Abstract
Objective: The aim of this study was to explore inflammatory response and identify early potential biomarkers in mid-trimester amniotic fluid associated with subsequent spontaneous preterm delivery (PTD).
Methods: A cohort study was performed at Sahlgrenska University Hospital/Östra, Gothenburg, Sweden, between 2008 and 2010. Amniotic fluid was collected from consecutive women undergoing mid-trimester transabdominal genetic amniocentesis at 14–19 gestational weeks. Clinical data and delivery outcome variables were obtained from medical records. The analysis included 19 women with spontaneous PTD and 118 women who delivered at term. A panel of 26 candidate proteins was analyzed using Luminex xMAP technology. Candidate protein concentrations were analyzed with ANCOVA and adjusted for plate effects.
Results: The median gestational age at delivery was 35 + 3 weeks in women with spontaneous PTD and 40 + 0 weeks in women who delivered at term. Nominally significantly lower amniotic fluid levels of adiponectin (PTD: median 130,695 pg/mL (IQR 71,852–199,414) vs term: median 185,329 pg/mL (IQR (135,815–290,532)), granulocyte-macrophage colony stimulating factor (PTD: median 137 pg/mL (IQR 74–156) vs term: median 176 pg/mL (IQR 111–262)), and macrophage migration inhibitory factor (PTD: median 3025 pg/mL (IQR 1885–3891) vs term: median 3400 pg/mL (IQR 2181–5231)) were observed in the spontaneous PTD group, compared with the term delivery group, after adjusting for plate effects. No significant differences remained after Bonferroni correction for multiple comparisons.
Conclusions: Our results are important in the process of determining the etiology behind spontaneous PTD but due to the non-significance after Bonferroni correction, the results should be interpreted with caution. Further analyses of larger sample size will be required to determine whether these results are cogent and to examine whether microbial invasion of the amniotic cavity or intra-amniotic inflammation occurs in asymptomatic women in the mid-trimester with subsequent spontaneous PTD.
Introduction
Preterm delivery (PTD), defined as delivery before 37 completed weeks of gestation, is a global concern in current obstetric and neonatal care [Citation1,Citation2]. It is related to short- and long-term morbidity in neonates [Citation3] and a leading cause of neonatal death worldwide [Citation4]. PTD is mainly categorized in two different phenotypes according to the clinical presentation: medically indicated (iatrogenic) and spontaneous PTD. The latter occurs in women with preterm labor (PTL) and preterm prelabor rupture of membranes (PPROM) [Citation5].
The etiology behind spontaneous PTD is complex. Previous studies have shown that infection and inflammation are among the main origins [Citation5–13]. Microbes are believed to ascend from the lower genital tract, cross the cervical barrier and invade the decidua, chorioamniotic membranes, and presumably sterile amniotic cavity [Citation6]. In some cases, this results in an inflammatory process leading to increased cytokine release, with subsequent synthesis and release of pro-inflammatory cytokines, prostaglandins, and proteases [Citation14]. This triggers myometrial contractions, rupture of the membranes and cervical maturation, leading to spontaneous PTD [Citation6,Citation14–16]. Recent research has, however, suggested that such infections or inflammations could also arise in utero [Citation17–19] and that some women have a preexisting subclinical intra-amniotic inflammation (IAI) very early in gestation. The IAI might cause part of the increase (0.5%) in miscarriages associated with mid-trimester amniocentesis [Citation20–23].
Amniotic fluid has a very complex and dynamic composition that changes as pregnancy progresses [Citation24]. Mid-trimester amniocentesis is a common procedure to diagnose fetal chromosomal abnormalities and it also enables investigating other adverse pregnancy outcomes by examining the protein composition of mid-trimester amniotic fluid [Citation23]. This can provide insights into basic biological mechanisms, detect different pathological conditions by reflecting the function of the maternal and fetal immune systems in the maternal–fetal interface [Citation25] and provide a unique opportunity to examine the intra-amniotic environment in asymptomatic women.
Identification of early predictors for adverse pregnancy outcomes and early detection of women at high risk of spontaneous PTD are of major physiological and clinical interest, as the consequences are detrimental to the neonate. However, no single biomarker that possesses sensitivity and predictive ability for the early detection of spontaneous PTD has yet been identified [Citation26]. Most likely, coordinated networks of biomarkers, rather than a single factor, affect the risk of spontaneous PTD [Citation27].
The purpose of this study was to evaluate the association between 26 selected mid-trimester amniotic fluid candidate cytokines and neuropeptides and the subsequent development of spontaneous PTD.
