Abstract
Objective
Baroreflex is a regulatory mechanism that slows the fetal heart rate. This study aimed to investigate the effects of lipopolysaccharide (LPS)-induced endotoxemia on fetal baroreceptor sensitivity in preterm fetal sheep.
Methods
The changes in fetal baroreceptor sensitivity were measured in seven chronically instrumented preterm fetal sheep. Fetal baroreceptor sensitivity was measured in three phases: (A) control phase, defined as the 24 h before the first injection of LPS; (B) acute phase, defined as the 24 h between the first and second injections of LPS; and (C) fetal acidosis phase, defined as the time from the second LPS injection until intrauterine fetal death. Histological examinations of the fetal membrane and umbilical cord were also conducted.
Results
Each fetus developed metabolic acidosis after the second injection of LPS. The fetuses died 24.7 (SD = 6.1) hours after the second injection of LPS. Both the umbilical cord and fetal membranes showed histological evidence of severe inflammation. In total, 163 fetal baroreceptor measurements were performed in this experiment (A, n = 77 times; B, n = 60 times; C, n = 26 times). Fetal baroreceptor sensitivity showed significant differences in all three phases (A: 2.7 [SD = 0.2]; B: 2.5 [SD = 0.2]; and C: 1.5 [SD = 0.2]). Post hoc tests showed that baroreceptor sensitivity in the acidosis phase had decreased significantly compared to that in the control and acute phases (p<.001 and p=.002, respectively).
Conclusions
Fetal baroreceptor sensitivity decreased during fetal acidosis induced by LPSs.
Introduction
Intrauterine inflammation, which is a form of chorioamnionitis (CAM), is related to preterm birth and carries an increased risk of adverse perinatal outcomes in term and near-term infants [Citation1], occasionally resulting in intrauterine fetal death (IUFD) [Citation2–5]. Intrauterine inflammation with or without intrapartum hypoxic-ischemic stress can cause fetal brain damage [Citation6,Citation7]. Therefore, careful intrapartum fetal management is essential in cases of intrauterine inflammation.
Electronic fetal heart rate (FHR) monitoring was introduced in the 1960s as an alternative to the laborious auscultation of the fetal heart and is now the standard technique for intrapartum FHR assessment. FHR deceleration such as variable decelerations and late decelerations represent periodic FHR patterns that are related to fetal oxygenation. In clinical settings, it is widely accepted that the deeper the FHR deceleration, the greater the likelihood of fetal hypoxemia [Citation8,Citation9]. The FHR decelerations by the baroreflex secondary to brief umbilical cord occlusion during the first couple of seconds are primarily mediated by fetal baroreceptor sensitivity [Citation10], this deceleration plays a fundamental role in the cardiovascular response to fetal hypoxemia by increasing the vagal cardioinhibitory activity and decreasing the sympathetic discharge, which acts to reduce heart rate and vascular resistance, buffering the increase in blood pressure (BP) [Citation11]. Presently, the effect of intrauterine inflammation on fetal baroreceptor sensitivity, which indicates the extent of FHR deceleration, is not well known [Citation12].
We had previously developed a model on fetal acidosis due to severe intrauterine inflammation using chronically instrumented preterm sheep [Citation13]. We also developed a chronically instrumented fetal sheep model that can examine fetal baroreceptor sensitivity [Citation14]. Hence, we aimed to examine the baroreceptor sensitivity during intrauterine inflammation using chronically instrumented preterm fetal sheep. Intrauterine inflammation was induced by the infusion of lipopolysaccharide (LPS) into the amniotic cavity and confirmed histologically [Citation13].
