Abstract
Objectives
Fetal death is a complication of pregnancy caused by multiple etiologies rather than being the end-result of a single disease process. Many soluble analytes in the maternal circulation, such as hormones and cytokines, have been implicated in its pathophysiology. However, changes in the protein content of extracellular vesicles (EVs), which could provide additional insight into the disease pathways of this obstetrical syndrome, have not been examined. This study aimed to characterize the proteomic profile of EVs in the plasma of pregnant women who experienced fetal death and to evaluate whether such a profile reflected the pathophysiological mechanisms of this obstetrical complication. Moreover, the proteomic results were compared to and integrated with those obtained from the soluble fraction of maternal plasma.
Methods
This retrospective case-control study included 47 women who experienced fetal death and 94 matched, healthy, pregnant controls. Proteomic analysis of 82 proteins in the EVs and the soluble fractions of maternal plasma samples was conducted by using a bead-based, multiplexed immunoassay platform. Quantile regression analysis and random forest models were implemented to assess differences in the concentration of proteins in the EV and soluble fractions and to evaluate their combined discriminatory power between clinical groups. Hierarchical cluster analysis was applied to identify subgroups of fetal death cases with similar proteomic profiles. A p-value of <.05 was used to infer significance, unless multiple testing was involved, with the false discovery rate controlled at the 10% level (q < 0.1). All statistical analyses were performed by using the R statistical language and environment-and specialized packages.
Results
Nineteen proteins (placental growth factor, macrophage migration inhibitory factor, endoglin, regulated upon activation normal T cell expressed and presumably secreted (RANTES), interleukin (IL)-6, macrophage inflammatory protein 1-alpha, urokinase plasminogen activator surface receptor, tissue factor pathway inhibitor, IL-8, E-Selectin, vascular endothelial growth factor receptor 2, pentraxin 3, IL-16, galectin-1, monocyte chemotactic protein 1, disintegrin and metalloproteinase domain-containing protein 12, insulin-like growth factor-binding protein 1, matrix metalloproteinase-1(MMP1), and CD163) were found to have different plasma concentrations (of an EV or a soluble fraction) in women with fetal death compared to controls. There was a similar pattern of change for the dysregulated proteins in the EV and soluble fractions and a positive correlation between the log2-fold changes of proteins significant in either the EV or the soluble fraction (ρ = 0.89, p < .001). The combination of EV and soluble fraction proteins resulted in a good discriminatory model (area under the ROC curve, 82%; sensitivity, 57.5% at a 10% false-positive rate). Unsupervised clustering based on the proteins differentially expressed in either the EV or the soluble fraction of patients with fetal death relative to controls revealed three major clusters of patients.
Conclusion
Pregnant women with fetal death have different concentrations of 19 proteins in the EV and soluble fractions compared to controls, and the direction of changes in concentration was similar between fractions. The combination of EV and soluble protein concentrations revealed three different clusters of fetal death cases with distinct clinical and placental histopathological characteristics.
Introduction
Fetal death occurs in six per 1000 deliveries in the United States [Citation1], and it is twice more common in African-American women than in Caucasian women [Citation1,Citation2]. In 2019, two million fetuses died during the third trimester of pregnancy [Citation3]. Fetal death is an obstetrical syndrome caused by multiple etiologies rather than the end-result of a single disease process [Citation4–11]. Although our understanding of the potential etiologies of fetal death has improved [Citation12], 25% to 60% of cases are still classified as “unexplained” [Citation4,Citation13–18], either for the lack of sufficient information or of the limitations in the available diagnostic tools [Citation19].
The identification of biomarkers for the accurate and early prediction or diagnosis of pregnancy complications is important for the improvement of obstetrical care. Proteins in maternal plasma play a role in the characterization and pathogenesis of the great obstetrical syndromes [Citation20–37], and changes in their concentrations—in particular, for angiogenic and antiangiogenic factors—have been well characterized in cases of fetal death [Citation38–44]. Until recently, the regulatory activity of these proteins was considered to be mediated via soluble autocrine [Citation45–49], paracrine [Citation45,Citation47,Citation49], and endocrine [Citation50–52] signaling pathways through the direct binding of cell surface receptors. However, the maternal-fetal dialogue is now recognized to be a more complex phenomenon in which EVs are also considered to be mediators in the cross-talk between the feto-placental unit and the mother [Citation53–61]. EVs are microparticles secreted by cells into the extracellular space [Citation57,Citation62] surrounded by a lipid membrane, and carrying proteins and nucleic acids [Citation63], which can have angiogenic-antiangiogenic, immune-regulatory, growth regulatory and other properties [Citation64–69]. EVs represent a cargo system that delivers packaged intercellular messengers to specific cells more efficiently than by the release of free molecules into the extracellular space. Being delivered intracellularly these messengers can alter cell physiology. Also, the release of vesicle cargos in the close vicinity of target cells would create a high surface concentration of messengers even with a small number of released molecules [Citation70]. Therefore, deciphering the protein content of EVs could provide further insight into the pathophysiology of pregnancy complications [Citation71].
The aim of this study was to characterize the protein profile of EVs in the maternal plasma of women who had fetal death and to evaluate if this profile could reflect the pathophysiological mechanisms implicated in this obstetrical syndrome. Moreover, the results based on molecular signals associated with EVs and those in the soluble fraction of maternal plasma were integrated for the discrimination between cases of fetal death and controls.
Material and methods
Study population
This retrospective case-control study was conducted at the Center for Advanced Obstetrical Care and Research of the Perinatology Research Branch of the Eunice Kennedy Shriver National Institute of Child Health and Human Development (NICHD), the Detroit Medical Center, and Wayne State University (Detroit, MI, USA). A total of 141 women were included in the study: 47 women with fetal death (cases) and 94 matched controls. Case matching was based on maternal characteristics (age, race, parity, body mass index, fetal sex, and gestational age at venipuncture). Cases of fetal death included those complicated by preeclampsia (PE) (n = 16), small for gestational age (SGA) (n = 16), clinical chorioamnionitis (n = 6), and placental abruption (n = 2), alone or in combination. The two placenta abruption cases were among those complicated also by preeclampsia. Patients presenting with congenital and/or chromosomal abnormalities, intrapartum fetal death, or miscarriage at <20 weeks of gestation were excluded from this study.
Maternal blood samples were collected in tubes containing ethylenediaminetetraacetic acid during prenatal care visits or at the time of diagnosis for fetal death. Plasma was separated by centrifugation and samples were stored at −80 °C until use. For five of the 47 fetal death cases, the sample was taken prior to diagnosis (between one week and five weeks). All patients provided written informed consent prior to the collection of samples. The use of clinical databases and biological samples was approved by the Institutional Review Boards of NICHD and Wayne State University.
Clinical definitions
Gestational age (GA) was determined by the last menstrual period and confirmed by ultrasound examination, or solely by the ultrasound crown-rump length measurement in the first trimester of pregnancy if the sonographic determination of gestational age was inconsistent with menstrual dating [Citation72].
Antepartum fetal death was defined as death diagnosed at ≥20 weeks of gestation and confirmed by ultrasound examination prior to the onset of labor [Citation73].
Small for gestational age was defined as a neonate with a birthweight of <10th percentile for gestational age at delivery according to a U.S. reference population [Citation74].
Preeclampsia was defined as new-onset high blood pressure after 20 weeks of gestation (systolic blood pressure of ≥140 mmHg and/or diastolic blood pressure of ≥90 mmHg, measured at least twice, 4 h to 1 week apart) and proteinuria (≥300 mg protein in 24-h urine, or two urine samples with ≥1+ test strip readings 4 h to 1 week apart, or one sample with a 2+ test strip reading) [Citation75]. Preterm- and term PE were defined according to GA at delivery (before or after 37 weeks gestation) [Citation75].
Placental histopathological examination
Placental histopathological information was available for 43 of the 47 patients with fetal death. Placental tissues were collected after delivery and examined by perinatal pathologists blinded to clinical diagnoses, according to standardized protocols [Citation76,Citation77]. Placental lesions, including maternal vascular malperfusion (MVM), were diagnosed based on criteria established by the Perinatal Section of the Society for Pediatric Pathology [Citation78] and on terminology consistent with the recommendation by the Amsterdam Placental Workshop Group consensus statement [Citation79].
