Abstract
Objective. Pyelonephritis has a more severe course during pregnancy than in the non-pregnant state. This has been attributed to the increased susceptibility of pregnant women to microbial products. An acquired protein Z deficiency has been reported when there is excessive thrombin activity. The aim of this study was to determine whether pyelonephritis during pregnancy is associated with changes in maternal plasma protein Z concentrations.
Study design. A cross-sectional study was conducted to compare plasma protein Z concentrations between normal pregnant women (N = 71) and pregnant women with pyelonephritis (N = 42). Protein Z concentrations were measured by enzyme-linked immunosorbent assay. Parametric and non-parametric statistics were used for analysis.
Results. Patients with pyelonephritis had a significantly lower median plasma concentration of protein Z than did patients with normal pregnancies (median 2.14 μg/mL (0.4–3.4) vs. median 2.36 μg/mL (1.09–3.36); p = 0.03). There was no difference in the median plasma concentration of anti-protein Z antibodies between patients with pyelonephritis and those with normal pregnancies.
Conclusion. The median maternal plasma protein Z concentration was significantly lower in patients with pyelonephritis during pregnancy than in patients with normal pregnancies.
Introduction
Pyelonephritis is a common complication of pregnancy, affecting 1–2% of pregnancies, and is a frequent cause of antepartum hospitalization Citation[1],Citation[2]. Pyelonephritis during pregnancy generally has a good prognosis Citation[1],Citation[2]; however, it can be associated with severe maternal morbidity such as Gram-negative sepsis Citation[3-6], renal failure Citation[7], and acute respiratory distress syndrome Citation[8-12].
Infection can induce a systemic inflammatory response syndrome (SIRS) and activation of the coagulation cascade Citation[13-16] leading to multi-organ damage and failure. During systemic inflammation, activated monocytes express tissue factor on their membrane Citation[17-21], and higher tissue factor concentrations in plasma Citation[22] and bronchoalveolar lavage Citation[23] have been associated with the pathophysiology of acute lung injury complicating sepsis. The generation of thrombin leads to amplification of inflammatory processes Citation[24-30], which are, at least partially, mediated through the thrombin receptor, also known as protease-activated receptor (PAR). This receptor can be activated also by the complex of tissue factor, activated factor VII (FVIIa), and activated factor X (FXa) [31–35]. Activation of PAR receptors leads to the synthesis and secretion of inflammatory mediators (e.g., interleukin (IL)-6 and IL-8) by monocyte and endothelial cells Citation[36-45].
Exposure of tissue factor due to tissue damage leads to the formation of a complex between tissue factor and activated factor VII that activates factor X Citation[19], Citation[46-48]. Activated factor Xa generates thrombin from prothrombin, and thrombin cleaves fibrinogen into fibrin and activates platelets, leading to clot formation (,b) Citation[46],Citation[48-51].
Figure 1. Factor X activation and protein Z/protein Z-dependent protease inhibitor (ZPI) inhibition of activated factor X. (a) The formation of the complex of tissue factor (TF) and factor VIIa (FVIIa) at the site of injury and activation of extrinsic coagulation cascade. (b) Activation of circulating factor X by the TF + FVIIa complex in the presence of exposed phospholipids and Ca2+. (c) Inhibition of factor Xa (FXa) by the protein Z/ZPI complex by binding to its active site. Modified from Broze JG, Lancet 2001;357:900–901.
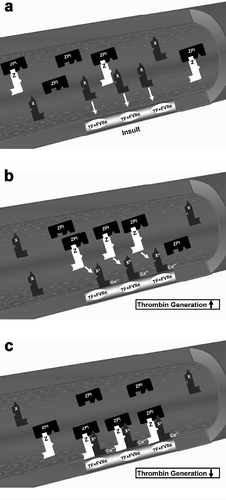
The coagulation cascade is tightly regulated, and the activation of prothrombin by factor Xa (FXa) is inhibited by antithrombin III, tissue factor pathway inhibitor Citation[50], and by the protein Z/protein Z-dependent protease inhibitor (ZPI) complex () Citation[52-54]. Protein Z is a vitamin K-dependent plasma glycoprotein Citation[55] that is an essential cofactor for ZPI activity. In the absence of protein Z, the activity of ZPI is reduced by more than 1000-fold Citation[54]. Patients with a factor V Leiden mutation and low protein Z plasma concentration have a higher risk for thrombosis than those with only a factor V Leiden mutation Citation[56],Citation[57]. Moreover, low plasma protein Z concentrations have been associated with acute coronary syndrome Citation[58], ischemic stroke Citation[59], and central retinal vein or artery occlusion Citation[60]; it has been proposed that protein Z deficiency is associated with a prothrombotic state Citation[58].
Low plasma protein Z concentrations have been reported in women with adverse pregnancy outcomes such as unexplained fetal loss Citation[61], intrauterine growth restriction (IUGR) Citation[62],Citation[63], vaginal bleeding Citation[63], preeclampsia Citation[63], and fetal demise Citation[62]. Moreover, women with complicated pregnancies (preeclampsia, IUGR, and fetal demise) have higher rates of low maternal plasma protein Z concentrations than patients with inherited thrombophilias (i.e., protein C deficiency, factor V Leiden mutations) and antiphospholipid antibodies Citation[62].
The aims of this study were to: (1) determine whether there are changes in maternal plasma concentrations of protein Z during an acute systemic inflammatory state (pyelonephritis), and (2) examine whether there is an association between low maternal plasma concentration of protein Z and pyelonephritis with associated complications.
Material and methods
A cross-sectional study was conducted in patients with (1) acute pyelonephritis (n = 42) and (2) uncomplicated pregnancies (n = 71). Patients with multiple pregnancies and with fetuses with congenital and chromosomal anomalies were excluded.