Methods
Animal preparation and postoperative care
This study was approved by the Animal Ethics Committee of the Fukushima Medical University (no. 26036) and the guidelines for the care and use of animals mandated by the local institution were followed. Seven pregnant Corriedale/Suffolk sheep with singleton fetuses (Japan-lamb Co., Ltd., Fukuyama City, Japan) were surgically instrumented at 113–120 days of gestation (term = 145 days). Pregnant sheep were purchased from a breeder and maintained in the animal laboratory facility with unlimited access to food and water. The sheep were kept without food overnight prior to the day of surgery and prior to anesthetic exposure. All surgeries were performed under general anesthesia induced by an intramuscular injection of xylazine (0.2 mg/kg; Bayer Yakuhin Ltd., Osaka, Japan), followed by an intravenous administration of dexmedetomidine hydrochloride (3 mg/kg/min; Maruishi Pharmaceutical Co., Ltd., Osaka, Japan) with an oxygen mask. Wool was shorn at the sites requiring access. Surgical sites were prepared using standard sterile procedures and intravascular access was obtained percutaneously at the left femoral artery (invasive BP monitoring) and left femoral vein (catheter access). Heart rate and invasive arterial BP were monitored throughout the procedures. Following all procedures, catheters and vascular access were removed, anesthesia was ceased, and the sheep were stabilized before being transferred to the appropriate holding pens. During the operation and experiment, acetaminophen (1000 mg; Terumo Co., Ltd., Tokyo, Japan) was intravenously administered to the sheep to control surgical and postoperative pain.
A midline skin incision was made under aseptic conditions. The fetal head was covered with a surgical glove filled with warm saline and delivered through a hysterotomy.
Polyvinyl catheters (1.8 mm outer diameter and 1.2 mm inner diameter; Imamura Co., Ltd., Tokyo, Japan) were placed on one side of both fetal carotid arteries and fetal jugular veins, in the amniotic cavity, and around maternal femoral veins. The fetal blood samples were collected from the carotid arteries, and the fetal jugular veins were used for injection of the drug agents. Antibiotics were administered via the maternal femoral veins. Electrodes attached to the polyvinyl-coated stainless-steel wires (Tesco Co., Ltd., Tokyo, Japan) were placed on the fetal trunk and the fetal bilateral upper limbs to record the fetal electrocardiograms. Fetal electrocardiograms were used to measure FHR. Subsequently, we reimplanted the fetus to the uterus and closed all uterine and abdominal incisions. All fetal catheters and electrodes and maternal catheters were exteriorized through the maternal flank.
Postoperatively, the ewes were housed in metabolic cages with free access to food and water and were exposed to a 12-h dark/light cycle (light 6:00–18:00 h). The room temperature was maintained at 18 ± 2 °C. Postoperative antibiotics were administered through the maternal vein (1 g, flomoxef sodium; Shionogi Co., Ltd., Tokyo, Japan) every 12 h for three days, until the first injection of LPS. All ewes were alive throughout the study period, and no ewes were euthanized.
Experimental protocol and measurement
The experimental procedures are shown in Supplemental Figure 1. Experiments were performed 48 h postoperatively. We developed the in utero inflammation model based on our previous study [Citation13]. Fifty micrograms of granulocyte-colony stimulating factor (G-CSF: Neutrogin R. Chugai Co., Ltd., Tokyo, Japan) solubilized in 2 mL of saline were injected intravenously into the fetuses on postoperative days 4–6, and 40 mg of LPS (Escherichia coli 055:B5 endotoxin; Sigma Aldrich Co., LLC, St. Louis, MO) solubilized in 10 mL of saline was administered by a bolus infusion into the amniotic cavity on postoperative days 4 and 5. We continued the experiment until IUFD.
We divided the present experiment into three phases. The control phase was defined as the 24-h period before the first LPS injection, the acute phase as the 24-h period between the first and second LPS injections, and the acidosis phase as the period from the second LPS injection until IUFD.
Fetal arterial pH, partial pressure of CO2 (pCO2) (mmHg), pO2 (mmHg), and base excess (BE, mmol/L) were analyzed during each of the three phases. The first sample was collected at the time of the first LPS injection. The second sample, for the 24-h data, was collected 24 h after the first LPS injection. The third sample was randomly collected during the acidosis phase. A pH/blood gas analyzer (Corning 170; Ciba Corning Diagnostics Corp., Medfield, MA) was used with the temperature corrected to 37 °C.
The FHR was measured using an acquisition system (Power Lab; AD Instruments, Castle Hill, Australia). For the FHR calculations, the individual R-waves from the fetal electrocardiograms, with sample rates of 1 kHz, were sequentially recognized. The distances between consecutive R-wave peaks were measured and then converted to beats per min. The FHR was continuously measured until IUFD.
