: 10.1016/j.cell.2022.06.005
- Hansen CH, Friis NU, Bager P, et al. Risk of reinfection, vaccine protection, and severity of infection with the BA.5 omicron subvariant: a nationwide population-based study in Denmark. Lancet Infect Dis. 2023;23(2):167–176. doi: 10.1016/S1473-3099(22)00595-3
- Kislaya I, Casaca P, Borges V, et al. Comparative effectiveness of COVID-19 vaccines in preventing infections and disease progression from SARS-CoV-2 omicron BA.5 and BA.2, Portugal. Emerg Infect Dis. 2023;29(3):569–575. doi: 10.3201/eid2903.221367
- Altarawneh HN, Chemaitelly H, Ayoub HH, et al. Protective effect of previous SARS-CoV-2 infection against omicron BA.4 and BA.5 subvariants. N Engl J Med. 2022;387(17):1620–1622. doi: 10.1056/NEJMc2209306
- Tan CY, Chiew CJ, Pang D, et al. Protective immunity of SARS-CoV-2 infection and vaccines against medically attended symptomatic omicron BA.4, BA.5, and XBB reinfections in Singapore: a national cohort study. Lancet Infect Dis. 2023;23(7):799–805. doi: 10.1016/S1473-3099(23)00060-9
- Ministry of Health, Labour and Welfare. Visualizing the data: information on COVID-19 infections. [cited 2023 Nov 20]. Available from: https://covid19.mhlw.go.jp/en/
- Bobrovitz N, Ware H, Ma X, et al. Protective effectiveness of previous SARS-CoV-2 infection and hybrid immunity against the omicron variant and severe disease: a systematic review and meta-regression. Lancet Infect Dis. 2023;23(5):556–567. doi: 10.1016/S1473-3099(22)00801-5
- Chin ET, Leidner D, Lamson L, et al. Protection against omicron from vaccination and previous infection in a prison system. N Engl J Med. 2022;387(19):1770–1782. doi: 10.1056/NEJMoa2207082