The study was conducted at the Detroit Medical Center/Wayne State University in Detroit, Michigan, USA. The utilization of samples for research purposes was approved by the institutional review boards of both the National Institute of Child Health and Human Development (Bethesda, MD, USA) and Wayne State University (Detroit, MI, USA). Patients provided written informed consent prior to enrollment in the study.
Clinical definitions
Pyelonephritis was diagnosed in the presence of the following criteria: (1) fever (temperature ≥38°C), (2) clinical signs of an upper urinary tract infection (e.g., back pain), (3) pyuria, and (4) a urine culture positive for microorganisms. Blood cultures were also performed in a subset of patients at the discretion of the treating physician. The control group consisted of pregnant women between 20 and 42 weeks of gestation who were not in labor and had no medical or obstetrical complications. Both groups were matched for gestational age. A small-for-gestational age (SGA) neonate was defined as having a birth weight below the 10th percentile Citation[64].
Blood samples collection
All blood samples were collected with a vacutainer into 0.109 M (3.2%) trisodium citrate anticoagulant solution (BD, San Jose, CA, USA). The samples were centrifuged at 1300 g for 10 minutes at 4°C and stored at −70°C until assay.
Human protein Z immunoassays
Concentrations of protein Z in maternal plasma were determined by sensitive and specific immunoassays obtained from Diagnostica Stago (Asnieres-sur-Seine, France). The assay was conducted according to the manufacturer's recommendations. The calculated inter- and intra-assay coefficients of variation (CVs) were 3.1% and 2.4%, respectively. The sensitivity of the protein Z immunoassay in our laboratory was 0.05 μg/mL.
Measurements of anti-protein Z IgG and IgM isotypes
Immunoassays to quantify anti-protein Z IgG and IgM isotypes were obtained from HYPHEN BioMed (Neuville-sur-Oise, France). The assays were conducted according to the manufacturer's recommendations. The concentrations of anti-protein Z IgG or IgM in samples were determined by interpolation from individual standard curves composed of purified human anti-protein Z IgG or IgM (calibrators). The calculated inter- and intra-assay CVs for anti-protein Z IgG isotype immunoassay in our laboratory were 6% and 5.4%, respectively. The detection limit (sensitivity) for the anti-protein Z IgG isotype immunoassay was 1.11 arbitrary units (AU)/mL. The calculated inter- and intra-assay CVs for anti-protein Z IgM isotype immunoassay were 7%, and 2.2%, respectively. The sensitivity for the anti-protein Z IgM isotype immunoassay was 2.02 AU/mL.
Statistical analysis
Shapiro–Wilk test was used to assess normality. Mann–Whitney U-test was used to compare medians, t-test was used to compare means, and Chi-square test and Fisher's exact test were used to compare proportions. A p value of <0.05 was considered statistically significant. Statistical analyses were preformed using SPSS, version 12 (SPSS Inc., Chicago, IL, USA).
Results
Demographic and clinical characteristics of the study groups are presented in . The mean birth weight was significantly lower in the pyelonephritis group than in the control group (3086 ± 584 g vs. 3340 ± 392 g, respectively, p = 0.02). The frequency of SGA in the pyelonephritis group was 12.2% (5/41).
Table I. Demographic and clinical characteristics of the study population.
In the control group, there was no correlation between gestational age at blood collection and protein Z plasma concentrations (r2 = 0.004, p = 0.6) (). The median maternal plasma concentration of protein Z was significantly lower in patients with pyelonephritis than in normal pregnant women (median 2.14 μg/mL (range 0.44–3.42) vs. median 2.36 μg/mL (range 1.09–3.36), p = 0.03) ().
Figure 2. Maternal plasma protein Z concentrations in patients with normal pregnancy (N = 71) according to gestational age.
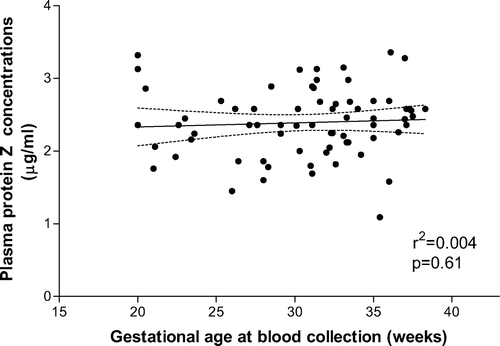
Figure 3. Maternal plasma protein Z concentrations in patients with normal pregnancy and patients with pyelonephritis. The median maternal plasma concentration of protein Z was significantly lower in patients with pyelonephritis than in normal pregnant women (2.14 μg/mL (range 0.44–3.42) vs. 2.36 μg/mL (range 1.09–3.36; p = 0.029).
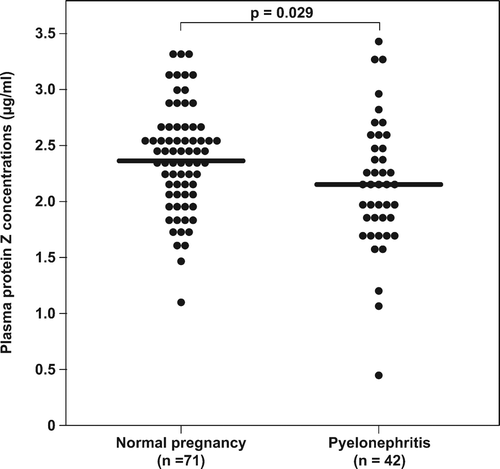
In contrast, the concentrations of anti-protein Z antibodies were not significantly different between the study groups (IgG, p = 0.12 and IgM, p = 0.76) (). In the pyelonephritis group, there was no difference in the median protein Z plasma concentration between who delivered an SGA neonate (median 2.21 μg/mL (range 1.89–3.25)) and those who delivered an appropriate for gestational age neonate (median 2.09 μg/mL (range 0.44–3.42); p = 0.307).