Baroreceptor sensitivity was calculated using changes in fetal mean arterial pressure (MAP) and FHR. The fetal baroreceptor sensitivity was quantified based on a previously described method [Citation14,Citation15]. In short, 30 μg of phenylephrine (Neosynesine, Kowa Company Ltd., Nagoya, Japan) dissolved in 2 mL saline was intravenously injected into the fetuses after at least two minutes of stabilization of the FHR and fetal BP. The ratio of maximal FHR decrease, and maximal fetal MAP increase (ΔFHR/ΔMAP) was calculated to quantify the fetal baroreceptor sensitivity (Supplemental Figure 2). MAP was determined using fetal systolic BP (SBP) and fetal diastolic BP (DBP) as follows: MAP=(SBP + 2 × DBP)/3. The dose of phenylephrine was determined by our preliminary study, using eight chronically instrumented fetal sheep at the gestational age of 120 days [Citation14]. The FHR was determined at the time of injection for phenylephrine. Fetal baroreceptor sensitivity was measured as far as possible if a stable FHR was confirmed in each phase. During the acidosis phase, baroreceptor sensitivity was measured after confirming fetal arterial pH at <7.200. There was a 10-min interval between successive phenylephrine injections.
Histological examination
All the fetuses were weighed after spontaneous vaginal delivery after fetal death. The umbilical cord and rolls of chorion and decidua were placed in 10% buffered formalin for fixation. We classified the severity of inflammation of the umbilical cord and fetal membranes into one of six scoring categories. This classification is based on the established methods and the scoring system by Salafia et al. [Citation16], with the addition of necrotizing funisitis, according to Navarro and Blanc [Citation17] (Supplemental Table 1). The scoring of inflammation was evaluated by a single pathologist who was unaware of the study design.
Statistical analyses
The FHR was determined before phenylephrine administration to calculate the fetal baroreceptor sensitivity. We assessed changes in FHR and baroreceptor sensitivity in the three phases (control, acute, and acidosis). Fetal arterial blood gas data were compared in the three phases. We used generalized linear mixed models to examine the changes in each parameter during the three phases, setting each animal as a random effect. When a significant overall effect was found, the Bonferroni test was performed post hoc. SPSS software version 24 (IBM Corp., Armonk, NY) was used for all statistical analyses. All data are presented as mean (standard error). A p value <.05 was accepted as statistically significant.
Results
The fetuses died 24.7 (SD = 6.1) hours after the second injection of LPS. The average fetal body weight at the end of the study was 2.7 kg (SD = 0.4, n = 7). All the maternal subjects remained healthy during the study.
Histological examination
The grading of inflammation for the umbilical cord and fetal membranes was generated from seven and five experiments, respectively. The scores for the umbilical cords and fetal membranes were 4.3 (SD = 0.2, n = 7) and 4.8 (SD = 0.2, n = 5), respectively, indicating severe inflammation (Supplemental Table 2 and Supplemental Figure 3).
Fetal arterial blood gas
Fetal arterial pH in the acidosis phase decreased significantly compared to the control and acute phases (p<.001 and p=.001, respectively) (). Fetal arterial BE also decreased significantly in the acidosis phase compared to the control and acute phases (p=.002 and p=.007, respectively). No significant changes were observed in fetal pCO2 and pO2 (p= .132 and p= .295, respectively).
Table 1. Changes in fetal arterial blood gas in each phase.
Change in FHR and baroreceptor sensitivity
In total, 163 FHR measurements and baroreceptor sensitivity were analyzed (control: n = 77; acute: n = 60; and acidosis: n = 26). Supplement Figure 4 shows the change in FHR among the three phases. The average (standard error) FHR at the acidosis phase was 167 (4) beats per min, and this was significantly lower than that in the control (175 (3), p= .003) and acute phases (175 (4), p= .016).
shows the changes in fetal baroreceptor sensitivity during the three phases. The average (standard error) fetal baroreceptor sensitivity at control, acute, and acidosis phase was 2.7 (0.2), 2.5 (0.2), and 1.5 (0.2), respectively. Baroreceptor sensitivity in the acidosis phase decreased significantly compared to the control and acute phases (p < .001 and p = .002, respectively).