Quantification of proteins in extracellular vesicles and soluble fractions
A total of 82 proteins (Supplementary Tables 1-4) in the EVs and soluble fractions of maternal plasma samples were analyzed with bead-based multiplexed platforms (Luminex Corporation, Austin, TX, USA) as described in previous publications [Citation70,Citation80,Citation81]. In brief, maternal blood samples processed for plasma were defrosted and centrifuged twice at 3000 g for 15 min: the first time to recover plasma and the second time to remove platelets. Next, platelet-poor plasma samples were treated with ExoQuickTM (System Biosciences, SBI, Palo Alto, CA, USA) to sediment the EVs. In total, 125 μl of plasma were incubated with 31.5 μl of ExoQuick™ for 30 min at 4 °C, then centrifuged at 1500g for 30 min to separate the EV-depleted supernatant from the EV-enriched pellet. The EV-depleted supernatant (soluble fraction) was collected for the quantification of proteins present as EV-free form in maternal blood samples. The EV-enriched pellet was centrifuged again at 1500 g for 5 min, residual supernatant was removed, and the pellet was resuspended into the original volume of 125 μl with sterile phosphate-buffered saline (PBS) and stored until use for the measurement of EV-associated proteins. Subsequently, four in-house multiplexed bead-based assays (panels 1–4) were used to quantify the concentrations of proteins in the EV-free (soluble) and EV-associated fractions. All soluble and EV fractions for panels 1–3 were diluted 1:3 in plasma diluent (ProcartaPlexTM Platinum Assay Buffer for Plasma Samples, ThermoFisher, Waltham, MA, USA) and 100 μg/mL Heteroblock (Omega Biologicals, Bozeman, MT, USA) and measured in duplicate. Panel 4 was run separately with samples diluted 1:30, 1:100, and 1:300 in 0.2% bovine serum albumin in PBS. A control sample of plasma and plasma EVs was run on each plate to ensure consistency between plate measurements. The EV samples were lysed with a final concentration of 0.1% Triton-X to allow measurement of surface and cargo proteins. Standards were run in duplicate in a 1:2 solution of SeraSub (CST Technologies, Great Neck, NY, USA) and plasma diluent, either without Triton-X (for soluble proteins) or with Triton-X (for EV proteins). Luminex beads coupled with specific antibodies were added to samples and standards, and plates were incubated overnight at 4 °C. Plates were washed, incubated with biotinylated secondary antibodies for 1 h at room temperature, washed, incubated with 12 μg/mL of Streptavidin-phycoerythrin (Thermo Fisher) for 30 min at room temperature, washed, and resuspended in 70 μl of PBS. Measurements were performed on Luminex 200 (Luminex) with the acquisition of 100 beads per region and analyzed with BioPlex Manager software (BioRad, Hercules, CA, USA). Protein concentrations were determined using 5 P regression algorithms. Seven proteins, i.e. Activin A; chemokine (C-X3-C motif) ligand 1 (CX3CL1); granulocyte-macrophage colony-stimulating factor (GM-CSF); IL-2; IL-13; macrophage inflammatory protein-3 (MIP3α); and transforming growth factor beta-3 (TGFβ3), were detected in less than 20% of the samples and hence were excluded from the analyses.
Statistical analyses
Demographics data analysis
Demographic and clinical variables were compared between the fetal death and control groups using Fisher’s exact and Wilcoxon signed rank tests, depending on whether the variable to be assessed was categorical or continuous. Summaries of the data were reported as frequency (%) or as median (interquartile range, IQR).
Comparisons of extracellular vesicle and soluble fraction protein concentrations
Quantile regression was used to analyze differences in the concentration of proteins in the EVs and in the soluble fractions between the fetal death and the control groups. An important feature of quantile regression is its robustness to outliers and the non-normality of the data distribution. Results are presented as log2 fold changes between groups while adjusting for gestational age at blood sample collection.
Discrimination analysis
Random forest models were implemented to assess the discrimination between groups based on protein data in the soluble fractions or a combination of concentrations in the soluble fractions and EVs. The mean decrease in the Gini index was calculated to provide a measure of variable importance in the random forest models while accounting for the complex structure of correlations and interactions in the multivariate dataset [Citation82–84]. The area under the receiver operating characteristic (ROC) curve (AUC), obtained through leave-one-out cross-validation, was used to determine discrimination accuracy. All of the statistical analyses were performed by using the R statistical language and the environment with specialized software packages. When multiple comparisons were involved in the analysis, p values were corrected by the Benjamini & Hochberg method, and the statistical significance was evaluated based on the corrected p value (q < 0.1).
Cluster analysis
Hierarchical clustering based on the proteins, significant in either the EVs or the soluble fractions in women with fetal death, was performed to uncover subgroups of patients with fetal death. Euclidean distance was used as a dissimilarity metric, followed by Ward’s minimum variance criteria to establish the clusters [Citation85,Citation86]. A heatmap, generated by the ComplexHeatmap package in R/Bioconductor [Citation87], visualized the clusters.
Both the discrimination and cluster analyses were performed based on the protein data expressed as multiple of the median (MoM) values for gestational age among the controls. Patients with fetal death were dichotomized into two groups according to the number of placental villous changes and vascular lesions (less than 2 and 2 or more features) consistent with MVM.
Results
Demographic and clinical characteristics
summarizes the demographic and clinical information of the study population. A significant difference was observed for gestational age at delivery and birthweight for fetal death cases compared to controls. However, by design, there was no difference between any other maternal characteristics shown in .
Table 1. Demographic and clinical characteristics of the study population.
EV-associated and soluble fraction proteins
The results of the differential concentration analysis in the EVs and soluble fractions between cases of fetal death and controls are reported in Supplementary Figure 1 and Supplementary Table 1. Of the 75 proteins analyzed, 19 proteins in the EV or soluble fractions had different plasma concentrations in women with fetal death compared to controls. Among them, eight were significant in the EV and soluble fractions, two in the EV fraction only, and nine in the soluble fraction only (). The log2-fold changes for these 19 proteins between fetal death cases and controls are reported in , which shows a similar pattern of change for the dysregulated proteins in the EV and soluble fractions. There was also a positive correlation between the log2-fold changes of all proteins significant in either the EV or the soluble fraction (ρ = .89, p < .001) (). Since one fetal death patient and 10 controls were in labour at the time of the blood draw, we performed a sensitivity analysis in which these patients were excluded from the analysis. The findings reported above remained true regarding protein differences between fetal death and controls in EV faction, while for the soluble fraction analysis, three additional proteins were found to increase in fetal death [MMP9 (q = 0.066), GROa (q = 0.025), and MMP1 (q = 0.089)]. These three proteins had adjusted p-values (q) in the 0.10-0.11 range in the original analysis and hence were not deemed significant in the original analysis. We have therefore concluded that the labor status at the time of sampling was not a confounding factor of the proteomic changes we have attributed to fetal death.
Figure 1. Log2-fold changes of 19 proteins in fetal death vs controls in the soluble and extracellular vesicle compartments of maternal plasma.
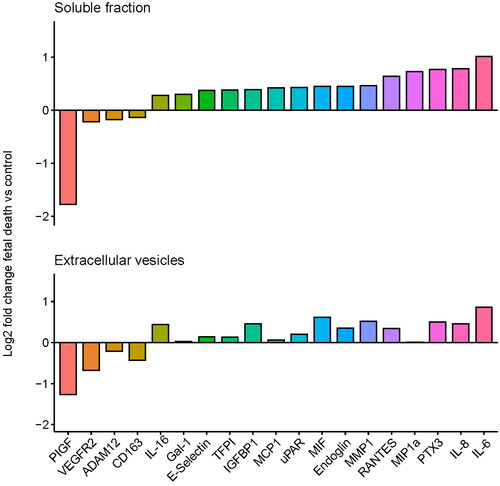
Figure 2. Correlation between the log2-fold changes of all proteins is significant in either the extracellular vesicles or soluble fractions.
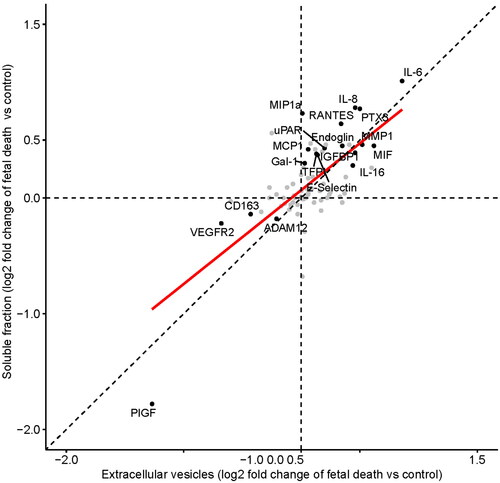
Table 2. Proteins with significantly different plasma concentrations in women with fetal death compared to controls from both EVs and/or soluble fractions.
The 19 proteins listed in are implicated in functions and processes such as angiogenesis/anti-angiogenesis (endoglin, PlGF, VEGFR2), blood coagulation (TFPI, uPAR), inflammatory response (CD163, E-Selectin, Gal-1, IL-6, IL-8, IL-16, MCP1, MIF, MIP1α, PTX3, RANTES), regulation of cell migration, and extracellular matrix remodeling (ADAM12, IGFBP1, MMP1).
Comparison of cases of fetal death with and without preeclampsia
Among women with fetal death, 16 patients developed preeclampsia in the index pregnancy while 31 did not. We found five proteins (CXCL6, endoglin, MIP3β, PlGF, and TFPI) to be differentially expressed between the two subgroups (). Concentrations of PlGF were lower in the EV and soluble fractions of fetal death cases related to preeclampsia, and the other four proteins had higher concentrations only in the soluble fraction in this subset of women.
Unsupervised clustering of cases with fetal death
Among cases of fetal death, unsupervised clustering—based on the proteins differentially expressed in either the EV or soluble fractions of patients with fetal death relative to controls—revealed three major clusters ( and Supplementary Table 5).
Cluster 1 included 19 cases of fetal death that had 33 differentially expressed proteins in either the EV or the soluble fraction. Of these, seven proteins were altered in both fractions (ADAM12, endoglin, IL-16, PlGF, PTX3, VEGFR1, VEGFR2), three proteins only in the EV fraction (CD163, fibronectin, thrombomodulin), and 16 only in the soluble fraction (E-Selectin, Gal-1, IGFBP1, IL-6, IL-8, IL-18, MCP1, MIF, MIG, MMP9, RANTES, TFPI, TIMP1, TLR2, TNFα, and uPAR). Of note, 68% (13/19) of cases of fetal death included in Cluster 1 had two or more features consistent with MVM, and 53% (10/19) had preeclampsia. The increase of VEGFR1 and decrease of PlGF in this cluster of fetal death cases was expected since PlGF and VEGFR1 are not only markers of fetal death and preeclampsia, but are also known to be associated with the presence of placental lesions consistent with vascular malperfusion, which are common in most obstetrical syndromes.
Cluster 2 contained 12 cases of fetal death, and only PDL1 in the soluble fraction had different concentrations in this subgroup compared to controls. MVM lesions with two or more features were observed in 16% (2/12) of the patients in this cluster, and one patient had preeclampsia.