Table II. Plasma concentrations of anti-protein Z antibodies in both study groups.
Escherichia coli was the most frequent microorganism isolated in urine cultures (73.8% (31/42)). Blood cultures were obtained in most patients with pyelonephritis (88.1% (37/42)), and 45.9% (17/37) were positive for microorganisms. Gram-negative bacteria constituted 70.6% (12/17) of the pathogens in blood cultures, and E. coli was detected in 83.3% (10/12) of the Gram-negative organisms isolated. A higher proportion of patients with positive blood cultures had plasma protein Z concentrations below the 5th percentile of the control group (<1.59 μg/mL) when compared to patients with negative blood cultures (23% (4/17) vs. 0% (0/20), respectively; p = 0.04). However, there was no significant difference in the median plasma protein Z concentration between patients with pyelonephritis with positive and negative blood cultures (positive blood cultures: median 2.13 μg/mL (range 0.44–3.42) vs. negative blood cultures: median 2.20 μg/mL (range 1.69–3.30); p = 0.8).
Thirteen patients in the pyelonephritis group had a chest X-ray, and 38.5% (5/13) had radiological signs of pulmonary congestion or mild to moderate pulmonary edema; 53.8% (7/13) had a normal chest X-ray and one patient had chronic abnormal findings. Patients with abnormal chest X-ray results had a lower median plasma protein Z concentration than those with a normal chest X-ray; however, this difference was not statistically significant (abnormal chest X-ray: median 1.12 μg/mL (range 0.48–3.26) vs. normal chest X-ray: median 1.89 μg/mL (range 0.95–2.58); p = 0.34).
Discussion
Principal findings
(1) Women with pyelonephritis during pregnancy have a significantly lower median plasma concentration of protein Z than women with normal pregnancies; (2) this finding cannot be attributed to differences in maternal plasma concentrations of anti-protein Z antibodies; and (3) a higher proportion of patients with pyelonephritis and a positive blood culture had a low plasma protein Z concentration (below the 5th percentile) than patients with pyelonephritis and a negative blood culture.
Protein Z: A vitamin K-dependent cofactor with anticoagulant properties
Originally described in 1977 as a circulating protein in bovine plasma Citation[65], protein Z is a 62-kDa single chain glycoprotein Citation[66]. The designation of ‘Z’ referred to the fact that this was the last vitamin K-dependent protein to elute during anion exchange chromatography. Subsequently, this protein was found in humans in 1984 Citation[66]. This protein serves as a cofactor for the inhibition of factor Xa by another protein, which is called protein Z-dependent protease inhibitor (ZPI) Citation[52-54]. ZPI is a member of the serpin superfamily of proteinase inhibitors Citation[53]. Importantly, ZPI can inhibit other coagulation factors, such as XIa in the absence of protein Z Citation[54],Citation[67].
The major source of protein Z is thought to be the liver, similarly to other vitamin K-dependent factors and patients with liver disease and newborns have lower concentrations of this protein in their plasma Citation[68],Citation[69]. However, other sources are possible. We have demonstrated immunoreactivity to protein Z in the human placenta (Romero, Broze, Kim, unpublished observations). Patients receiving oral contraceptives have higher plasma concentrations of protein Z Citation[70]. There is a controversy concerning the changes in protein Z plasma concentration between pregnant and non-pregnant women Citation[62],Citation[63],Citation[71],Citation[72]. The plasma concentrations of protein Z in normal individuals vary widely and this appears to be under genetic control Citation[73-75]. The same is the case for ZPI Citation[70].
The precise functions of protein Z and ZPI remain controversial. However, accumulating evidence suggests that this complex inhibits, retards, and reduces thrombin generation through inhibition of factor X activity Citation[52-54]. Protein Z deficiency has been associated with a moderate risk for thrombosis Citation[58] and this risk is magnified when protein Z deficiency is associated with a thrombophilic state Citation[56],Citation[57]. Of interest is that some investigators have postulated that protein Z deficiency may predispose to hemorrhage Citation[76]. However, these observations have not been replicated Citation[77].
Protein Z deficiency has been associated with adverse pregnancy outcomes: (1) a higher proportion of patients with a previous fetal death (10–15 weeks of gestation) had protein Z deficiency (<1 mg/L) compared to the control group Citation[61], and (2) patients with adverse pregnancy outcome (including preeclampsia, IUGR, recurrent unexplained vaginal bleeding, and preterm parturition) had a significantly lower mean plasma concentration of protein Z than patients with normal pregnancy outcome. The authors proposed that changes in the maternal plasma protein Z concentration may have an important role in the regulation of thrombin generation during pregnancy Citation[63]. This is relevant because excessive thrombin generation is associated with preterm parturition with intact or ruptured membranes Citation[78], preeclampsia, and SGA fetuses Citation[79]. In contrast, a recent study Citation[62] demonstrated that the median plasma concentration of protein Z in patients with preeclampsia, IUGR, and late fetal demise (IUFD) was not significantly different than that of patients with normal pregnancy. However, the authors reported a higher frequency of protein Z deficiency (<1.2 mg/L) among patients with IUFD and IUGR compared to those with normal pregnancy Citation[62].