Figure 1. Changes in fetal baroreceptor sensitivity among the three phases. One hundred and sixty-three FHR baroreceptor sensitivity were analyzed (control: n = 77; acute: n = 60; and acidosis: n = 26). The average (standard error) fetal baroreceptor sensitivity at control, acute, and acidosis phases was 2.7 (0.2), 2.5 (0.2), and 1.5 (0.2), respectively. The average baroreceptor sensitivity in the acidosis phase was significantly lower than that in the control (p<.001) and acute phases (p = .002). ΔMAP: change in mean arterial pressure; ΔFHR: change in fetal heart rate changes in fetal heart rate (FHR) among the three phases.
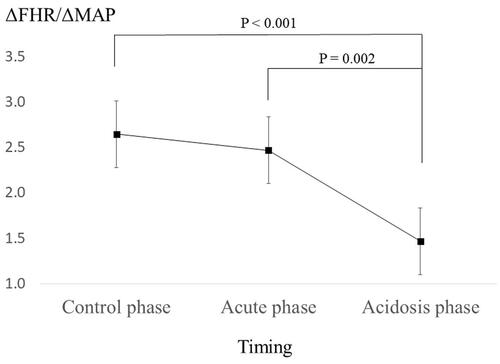
Discussion
To our knowledge, this is the first study to examine the changes in the fetal baroreceptor sensitivity during fetal acidosis due to intrauterine inflammation. Overall, the fetal baroreceptor sensitivity remained unchanged when fetal wellbeing was good but decreased when the condition of the fetus deteriorated over the course of intrauterine inflammation induced by LPS.
We created a histological intrauterine infection model that resulted in an IUFD approximately 24 h after the second injection of LPS into the amniotic cavity, referring to our previous experimental model [Citation13]. Using this model, we previously reported that LPS-induced endotoxemia resulted in increases in the short-term FHR variability, which was determined based on fetal electrocardiogram, along with a deterioration in the fetal condition [Citation13].
Usually, fetal metabolic acidosis is established as a result of a hypoxic-ischemic event. Furthermore, it is thought that inflammation, secondary to infection, can cause direct fetal neurological injury and acts synergistically with hypoxia to increase the risk of encephalopathy [Citation7]. A fetal inflammatory response secondary to infection could lead to fetal hypotension like a drop in BP seen in septic shock with subsequent decreased perfusion in these vulnerable watershed regions of the fetal brain [Citation18]. However, the current study showed that fetuses developed metabolic acidosis via inflammation without a significant change in the fetal arterial pO2, suggesting metabolic acidosis occurred because of a nonhypoxic event. This contradictory event may be explained by the theory that pro-inflammatory cytokines could reduce the fetal threshold at which hypoxia becomes neurotoxic, thereby making the brain much more vulnerable to even mild hypoxia [Citation19].
In this study, fetal blood gas in the control phase (pO2 of 17 mmHg and a pCO2 of 35 mmHg) was indicative of mild hypoxia and severe hypocapnia that made it difficult to understand the change in fetal blood gas during inflammation. Two potential reasons for the mild hypoxia and severe hypocapnia at the beginning of the experiments exist. One is temperature induced change; the blood gas temperature correction was set as 37 °C, resulting in a low pO2 and pCO2 levels compared to temperature set as 38 °C [Citation20]. Second, although we did not measure the maternal sheep blood gas condition, hyperoxygenation of the maternal sheep during the procedure could lead to hypocapnia in fetal sheep.