Cluster 3 included 16 cases of fetal death. Among these patients, 18.8% (3/16) had two or more features consistent with MVM, and 31.3% (5/16) had preeclampsia. A total of 21 proteins were differentially expressed in either the EV or the soluble fraction of patients in this cluster compared to the controls. Four proteins were altered in both fractions (IL-6, IL-7, IL-17, and MMP1), one was changed only in the EV fraction (IFNL), and 15 only in the soluble fraction (e.g. IL-8, IP-10, MMP1, RANTES). Of note, the fraction of unexplained fetal death cases was the lowest in Cluster 1: 16% (3/19), followed by Cluster 3: 50% (8/16), and was the highest in Cluster 2: 66% (8/12). The latter cluster showed the least dysregulation in the proteomic profiles relative to controls. This suggests that this group may be representative of unexplained fetal death, which in general accounts for 25% to 60% of fetal deaths.
EV-associated and soluble fraction protein concentrations discriminate fetal death from controls
The leave-one-out cross-validation method was used to calculate the AUC for discriminating fetal death from controls firstly based on the protein concentrations in the EV fraction. The AUC was 76% (95% confidence interval (CI), 67–85%; ) with a sensitivity of 38.3% at a 10% false-positive rate (FPR). The combination of proteins from the EV and soluble fractions resulted in improved discrimination (AUC, 82%; 95% CI, 74–90%; 57.5% sensitivity at a 10% FPR). provides the Gini index scores for the top 20 proteins involved in the discrimination of fetal death. Of interest, while accounting for the information provided by all proteins considered, the top 20 most relevant predictors included five EV-associated proteins, with PlGF as the top predictor in the soluble and EV fractions.
Discussion
Principal findings of this study
(1) This study includes the first characterization of EV protein profile in the maternal blood of women with pregnancies complicated with fetal death. (2) Out of eighty-two proteins measured in the EV and soluble fractions nineteen were found to have different concentrations in cases of fetal death compared to controls and the direction of changes in concentrations was similar in the EV and soluble fractions. (3) The differentially expressed proteins are implicated in functions and processes such as angiogenesis/anti-angiogenesis, blood coagulation, inflammatory response, regulation of cell migration and extracellular matrix remodelling. (4) The top discriminating features between fetal death cases and controls included both EV and soluble fraction proteins, suggesting that the EV fraction provides independent information. (5) The combination of soluble and EV protein concentrations revealed three separate clusters of women with fetal death who had distinct clinical and placental histopathological characteristics.
Results in the context of what is known
EVs are cell-derived microparticles released into the extracellular space [Citation63]. The secretion of EVs was initially described as a way to eliminate unnecessary compounds from the cells [Citation88] however, it is now clear that EVs are implicated in exchanging components (proteins, nucleic acids and lipids) between cells and that they act as signalling vesicles in both physiologic and pathologic processes [Citation57,Citation63,Citation89]. EVs are suggested as essential modulators of multiple processes of pregnancy, including implantation, migration and invasion of trophoblasts, and cellular adaptations to physiological changes [Citation90–99]. Abnormal changes of EV concentration and composition are involved in pregnancy complications, including preeclampsia (PE) [Citation100–105], gestational diabetes mellitus (GDM) [Citation106–110], preterm labor and preterm premature rupture of membranes (pPROM) leading to preterm birth (PTB) [Citation80,Citation111–115]. However, the proteomic profile of EVs has not been reported in fetal death yet. In this study, we performed the first proteomic analysis of the EV-associated and soluble fractions of maternal plasma of women who had fetal death, demonstrating that 19 proteins in the EV and soluble fractions were different in concentrations compared to controls.
Although in general, the direction of changes in concentration was similar in the EV and soluble fractions, a discrimination analysis suggested that the EVs’ proteome might contribute with independent information. Angiogenesis/anti-angiogenesis, blood coagulation, inflammatory response, regulation of cell migration and extracellular matrix remodeling were functions and processes related to the significantly different proteins.
There is a compelling body of evidence showing that an abnormal profile of angiogenic and antiangiogenic factors is present at the time and before the diagnosis of fetal death [Citation38–41,Citation116–118]. In conjunction, placental lesions consistent with maternal vascular malperfusion are known to be pathogenically linked to fetal death as well as preeclampsia or SGA [Citation6,Citation119]. Our results are consistent with these previous findings as cluster 1 of women with fetal death is characterized by lower concentrations of angiogenic PlGF, VEGFR2 as well as higher concentrations of anti-angiogenic endoglin [Citation30,Citation120–129] in both the EV and soluble fractions in patients with fetal death compared to controls. Moreover, we found preeclampsia in more than 50% of the cases in this cluster, and almost 70% of them had more than two MVM lesions at placental histology. Concentration changes of angiogenic and anti-angiogenic factors in the EV and soluble fractions seem to reflect the burden of uteroplacental vasculopathy indicated by histologic examination of the placenta and identified the most severe spectrum of this obstetrical syndrome. While fetal vascular malperfusion was also found in 32% (15/45) of fetal death cases, the interpretation of such lesions in fetal death placentas requires caution because they may reflect the consequence rather than the cause of fetal death [Citation6].
Intravascular inflammation has also been implicated in fetal death [Citation130,Citation131]. Indeed, proinflammatory proteins such as C5a, E-selectin and P-selectin [Citation132,Citation133], have been reported to have higher maternal plasma concentrations in women who had fetal death compared to those with normal pregnancy [Citation20,Citation44]. Consistently, our results showed that E-selectin concentrations were higher in the soluble fraction in patients with fetal death. We also identified a subset of patients in clusters 1 and 3 who had fetal death with a pro-inflammatory profile.
Increased IL-6 concentrations in maternal plasma has been implicated in several obstetrical syndromes [Citation134–137]. Indeed, IL-6 is a major mediator of the host response to infection and tissue damage associated with intra-amniotic inflammation [Citation138–140], spontaneous preterm delivery [Citation141,Citation142], acute placental inflammatory lesions [Citation143], and adverse neonatal outcomes such as cerebral palsy [Citation144]. However, this is the first study reporting the increased concentrations of IL-6 in fetal death, which underlines a proinflammatory proteomic profile in the maternal circulation in at least a subset of patients.
In accord, higher plasma concentrations of chemotactic proteins such as IL-8 (CXCL8), MCP1 (CCL2) and RANTES were seen in both soluble and EV fractions in women who had fetal death compared to normal pregnancies. These proteins are well-known markers of pro-inflammatory state in pregnancy [Citation145,Citation146] and are involved in the recruitment of neutrophils [Citation147–149] monocytes [Citation150,Citation151] and eosinophils [Citation152].
PTX3, a soluble pattern recognition receptor (sPRR) [Citation153,Citation154], acts as an acute phase response protein and has elevated concentrations in plasma of patients with various pregnancy complications such as preeclampsia [Citation155–159], HELLP syndrome [Citation159], preterm birth [Citation35,Citation160] and fetal growth restriction [Citation155,Citation156,Citation161]. This is the first time that PTX3 is associated with fetal death.
Galectin-1, a glycan-binding protein expressed by the placenta, fetal membranes and decidua [Citation162,Citation163] acts as an alarmin [Citation164–166], an endogenous danger signal secreted by damaged cells, which can regulate the immune and inflammatory response [Citation167]. An increased placental expression and maternal serum concentrations of galectin-1 have been reported in severe preeclampsia and HELLP syndrome [Citation164,Citation168,Citation169]. Our results have shown that the upregulation of galectin-1 in fetal death may be an additional sign of inflammation and danger signaling.
Plasma concentrations of tissue factor pathway inhibitor (TFPI) and urokinase plasminogen activator surface receptor (uPAR) were also increased in women who had fetal death, and this may reflect dysregulation at the cross-roads of inflammation and coagulation. TFPI is a protease inhibitor involved in the downregulation of the tissue factor (TF)-dependent coagulation cascade (i.e. extrinsic coagulation pathway) [Citation170–172], which can have a protective role in disseminated intravascular coagulation (DIC) [Citation173,Citation174]. Elevated levels of TFPI have been found in patients with sepsis-induced DIC [Citation175–179] in association with elevated TF levels [Citation180,Citation181] suggesting a relative deficiency of TFPI to neutralize TF pathway activation. uPAR was one of the proteins with the top discriminative scores (Gini index) in fetal death. In vivo studies have demonstrated that uPAR may play an important role in trophoblast invasion and angiogenesis during early implantation [Citation182], however, the pathophysiological role of this protein in fetal death is unknown.
We also found dysregulated expression of proteins with matrix remodeling properties in cases of fetal death compared to controls, such as MMP1 and ADAM12. Beyond matrix remodeling, metalloproteinases are involved in various biological processes such as the regulation of vascular reactivity, leukocyte activation [Citation183–186] and cellular interaction [Citation187]. A role for these proteases has also been reported in complications of pregnancy such as preeclampsia and fetal growth restriction [Citation149,Citation188–191]. Herein, we found increased MMP1 concentrations in the EV fraction of patients with fetal death, and this direction of change is consistent with the results of Walsh at el. who found that circulating MMP1 levels were increased in women with preeclampsia compared to controls [Citation188].
On the contrary, ADAM12 was decreased in concentrations in both the EV and soluble fractions in women who had fetal death. Similar direction of changes has also been reported in other obstetrical syndromes, including fetal growth restriction [Citation190,Citation192,Citation193] and preeclampsia [Citation191,Citation194,Citation195], suggesting that low maternal blood ADAM12 concentrations may reflect impaired placental functions.
There was an interesting group of patients in cluster 2 who had fetal death and did not have any specific altered molecular pathways. This group, which featured a single case complicated also by preeclampsia, may represent the unexplained fetal death that accounts for 25 to 60% of fetal deaths [Citation4,Citation13,Citation16].