Materials and methods
Study design
This is a cohort study of women, aged over 18 years and with a viable singleton pregnancy, who underwent mid-trimester transabdominal genetic amniocentesis at 14–19 weeks of gestation at Sahlgrenska University Hospital/Östra, Gothenburg, Sweden, between September 2008 and July 2010. Women were consecutively enrolled and amniocentesis indications complied with local guidelines: advanced maternal age (≥35 years), anxiety, high risk at first-trimester combined screening or a family history of chromosomal abnormalities or genetic diseases. The following women were not eligible: aged under 18 years, multiple pregnancies, positive for HIV or hepatitis B, and known or suspected fetal malformations. Exclusion criteria were language difficulties, declined participation, and insufficient volume of amniotic fluid retrieved at amniocentesis.
Regional medical records were scrutinized at inclusion and after delivery for clinical data and delivery outcome variables. Women lost to follow-up, women who had a legal abortion, and women with severe chronic diseases (severe cases of, for instance, rheumatic disease, hypothyroidism, diabetes mellitus, asthma, or neurological disorders) were also excluded.
Women with a subsequent spontaneous PTD were compared with those who delivered at term. For the sake of homogeneity, the term delivery group was limited to women delivering between 38 + 0 and 41 + 6 gestational weeks. In order to obtain a representative estimation of the term delivery group, women were randomly selected to constitute the reference group. The group size was determined based on available samples.
Sample collection and processing
The mid-trimester genetic transabdominal amniocentesis was performed by an experienced operator using a 22-gauge needle under ultrasound guidance. An additional 3 ml of amniotic fluid was collected for the study and immediately stored at 4–8 °C. Within a few hours of sampling, the amniotic fluid was centrifuged for 20 min at 4 °C and 12,000 g. The supernatant was separated from the pellet and divided into aliquots that were frozen and stored at –80 °C, awaiting various batch analyses. All samples were handled according to a standardized protocol at the same laboratory.
Development of the assay panel
A panel of 26 selected mid-trimester amniotic fluid candidate cytokines and neuropeptides was developed based on previous studies in which these proteins have been associated with spontaneous PTD pathways in symptomatic women in the late second or third trimester [Citation28–30]. The panel consisted of the following selected candidate proteins with the short-form abbreviations assigned by the UniProt Consortium within parentheses; interleukin-1 beta (IL-1β), interleukin-6 (IL-6), interleukin-8 (IL-8), interleukin-10 (IL-10), interleukin-12A (IL12A), interleukin-17 [IL17 (IL17A)], interleukin-18 (IL18), interleukin-6 receptor subunit soluble α (sIL-6RA), adiponectin, brain-derived neurotropic factor (BDNF), C-reactive protein (CRP), interferon gamma (IFN-γ), granulocyte-macrophage colony stimulating factor (GM-CSF), insulin-like growth factor binding protein 1 (IGFBP-1), insulin-like growth factor binding protein 3 (IGFBP-3), leptin, C-C motif chemokine 2 [monocyte chemotactic protein 1 (MCP-1)], macrophage migration inhibitory factor (MIF), C-C motif chemokine 3 [macrophage inflammatory protein 1-α (MIP-1-α,)], matrix metalloproteinase-9 (MMP9), neurotrophin-3 (NT-3), C-C motif chemokine 5 [T-cell-specific protein RANTES or T cell-specific protein P228 (RANTES or TCP228)], tumor necrosis factor α (TNF-α), tumor necrosis factor β (TNF-β), tumor necrosis factor receptor 1 (TNF-RI), and triggering receptor expressed on myeloid cells 1 (TREM-1).
Amniotic fluid analysis
The Luminex analysis was planned in advance and performed at a specific time point. An aliquot of 200 µL of amniotic fluid supernatant from each subject was sent to the Department of Clinical Biochemistry and Immunology (Statens Serum Institut, Copenhagen, Denmark), where the concentrations of the selected candidate proteins were analyzed using an in-house multiple sandwich immunoassay based on flowmetric Luminex xMAP technology, as described previously [Citation31,Citation32]. The samples were distributed in chronological order over four plates and analyzed undiluted in duplicates by investigators blinded to clinical background and outcome information.
Ethical approval
The study was approved by the Central Ethics Review Board at the University of Gothenburg, Sweden (Dnr Ö 639-03 with several subsequent amendments). Written informed consent was obtained from all participants.
Statistical analyses
Continuous variables were compared using a nonparametric Mann–Whitney U Test and presented as median and interquartile range (IQR: 25th; 75th percentiles). Categorical variables were compared using Fisher’s exact test and presented as number (%). The concentrations of the candidate proteins were logarithmically transformed and differences between the groups were analyzed with ANCOVA, adjusting for plate effects as a potential confounder. Bonferroni correction for multiple comparisons was subsequently performed. Differences were considered statistically significant at p < .05 using a two-sided alternative hypothesis. With the current sample, we had 80% power to detect effect sizes (Cohen’s d) of 0.7 at the nominal significance level 0.05. The statistical analyses were performed in SPSS 20.0 for Windows XP OS (SPSS Inc, Chicago, IL) and R version 3.3.1. The control group was selected by simple random sampling.
