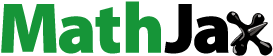
Abstract
Objective
During pregnancy, the stiffness of the cervical tissue decreases long before the cervical length decreases. Therefore, several approaches have been proposed in order to ensure a more objective assessment of cervical stiffness than that achieved by digital evaluation. Strain elastography has shown promising results. This technique is based on an ultrasound assessment of the tissue deformation that occurs when the examiner applies pressure on the tissue with the ultrasound probe. However, the results are only semi-quantitative as they depend on the unmeasured force used by the examiner. We, therefore, hypothesized that a force-measuring device applied to the handle of the ultrasound probe may render the technique quantitative. With this approach, the stiffness is the force (measured by the device) divided by the compression (measured by the elastography platform). One perspective is the early identification of women at risk of preterm birth in whom cervical stiffness may decrease long before cervical shortening. Another perspective is cervical evaluation when planning labor induction. In this feasibility study, we aimed to evaluate how quantitative strain elastography performs when a commercially available strain elastography platform (by which the algorithm is unavailable) is combined with a custom-made, force-measuring device. We studied how the assessments were associated with the gestational age in women with uncomplicated pregnancies and how they were associated with cervical dilatation time from 4 to 10 cm in women undergoing labor induction.
Methods
In the analysis, we included quantitative strain elastography assessments from 47 women with uncomplicated singleton pregnancies, with gestational age between 12+0 and 40+0, and from 27 singleton term-pregnant women undergoing labor induction. The force-measuring device was mounted on the handle of a transvaginal probe. The strain values (i.e. the compression of the cervical tissue) were obtained by the elastography software of the ultrasound scanner (GE Voluson E10). The region of interest was placed within the central part of the anterior cervical lip. Based on the force data and strain values, we calculated the outcomes cervical elastography indexGE (CEIGE) and the cervical strength indexGE (CEIGE x cervical length: CSIGE).
Results
The average CEIGE was 0.24 N at week 12 and 0.15 N at week 30–34. For CSIGE these figures were 8.2 and 4.7 N mm, respectively (p = 0.002). Among women undergoing labor induction, the CEIGE was associated with a cervical dilatation time (4–10 cm) beyond 7 h. For nulliparous women, this area under the ROC curve was 0.94.
Conclusion
Quantitative strain elastography may constitute a tool for the evaluation of a uterine cervix with normal length in women at risk of preterm birth and in women undergoing labor induction. The performance of this tool deserves evaluation in larger clinical trials.
Introduction
During most pregnancies, the cervical stiffness decreases long before the cervical length [Citation1]. Therefore, it is a challenge that the digital evaluation of cervical stiffness is rather subjective [Citation2]. This hampers the evaluation of women at risk of preterm birth and the planning of labor induction of women at the term of pregnancy. Therefore, more approaches have been suggested for the objective assessment of cervical stiffness [Citation3,Citation4].
One of these approaches is strain elastography. By this technique, the compression of the tissue is assessed by ultrasound when the examiner applies pressure with the ultrasound probe. Transvaginal strain elastography has shown promising results. However, a major limitation of the method is the lack of force control, implying that the obtained elastographic measurements cannot be quantified [Citation5].
Quantitative strain elastography of the uterine cervix can be obtained when results from conventional strain elastography are combined with results from a force-measuring device [Citation6] applied to the handle of the ultrasound probe (). The stiffness of the cervical tissue can then be expressed as the force (measured by the device) divided by the compression of the tissue (measured by the elastography platform). However, we do not know how well the cervical stiffness obtained in this way is physiologically and clinically relevant.
Currently, cervical competence is best assessed by a vaginal ultrasound measurement of the cervical length. For the prediction of the risk of spontaneous preterm birth, cervical length measurements performed with sensitivities of 2–70%, false positive rates of 0–52%, and positive predictive values of 1–40%, depending on the outcome measure and the population [Citation7–12]. For prediction of cesarean delivery after labor induction, cervical length assessments are performed with sensitivities of 14–92% and false positive rates of 0–65% [Citation13].
These modest performances may partly be caused by differences among women in the biomechanical strength of their cervical tissues [Citation14]. Hence, some women may have competent cervices even though their cervical length is relatively short, whereas other women may have incompetent cervices despite the normal cervical length.
The algorithms used by commercially available ultrasound platforms are unavailable, so prior studies of quantitative strain elastography have been conducted by the use of a custom-made algorithm for elastography [Citation6]. It is, however, a challenge that this custom-made setup is difficult to implement in a clinical setting.
We hypothesized that quantitative assessments of the cervical stiffness could be obtained by introducing a force-measuring device applied to the handle of the ultrasound probe in combination with the results from conventional strain elastography.
We, therefore, aimed to evaluate assessments obtained by quantitative elastography based on a custom-made, force-measuring device and GE (General Electric Company) Voluson E10 elastography software. We compared the assessments with cervical length assessment in women with uncomplicated pregnancies at different gestational ages and in women undergoing labor induction. As an evaluation of the risk of cesarean section is beyond the scope of this feasibility study, we chose relatively long latent and active phases as outcomes.