Fetal death with and without preeclampsia
Preeclampsia is a leading cause of fetal death. Indeed, the relative risk of fetal death at the end of mid-trimester is 86-fold higher in women with preeclampsia compared to those without, and it is still more than sevenfold higher at 34 weeks of gestation [Citation196]. Since nearly one-third of our fetal death cases (16/47) originated from preeclampsia, we were interested in the distinctive proteomic profile of women with preeclampsia among cases with fetal death. We found five proteins to be different in concentrations in cases of fetal death complicated by preeclampsia compared to those without preeclampsia. Fetal death with preeclampsia is characterized by a more extreme imbalance of angiogenic/antiangiogenic factors, as reflected by the lower concentrations of PlGF and higher concentrations of endoglin compared to fetal death without preeclampsia. This imbalance in angiogenic and antiangiogenic factors reflects the presence and burden of placental vascular lesions consistent with MVM that have been proposed to be the leading cause of fetal death in the late second and third trimesters until term [Citation41]. Additionally, TFPI, CXCL6 and MIP3β were also found to be higher in the soluble fraction of women with fetal death and preeclampsia. Indeed, preeclampsia is associated with an increased placental expression [Citation197], maternal plasma concentrations and activity of TFPI and its close homologue TFPI2 [Citation42,Citation197,Citation198], Earlier we showed higher maternal plasma TFPI activity in patients with fetal death complicated with preeclampsia [Citation42], consistent with the current results. CXCL6, and MIP3β (also known as CCL19) are chemotactic factors for neutrophils and monocytes that were reported to be implicated in preeclampsia and preterm labor respectively [Citation199–203]. Overall, our data suggest that mechanisms of fetal death complicated with preeclampsia include the dysregulation of angiogenic, coagulation and inflammatory pathways.
Clinical and research implications
Models including soluble maternal blood biomarkers such as pregnancy-associated plasma protein A (PAPP-A) and PlGF have been proposed for the prediction of fetal death [Citation204–206], however, we still lack sensitive methods for early screening. Biomarkers from EVs could improve the prediction of this obstetrical syndrome. Indeed, this study revealed that nineteen proteins in the EV and soluble fractions of maternal plasma have different concentrations in cases of fetal death, EV and soluble fractions provide independent information, and the combination of information from these two fractions increases the detection rate. Studies using high-throughput omics technologies may provide even more comprehensive insights into the pathophysiology of fetal death and the role of EVs as the source of biomarkers.
Strengths and limitations
This is the first study to characterize the proteomic profile of EV and soluble fractions in maternal plasma in women who had fetal death. Systematically collected fetal and placental pathology data enabled the inclusion only of cases where fetuses were structurally normal. The number of fetal death cases included is rather large considering the prevalence of this outcome in the general population. The proteins measured (n = 82) were selected based on existing customized panels and relevance to the condition under the study, yet higher-throughput studies will be needed to identify disease-specific EV-associated biomarkers. The robust analysis using quantile regression and the use of cross-validation are important strengths. Our study of fetal death included the combination of cases with placental insufficiency associated with different obstetrical syndromes. A cohort study design and a deeper coverage of the proteome would be ideal for a more in-depth insight into the pathophysiology of fetal death and the contribution of EVs as the source of biomarkers.
Although specific proteins are associated with EVs in pregnancies complicated by fetal death, we do not know whether these proteins are carried by EVs together or are distributed among different EVs. Future analysis of individual EVs may provide this information as well as identify the cells of origin of these EVs. Furthermore, since the current study presented only data on the concentration of proteins in the soluble fraction or EV-associated, future studies will be needed to determine whether the total protein amount or the total EV content is different in the plasma of women who experience fetal death compared to controls.
Conclusions
Pregnant women with fetal death have different concentrations of nineteen proteins in the EV and soluble fractions compared to controls and the direction of changes in concentration was similar in the EV and soluble fractions. The combination of EV and soluble protein concentrations revealed three different clusters of fetal death with different clinical and placental histopathological characteristics.
Author contributions
Conceptualization, WF, RR, TC, ALT, LM; analysis, DWG, WF, NGL, DMG, NGT, ALT; visualization, DMG, WF, NGL, DWG, NGT, ALT writing—original draft, DMG, WF, RR, NGL, DWG, NGT, ALT; writing, review and editing, all authors; project administration, ALT, NGL, RR; resources and funding acquisition, RR, LM.
Supplemental Material
Download Zip (62.7 KB)Acknowledgements
The authors thank Maureen McGerty (Wayne State University) for her critical reading of the manuscript.
Disclosure statement
The authors report no potential conflicts of interest. The funders had no role in the design of the study, in the collection, analyses, or interpretation of data, in the writing of the manuscript, or in the decision to publish the results. RR has contributed to this work as part of his official duties as an employee of the United States Federal Government.
Additional information
Funding
References
- MacDorman MF, Gregory EC. Fetal and perinatal mortality: United States, 2013. Natl Vital Stat Rep. 2015;64(8):1–24.
- Willinger M, Ko CW, Reddy UM. Racial disparities in stillbirth risk across gestation in the United States. Am J Obstet Gynecol. 2009;201(5):469.e1-8–469.e8.
- Hug L, You D, Blencowe H, et al. Global, regional, and national estimates and trends in stillbirths from 2000 to 2019: a systematic assessment. Lancet. 2021;398(10302):772–785.
- Silver RM, Varner MW, Reddy U, et al. Work-up of stillbirth: a review of the evidence. Am J Obstet Gynecol. 2007;196(5):433–444.
- Pacora P, Romero R, Jaiman S, et al. Mechanisms of death in structurally normal stillbirths. J Perinat Med. 2019;47(2):222–240.
- Jaiman S, Romero R, Pacora P, Jung E, Bhatti G, Yeo L, et al. Disorders of placental villous maturation in fetal death. J Perinat Med. 2020.
- Silver RM, Saade GR, Thorsten V, et al. Factor V Leiden, prothrombin G20210A, and methylene tetrahydrofolate reductase mutations and stillbirth: the Stillbirth Collaborative Research Network. Am J Obstet Gynecol. 2016;215(4):468.e1–e17.
- Takita H, Hasegawa J, Nakamura M, et al. Causes of intrauterine fetal death are changing in recent years. J Perinat Med. 2018;46(1):97–101.
- Reddy UM, Goldenberg R, Silver R, et al. Stillbirth classification–developing an international consensus for research: executive summary of a National Institute of Child Health and Human Development workshop. Obstet Gynecol. 2009;114(4):901–914.
- Hoyert DL, Gregory EC. Cause of fetal death: data from the fetal death report, 2014. Natl Vital Stat Rep. 2016;65(7):1–25.
- Romero R. Prenatal medicine: the child is the father of the man. Prenat Neonat Med. 1996;1:8–11.
- McPherson EW. 3137 fetuses in 33 years: what we have learned from the Wisconsin stillbirth service program. Am J Med Genet. 2021;185(9):2683–2689.
- Huang DY, Usher RH, Kramer MS, et al. Determinants of unexplained antepartum fetal deaths. Obstet Gynecol. 2000;95(2):215–221.
- Frøen JF, Arnestad M, Frey K, et al. Risk factors for sudden intrauterine unexplained death: epidemiologic characteristics of singleton cases in Oslo, Norway, 1986–1995. Am J Obstet Gynecol. 2001;184(4):694–702.
- Yudkin PL, Wood L, Redman CW. Risk of unexplained stillbirth at different gestational ages. Lancet. 1987;1(8543):1192–1194.
- Causes of death among stillbirths. Jama. 2011;306:2459–2468.
- Nappi L, Trezza F, Bufo P, et al. Classification of stillbirths is an ongoing dilemma. J Perinat Med. 2016;44(7):837–843.
- Smith GC, Fretts RC. Stillbirth. Lancet. 2007;370(9600):1715–1725.
- Aminu M, Bar-Zeev S, van den Broek N. Cause of and factors associated with stillbirth: a systematic review of classification systems. Acta Obstet Gynecol Scand. 2017;96(5):519–528.
- Docheva N, Romero R, Chaemsaithong P, et al. The profiles of soluble adhesion molecules in the "great obstetrical syndromes. J Matern Fetal Neonatal Med. 2019;32(13):2113–2136.
- Chaemsaithong P, Chaiworapongsa T, Romero R, et al. Maternal plasma soluble TRAIL is decreased in preeclampsia. J Matern Fetal Neonatal Med. 2014;27(3):217–227.
- Chaiworapongsa T, Romero R, Korzeniewski SJ, et al. Maternal plasma concentrations of angiogenic/antiangiogenic factors in the third trimester of pregnancy to identify the patient at risk for stillbirth at or near term and severe late preeclampsia. Am J Obstet Gynecol. 2013;208(4):287.e1–e15.
- Crispi F, Domínguez C, Llurba E, et al. Placental angiogenic growth factors and uterine artery Doppler findings for characterization of different subsets in preeclampsia and in isolated intrauterine growth restriction. Am J Obstet Gynecol. 2006;195(1):201–207.
- Chaiworapongsa T, Espinoza J, Gotsch F, et al. The maternal plasma soluble vascular endothelial growth factor receptor-1 concentration is elevated in SGA and the magnitude of the increase relates to Doppler abnormalities in the maternal and fetal circulation. J Matern Fetal Neonatal Med. 2008;21(1):25–40.
- Maynard SE, Min JY, Merchan J, et al. Excess placental soluble fms-like tyrosine kinase 1 (sFlt1) may contribute to endothelial dysfunction, hypertension, and proteinuria in preeclampsia. J Clin Invest. 2003;111(5):649–658.
- Levine RJ, Maynard SE, Qian C, et al. Circulating angiogenic factors and the risk of preeclampsia. N Engl J Med. 2004;350(7):672–683.
- Gotsch F, Romero R, Friel L, et al. CXCL10/IP-10: a missing link between inflammation and anti-angiogenesis in preeclampsia? J Matern Fetal Neonatal Med. 2007;20(11):777–792.