Results
Characteristics of the entire study group
Of the 1030 women undergoing mid-trimester genetic amniocentesis during the study period, 185 women were not eligible, 387 women were excluded (the majority of whom declined participation) and 458 were included in the study (54.2%). PTD occurred in 6.3% (29/458), of whom 4.1% (19/458) and 2.2% (10/458) had a spontaneous and iatrogenic PTD, respectively.
Of the 429 women with a term delivery, the following were excluded: deliveries prior to gestational week 38 + 0 (n = 19), deliveries after gestational week 41 + 6 (n = 26) and women with severe chronic disease (n = 3). From the remaining group of 381 women, 120 were randomly selected. After analysis and quality control by perusing medical records, two women were excluded due to chronic disease, leaving 118 women in the final reference group ().
Maternal and neonatal characteristics of the spontaneous PTD and term delivery groups are presented in , with gestational age at delivery as the variable defining the groups. A significant difference in maternal age at sampling (PTD: median 38 years vs term: median 36 years; p = .03) was observed between the groups. No other significant differences were found except the obvious difference in birth weight (PTD: median 2483 grams vs term: median 3555 grams; p < .0001). There were no differences between the groups regarding gestational age at sampling (PTD: median 15 + 5 weeks (IQR 15 + 2–16 + 1) vs term: median 15 + 5 weeks (IQR 15 + 2–16 + 1); p = .97) nor sample processing or storage times (p ≥ .375).
Table 1. Maternal and neonatal characteristics in the spontaneous preterm delivery and term delivery groups.
Candidate protein levels
The working ranges were set as all concentrations measurable with a CV% below 20 [Citation31,Citation32] (). Concentrations below the working range were set to half of the lowest concentration in the working range: Adiponectin, 488.5 pg/mL; BDNF, 10.0 pg/mL; CRP, 200.0 pg/mL; GM-CSF, 4.0 pg/mL; IGFBP-1, 97.5 pg/mL; IGFBP-3, 97.5 pg/mL; IL-1β, 5.0 pg/mL; IL-6, 19.5 pg/mL; sIL-6RA, 19.5 pg/mL; IL-8, 2.5 pg/mL; IL-10, 10.0 pg/mL; IL12A, 4.0 pg/mL; IL-17, 4.0 pg/mL; IL-18, 10.0 pg/mL; Leptin, 97.5 pg/mL; IFN-γ, 4.0 pg/mL; MCP-1, 2.5 pg/mL; MIF, 49.0 pg/mL; MIP-1-α, 39.0 pg/mL; MMP-9, 244.0 pg/mL; NT-3, 39.0 pg/mL; RANTES, 2.5 pg/mL; TNF-α, 4.0 pg/mL; TNF-β, 4.0 pg/mL; TNF-RI, 156.5 pg/mL, and TREM-1, 97.5 pg/mL. Concentrations exceeding the working range were set to the highest concentration in the working range. In accordance with the approach reported in previous papers by our group [Citation33,Citation34], proteins with detectable amniotic fluid levels in less than 50% of the samples were excluded from further analyses. This was the case for one protein, IFN-γ, detected in 0.7% of the samples. Concentrations of the analyzed candidate proteins for the respective groups are presented in . Nominally significantly lower amniotic fluid levels of adiponectin (PTD: median 130,695 pg/mL (IQR 71,852–199,414) vs term: median 185,329 pg/mL (IQR 135,815–290,532); p = .033), GM-CSF (PTD: median 137 pg/mL (IQR 74–156) vs term: median 176 pg/mL (IQR 111–262); p = .049), and MIF (PTD: median 3025 pg/mL (IQR 1885–3891) vs term: median 3400 pg/mL (IQR 2181–5231); p = .042) were found in women with spontaneous PTD, compared with women with term delivery, after adjusting for plate effects. illustrates the correlation between the log concentrations of the proteins. There was a strong correlation between the three statistically significant proteins. No significance remained after the Bonferroni correction for multiple comparisons.
Figure 2. Heat map demonstrating the correlation (r) between the log concentrations of the examined candidate proteins. Candidate proteins in bold and italics indicate nominal significance.
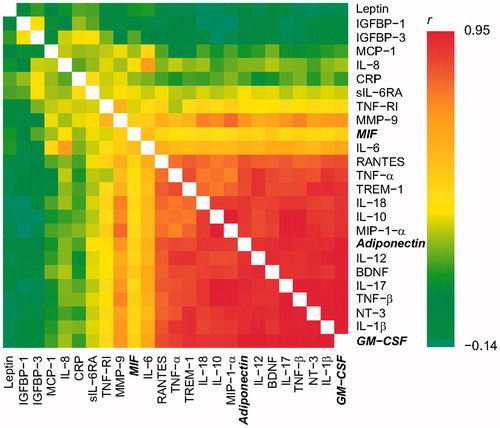
Table 2. Number of samples of each of the selected candidate proteins in the mid-trimester amniotic fluid that were below the lowest, respectively, exceeded the highest concentrations in the working range.