The baroreceptor reflex mediates the synergistic control of BP by the autonomic nerve system (ANS) [Citation21]. Increases in BP activate the baroreceptor in the carotid sinuses and the aortic arch, which leads to a decrease in the sympathetic tone and an increase in the vagal tone, resulting in a drop in the heart rate and BP. The change in FHR via the baroreceptor is thought to be a fetal defense mechanism to support the myocardial function or meet the oxygenation requirements [Citation22]. Previously, experiments on fetal baroreceptor sensitivity using chronically instrumented fetal sheep were conducted to examine the fetal cardiovascular response to a fetal hypoxic state induced by umbilical cord occlusion. Green et al. [Citation23] reported that fetal cardiovascular responses to umbilical cord occlusion were altered following repetitive occlusions during their extended study period, with a decrease in ΔFHR/ΔMAP. Pulgar et al. [Citation24] reported a decrease in the spontaneous baroreceptor sensitivity in fetal sheep during chronic mild fetal hypoxemia induced by maternal intratracheal nitrogen gas administration with increased binding of angiotensin receptors in the brainstem nuclei, indicating fetal adaptation to chronic hypoxemia. As shown in our study, baroreceptor sensitivity could also be induced by the infusion of vasopressor drugs, such as phenylephrine. Wakatsuki et al. [Citation25] previously studied chronically instrumented preterm fetal sheep and reported that fetal baroreceptor sensitivity induced by a vasopressor drug was affected by the fetal behavioral state and gestational age. We applied a drug infusion method to induce baroreceptor sensitivity as this allowed the exploration of the sigmoid relationship between a harmful fetal condition and baroreceptor sensitivity [Citation24]. The timing of infusion of phenylephrine is determined based on a stabilized baseline and FHR and at ≥10 min interval. Therefore, the frequency of measurement for baroreceptor sensitivity was different among each phase (77, 60, and 26 times for the control, acute, and acidosis phases, respectively).
Decreased baroreflex sensitivity has been documented in critically ill adults with organ dysfunction and in animal models of sepsis [Citation26,Citation27]. However, the mechanism that decreases fetal baroreceptor sensitivity during fetal deterioration induced by LPS is unclear. One possible explanation is the collapse of the ANS. In response to severe infections and other injuries, the central nervous system activates the sympathetic branch of the ANS to make the necessary physiological and metabolic adjustments to overcome the acute physiologic stress [Citation28]. Therefore, a decrease in baroreceptor sensitivity due to the disruption of the hypothalamic-pituitary-adrenal axis is a common feature in severe sepsis. It is now recognized that, in sepsis, adrenal insufficiency partly accounts for a reduced vascular sensitivity to vasopressors [Citation29].
The other possible explanation is the failure of a cholinergic anti-inflammatory response via the parasympathetic nervous system, resulting in a shallow FHR deceleration. The parasympathetic nerves facilitate two important biological defense mechanisms during stressful conditions: (1) a tonic influence that slows the heart rate is purported, which is a cardiovascular response to the myocardial function or oxygenation requirements. (2) The parasympathetic nervous system exerts an oscillatory influence that results in increased FHR variability, which is a side effect of the anti-inflammatory response [Citation30]. Previously, we reported that FHR variability increases along with fetal deterioration in an in utero sheep infection model as a result of a cholinergic anti-inflammatory response via the parasympathetic nervous system that results in a decreased FHR variability toward the end [Citation13]. Therefore, in the present study, another potential explanation for the significant decrease in baroreceptor sensitivity seen during the acidosis phase could be the dysfunction of the parasympathetic nervous system. However, the possibility exists that the MAP sensitivity to phenylephrine had not changed in response to the LPS. Usually, a septic condition is accompanied by a drop in MAP. One reason that makes it difficult to discuss the mechanism is the absence of absolute MAP data that accounts for maternal position or intrauterine pressure, although we have relative MAP data in this study. Further investigations using vasopressin or parasympathetic blockades with atropine during endotoxemia-related fetal acidosis may help to elucidate the pathophysiology of the decrease in fetal baroreceptor sensitivity.
Clinically, a deep FHR deceleration pattern in fetal cardiotocography (CTG), which is mainly mediated by baroreceptor sensitivity, needs attention because it is thought to be related to an increase in the risk of fetal acidemia [Citation8,Citation9,Citation31]. Based on the FHR pattern, intrapartum management is recommended [Citation32,Citation33]. However, our results show that intrauterine infections induced by LPS decrease fetal baroreceptor sensitivity, indicating that when the fetus develops fetal acidosis due to intrauterine inflammation, actual fetal CTG pattern could show a smaller FHR deceleration than expected. Therefore, decelerations during infection must be interpreted with caution because severe infections that result in fetal acidosis may modify decelerations, leading to the underestimation of the actual fetal condition.