- Staff AC, Braekke K, Johnsen GM, et al. Circulating concentrations of soluble endoglin (CD105) in fetal and maternal serum and in amniotic fluid in preeclampsia. Am J Obstet Gynecol. 2007;197(2):176.e1-6–176.e6.
- Baumann MU, Bersinger NA, Mohaupt MG, et al. First-trimester serum levels of soluble endoglin and soluble fms-like tyrosine kinase-1 as first-trimester markers for late-onset preeclampsia. Am J Obstet Gynecol. 2008;199(3):266.e1-6–266.e6.
- Chaiworapongsa T, Romero R, Whitten AE, et al. The use of angiogenic biomarkers in maternal blood to identify which SGA fetuses will require a preterm delivery and mothers who will develop pre-eclampsia. J Matern Fetal Neonatal Med. 2016;29(8):1214–1228.
- Birdir C, Fryze J, Frölich S, et al. Impact of maternal serum levels of visfatin, AFP, PAPP-A, sFlt-1 and PlGF at 11-13 weeks gestation on small for gestational age births. J Matern Fetal Neonatal Med. 2017;30(6):629–634.
- Chaiworapongsa T, Romero R, Gotsch F, et al. Acute pyelonephritis during pregnancy changes the balance of angiogenic and anti-angiogenic factors in maternal plasma. J Matern Fetal Neonatal Med. 2010;23(2):167–178.
- Soto E, Romero R, Vaisbuch E, et al. Fragment bb: evidence for activation of the alternative pathway of the complement system in pregnant women with acute pyelonephritis. J Matern Fetal Neonatal Med. 2010;23(10):1085–1090.
- Assi F, Fruscio R, Bonardi C, et al. Pentraxin 3 in plasma and vaginal fluid in women with preterm delivery. BJOG. 2007;114(2):143–147.
- Cruciani L, Romero R, Vaisbuch E, et al. Pentraxin 3 in maternal circulation: an association with preterm labor and preterm PROM, but not with intra-amniotic infection/inflammation. J Matern Fetal Neonatal Med. 2010;23(10):1097–1105.
- Chen X, Scholl TO. Maternal biomarkers of endothelial dysfunction and preterm delivery. PLoS One. 2014;9(1):e85716.
- Tarca AL, Pataki B, Romero R, DREAM Preterm Birth Prediction Challenge Consortium, et al. Crowdsourcing assessment of maternal blood multi-omics for predicting gestational age and preterm birth. Cell Rep Med. 2021;2(6):100323.
- Romero R, Chaiworapongsa T, Erez O, et al. An imbalance between angiogenic and anti-angiogenic factors precedes fetal death in a subset of patients: results of a longitudinal study. J Matern Fetal Neonatal Med. 2010;23(12):1384–1399.
- Espinoza J, Chaiworapongsa T, Romero R, et al. Unexplained fetal death: another anti-angiogenic state. J Matern Fetal Neonatal Med. 2007;20(7):495–507.
- Smith GC, Crossley JA, Aitken DA, et al. Circulating angiogenic factors in early pregnancy and the risk of preeclampsia, intrauterine growth restriction, spontaneous preterm birth, and stillbirth. Obstet Gynecol. 2007;109(6):1316–1324.
- Chaiworapongsa T, Romero R, Erez O, et al. The prediction of fetal death with a simple maternal blood test at 20-24 weeks: a role for angiogenic index-1 (PlGF/sVEGFR-1 ratio). Am J Obstet Gynecol. 2017;217(6):682.e1–e13.
- Erez O, Gotsch F, Mazaki-Tovi S, et al. Evidence of maternal platelet activation, excessive thrombin generation, and high amniotic fluid tissue factor immunoreactivity and functional activity in patients with fetal death. J Matern Fetal Neonatal Med. 2009;22(8):672–687.
- Ay C, Kaider A, Koder S, et al. Association of elevated soluble P-selectin levels with fetal loss in women with a history of venous thromboembolism. Thromb Res. 2012;129(6):725–728.
- Richani K, Romero R, Soto E, et al. Unexplained intrauterine fetal death is accompanied by activation of complement. J Perinat Med. 2005;33(4):296–305.
- Rieckmann P, D'Alessandro F, Nordan RP, et al. IL-6 and tumor necrosis factor-alpha. Autocrine and paracrine cytokines involved in B cell function. J Immunol. 1991;146(10):3462–3468.
- Cassatella MA, Meda L, Bonora S, et al. Interleukin 10 (IL-10) inhibits the release of proinflammatory cytokines from human polymorphonuclear leukocytes. Evidence for an autocrine role of tumor necrosis factor and IL-1 beta in mediating the production of IL-8 triggered by lipopolysaccharide. J Exp Med. 1993;178(6):2207–2211.
- Arzt E, Stalla GK. Cytokines: autocrine and paracrine roles in the anterior pituitary. Neuroimmunomodulation. 1996;3(1):28–34.
- Kaplan D. Autocrine secretion and the physiological concentration of cytokines. Immunol Today. 1996;17(7):303–304.
- Renner U, Pagotto U, Arzt E, et al. Autocrine and paracrine roles of polypeptide growth factors, cytokines and vasogenic substances in normal and tumorous pituitary function and growth: a review. Eur J Endocrinol. 1996;135(5):515–532.
- Rothwell NJ. The endocrine significance of cytokines. J Endocrinol. 1991;128(2):171–173.
- Vassilopoulou-Sellin R. Endocrine effects of cytokines. Oncology. 1994;8(10):43–50.
- Silva CM, Isgaard J, Thorner MO. Cytokines in endocrine function. Adv Protein Chem. 1998;52:199–221.
- Smárason AK, Sargent IL, Starkey PM, et al. The effect of placental syncytiotrophoblast microvillous membranes from normal and pre-eclamptic women on the growth of endothelial cells in vitro. Br J Obstet Gynaecol. 1993;100(10):943–949.
- Cockell AP, Learmont JG, Smárason AK, et al. Human placental syncytiotrophoblast microvillous membranes impair maternal vascular endothelial function. Br J Obstet Gynaecol. 1997;104(2):235–240.
- Redman CW, Sargent IL. Placental debris, oxidative stress and pre-eclampsia. Placenta. 2000;21(7):597–602.
- Sabapatha A, Gercel-Taylor C, Taylor DD. Specific isolation of placenta-derived exosomes from the circulation of pregnant women and their immunoregulatory consequences. Am J Reprod Immunol. 2006;56(5-6):345–355.
- Yáñez-Mó M, Siljander PR, Andreu Z, et al. Biological properties of extracellular vesicles and their physiological functions. J Extracell Vesicles. 2015;4:27066.
- Ouyang Y, Mouillet JF, Coyne CB, et al. Review: placenta-specific microRNAs in exosomes - good things come in nano-packages. Placenta. 2014;35 Suppl: s 69–73.
- Delorme-Axford E, Donker RB, Mouillet JF, et al. Human placental trophoblasts confer viral resistance to recipient cells. Proc Natl Acad Sci U S A. 2013;110(29):12048–12053.
- Gardiner C, Tannetta DS, Simms CA, et al. Syncytiotrophoblast microvesicles released from pre-eclampsia placentae exhibit increased tissue factor activity. PLOS One. 2011;6(10):e26313.
- Taylor DD, Akyol S, Gercel-Taylor C. Pregnancy-associated exosomes and their modulation of T cell signaling. J Immunol. 2006;176(3):1534–1542.
- Zaborowski MP, Balaj L, Breakefield XO, et al. Extracellular vesicles: composition, biological relevance, and methods of study. Bioscience. 2015;65(8):783–797.
- Colombo M, Raposo G, Théry C. Biogenesis, secretion, and intercellular interactions of exosomes and other extracellular vesicles. Annu Rev Cell Dev Biol. 2014;30:255–289.
- Olejarz W, Kubiak-Tomaszewska G, Chrzanowska A, et al. Exosomes in angiogenesis and anti-angiogenic therapy in cancers. Int J Mol Sci. 2020;21(16):5840.
- Gai C, Pomatto MAC, Grange C, et al. Extracellular vesicles in onco-nephrology. Exp Mol Med. 2019;51(3):1–8.
- Marar C, Starich B, Wirtz D. Extracellular vesicles in immunomodulation and tumor progression. Nat Immunol. 2021;22(5):560–570.
- Croft PK, Sharma S, Godbole N, et al. Ovarian-Cancer-Associated extracellular vesicles: microenvironmental regulation and potential clinical applications. Cells. 2021;10(9):2272.
- van Niel G, D'Angelo G, Raposo G. Shedding light on the cell biology of extracellular vesicles. Nat Rev Mol Cell Biol. 2018;19(4):213–228.
- Karpman D, Ståhl AL, Arvidsson I. Extracellular vesicles in renal disease. Nat Rev Nephrol. 2017;13(9):545–562.
- Fitzgerald W, Freeman ML, Lederman MM, et al. A system of cytokines encapsulated in ExtraCellular vesicles. Sci Rep. 2018;8(1):8973.
- Fitzgerald W, Gomez-Lopez N, Erez O, et al. Extracellular vesicles generated by placental tissues ex vivo: a transport system for immune mediators and growth factors. Am J Reprod Immunol. 2018;80(1):e12860.
- Committee opinion no 700: methods for estimating the due date. Obstet Gynecol. 2017;129:e150–e4.
- Macdorman MF, Kirmeyer S. The challenge of fetal mortality. NCHS Data Brief. 2009;(16):1–8.
- Alexander GR, Himes JH, Kaufman RB, et al. A United States national reference for fetal growth. Obstet Gynecol. 1996;87(2):163–168.
- ACOG practice bulletin. Diagnosis and management of preeclampsia and eclampsia. Number 33, January 2002. Obstet Gynecol. 2002;99:159–167.