Table 3. Amniotic fluid levels of selected candidate proteins in relation to spontaneous preterm and term delivery.
The significant difference in maternal age at sampling observed between the groups was analyzed in relation to the protein levels. The age difference between the groups was only significant for IGFBP-1 (p = .04). Therefore, maternal age was not considered to be related to the overall protein levels (p = .10–.93). Further, in a multivariable model, we found a correlation between the fetus gender and Leptin, but the inclusion of the gender in that analysis did not affect the non-significant relation to spontaneous PTD.
Discussion
This study aimed at evaluating the associations between the concentrations of 26 selected candidate proteins in mid-trimester amniotic fluid and the subsequent development of spontaneous PTD. The key findings were as follows: (i) amniotic fluid levels of adiponectin, GM-CSF, and MIF were nominally significantly lower in the group of women with subsequent spontaneous PTD; (ii) a statistically strong correlation was identified among these three proteins; and iii) no statistically significant difference remained between the spontaneous PTD group, compared with the term delivery group after Bonferroni correction for multiple comparisons.
Pregnancy and parturition are physiological processes where inflammatory processes play a central role [Citation35]. Changes in the immune system must occur to prevent immune-mediated rejection of the semi-allogenic fetus while it must also be maintained, to prevent external pathogens that can affect the mother or fetus. A strong expression of anti-inflammatory mediators and reduced expression of many, but not all, cytokines linked to host defense and inflammatory immune capacity are found already in the second trimester of healthy pregnancy [Citation36], leading to an inflammatory response deployed by the fetus [Citation35]. The anti-inflammatory phenotype intensifies with increasing gestational age and is self-resolving by one year post-partum in the absence of repeat pregnancy [Citation36].
Several studies have analyzed the concentrations of one or a few proteins from mid-trimester amniotic fluid and their relation to, or possible prediction of, subsequent spontaneous PTD [Citation21,Citation23,Citation37–53]. A few studies have examined a multiplex assay panel of 5–8 candidate proteins from mid-trimester amniotic fluid in relation to spontaneous PTD [Citation25,Citation54,Citation55]. More comprehensive multiplex assay panels from mid-trimester amniotic fluid have been reported on patients with cerclage [Citation56], in women expecting twins [Citation57] and in women with symptoms of threatening PTD in the second and third trimester [Citation28–30]. A few systematic reviews and meta-analyses of mid-trimester amniotic fluid protein levels in relation to spontaneous PTD have been performed [Citation26,Citation58,Citation59]. The results of previous studies are conflicting, most likely due to dissimilarities in study design and diversities in study populations [Citation26].
Some of the previous studies have reported higher levels of inflammatory biomarkers in mid-trimester amniotic fluid in women with a subsequent spontaneous PTD [Citation21,Citation23,Citation37,Citation40–43,Citation47–50,Citation53,Citation54,Citation58], whereas other studies did not find any significant differences [Citation25,Citation26,Citation38,Citation39,Citation44–46,Citation51,Citation52,Citation55,Citation59]. Our study design, definition of groups and gestational age at sampling were most similar to the research by Bamberg et al. [Citation38] and Puchner et al. [Citation51], who found lower, but not significantly lower, levels of selected inflammatory mediators in women with spontaneous PTD, compared with women delivering at term. However, due to general inconsistency in study design and diversities in study populations, the results from previous studies are probably incomparable and further studies with larger cohorts are needed to elucidate whether candidate proteins from the mid-trimester amniotic fluid can predict subsequent spontaneous PTD.
In this study, we found nominally significantly lower amniotic fluid levels of adiponectin, GM-CSF, and MIF in the group of women with subsequent spontaneous PTD, compared with the group of women with a term delivery. However, the significance did not remain after Bonferroni correction for multiple comparisons.
Adiponectin is an adipokine with a well-established role in glucose metabolism and regulation of vasculature. It also has a potent anti-inflammatory effect with important regulatory effects on both the innate and adaptive immune response [Citation60]. Adiponectin appears to be a physiological component of human amniotic fluid [Citation60–62] and an association between higher levels of adiponectin, PTL, and IAI has previously been reported [Citation60]. Little is, however, known about the concentration, origin or role of amniotic fluid adiponectin. Baviera et al. [Citation61] suggested that adiponectin found in amniotic fluid might be of fetal origin.
Amniotic fluid contains large amounts of GM-CSF, which probably has various functions [Citation63]. GM-CSF is a cytokine that promotes white cell maturation and it participates in the metabolism of pulmonary surfactant [Citation64]. In early pregnancy, it has also been postulated to be involved in normal placental function by regulating the invasion and differentiation of placental trophoblast [Citation63]. Little is known about the production of GM-CSF during pregnancy or in the neonatal period but Bry et al. [Citation64] suggested that it is developmentally regulated and that production increases in inflammatory conditions during pregnancy.