Pyelonephritis, systemic inflammation, and the coagulation system during pregnancy
Patients with pyelonephritis during pregnancy have phenotypic and metabolic changes in granulocytes and monocytes which are consistent with an exaggerated maternal systemic intravascular inflammation Citation[80]. Systemic inflammation is associated with activation of the hemostatic system Citation[14],Citation[81],Citation[82], particularly among patients with sepsis Citation[22],Citation[42],Citation[83-86]. Three mechanisms have been proposed to explain this and are detailed below.
Activation of the extrinsic coagulation pathway by inflammation
Proinflammatory cytokines such as IL-1β and tumor necrosis factor-α increase mRNA and protein expression of tissue factor by monocytes Citation[18-22],Citation[87-89] and macrophages Citation[90]. Increased bioavailability of tissue factor could lead to generation of the complex of tissue factor/FVIIa/FXa, which, in turn, generates thrombin and activates the protease-activated receptor, PAR-2. The latter enhances the production of IL-6 Citation[36],Citation[45],Citation[82],Citation[91]. Thus, an amplification loop between inflammation and the coagulation system is in place. For a more detailed account of the close relationship and the importance of the link between inflammation and coagulation, the reader is referred to recent reviews of this subject Citation[14],Citation[82],Citation[90],Citation[92]. This interaction is particularly important in obstetrics because placentas often have mixture of thrombotic and inflammatory Citation[93],Citation[94]. Evidence in support of this concept includes the observation that administration of antibodies against the binding site of FXa to tissue factor/FVIIa complexes attenuates tissue damage and thrombosis in baboons with E. coli-induced sepsis Citation[95]. The proposed mechanism of action is that the complex tissue factor/FVIIa cannot activate PAR-2 without the contribution of factor Xa Citation[95]. Thus, during systemic inflammation FXa plays a dual role: (1) activation of the prothrombinase complex leading to the generation of thrombin, and (2) amplification of the inflammatory process by inducing IL-6 secretion from endothelial cells Citation[96],Citation[97] and monocytes Citation[36],Citation[91],Citation[95]. As demonstrated in a previous study Citation[95], inhibition of factor Xa reduces the inflammatory and thrombotic complications of systemic inflammation during sepsis. Patients in the intensive care unit who developed acute respiratory distress syndrome had higher plasma concentrations of tissue factor but not tissue factor pathway inhibitor in comparison to intensive care unit patients who did not develop acute respiratory distress syndrome Citation[22]. This observation suggests that the lung injury observed during systemic inflammation may result from a failure to maintain an adequate balance between the activated proteases of the coagulation cascade (i.e., tissue factor, FXa) and their natural inhibitors (i.e., tissue factor pathway inhibitor). One possibility is that the low maternal protein Z plasma concentrations observed in patients with pyelonephritis are due to the increased consumption of protein Z resulting from the increased activation of FX during systemic inflammation Citation[98].
Activation of the extrinsic coagulation pathway through the complement system
Normal pregnancy is characterized by complement activation Citation[99] and we have proposed that this phenomenon may be a compensatory mechanism aimed at protecting the host against infection Citation[99]. Pregnant patients with pyelonephritis have significantly higher concentrations of the complement protein, C5a, than those without pyelonephritis Citation[100]. C5a induces a 4.9-fold increase in tissue factor activity and a 3.75-fold increase in tissue factor mRNA expression by endothelial cells Citation[101]. Moreover, administration of C5a to animals increases 5- to 6-fold the procoagulant activity of alveolar macrophages through tissue factor activation Citation[102]. Collectively, these observations suggest that complement activation in pyelonephritis contributes to the activation of the coagulation cascade.
Inhibition of the anticoagulation mechanisms
During severe systemic inflammation, the physiologic anticoagulant pathways are down-regulated Citation[82],Citation[92]. The plasma concentration of anti-thrombin III is markedly decreased Citation[103,104], and protein C activity is impaired Citation[103],Citation[105-107], resulting in decreased anticoagulant activity that further increases the hypercoagulable state observed during systemic inflammation.
A potential role for protein Z in acute maternal systemic inflammation during pregnancy
The lower median concentration of protein Z observed in patients with pyelonephritis during pregnancy is novel. One explanation for this finding is that the prothrombotic state of normal pregnancy Citation[108-112], associated with increased generation of factor Xa, is exacerbated during the course of acute infection/inflammation. The complex of protein Z and ZPI participates in counteracting the effects of an excess of factor Xa and may be consumed in this context. It is important to note that in vitro experiments have reported consumption of ZPI but not of protein Z Citation[54]. However, because protein Z circulates bound to ZPI, immunoreactive protein Z determinations reflect not only protein Z but also the concentration of protein Z/ZPI complexes Citation[67]. Therefore, the lower median plasma concentration of protein Z may reflect decreased circulating concentrations of this complex. The extent to which this phenomenon occurs in the non-pregnant state requires further investigation.
In conclusion, patients with pyelonephritis during pregnancy have a lower median maternal plasma concentration of protein Z than normal pregnant women.
Declaration of interest: The authors report no conflicts of interest. The authors alone are responsible for the content and writing of the paper.