- Langston C, Kaplan C, Macpherson T, et al. Practice guideline for examination of the placenta: developed by the placental pathology practice guideline development task force of the College of American Pathologists. Arch Pathol Lab Med. 1997;121(5):449–476.
- Romero R, Kim YM, Pacora P, et al. The frequency and type of placental histologic lesions in term pregnancies with normal outcome. J Perinat Med. 2018;46(6):613–630.
- Redline RW, Heller D, Keating S, et al. Placental diagnostic criteria and clinical correlation–a workshop report. Placenta. 2005;26 (Suppl A): s 114–7.
- Khong TY, Mooney EE, Ariel I, et al. Sampling and definitions of placental lesions: amsterdam placental workshop group consensus statement. Arch Pathol Lab Med. 2016;140(7):698–713.
- Bhatti G, Romero R, Rice GE, et al. Compartmentalized profiling of amniotic fluid cytokines in women with preterm labor. PLoS ONE. 2020;15(1):e0227881.
- Sass D, Fitzgerald W, Wolff BS, et al. Differences in circulating extracellular vesicle and soluble cytokines in older versus younger breast cancer patients with distinct symptom profiles. Front Genet. 2022;13:869044.
- Uddin S, Khan A, Hossain ME, et al. Comparing different supervised machine learning algorithms for disease prediction. BMC Med Inform Decis Mak. 2019;19(1):281.
- Díaz-Uriarte R, Alvarez de Andrés S. Gene selection and classification of microarray data using random Forest. BMC Bioinformatics. 2006;7:3.
- Goldstein BA, Hubbard AE, Cutler A, et al. An application of random forests to a genome-wide association dataset: methodological considerations & new findings. BMC Genet. 2010;11:49.
- Zolfaghari F, Khosravi H, Shahriyari A, et al. Hierarchical cluster analysis to identify the homogeneous desertification management units. PLOS One. 2019;14(12):e0226355.
- Zhang Z, Murtagh F, Van Poucke S, et al. Hierarchical cluster analysis in clinical research with heterogeneous study population: highlighting its visualization with R. Ann Transl Med. 2017;5(4):75.
- Gu Z, Eils R, Schlesner M. Complex heatmaps reveal patterns and correlations in multidimensional genomic data. Bioinformatics. 2016;32(18):2847–2849.
- Johnstone RM, Adam M, Hammond JR, et al. Vesicle formation during reticulocyte maturation. Association of plasma membrane activities with released vesicles (exosomes). J Biol Chem. 1987;262(19):9412–9420.
- Lo Cicero A, Stahl PD, Raposo G. Extracellular vesicles shuffling intercellular messages: for good or for bad. Curr Opin Cell Biol. 2015;35:69–77.
- Zhang J, Li H, Fan B, et al. Extracellular vesicles in normal pregnancy and pregnancy-related diseases. J Cell Mol Med. 2020;24(8):4377–4388.
- Mitchell MD, Peiris HN, Kobayashi M, et al. Placental exosomes in normal and complicated pregnancy. Am J Obstet Gynecol. 2015;213(4 Suppl):S173–S81.
- Buca D, D'Antonio F, Buca D, et al. Extracellular vesicles in pregnancy: their potential role as a liquid biopsy. J Reprod Immunol. 2022;154:103734.
- Hashimoto A, Sugiura K, Hoshino A. Impact of exosome-mediated feto-maternal interactions on pregnancy maintenance and development of obstetric complications. J Biochem. 2021;169(2):163–171.
- Mincheva-Nilsson L, Baranov V. Placenta-derived exosomes and syncytiotrophoblast microparticles and their role in human reproduction: immune modulation for pregnancy success. Am J Reprod Immunol. 2014;72(5):440–457.
- Truong G, Guanzon D, Kinhal V, et al. Oxygen tension regulates the miRNA profile and bioactivity of exosomes released from extravillous trophoblast cells – liquid biopsies for monitoring complications of pregnancy. PLOS One. 2017;12(3):e0174514.
- Hadley EE, Sheller-Miller S, Saade G, et al. Amnion epithelial cell-derived exosomes induce inflammatory changes in uterine cells. Am J Obstet Gynecol. 2018;219(5):e1–e21.
- Menon R, Bonney EA, Condon J, et al. Novel concepts on pregnancy clocks and alarms: redundancy and synergy in human parturition. Hum Reprod Update. 2016;22(5):535–560.
- Toth B, Lok CA, Böing A, et al. Microparticles and exosomes: impact on normal and complicated pregnancy. Am J Reprod Immunol. 2007;58(5):389–402.
- Mouillet JF, Ouyang Y, Coyne CB, et al. MicroRNAs in placental health and disease. Am J Obstet Gynecol. 2015;213(4 Suppl):S163–S72.
- Jia R, Li J, Rui C, et al. Comparative proteomic profile of the human umbilical cord blood exosomes between normal and preeclampsia pregnancies with high-resolution mass spectrometry. Cell Physiol Biochem. 2015;36(6):2299–2306.
- Dragovic RA, Southcombe JH, Tannetta DS, et al. Multicolor flow cytometry and nanoparticle tracking analysis of extracellular vesicles in the plasma of normal pregnant and pre-eclamptic women. Biol Reprod. 2013;89(6):151.
- Luo R, Shao X, Xu P, et al. MicroRNA-210 contributes to preeclampsia by downregulating potassium channel modulatory factor 1. Hypertension. 2014;64(4):839–845.
- Biró O, Alasztics B, Molvarec A, et al. Various levels of circulating exosomal total-miRNA and miR-210 hypoxamiR in different forms of pregnancy hypertension. Pregnancy Hypertens. 2017;10:207–212.
- Salomon C, Guanzon D, Scholz-Romero K, et al. Placental exosomes as early biomarker of preeclampsia: potential role of exosomal MicroRNAs across gestation. J Clin Endocrinol Metab. 2017;102(9):3182–3194.
- Tan KH, Tan SS, Sze SK, et al. Plasma biomarker discovery in preeclampsia using a novel differential isolation technology for circulating extracellular vesicles. Am J Obstet Gynecol. 2014;211(4):380.e1-13–380.13.
- Salomon C, Scholz-Romero K, Sarker S, et al. Gestational diabetes mellitus is associated with changes in the concentration and bioactivity of placenta-derived exosomes in maternal circulation across gestation. Diabetes. 2016;65(3):598–609.
- James-Allan LB, Devaskar SU. Extracellular vesicles and their role in gestational diabetes mellitus. Placenta. 2021;113:15–22.
- Palma C, McIntyre HD, Salomon C. Extracellular vesicles-New players in cell-to-cell communication in gestational diabetes mellitus. Biomedicines. 2022;10(2):462.
- Ye Z, Wang S, Huang X, Chen P, Deng L, Li S, et al. Plasma exosomal microRNAs associated with metabolism as early predictor of gestational diabetes mellitus. Diabetes. 2022;71(11):2272–2283.
- Nair S, Ormazabal V, Lappas M, et al. Extracellular vesicles and their potential role inducing changes in maternal insulin sensitivity during gestational diabetes mellitus. Am J Reprod Immunol. 2021;85(2):e13361.
- Dixon CL, Sheller-Miller S, Saade GR, et al. Amniotic fluid exosome proteomic profile exhibits unique pathways of term and preterm labor. Endocrinology. 2018;159(5):2229–2240.
- Menon R, Debnath C, Lai A, et al. Protein profile changes in circulating placental extracellular vesicles in term and preterm births: a longitudinal study. Endocrinology. 2020;161(4):161.
- Cook J, Bennett PR, Kim SH, et al. First trimester circulating microRNA biomarkers predictive of subsequent preterm delivery and cervical shortening. Sci Rep. 2019;9(1):5861.
- Fallen S, Baxter D, Wu X, et al. Extracellular vesicle RNAs reflect placenta dysfunction and are a biomarker source for preterm labour. J Cell Mol Med. 2018;22(5):2760–2773.
- Menon R, Debnath C, Lai A, Garbhini Study Team, et al. Circulating exosomal miRNA profile during term and preterm birth pregnancies: a longitudinal study. Endocrinology. 2019;160(2):249–275.
- Chaiworapongsa T, Kusanovic JP, Savasan ZA, et al. Fetal death: a condition with a dissociation in the concentrations of soluble vascular endothelial growth factor receptor-2 between the maternal and fetal compartments. J Matern Fetal Neonatal Med. 2010;23(9):960–972.
- Chaiworapongsa T, Romero R, Kusanovic JP, et al. Unexplained fetal death is associated with increased concentrations of anti-angiogenic factors in amniotic fluid. J Matern Fetal Neonatal Med. 2010;23(8):794–805.
- Whitten AE, Romero R, Korzeniewski SJ, et al. Evidence of an imbalance of angiogenic/antiangiogenic factors in massive perivillous fibrin deposition (maternal floor infarction): a placental lesion associated with recurrent miscarriage and fetal death. Am J Obstet Gynecol. 2013;208(4):e1–e11.
- Korzeniewski SJ, Romero R, Chaiworapongsa T, et al. Maternal plasma angiogenic index-1 (placental growth factor/soluble vascular endothelial growth factor receptor-1) is a biomarker for the burden of placental lesions consistent with uteroplacental underperfusion: a longitudinal case-cohort study. Am J Obstet Gynecol. 2016;214(5):629.e1–629.e17.
- Venkatesha S, Toporsian M, Lam C, et al. Soluble endoglin contributes to the pathogenesis of preeclampsia. Nat Med. 2006;12(6):642–649.
- Levine RJ, Lam C, Qian C, et al. Soluble endoglin and other circulating antiangiogenic factors in preeclampsia. N Engl J Med. 2006;355(10):992–1005.
- Fisher SJ. The placental problem: linking abnormal cytotrophoblast differentiation to the maternal symptoms of preeclampsia. Reprod Biol Endocrinol. 2004;2(1):53.