MIF has been suggested to be produced by embryonic tissues and to have an important function in embryonic development [Citation65]. MIF has also emerged as an important cytokine for preventing endotoxic shock and death associated with bacterial infection. It has also been studied in relation to IAI and PTD, and PTL with intact membranes, suggesting that elevated amniotic fluid concentrations of MIF were associated with IAI, histologic chorioamnionitis, and shorter latency from sampling to delivery [Citation66].
Correlations between inflammatory biomarkers have previously been studied in women with PTL [Citation67] and women with spontaneous PTD [Citation27], comparing IAI, sterile IAI, and microbial-associated IAI [Citation67]. Correlations have also been compared in African-American and Caucasian women with and without microbial invasion of the intra-amniotic cavity (MIAC) [Citation27]. However, to the best of our knowledge, this is the first study specifically focusing on mid-trimester amniotic fluid and the correlations between the log concentrations of the examined candidate proteins in relation to spontaneous PTD.
The strengths of this study are that a broad panel of selected candidate proteins, previously associated with spontaneous PTD pathways [Citation28–30], was analyzed, enabling us to examine how these proteins correlate with each other. None of the examined proteins were very strong in itself, suggesting that a network of proteins, rather than a single protein, would affect the risk of spontaneous PTD. All the amniotic fluid samples were handled according to a standardized laboratory protocol and analyzed in one batch at the same facility. The very low rate of lost-to-follow-ups and the very thorough correction for plate effects should also be considered as strengths.
The weaknesses of the study were the low sample size, which limited the detection of subtle differences between biomarker concentrations, and the lack of replication. One limitation might be that the respective PTL and PPROM phenotypes may differ somewhat, but the low sample size did not allow this differentiation in the spontaneous PTD group. Another potential weakness is that most women were of advanced maternal age or had a high risk of chromosomal abnormalities, as they were undergoing amniocentesis for genetic analysis. Hence, the findings do not reflect the general population. Finally, sample size calculation was not performed, instead, power analysis was used to calculate the smallest effect size possible to detect given a set sample size. We do not consider the effect size to be unrealistically large. With our sample size, we can conclude that there are no major differences between the groups. Similar studies have not previously been performed so this study forms a basis for future larger studies.
The median gestational age at delivery among women with a spontaneous PTD was 35 + 3 weeks in this cohort. Late spontaneous PTD does not usually have an infectious or acute inflammatory etiology. It could thus be discussed whether these results are primarily relevant in the case of late spontaneous PTD, and whether or not these cases of spontaneous PTD were based on an infection or inflammation.
The dynamic environment in utero changes as pregnancy progresses. Because of the increase in the miscarriage rate (∼0.5%) after mid-trimester amniocentesis [Citation68], early amniotic fluid samples for research can only be collected according to clear clinical indications. The timing of cytokine release, the local environment in which they act, the presence of competing or synergistic elements, cytokine receptor density, and tissue responsiveness to each cytokine could potentially differ during pregnancy, raising the question of whether this gestational age is the optimal timing for detection of early IAI. As spontaneous PTD can be considered a syndrome caused by heterogeneous pathways, the respective optimal gestational ages might either overlap or digress.
Conclusions
The etiology behind spontaneous PTD is complex and understanding of the sequence and timing of events preceding this condition is still incomplete [Citation69]. There is, thus, an urgent need to study asymptomatic women and to detect the underlying condition at an early gestation before PTL or PPROM occur. The results of this study are important in the process of determining the etiology behind spontaneous PTD but due to the non-significance after Bonferroni correction, the results should be interpreted with caution. Previously published research on the mid-trimester amniotic fluid in relation to spontaneous PTD is inconclusive and larger studies are required to examine whether one or several candidate proteins can predict subsequent spontaneous PTD in asymptomatic women in the mid-trimester.
Acknowledgments
The authors would like to acknowledge the Antenatal Clinic at the Department of Obstetrics and Gynecology, Sahlgrenska University Hospital/Östra, Gothenburg, Sweden, for their help with recruitment and sampling and the staff at the Perinatal Laboratory for their help with recruitment and processing of samples.
Disclosure statement
No potential conflict of interest was reported by the authors.
Additional information
Funding
References
- Bryce J, Boschi-Pinto C, Shibuya K, et al. WHO estimates of the causes of death in children. Lancet. 2005;365:1147–1152.
- Goldenberg RL, Andrews WW, Hauth JC. Choriodecidual infection and preterm birth. Nutr Rev. 2002;60:S19–S25.
- Woodward LJ, Moor S, Hood KM, et al. Very preterm children show impairments across multiple neurodevelopmental domains by age 4 years. Arch Dis Child. 2009;94:339–344.
- Goldenberg RL, Culhane JF, Iams JD, et al. Epidemiology and causes of preterm birth. Lancet. 2008;371:75–84.
- Morken NH, Kallen K, Hagberg H, et al. Preterm birth in Sweden 1973–2001: rate, subgroups, and effect of changing patterns in multiple births, maternal age, and smoking. Acta Obstet Gynecol Scand. 2005;84:558–565.