References
- Gilstrap L C III, Cunningham F G, Whalley P J. Acute pyelonephritis in pregnancy: An anterospective study. Obstet Gynecol 1981; 57: 409–413
- Wing D A. Pyelonephritis. Clin Obstet Gynecol 1998; 41: 515–526
- Bubeck R W. Acute pyelonephritis during pregnancy with anuria, septicemia and thrombocytopenia. Del Med J 1968; 40: 143–147
- Cunningham F G, Morris G B, Mickal A. Acute pyelonephritis of pregnancy: A clinical review. Obstet Gynecol 1973; 42: 112–117
- Dilworth E E, Ward J V. Bacteremic shock in pyelonephritis and criminal abortion. Obstet Gynecol 1961; 17: 160–167
- Mabie W C, Barton J R, Sibai B. Septic shock in pregnancy. Obstet Gynecol 1997; 90: 553–561
- Ventura J E, Villa M, Mizraji R, Ferreiros R. Acute renal failure in pregnancy. Ren Fail 1997; 19: 217–220
- Pruett K, Faro S. Pyelonephritis associated with respiratory distress. Obstet Gynecol 1987; 69: 444–446
- Cunningham F G, Lucas M J, Hankins G D. Pulmonary injury complicating antepartum pyelonephritis. Am J Obstet Gynecol 1987; 156: 797–807
- Cunningham F G, Lucas M J. Urinary tract infections complicating pregnancy. Baillieres Clin Obstet Gynaecol 1994; 8: 353–373
- Catanzarite V A, Willms D. Adult respiratory distress syndrome in pregnancy: Report of three cases and review of the literature. Obstet Gynecol Surv 1997; 52: 381–392
- Hill J B, Sheffield J S, McIntire D D, Wendel G D, Jr. Acute pyelonephritis in pregnancy. Obstet Gynecol 2005; 105: 18–23
- Gando S, Nanzaki S, Sasaki S, Aoi K, Kemmotsu O. Activation of the extrinsic coagulation pathway in patients with severe sepsis and septic shock. Crit Care Med 1998; 26: 2005–2009
- Esmon C T, Fukudome K, Mather T, Bode W, Regan L M, Stearns-Kurosawa D J, Kurosawa S. Inflammation, sepsis, and coagulation. Haematologica 1999; 84: 254–259
- Fernandez-Perez E R, Salman S, Pendem S, Farmer J C. Sepsis during pregnancy. Crit Care Med 2005; 33: S286–293
- Corrigan J J, Jr, Ray W L, May N. Changes in the blood coagulation system associated with septicemia. N Engl J Med 1968; 279: 851–856
- Hetland O, Brovold A B, Holme R, Gaudernack G, Prydz H. Thromboplastin (tissue factor) in plasma membranes of human monocytes. Biochem J 1985; 228: 735–743
- Almdahl S M, Osterud B. Experimental Gram-negative septicemia: Thromboplastin generation in mononuclear phagocytes from different anatomical sites. Thromb Res 1987; 47: 37–46
- Salgado A, Boveda J L, Monasterio J, Segura R M, Mourelle M, Gomez-Jimenez J, Peracaula R. Inflammatory mediators and their influence on haemostasis. Haemostasis 1994; 24: 132–138
- Semeraro N, Colucci M. Tissue factor in health and disease. Thromb Haemost 1997; 78: 759–764
- Bouwman J J, Visseren F L, Bosch M C, Bouter K P, Diepersloot R J. Procoagulant and inflammatory response of virus-infected monocytes. Eur J Clin Invest 2002; 32: 759–766
- Gando S, Kameue T, Matsuda N, Hayakawa M, Morimoto Y, Ishitani T, Kemmotsu O. Imbalances between the levels of tissue factor and tissue factor pathway inhibitor in ARDS patients. Thromb Res 2003; 109: 119–124
- Idell S, James K K, Levin E G, Schwartz B S, Manchanda N, Maunder R J, Martin T R, McLarty J, Fair D S. Local abnormalities in coagulation and fibrinolytic pathways predispose to alveolar fibrin deposition in the adult respiratory distress syndrome. J Clin Invest 1989; 84: 695–705
- Bar-Shavit R, Kahn A, Wilner G D, Fenton J W. Monocyte chemotaxis: Stimulation by specific exosite region in thrombin. Science 1983; 220: 728–731
- Bizios R, Lai L, Fenton J W, Malik A B. Thrombin-induced chemotaxis and aggregation of neutrophils. J Cell Physiol 1986; 128: 485–490
- Sonne O. The specific binding of thrombin to human polymorphonuclear leucocytes. Scand J Clin Lab Invest 1988; 48: 831–838
- Drake W T, Issekutz A C. A role for alpha-thrombin in polymorphonuclear leukocyte recruitment during inflammation. Semin Thromb Hemost 1992; 18: 333–340
- Drake W T, Lopes N N, Fenton J W, Issekutz A C. Thrombin enhancement of interleukin-1 and tumor necrosis factor-alpha induced polymorphonuclear leukocyte migration. Lab Invest 1992; 67: 617–627
- Naldini A, Carney D H, Bocci V, Klimpel K D, Asuncion M, Soares L E, Klimpel G R. Thrombin enhances T cell proliferative responses and cytokine production. Cell Immunol 1993; 147: 367–377
- Franchini M, Veneri D, Lippi G. Inflammation and hemostasis: A bidirectional interaction. Clin Lab 2007; 53: 63–67
- Dery O, Corvera C U, Steinhoff M, Bunnett N W. Proteinase-activated receptors: Novel mechanisms of signaling by serine proteases. Am J Physiol 1998; 274: C1429–1452
- Coughlin S R. Protease-activated receptors and platelet function. Thromb Haemost 1999; 82: 353–356
- Coughlin S R. Thrombin signalling and protease-activated receptors. Nature 2000; 407: 258–264