- Soto E, Romero R, Kusanovic JP, et al. Late-onset preeclampsia is associated with an imbalance of angiogenic and anti-angiogenic factors in patients with and without placental lesions consistent with maternal underperfusion. J Matern Fetal Neonatal Med. 2012;25(5):498–507.
- Romero R, Nien JK, Espinoza J, et al. A longitudinal study of angiogenic (placental growth factor) and anti-angiogenic (soluble endoglin and soluble vascular endothelial growth factor receptor-1) factors in normal pregnancy and patients destined to develop preeclampsia and deliver a small for gestational age neonate. J Matern Fetal Neonatal Med. 2008;21:9–23.
- Erez O, Romero R, Espinoza J, et al. The change in concentrations of angiogenic and anti-angiogenic factors in maternal plasma between the first and second trimesters in risk assessment for the subsequent development of preeclampsia and small-for-gestational age. J Matern Fetal Neonatal Med. 2008;21(5):279–287.
- Kusanovic JP, Romero R, Chaiworapongsa T, et al. A prospective cohort study of the value of maternal plasma concentrations of angiogenic and anti-angiogenic factors in early pregnancy and midtrimester in the identification of patients destined to develop preeclampsia. J Matern Fetal Neonatal Med. 2009;22(11):1021–1038.
- Chaiworapongsa T, Romero R, Savasan ZA, et al. Maternal plasma concentrations of angiogenic/anti-angiogenic factors are of prognostic value in patients presenting to the obstetrical triage area with the suspicion of preeclampsia. J Matern Fetal Neonatal Med. 2011;24(10):1187–1207.
- Stepan H, Geipel A, Schwarz F, et al. Circulatory soluble endoglin and its predictive value for preeclampsia in second-trimester pregnancies with abnormal uterine perfusion. Am J Obstet Gynecol. 2008;198(2):175.e1-6–175.e6.
- Toft JH, Lian IA, Tarca AL, et al. Whole-genome microarray and targeted analysis of angiogenesis-regulating gene expression (ENG, FLT1, VEGF, PlGF) in placentas from pre-eclamptic and small-for-gestational-age pregnancies. J Matern Fetal Neonatal Med. 2008;21(4):267–273.
- Jaiman S, Romero R, Gotsch F, et al. Fetal sepsis: a cause of stillbirth. J Matern Fetal Neonatal Med. 2022;35(25):9966–9970.
- McClure EM, Saleem S, Goudar SS, et al. Stillbirth 2010–2018: a prospective, population-based, multi-country study from the global network. Reprod Health. 2020;17(Suppl 2):146.
- Fernandez HN, Hugli TE. Primary structural analysis of the polypeptide portion of human C5a anaphylatoxin. Polypeptide sequence determination and assignment of the oligosaccharide attachment site in C5a. J Biol Chem. 1978;253(19):6955–6964.
- Ward PA. The dark side of C5a in sepsis. Nat Rev Immunol. 2004;4(2):133–142.
- Kuzmicki M, Telejko B, Zonenberg A, et al. Circulating pro- and anti-inflammatory cytokines in polish women with gestational diabetes. Horm Metab Res. 2008;40(8):556–560.
- Gallo DM, Romero R, Bosco M, Chaiworapongsa T, Gomez-Lopez N, Arenas-Hernandez M, et al. Maternal plasma cytokines and the subsequent risk of uterine atony and postpartum hemorrhage. J Perinat Med. 2022;
- Brien ME, Boufaied I, Bernard N, et al. Specific inflammatory profile in each pregnancy complication: a comparative study. Am J Reprod Immunol. 2020;84(6):e13316.
- Lumbreras-Marquez MI, Lumbreras-Marquez J, Barraza-Salas M, et al. Maternal and umbilical cord procalcitonin, high-sensitivity C-reactive protein, and interleukin-6 levels in preeclamptic and normotensive patients: a cross-sectional study. Pregnancy Hypertens. 2020;21:218–223.
- Romero R, Chaemsaithong P, Chaiyasit N, et al. CXCL10 and IL-6: markers of two different forms of intra-amniotic inflammation in preterm labor. Am J Reprod Immunol. 2017;78(1):e12685.
- Chaemsaithong P, Romero R, Korzeniewski SJ, et al. A point of care test for the determination of amniotic fluid interleukin-6 and the chemokine CXCL-10/IP-10. J Matern Fetal Neonatal Med. 2015;28(13):1510–1519.
- Stranik J, Kacerovsky M, Andrys C, et al. Intra-amniotic infection and sterile intra-amniotic inflammation are associated with elevated concentrations of cervical fluid interleukin-6 in women with spontaneous preterm labor with intact membranes. J Matern Fetal Neonatal Med. 2021;35(25):4861–4869.
- Chaemsaithong P, Romero R, Docheva N, et al. Comparison of rapid MMP-8 and interleukin-6 point-of-care tests to identify intra-amniotic inflammation/infection and impending preterm delivery in patients with preterm labor and intact membranes. J Matern Fetal Neonatal Med. 2018;31(2):228–244.
- Chaemsaithong P, Romero R, Korzeniewski SJ, et al. A rapid interleukin-6 bedside test for the identification of intra-amniotic inflammation in preterm labor with intact membranes. J Matern Fetal Neonatal Med. 2016;29(3):349–359.
- Yoon BH, Romero R, Kim CJ, et al. Amniotic fluid interleukin-6: a sensitive test for antenatal diagnosis of acute inflammatory lesions of preterm placenta and prediction of perinatal morbidity. Am J Obstet Gynecol. 1995;172(3):960–970.
- Yoon BH, Jun JK, Romero R, et al. Amniotic fluid inflammatory cytokines (interleukin-6, interleukin-1beta, and tumor necrosis factor-alpha), neonatal brain white matter lesions, and cerebral palsy. Am J Obstet Gynecol. 1997;177(1):19–26.
- Kim CJ, Romero R, Chaemsaithong P, et al. Acute chorioamnionitis and funisitis: definition, pathologic features, and clinical significance. Am J Obstet Gynecol. 2015;213(4):S29–S52.
- Lagodka S, Petrucci S, Moretti ML, et al. Fetal and maternal inflammatory response in the setting of maternal intrapartum fever with and without clinical and histologic chorioamnionitis. Am J Obstet Gynecol MFM. 2022;4(2):100539.
- Hammond ME, Lapointe GR, Feucht PH, et al. IL-8 induces neutrophil chemotaxis predominantly via type I IL-8 receptors. J Immunol. 1995;155(3):1428–1433.
- Zinkernagel AS, Timmer AM, Pence MA, et al. The IL-8 protease SpyCEP/ScpC of group a Streptococcus promotes resistance to neutrophil killing. Cell Host Microbe. 2008;4(2):170–178.
- Estrada-Gutierrez G, Cappello RE, Mishra N, et al. Increased expression of matrix metalloproteinase-1 in systemic vessels of preeclamptic women: a critical mediator of vascular dysfunction. Am J Pathol. 2011;178(1):451–460.
- Zhang YJ, Rutledge BJ, Rollins BJ. Structure/activity analysis of human monocyte chemoattractant protein-1 (MCP-1) by mutagenesis. Identification of a mutated protein that inhibits MCP-1-mediated monocyte chemotaxis. J Biol Chem. 1994;269(22):15918–15924.
- Weber M, Uguccioni M, Baggiolini M, et al. Deletion of the NH2-terminal residue converts monocyte chemotactic protein 1 from an activator of basophil mediator release to an eosinophil chemoattractant. J Exp Med. 1996;183(2):681–685.
- Kameyoshi Y, Dörschner A, Mallet AI, et al. Cytokine RANTES released by thrombin-stimulated platelets is a potent attractant for human eosinophils. J Exp Med. 1992;176(2):587–592.
- Garlanda C, Bottazzi B, Bastone A, et al. Pentraxins at the crossroads between innate immunity, inflammation, matrix deposition, and female fertility. Annu Rev Immunol. 2005;23:337–366.
- Bottazzi B, Garlanda C, Cotena A, et al. The long pentraxin PTX3 as a prototypic humoral pattern recognition receptor: interplay with cellular innate immunity. Immunol Rev. 2009;227(1):9–18.
- Cetin I, Cozzi V, Pasqualini F, et al. Elevated maternal levels of the long pentraxin 3 (PTX3) in preeclampsia and intrauterine growth restriction. Am J Obstet Gynecol. 2006;194(5):1347–1353.
- Cetin I, Cozzi V, Papageorghiou AT, et al. First trimester PTX3 levels in women who subsequently develop preeclampsia and fetal growth restriction. Acta Obstet Gynecol Scand. 2009;88(7):846–849.
- Galbiati S, Causarano V, Pinzani P, et al. Evaluation of a panel of circulating DNA, RNA and protein potential markers for pathologies of pregnancy. Clin Chem Lab Med. 2010;48(6):791–794.
- Boij R, Svensson J, Nilsson-Ekdahl K, et al. Biomarkers of coagulation, inflammation, and angiogenesis are independently associated with preeclampsia. Am J Reprod Immunol. 2012;68(3):258–270.
- Colmenares-Mejía CC, Quintero-Lesmes DC, Bautista-Niño PK, et al. Pentraxin-3 is a candidate biomarker on the spectrum of severity from pre-eclampsia to HELLP syndrome: genPE study. Hypertens Res. 2020;43(9):884–891.
- Cruciani L, Romero R, Vaisbuch E, et al. Pentraxin 3 in amniotic fluid: a novel association with intra-amniotic infection and inflammation. J Perinat Med. 2010;38(2):161–171.
- Cozzi V, Garlanda C, Nebuloni M, et al. PTX3 as a potential endothelial dysfunction biomarker for severity of preeclampsia and IUGR. Placenta. 2012;33(12):1039–1044.