The present study had limitations. First, we did not measure fetal inflammatory cytokines, such as interleukin (IL)-6, IL-10, and tumor necrosis factor (TNF)-α. These data may strengthen the argument for the correlation between fetal baroreceptor sensitivity and fetal inflammatory cytokine response. Second, because we collected biological sample after the delivery, pathological lesions, especially in the fetal membrane, may have been affected by maternal inflammation response [Citation34]. Third, because we tried to reduce the number of experimental sheep for ethical reasons, we used the same sheep as controls. Finally, the clinical utility of the findings is debatable because it may be argued that, in most cases, the FHR pattern is in a benign state, while this study represents an extreme case.
In conclusion, we found severe intrauterine inflammation induced by LPS impaired the fetal baroreceptor sensitivity in chronically instrumented sheep. This finding suggests that intrauterine inflammation may modify the intrapartum FHR deceleration and could lead to the underestimation of the actual fetal condition. Our findings could guide maternal intrapartum management in cases with intrauterine inflammation.
Supplementary Material
Download Zip (438.3 KB)Acknowledgements
We would like to thank Hideki Chiba MD, PhD, for providing the pathological analysis.
Disclosure statement
The authors declare that they have no conflict of interest.
Data availability statement
The data that support the findings of this study are available from the corresponding author, H.K., upon reasonable request.
Additional information
Funding
References
- Nelson KB, Willoughby RE. Infection, inflammation and the risk of cerebral palsy. Curr Opin Neurol. 2000;13(2):133–139.
- Rouse DJ, Landon M, Leveno KJ, et al. The maternal– fetal medicine units cesarean registry: chorioamnionitis at term and its duration-relationship to outcomes. Am J Obstet Gynecol. 2004;191(1):211–216.
- Lau J, Magee F, Qiu Z, et al. Chorioamnionitis with a fetal inflammatory response is associated with higher neonatal mortality, morbidity, and resource use than chorioamnionitis displaying a maternal inflammatory response only. Am J Obstet Gynecol. 2005;193(3 Pt 1):708–713.
- Wu YW, Colford JM Jr. Chorioamnionitis as a risk factor for cerebral palsy: a meta-analysis. JAMA. 2000;284(11):1417–1424.
- Blackwell S, Romero R, Chaiworapongsa T, et al. Maternal and fetal inflammatory responses in unexplained fetal death. J Matern Fetal Neonatal Med. 2003;14(3):151–157.
- Nelson KB, Grether JK. Potentially asphyxiating conditions and spastic cerebral palsy in infants of normal birth weight. Am J Obstet Gynecol. 1998;179(2):507–513.
- Peebles DM, Wyatt JS. Synergy between antenatal exposure to infection and intrapartum events in causation of perinatal brain injury at term. BJOG. 2002;109(7):737–739.
- Kubli FW, Hon EH, Khazin AF, et al. Observations on heart rate and pH in the human fetus during labor. Am J Obstet Gynecol. 1969;104(8):1190–1206.
- Paul RH, Suidan AK, Yeh S, et al. Clinical fetal monitoring. VII. The evaluation and significance of intrapartum baseline FHR variability. Am J Obstet Gynecol. 1975;123(2):206–210.
- Lear CA, Kasai M, Booth LC, et al. Peripheral chemoreflex control of fetal heart rate decelerations overwhelms the baroreflex during brief umbilical cord occlusions in fetal sheep. J Physiol. 2020;598(20):4523–4536.
- Segar JL. Ontogeny of the arterial and cardiopulmonary baroreflex during fetal and postnatal life. Am J Physiol. 1997;273(2 Pt 2):R457–R471.
- Kyozuka H, Yasuda S, Hiraiwa T, et al. Histological chorioamnionitis as a risk factor for preterm birth without disturbing fetal heart rate: a case-control study. Tohoku J Exp Med. 2017;243(4):289–295.
- Kyozuka H, Yasuda S, Hiraiwa T, et al. The change of fetal heart rate short-term variability during the course of histological chorioamnionitis in fetal sheep. Eur J Obstet Gynecol Reprod Biol. 2018;228:32–37.