- Coughlin S R. Protease-activated receptors in vascular biology. Thromb Haemost 2001; 86: 298–307
- Landis R C. Protease activated receptors: Clinical relevance to hemostasis and inflammation. Hematol Oncol Clin North Am 2007; 21: 103–113
- Camerer E, Huang W, Coughlin S R. Tissue factor- and factor X-dependent activation of protease-activated receptor 2 by factor VIIa. Proc Natl Acad Sci U S A 2000; 97: 5255–5260
- Taylor F B, Chang A C, Peer G, Li A, Ezban M, Hedner U. Active site inhibited factor VIIa (DEGR VIIa) attenuates the coagulant and interleukin-6 and -8, but not tumor necrosis factor, responses of the baboon to LD100 Escherichia coli. Blood 1998; 91: 1609–1615
- Cunningham M A, Romas P, Hutchinson P, Holdsworth S R, Tipping P G. Tissue factor and factor VIIa receptor/ligand interactions induce proinflammatory effects in macrophages. Blood 1999; 94: 3413–3420
- Siegbahn A. Cellular consequences upon factor VIIa binding to tissue factor. Haemostasis 2000; 30(Suppl 2)41–47
- Asokananthan N, Graham P T, Fink J, Knight D A, Bakker A J, McWilliam A S, Thompson P J, Stewart G A. Activation of protease-activated receptor (PAR)- 1, PAR-2, and PAR-4 stimulates IL-6, IL-8, and prostaglandin E2 release from human respiratory epithelial cells. J Immunol 2002; 168: 3577–3585
- Ruf W, Riewald M. Tissue factor-dependent coagulation protease signaling in acute lung injury. Crit Care Med 2003; 31: S231–237
- Carraway M S, Welty-Wolf K E, Miller D L, Ortel T L, Idell S, Ghio A J, Petersen L C, Piantadosi C A. Blockade of tissue factor: Treatment for organ injury in established sepsis. Am J Respir Crit Care Med 2003; 167: 1200–1209
- Hjortoe G M, Petersen L C, Albrektsen T, Sorensen B B, Norby P L, Mandal S K, Pendurthi U R, Rao L V. Tissue factor–factor VIIa-specific up-regulation of IL-8 expression in MDA-MB-231 cells is mediated by PAR-2 and results in increased cell migration. Blood 2004; 103: 3029–3037
- Chu A J. Role of tissue factor in thrombosis. Coagulation–inflammation–thrombosis circuit. Front Biosci 2006; 11: 256–271
- Chi L, Li Y, Stehno-Bittel L, Gao J, Morrison D C, Stechschulte D J, Dileepan K N. Interleukin-6 production by endothelial cells via stimulation of protease-activated receptors is amplified by endotoxin and tumor necrosis factor-alpha. J Interferon Cytokine Res 2001; 21: 231–240
- Camerer E, Kolsto A B, Prydz H. Cell biology of tissue factor, the principal initiator of blood coagulation. Thromb Res 1996; 81: 1–41
- Rao L V, Rapaport S I. Activation of factor VII bound to tissue factor: A key early step in the tissue factor pathway of blood coagulation. Proc Natl Acad Sci U S A 1988; 85: 6687–6691
- ten Cate H, Bauer K A, Levi M, Edgington T S, Sublett R D, Barzegar S, Kass B L, Rosenberg R D. The activation of factor X and prothrombin by recombinant factor VIIa in vivo is mediated by tissue factor. J Clin Invest 1993; 92: 1207–1212
- Rodgers G M, Shuman M A. Prothrombin is activated on vascular endothelial cells by factor Xa and calcium. Proc Natl Acad Sci U S A 1983; 80: 7001–7005
- Butenas S, Mann K G. Blood coagulation. Biochemistry (Mosc.) 2002; 67: 3–12
- Lu G, Broze G J, Jr, Krishnaswamy S. Formation of factors IXa and Xa by the extrinsic pathway: Differential regulation by tissue factor pathway inhibitor and antithrombin III. J Biol Chem 2004; 279: 17 241–17 249
- Han X, Fiehler R, Broze G J, Jr. Isolation of a protein Z-dependent plasma protease inhibitor. Proc Natl Acad Sci U S A 1998; 95: 9250–9255
- Han X, Huang Z F, Fiehler R, Broze G J, Jr. The protein Z-dependent protease inhibitor is a serpin. Biochemistry 1999; 38: 11 073–11 078
- Han X, Fiehler R, Broze G J, Jr. Characterization of the protein Z-dependent protease inhibitor. Blood 2000; 96: 3049–3055
- Yin Z F, Huang Z F, Cui J, Fiehler R, Lasky N, Ginsburg D, Broze G J, Jr. Prothrombotic phenotype of protein Z deficiency. Proc Natl Acad Sci U S A 2000; 97: 6734–6738
- Kemkes-Matthes B, Nees M, Kuhnel G, Matzdorff A, Matthes K J. Protein Z influences the prothrombotic phenotype in factor V Leiden patients. Thromb Res 2002; 106: 183–185
- Martinelli I, Razzari C, Biguzzi E, Bucciarelli P, Mannucci P M. Low levels of protein Z and the risk of venous thromboembolism. J Thromb Haemost 2005; 3: 2817–2819
- Fedi S, Sofi F, Brogi D, Tellini I, Cesari F, Sestini I, Gazzini A, Comeglio M, Abbate R, Gensini G F. Low protein Z plasma levels are independently associated with acute coronary syndromes. Thromb Haemost 2003; 90: 1173–1178
- Vasse M, Guegan-Massardier E, Borg J Y, Woimant F, Soria C. Frequency of protein Z deficiency in patients with ischaemic stroke. Lancet 2001; 357: 933–934
- Koren-Michowitz M, Eting E, Rahimi-Levene N, Garach-Jehoshua O, Volcheck Y, Kornberg A. Protein Z levels and central retinal vein or artery occlusion. Eur J Haematol 2005; 75: 401–405