- El-Azzamy H, Balogh A, Romero R, et al. Characteristic changes in decidual gene expression signature in spontaneous term parturition. J Pathol Transl Med. 2017;51(3):264–283.
- Than NG, Romero R, Erez O, et al. Emergence of hormonal and redox regulation of galectin-1 in placental mammals: implication in maternal-fetal immune tolerance. Proc Natl Acad Sci U S A. 2008;105(41):15819–15824.
- Than NG, Erez O, Wildman DE, et al. Severe preeclampsia is characterized by increased placental expression of galectin-1. J Matern Fetal Neonatal Med. 2008;21(7):429–442.
- Than NG, Kim SS, Abbas A, et al. Chorioamnionitis and increased galectin-1 expression in PPROM –an anti-inflammatory response in the fetal membranes? Am J Reprod Immunol. 2008;60(4):298–311.
- Than NG, Romero R, Kim CJ, et al. Galectins: guardians of eutherian pregnancy at the maternal-fetal interface. Trends Endocrinol Metab. 2012;23(1):23–31.
- Bianchi ME. DAMPs, PAMPs and alarmins: all we need to know about danger. J Leukoc Biol. 2007;81(1):1–5.
- Schnabel A, Blois SM, Meint P, et al. Elevated systemic galectin-1 levels characterize HELLP syndrome. J Reprod Immunol. 2016;114:38–43.
- Jeschke U, Mayr D, Schiessl B, et al. Expression of galectin-1, -3 (gal-1, gal-3) and the Thomsen-Friedenreich (TF) antigen in normal, IUGR, preeclamptic and HELLP placentas. Placenta. 2007;28(11-12):1165–1173.
- Broze GJ, Jr., Warren LA, Novotny WF, et al. The lipoprotein-associated coagulation inhibitor that inhibits the factor VII-tissue factor complex also inhibits factor Xa: insight into its possible mechanism of action. Blood. 1988;71(2):335–343.
- Wun TC, Kretzmer KK, Girard TJ, et al. Cloning and characterization of a cDNA coding for the lipoprotein-associated coagulation inhibitor shows that it consists of three tandem Kunitz-type inhibitory domains. J Biol Chem. 1988;263(13):6001–6004.
- Girard TJ, Warren LA, Novotny WF, et al. Functional significance of the Kunitz-type inhibitory domains of lipoprotein-associated coagulation inhibitor. Nature. 1989;338(6215):518–520.
- Kaiser B, Fareed J. Recombinant full-length tissue factor pathway inhibitor (TFPI) prevents thrombus formation and rethrombosis after lysis in a rabbit model of jugular vein thrombosis. Thromb Haemost. 1996;76(04):615–620.
- Sandset PM, Warn-Cramer BJ, Rao LV, et al. Depletion of extrinsic pathway inhibitor (EPI) sensitizes rabbits to disseminated intravascular coagulation induced with tissue factor: evidence supporting a physiologic role for EPI as a natural anticoagulant. Proc Natl Acad Sci U S A. 1991;88(3):708–712.
- Levi M, de Jonge E, van der Poll T. Rationale for restoration of physiological anticoagulant pathways in patients with sepsis and disseminated intravascular coagulation. Crit Care Med. 2001;29(7 Suppl):S90–S4.
- Mosad E, Elsayh KI, Eltayeb AA. Tissue factor pathway inhibitor and P-selectin as markers of sepsis-induced non-overt disseminated intravascular coagulopathy. Clin Appl Thromb Hemost. 2011;17(1):80–87.
- Takahashi H, Sato N, Shibata A. Plasma tissue factor pathway inhibitor in disseminated intravascular coagulation: comparison of its behavior with plasma tissue factor. Thromb Res. 1995;80(4):339–348.
- Shimura M, Wada H, Wakita Y, et al. Plasma tissue factor and tissue factor pathway inhibitor levels in patients with disseminated intravascular coagulation. Am J Hematol. 1997;55(4):169–174.
- Asakura H, Ontachi Y, Mizutani T, et al. Elevated levels of free tissue factor pathway inhibitor antigen in cases of disseminated intravascular coagulation caused by various underlying diseases. Blood Coagul Fibrinolysis. 2001;12(1):1–8.
- Gando S, Kameue T, Morimoto Y, et al. Tissue factor production not balanced by tissue factor pathway inhibitor in sepsis promotes poor prognosis. Crit Care Med. 2002;30:1729–1734.
- Lwaleed BA, Bass PS. Tissue factor pathway inhibitor: structure, biology and involvement in disease. J Pathol. 2006;208(3):327–339.
- Feng Q, Liu K, Liu YX, et al. Plasminogen activators and inhibitors are transcribed during early macaque implantation. Placenta. 2001;22(2–3):186–199.
- Streuli C. Extracellular matrix remodelling and cellular differentiation. Curr Opin Cell Biol. 1999;11(5):634–640.
- Shapiro SD. Matrix metalloproteinase degradation of extracellular matrix: biological consequences. Curr Opin Cell Biol. 1998;10(5):602–608.
- Fernandez-Patron C, Radomski MW, Davidge ST. Vascular matrix metalloproteinase-2 cleaves big endothelin-1 yielding a novel vasoconstrictor. Circ Res. 1999;85(10):906–911.
- Fernandez-Patron C, Stewart KG, Zhang Y, et al. Vascular matrix metalloproteinase-2-dependent cleavage of calcitonin gene-related peptide promotes vasoconstriction. Circ Res. 2000;87(8):670–676.
- Kveiborg M, Albrechtsen R, Couchman JR, et al. Cellular roles of ADAM12 in health and disease. Int J Biochem Cell Biol. 2008;40(9):1685–1702.
- Walsh SW, Strauss JF. Pregnancy-specific expression of protease-activated receptor 1: a therapeutic target for prevention and treatment of preeclampsia? Am J Obstet Gynecol. 2022;226(2S):S945–S953.
- Walsh SW, Nugent WH, Al Dulaimi M, et al. Proteases activate pregnancy neutrophils by a Protease-Activated receptor 1 pathway: epigenetic implications for preeclampsia. Reprod Sci. 2020;27(11):2115–2127.
- Cowans NJ, Spencer K. First-trimester ADAM12 and PAPP-A as markers for intrauterine fetal growth restriction through their roles in the insulin-like growth factor system. Prenat Diagn. 2007;27(3):264–271.
- Laigaard J, Sørensen T, Placing S, et al. Reduction of the disintegrin and metalloprotease ADA. M12 in preeclampsia. Obstet Gynecol. 2005;106(1):144–149.
- Karagiannis G, Akolekar R, Sarquis R, et al. Prediction of small-for-gestation neonates from biophysical and biochemical markers at 11–13 weeks. Fetal Diagn Ther. 2011;29(2):148–154.
- Andres F, Wong GP, Walker SP, et al. A disintegrin and metalloproteinase 12 (ADAM12) is reduced at 36 weeks’ gestation in pregnancies destined to deliver small for gestational age infants. Placenta. 2022;117:1–4.
- El-Sherbiny W, Nasr A, Soliman A. Metalloprotease (ADAM12-S) as a predictor of preeclampsia: correlation with severity, maternal complications, fetal outcome, and Doppler parameters. Hypertens Pregnancy. 2012;31(4):442–450.
- Yu N, Cui H, Chen X, et al. First trimester maternal serum analytes and second trimester uterine artery Doppler in the prediction of preeclampsia and fetal growth restriction. Taiwan J Obstet Gynecol. 2017;56(3):358–361.
- Harmon QE, Huang L, Umbach DM, et al. Risk of fetal death with preeclampsia. Obstet Gynecol. 2015;125(3):628–635.
- Karaszi K, Szabo S, Juhasz K, et al. Increased placental expression of placental protein 5 (PP5)/tissue factor pathway inhibitor-2 (TFPI-2) in women with preeclampsia and HELLP syndrome: relevance to impaired trophoblast invasion? Placenta. 2019;76:30–39.
- MacDonald TM, Tong S, Myers J, et al. Circulating tissue factor pathway inhibitor (TFPI) is increased preceding preeclampsia diagnosis and in established preeclampsia. Placenta. 2021;105:32–40.
- Walsh SW, Nugent WH, Archer KJ, et al. Epigenetic regulation of interleukin-17-Related genes and their potential roles in neutrophil vascular infiltration in preeclampsia. Reprod Sci. 2022;29(1):154–162.
- Laudanski P, Raba G, Kuc P, et al. Assessment of the selected biochemical markers in predicting preterm labour. J Matern Fetal Neonatal Med. 2012;25(12):2696–2699.
- Raba G, Tabarkiewicz J. Cytokines in preterm delivery: proposal of a new diagnostic algorithm. J Immunol Res. 2018;2018:8073476.
- Zhang S, Wang Y, Li J, et al. MiR-101 inhibits migration and invasion of trophoblast HTR-8/SVneo cells by targeting CXCL6 in preeclampsia. Minerva Med. 2021;112(2):302–303.
- Mittal P, Romero R, Kusanovic JP, et al. CXCL6 (granulocyte chemotactic protein-2): a novel chemokine involved in the innate immune response of the amniotic cavity. Am J Reprod Immunol. 2008;60(3):246–257.
- Mastrodima S, Akolekar R, Yerlikaya G, et al. Prediction of stillbirth from biochemical and biophysical markers at 11–13 weeks. Ultrasound Obstet Gynecol. 2016;48(5):613–617.
- Akolekar R, Machuca M, Mendes M, et al. Prediction of stillbirth from placental growth factor at 11–13 weeks. Ultrasound Obstet Gynecol. 2016;48(5):618–623.
- Smith GC, Stenhouse EJ, Crossley JA, et al. Early pregnancy levels of pregnancy-associated plasma protein a and the risk of intrauterine growth restriction, premature birth, preeclampsia, and stillbirth. J Clin Endocrinol Metab. 2002;87(4):1762–1767.