- Romero R, Espinoza J, Goncalves LF, et al. The role of inflammation and infection in preterm birth. Semin Reprod Med. 2007;25:021–039.
- Holst RM, Mattsby-Baltzer I, Wennerholm UB, et al. Interleukin-6 and interleukin-8 in cervical fluid in a population of Swedish women in preterm labor: relationship to microbial invasion of the amniotic fluid, intra-amniotic inflammation, and preterm delivery. Acta Obstet Gynecol Scand. 2005;84:551–557.
- Jacobsson B, Holst RM, Andersson B, et al. Monocyte chemotactic protein-2 and -3 in amniotic fluid: relationship to microbial invasion of the amniotic cavity, intra-amniotic inflammation and preterm delivery. Acta Obstet Gynecol Scand. 2005;84:566–571.
- Jacobsson B, Holst RM, Mattsby-Baltzer I, et al. Interleukin-18 in cervical mucus and amniotic fluid: relationship to microbial invasion of the amniotic fluid, intra-amniotic inflammation and preterm delivery. BJOG. 2003;110:598–603.
- Jacobsson B, Holst RM, Wennerholm UB, et al. Monocyte chemotactic protein-1 in cervical and amniotic fluid: relationship to microbial invasion of the amniotic cavity, intra-amniotic inflammation, and preterm delivery. Am J Obstet Gynecol. 2003;189:1161–1167.
- Jacobsson B, Mattsby-Baltzer I, Andersch B, et al. Microbial invasion and cytokine response in amniotic fluid in a Swedish population of women with preterm prelabor rupture of membranes. Acta Obstet Gynecol Scand. 2003;82:423–431.
- Jacobsson B, Mattsby-Baltzer I, Andersch B, et al. Microbial invasion and cytokine response in amniotic fluid in a Swedish population of women in preterm labor. Acta Obstet Gynecol Scand. 2003;82:120–128.
- Ruetschi U, Rosen A, Karlsson G, et al. Proteomic analysis using protein chips to detect biomarkers in cervical and amniotic fluid in women with intra-amniotic inflammation. J Proteome Res. 2005;4:2236–2242.
- El-Bastawissi AY, Williams MA, Riley DE, et al. Amniotic fluid interleukin-6 and preterm delivery: a review. Obstet Gynecol. 2000;95:1056–1064.
- Andrews WW, Goldenberg RL, Hauth JC. Preterm labor: emerging role of genital tract infections. Infect Agents Dis. 1995;4:196–211.
- Goldenberg RL, Hauth JC, Andrews WW. Intrauterine infection and preterm delivery. N Engl J Med. 2000;342:1500–1507.
- Aagaard K, Ma J, Antony KM, et al. The placenta harbors a unique microbiome. Sci Transl Med. 2014;6:237ra65.
- Jimenez E, Fernandez L, Marin ML, et al. Isolation of commensal bacteria from umbilical cord blood of healthy neonates born by cesarean section. Curr Microbiol. 2005;51:270–274.
- Jimenez E, Marin ML, Martin R, et al. Is meconium from healthy newborns actually sterile? Res Microbiol. 2008;159:187–193.
- Chaiworapongsa T, Romero R, Tolosa JE, et al. Elevated monocyte chemotactic protein-1 in amniotic fluid is a risk factor for pregnancy loss. J Matern Fetal Neonatal Med. 2002;12:159–164.
- Wenstrom KD, Andrews WW, Hauth JC, et al. Elevated second-trimester amniotic fluid interleukin-6 levels predict preterm delivery. Am J Obstet Gynecol. 1998;178:546–550.
- Wenstrom KD, Andrews WW, Tamura T, et al. Elevated amniotic fluid interleukin-6 levels at genetic amniocentesis predict subsequent pregnancy loss. Am J Obstet Gynecol. 1996;175:830–833.
- Yoon BH, Oh SY, Romero R, et al. An elevated amniotic fluid matrix metalloproteinase-8 level at the time of mid-trimester genetic amniocentesis is a risk factor for spontaneous preterm delivery. Am J Obstet Gynecol. 2001;185:1162–1167.
- Underwood MA, Gilbert WM, Sherman MP. Amniotic fluid: not just fetal urine anymore. J Perinatol. 2005;25:341–348.
- Heikkinen J, Mottonen M, Pulkki K, et al. Cytokine levels in midtrimester amniotic fluid in normal pregnancy and in the prediction of pre-eclampsia. Scand J Immunol. 2001;53:310–314.
- Shah J, Baxi B. Identification of biomarkers for prediction of preterm delivery. J Med Soc. 2016;30:3–14.
- Velez DR, Fortunato SJ, Morgan N, et al. Patterns of cytokine profiles differ with pregnancy outcome and ethnicity. Hum Reprod. 2008;23:1902–1909.