- Gris J C, Quere I, Dechaud H, Mercier E, Pincon C, Hoffet M, Vasse M, Mares P. High frequency of protein Z deficiency in patients with unexplained early fetal loss. Blood 2002; 99: 2606–2608
- Bretelle F, Arnoux D, Shojai R, D'Ercole C, Sampol J, Dignat F, Camoin-Jau L. Protein Z in patients with pregnancy complications. Am J Obstet Gynecol 2005; 193: 1698–1702
- Paidas M J, Ku D H, Lee M J, Manish S, Thurston A, Lockwood C J, Arkel Y S. Protein Z, protein S levels are lower in patients with thrombophilia and subsequent pregnancy complications. J Thromb Haemost 2005; 3: 497–501
- Alexander G R, Himes J H, Kaufman R B, Mor J, Kogan M. A United States national reference for fetal growth. Obstet Gynecol 1996; 87: 163–168
- Prowse C V, Esnouf M P. The isolation of a new warfarin-sensitive protein from bovine plasma. Biochem Soc Trans 1977; 5: 255–256
- Broze G J, Jr, Miletich J P. Human protein Z. J Clin Invest 1984; 73: 933–938
- Tabatabai A, Fiehler R, Broze G J, Jr. Protein Z circulates in plasma in a complex with protein Z-dependent protease inhibitor. Thromb Haemost 2001; 85: 655–660
- Kemkes-Matthes B, Preissner K T, Langenscheidt F, Matthes K J, Muller-Berghaus G. S protein/vitronectin in chronic liver diseases: Correlations with serum cholinesterase, coagulation factor X and complement component C3. Eur J Haematol 1987; 39: 161–165
- Yurdakok M, Gurakan B, Ozbag E, Vigit S, Dundar S, Kirazli S. Plasma protein Z levels in healthy newborn infants. Am J Hematol 1995; 48: 206–207
- Al-Shanqeeti A, van Hylckmama V, Berntorp E, Rosendaal F R, Broze G J, Jr. Protein Z and protein Z-dependent protease inhibitor. Determinants of levels and risk of venous thrombosis. Thromb Haemost 2005; 93: 411–413
- Quack Loetscher K C, Stiller R, Roos M, Zimmermann R. Protein Z in normal pregnancy. Thromb Haemost 2005; 93: 706–709
- Kusanovic J P, Espinoza J, Romero R, Hoppensteadt D, Nien J K, Kim C J, Erez O, Soto E, Fareed J, Edwin S, et al. Plasma protein Z concentrations in pregnant women with idiopathic intrauterine bleeding and in women with spontaneous preterm labor. J Matern Fetal Med 2007; 20(6)453–463
- Lichy C, Kropp S, Dong-Si T, Genius J, Dolan T, Hampe T, Stoll F, Reuner K, Grond-Ginsbach C, Grau A. A common polymorphism of the protein Z gene is associated with protein Z plasma levels and with risk of cerebral ischemia in the young. Stroke 2004; 35: 40–45
- Miletich J P, Broze G J, Jr. Human plasma protein Z antigen: Range in normal subjects and effect of warfarin therapy. Blood 1987; 69: 1580–1586
- Vossen C Y, Hasstedt S J, Rosendaal F R, Callas P W, Bauer K A, Broze G J, Hoogendoorn H, Long G L, Scott B T, Bovill E G. Heritability of plasma concentrations of clotting factors and measures of a prethrombotic state in a protein C-deficient family. J Thromb Haemost 2004; 2: 242–247
- Kemkes-Matthes B, Matthes K J. Protein Z deficiency: A new cause of bleeding tendency. Thromb Res 1995; 79: 49–55
- Gamba G, Bertolino G, Montani N, Spedini P, Balduini C L. Bleeding tendency of unknown origin and protein Z levels. Thromb Res 1998; 90: 291–295
- Chaiworapongsa T, Espinoza J, Yoshimatsu J, Kim Y M, Bujold E, Edwin S, Yoon B H, Romero R. Activation of coagulation system in preterm labor and preterm premature rupture of membranes. J Matern Fetal Neonatal Med 2002; 11: 368–373
- Chaiworapongsa T, Yoshimatsu J, Espinoza J, Kim Y M, Berman S, Edwin S, Yoon B H, Romero R. Evidence of in vivo generation of thrombin in patients with small-for-gestational-age fetuses and pre-eclampsia. J Matern Fetal Neonatal Med 2002; 11: 362–367
- Naccasha N, Gervasi M T, Chaiworapongsa T, Berman S, Yoon B H, Maymon E, Romero R. Phenotypic and metabolic characteristics of monocytes and granulocytes in normal pregnancy and maternal infection. Am J Obstet Gynecol 2001; 185: 1118–1123
- Levi M, van der Poll T, Buller H R. Bidirectional relation between inflammation and coagulation. Circulation 2004; 109: 2698–2704
- Levi M, van der Poll T. Two-way interactions between inflammation and coagulation. Trends Cardiovasc Med 2005; 15: 254–259
- Cavanagh D, Rao P S. Septic shock (endotoxic shock). Clin Obstet Gynecol 1973; 16: 25–39
- Ozge-Anwar A H, Freedman J J, Senyi A F, Cerskus A L, Blajchman M A. Enhanced prothrombin-converting activity and factor Xa binding of platelets activated by the alternative complement pathway. Br J Haematol 1984; 57: 221–228
- Pawlinski R, Mackman N. Tissue factor, coagulation proteases, and protease-activated receptors in endotoxemia and sepsis. Crit Care Med 2004; 32: S293–297
- Mackman N. Role of tissue factor in hemostasis, thrombosis, and vascular development. Arterioscler Thromb Vasc Biol 2004; 24: 1015–1022
- Semeraro N, Lattanzio A, Montemurro P, Papanice M, De Lucia O, De Bellis G, Giordano D. Mechanisms of blood clotting activation in inflammation: The role of mononuclear phagocytes. Int J Tissue React 1985; 7: 313–320