- Yasuda S, Kyozuka H, Nomura Y, et al. Effect of magnesium sulfate on baroreflex during acute hypoxemia in chronically instrumented fetal sheep. J Obstet Gynaecol Res. 2020;46(7):1035–1043.
- Coleman TG. Arterial baroreflex control of heart rate in the conscious rat. Am J Physiol. 1980;238(4):H515–H520.
- Salafia CM, Weigl C, Silberman L. The prevalence and distribution of acute placental inflammation in uncomplicated term pregnancies. Obstet Gynecol. 1989;73(3 Pt 1):383–389.
- Navarro C, Blanc WA. Subacute necrotizing funisitis. A variant of cord inflammation with a high rate of perinatal infection. J Pediatr. 1974;85(5):689–697.
- Holcroft CJ, Askin FB, Patra A, et al. Are histopathologic chorioamnionitis and funisitis associated with metabolic acidosis in the preterm fetus? Am J Obstet Gynecol. 2004;191(6):2010–2015.
- Ugwumadu A. Infection and fetal neurologic injury. Curr Opin Obstet Gynecol. 2006;18(2):106–111.
- Ashwood ER, Kost G, Kenny M. Temperature correction of blood-gas and pH measurements. Clin Chem. 1983;29(11):1877–1885.
- Badke CM, Marsillio LE, Weese-Mayer DE, et al. Autonomic nervous system dysfunction in pediatric sepsis. Front Pediatr. 2018;6:280.
- Fletcher AJW, Gardner DS, Edwards CMB, et al. Development of the ovine fetal cardiovascular defense to hypoxemia towards full term. Am J Physiol Heart Circ Physiol. 2006;291(6):H3023–H3034.
- Green LR, Kawagoe Y, Homan J, et al. Adaptation of cardiovascular responses to repetitive umbilical cord occlusion in the late gestation ovine fetus. J Physiol. 2001;535(Pt 3):879–888.
- Pulgar VM, Zhang J, Massmann GA, et al. Mild chronic hypoxia modifies the fetal sheep neural and cardiovascular responses to repeated umbilical cord occlusion. Brain Res. 2007;1176:18–26.
- Wakatsuki A, Murata Y, Ninomiya Y, et al. Physiologic baroreceptor activity in the fetal lamb. Am J Obstet Gynecol. 1992;167(3):820–827.
- Schmidt H, Müller-Werdan U, Hoffmann T, et al. Autonomic dysfunction predicts mortality in patients with multiple organ dysfunction syndrome of different age groups. Crit Care Med. 2005;33(9):1994–2002.
- Pancoto JA, Corrêa PB, Oliveira-Pelegrin GR, et al. Autonomic dysfunction in experimental sepsis induced by cecal ligation and puncture. Auton Neurosci. 2008;138(1–2):57–63.
- Sharshar T, Hopkinson NS, Orlikowski D, et al. Science review: the brain in sepsis – culprit and victim. Crit Care. 2005;9(1):37–44.
- Annane D, Bellissant E, Sebille V, et al. Impaired pressor sensitivity to noradrenaline in septic shock patients with and without impaired adrenal function reserve. Br J Clin Pharmacol. 1998;46(6):589–597.
- Lear CA, Davidson JO, Galinsky R, et al. Subclinical decelerations during developing hypotension in preterm fetal sheep after acute on chronic lipopolysaccharide exposure. Sci Rep. 2015;5:16201.
- Okai T, Ikeda T, Kawarabayashi T, et al. Intrapartum management guidelines based on fetal heart rate pattern classification. J Obstet Gynaecol Res. 2010;36(5):925–928.
- American College of Obstetricians and Gynecologists. Practice bulletin no. 116: management of intrapartum fetal heart rate tracings. Obstet Gynecol. 2010;116:1232–1240.
- Clark SL, Nageotte MP, Garite TJ, et al. Intrapartum management of category II fetal heart rate tracings: towards standardization of care. Am J Obstet Gynecol. 2013;209(2):89–97.
- Kyozuka H, Murata T, Fukuda T, et al. Labor dystocia and risk of histological chorioamnionitis and funisitis: a study from a single tertiary referral center. BMC Pregnancy Childbirth. 2021;21(1):263.