- Holst RM, Hagberg H, Wennerholm UB, et al. Prediction of microbial invasion of the amniotic cavity in women with preterm labour: analysis of multiple proteins in amniotic and cervical fluids. BJOG. 2011;118:240–249.
- Holst RM, Hagberg H, Wennerholm UB, et al. Prediction of spontaneous preterm delivery in women with preterm labor: analysis of multiple proteins in amniotic and cervical fluids. Obstet Gynecol. 2009;114:268–277.
- Vogel I, Goepfert AR, Thorsen P, et al. Early second-trimester inflammatory markers and short cervical length and the risk of recurrent preterm birth. J Reprod Immunol. 2007;75:133–140.
- Skogstrand K, Thorsen P, Norgaard PB, et al. Simultaneous measurement of 25 inflammatory markers and neurotrophins in neonatal dried blood spots by immunoassay with xMAP technology. Clin Chem. 2005;51:1854–1866.
- Skogstrand K. Multiplex assays of inflammatory markers, a description of methods and discussion of precautions – our experience through the last ten years. Methods. 2012;56:204–212.
- Cobo T, Kacerovsky M, Palacio M, et al. Intra-amniotic inflammatory response in subgroups of women with preterm prelabor rupture of the membranes. PLoS One. 2012;7:e43677.
- Kacerovsky M, Musilova I, Khatibi A, et al. Intraamniotic inflammatory response to bacteria: analysis of multiple amniotic fluid proteins in women with preterm prelabor rupture of membranes. J Matern Fetal Neonatal Med. 2012;25:2014–2019.
- Romero R, Gotsch F, Pineles B, et al. Inflammation in pregnancy: its roles in reproductive physiology, obstetrical complications, and fetal injury. Nutr Rev. 2008;65:S194–S202.
- Graham C, Chooniedass R, Stefura WP, et al. In vivo immune signatures of healthy human pregnancy: inherently inflammatory or anti-inflammatory? PLoS One. 2017;12:e0177813.
- Apuzzio J, Chan Y, Al-Khan A, et al. Second-trimester amniotic fluid interleukin-10 concentration predicts preterm delivery. J Matern Fetal Neonatal Med. 2004;15:313–317.
- Bamberg C, Fotopoulou C, Thiem D, et al. Correlation of midtrimester amniotic fluid cytokine concentrations with adverse pregnancy outcome in terms of spontaneous abortion, preterm birth, and preeclampsia. J Matern Fetal Neonatal Med. 2012;25:812–817.
- Borna S, Mirzaie F, Abdollahi A. Mid-trimester amniotic fluid C-reactive protein, ferritin and lactate dehydrogenase concentrations and subsequent risk of spontaneous preterm labour. Aust N Z J Obstet Gynaecol. 2009;49:400–403.
- Daskalakis G, Thomakos N, Papapanagiotou A, et al. Amniotic fluid interleukin-18 at mid-trimester genetic amniocentesis: relationship to intraamniotic microbial invasion and preterm delivery. BJOG. 2009;116:1743–1748.
- Gervasi MT, Romero R, Bracalente G, et al. Midtrimester amniotic fluid concentrations of interleukin-6 and interferon-gamma-inducible protein-10: evidence for heterogeneity of intra-amniotic inflammation and associations with spontaneous early (<32 weeks) and late (>32 weeks) preterm delivery. J Perinat Med. 2012;40:329–343.
- Ghezzi F, Franchi M, Raio L, et al. Elevated amniotic fluid C-reactive protein at the time of genetic amniocentesis is a marker for preterm delivery. Am J Obstet Gynecol. 2002;186:268–273.
- Ghidini A, Jenkins CB, Spong CY, et al. Elevated amniotic fluid interleukin-6 levels during the early second trimester are associated with greater risk of subsequent preterm delivery. Am J Reprod Immunol. 1997;37:227–231.
- Hong SN, Joo BS, Chun S, et al. Prediction of preterm delivery using levels of vascular endothelial growth factor and leptin in amniotic fluid from the second trimester. Arch Gynecol Obstet. 2015;291:265–271.
- Kesrouani A, Chalhoub E, El Rassy E, et al. Data on clinical significance of second trimester inflammatory biomarkers in the amniotic fluid in predicting preterm delivery. Data Brief. 2016;9:47–50.
- Kesrouani A, Chalhoub E, El Rassy E, et al. Prediction of preterm delivery by second trimester inflammatory biomarkers in the amniotic fluid. Cytokine. 2016;85:67–70.
- Kim A, Lee ES, Shin JC, et al. Identification of biomarkers for preterm delivery in mid-trimester amniotic fluid. Placenta. 2013;34:873–878.
- Oz M, Polat B, Ozgu E, et al. Interleukin-6 and C-reactive protein levels in the amniotic fluid as indicators of preterm delivery in Turkish women. Clin Exp Obstet Gynecol. 2015;42:801–804.