- Oeth P, Parry G C, Mackman N. Regulation of the tissue factor gene in human monocytic cells. Role of AP-1, NF-kappa B/Rel, and Sp1 proteins in uninduced and lipopolysaccharide-induced expression. Arterioscler Thromb Vasc Biol 1997; 17: 365–374
- Heyderman R S, Klein N J, Daramola O A, Hammerschmidt S, Frosch M, Robertson B D, Levin M, Ison C A. Induction of human endothelial tissue factor expression by Neisseria meningitidis: The influence of bacterial killing and adherence to the endothelium. Microb Pathog 1997; 22: 265–274
- Levi M, van der Poll T, ten Cate H. Tissue factor in infection and severe inflammation. Semin Thromb Hemost 2006; 32: 33–39
- Ahamed J, Ruf W. Protease-activated receptor 2-dependent phosphorylation of the tissue factor cytoplasmic domain. J Biol Chem 2004; 279: 23 038–23 044
- Esmon C T. The interactions between inflammation and coagulation. Br J Haematol 2005; 131: 417–430
- Arias F, Rodriquez L, Rayne S C, Kraus F T. Maternal placental vasculopathy and infection: Two distinct subgroups among patients with preterm labor and preterm ruptured membranes. Am J Obstet Gynecol 1993; 168: 585–591
- Arias F, Victoria A, Cho K, Kraus F. Placental histology and clinical characteristics of patients with preterm premature rupture of membranes. Obstet Gynecol 1997; 89: 265–271
- Welty-Wolf K E, Carraway M S, Ortel T L, Ghio A J, Idell S, Egan J, Zhu X, Jiao J A, Wong H C, Piantadosi C A. Blockade of tissue factor–factor X binding attenuates sepsis-induced respiratory and renal failure. Am J Physiol Lung Cell Mol Physiol 2006; 290: L21–31
- Busch G, Seitz I, Steppich B, Hess S, Eckl R, Schomig A, Ott I. Coagulation factor Xa stimulates interleukin-8 release in endothelial cells and mononuclear leukocytes: Implications in acute myocardial infarction. Arterioscler Thromb Vasc Biol 2005; 25: 461–466
- Daubie V, Cauwenberghs S, Senden N H, Pochet R, Lindhout T, Buurman W A, Heemskerk J W. Factor Xa and thrombin evoke additive calcium and proinflammatory responses in endothelial cells subjected to coagulation. Biochim Biophys Acta 2006; 1763(8)860–869
- Kemkes-Matthes B, Matthes K J. Protein Z. Semin Thromb Hemost 2001; 27: 551–556
- Richani K, Soto E, Romero R, Espinoza J, Chaiworapongsa T, Nien J K, Edwin S, Kim Y M, Hong J S, Mazor M. Normal pregnancy is characterized by systemic activation of the complement system. J Matern Fetal Neonatal Med 2005; 17: 239–245
- Soto E, Richani K, Romero R, Espinoza J, Chaiworapongsa T, Nien J K, Edwin S, Kim Y M, Hong J S, Goncalves L, et al. Increased concentration of the complement split product C5a in acute pyelonephritis during pregnancy. J Matern Fetal Neonatal Med 2005; 17: 247–252
- Ikeda K, Nagasawa K, Horiuchi T, Tsuru T, Nishizaka H, Niho Y. C5a induces tissue factor activity on endothelial cells. Thromb Haemost 1997; 77: 394–398
- Sitrin R G, Kaltreider H B, Ansfield M J, Webster R O. Procoagulant activity of rabbit alveolar macrophages. Am Rev Respir Dis 1983; 128: 282–287
- White B, Perry D. Acquired antithrombin deficiency in sepsis. Br J Haematol 2001; 112: 26–31
- Martinez M A, Pena J M, Fernandez A, Jimenez M, Juarez S, Madero R, Vazquez J J. Time course and prognostic significance of hemostatic changes in sepsis: Relation to tumor necrosis factor-alpha. Crit Care Med 1999; 27: 1303–1308
- Nawroth P P, Stern D M. Modulation of endothelial cell hemostatic properties by tumor necrosis factor. J Exp Med 1986; 163: 740–745
- Eckle I, Seitz R, Egbring R, Kolb G, Havemann K. Protein C degradation in vitro by neutrophil elastase. Biol Chem Hoppe Seyler 1991; 372: 1007–1013
- Madden R M, Ward M, Marlar R A. Protein C activity levels in endotoxin-induced disseminated intravascular coagulation in a dog model. Thromb Res 1989; 55: 297–307
- de Boer K, ten Cate J W, Sturk A, Borm J J, Treffers P E. Enhanced thrombin generation in normal and hypertensive pregnancy. Am J Obstet Gynecol 1989; 160: 95–100
- Yuen P M, Yin J A, Lao T T. Fibrinopeptide A levels in maternal and newborn plasma. Eur J Obstet Gynecol Reprod Biol 1989; 30: 239–244
- Sorensen J D, Secher N J, Jespersen J. Perturbed (procoagulant) endothelium and deviations within the fibrinolytic system during the third trimester of normal pregnancy. A possible link to placental function. Acta Obstet Gynecol Scand 1995; 74: 257–261
- Walker M C, Garner P R, Keely E J, Rock G A, Reis M D. Changes in activated protein C resistance during normal pregnancy. Am J Obstet Gynecol 1997; 177: 162–169
- Bellart J, Gilabert R, Miralles R M, Monasterio J, Cabero L. Endothelial cell markers and fibrinopeptide A to D-dimer ratio as a measure of coagulation and fibrinolysis balance in normal pregnancy. Gynecol Obstet Invest 1998; 46: 17–21