- Ozer KT, Kavak ZN, Gokaslan H, et al. Predictive power of maternal serum and amniotic fluid CRP and PAPP-A concentrations at the time of genetic amniocentesis for the preterm delivery. Eur J Obstet Gynecol Reprod Biol. 2005;122:187–190.
- Puchner K, Iavazzo C, Gourgiotis D, et al. Mid-trimester amniotic fluid interleukins (IL-1beta, IL-10 and IL-18) as possible predictors of preterm delivery. In Vivo. 2011;25:141–148.
- Puchner K, Iavazzo C, Gourgiotis D, et al. The implication of second-trimester amniotic fluid TNF-alpha, cytochrome C and cell death nucleosomes in the prediction of preterm labor and/or premature rupture of membranes. Arch Gynecol Obstet. 2012;285:37–43.
- Tarim E, Bagis T, Kilicdag EB, et al. Are amniotic fluid C-reactive protein and glucose levels, and white blood cell counts at the time of genetic amniocentesis related with preterm delivery? J Perinat Med. 2005;33:524–529.
- Thomakos N, Daskalakis G, Papapanagiotou A, et al. Amniotic fluid interleukin-6 and tumor necrosis factor-alpha at mid-trimester genetic amniocentesis: relationship to intra-amniotic microbial invasion and preterm delivery. Eur J Obstet Gynecol Reprod Biol. 2010;148:147–151.
- La Sala GB, Ardizzoni A, Capodanno F, et al. Protein microarrays on midtrimester amniotic fluids: a novel approach for the diagnosis of early intrauterine inflammation related to preterm delivery. Int J Immunopathol Pharmacol. 2012;25:1029–1040.
- Payne MS, Feng Z, Li S, et al. Second trimester amniotic fluid cytokine concentrations, Ureaplasma sp. colonisation status and sexual activity as predictors of preterm birth in Chinese and Australian women. BMC Pregnancy Childbirth. 2014;14:340.
- Son GH, You YA, Kwon EJ, et al. Comparative analysis of midtrimester amniotic fluid cytokine levels to predict spontaneous very pre-term birth in patients with cervical insufficiency. Am J Reprod Immunol. 2016;75:155–161.
- Lee SM, Park JS, Norwitz ER, et al. Mid-trimester amniotic fluid pro-inflammatory biomarkers predict the risk of spontaneous preterm delivery in twins: a retrospective cohort study. J Perinatol. 2015;35: 542–546.
- Liu Y, Liu Y, Zhang R, et al. Early- or mid-trimester amniocentesis biomarkers for predicting preterm delivery: a meta-analysis. Ann Med. 2017;49:1–10.
- Polettini J, Cobo T, Kacerovsky M, et al. Biomarkers of spontaneous preterm birth: a systematic review of studies using multiplex analysis. J Perinat Med. 2017;45:71–84.
- Mazaki-Tovi S, Romero R, Vaisbuch E, et al. Adiponectin in amniotic fluid in normal pregnancy, spontaneous labor at term, and preterm labor: a novel association with intra-amniotic infection/inflammation. J Matern Fetal Neonatal Med. 2010;23:120–130.
- Baviera G, Corrado F, Dugo C, et al. Midtrimester amniotic fluid adiponectin in normal pregnancy. Clin Chem. 2007;53:1723–1724.
- De Leon-Luis J, Perez R, Pintado Recarte P, et al. Second trimester amniotic fluid adiponectin level is affected by maternal tobacco exposure, insulin, and PAPP-A level. Eur J Obstet Gynecol Reprod Biol. 2012;165:189–193
- Hayashi M, Sohma R, Sumioka Y, et al. Granulocyte-macrophage colony-stimulating factor levels in amniotic fluid before the onset of labor and during labor do not differ in normal pregnancies. Am J Reprod Immunol. 2006;55:69–75.
- Bry K, Hallman M, Teramo K, et al. Granulocyte-macrophage colony-stimulating factor in amniotic fluid and in airway specimens of newborn infants. Pediatr Res. 1997;41:105–109.
- Suzuki H, Nishihira J, Koyama Y, et al. The role of macrophage migration inhibitory factor in pregnancy and development of murine embryos. Biochem Mol Biol Int. 1996;38:409–416.
- Chaiworapongsa T, Romero R, Espinoza J, et al. Macrophage migration inhibitory factor in patients with preterm parturition and microbial invasion of the amniotic cavity. J Matern Fetal Neonatal Med. 2005;18:405–416.
- Romero R, Grivel JC, Tarca AL, et al. Evidence of perturbations of the cytokine network in preterm labor. Am J Obstet Gynecol. 2015;213:836 e1–e18.
- Esrig SM, Leonardi DE. Spontaneous abortion after amniocentesis in women with a history of spontaneous abortion. Prenat Diagn. 1985;5:321–328.
- Kacerovsky M, Lenco J, Musilova I, et al. Proteomic biomarkers for spontaneous preterm birth: a systematic review of the literature. Reprod Sci. 2014;21:283–295.