Material and methods
Participants
Cervical strain elastography at different gestational ages: For the evaluation of the associations with gestational age, we included 66 women with a singleton pregnancy at a gestational age within 12+0–14+0; 19+0–21+0; 30+0–34+6 and 35+0–40+0 weeks [Citation6]. All women had an uncomplicated pregnancy at the time of inclusion. This cross-sectional study was conducted at Randers Regional Hospital and Aarhus University Hospital from June through July 2020. Women with an appointment in the obstetric ultrasound clinic were invited to participate.
Cervical strain elastography in women undergoing labor induction: For the evaluation of the associations between the elastography findings and the cervical dilatation time, we included 38 singleton term pregnant women (gestational age 37+0–42+0 weeks), admitted for labor induction by prostaglandin (n = 31), double balloon catheter (n = 2), or amniotomy (n = 5). The Bishop score was determined before the intervention [Citation15]. This prospective cohort study was conducted at Aarhus University Hospital from August through September 2020.
The start of the active phase was defined by an orificium of 4 cm, which is in accordance with the national Danish guidelines. Based on the median values, we categorized the duration of the latent phase (i.e. time from induction of labor to 4 cm of cervix dilatation) as relatively long if it exceeded 24 h, and the duration of the active phase (cervical dilatation from 4 to 10 cm) as relatively long if it exceeded 7 h.
Exclusion criteria: Rupture of fetal membranes, uterine contractions, any connective tissue disease, non-Danish speaking, and age <18 years.
Ultrasound equipment and software
Cervical scans were conducted using a two-dimensional transvaginal ultrasound probe (IC5-9-D). As inclusion took place at several departments, three different VolusonTM E10 Expert scanners were used: one with BT18, one with BT17, and one with BT20 software. However, the elastography software was identical at all three scanners. The probe and scanners were from GE Healthcare, Zipf, Austria.
Strain elastography was used, as in accordance with the available software on the VolusonTM E10 Expert scanners. The elastography color box was placed as close as possible to the probe, with the center of the color box following the probe-centerline.
To unify the elastography measurements, the following default settings were used: gain: 0; dynamic contrast: 6; magnification factor: 1.3; elasto map: 5; persistence filter: 6; line density: 1; window length: 22; window step 4; filter axial: 2; filter lateral: 7; frame reject: 0; pixel reject: 0; transparency: 195; pulse repetition frequency: 25 Hz; lateral resolutions: 0.5 cm, smoothing filter: average 3 sample; B-mode image depth: 3 cm and 120° angle; color box depth: 2 cm and 75° angle.
Force-measuring device
Custom-built, force-measuring shells were mounted on the handle of a transvaginal probe (), and the force was measured by a button load cell (0–20 N, OMEGATM, UK) along the probe-centerline. Subsequently, the force was sampled at 80 Hz with a HX711 (Elextra.dk) connected to an Arduino Nano (RS Components, DK) that transferred the force and time to a computer [Citation6].
Scans
All women were scanned by an examiner (CRT) certified in cervical assessment by the Fetal Medicine Foundation [Citation16]. The probe was placed in the anterior vaginal fornix, a sagittal image of the uterine cervix was obtained, and the cervical length was measured in accordance with The Fetal Medicine Foundation guideline [Citation16]. The probe was then placed perpendicular to the cervical canal, though a deviation of ±35° was accepted. Two or three elastography recordings were conducted for every woman, and each recording was comprised of six to ten compression-decompression cycles at ≈1 Hz.
For elastography, a depicted region of interest (ROI) was placed within the middle third of the anterior cervical lip (), which is dominated by dense strength-determining connective tissue [Citation17]. The cervical canal (red), the inner cervical os, and the sub-epithelial area (orange) was avoided in the ROI. To ensure that softer tissues (appearing red and orange at the elastogram) were not included in the ROI during the compression-decompression cycles, the ROI was surrounded by a buffer zone without such tissues. The ROI was placed within 30° from the probe-centerline ().
Figure 2. Left, B-mode image with the elastography color box superimposed on a cervix at gestational week 38. The ROI (yellow) is placed within the anatomical area of interest within the middle third of the anterior cervical lip. The probe-centerline is marked with the white dashed line. Right, in the resulting strain curve calculated by the GE-software, the strain peak height (Sδ) is marked.

Data processing
The quantitative strain elastography measurements were based on values obtained simultaneously by the elastography software and the force-measuring device. The force-measuring device assessed the force applied to the tissue, and the elastography software assessed the resulting compression of the tissue.
The tissue strain within the given ROI was determined by the strain elastography software (GE Healthcare Austria) and presented as a strain curve (the algorithm for this was unavailable). For each recording, three strain peaks were selected if the strain peak (1) started and ended at the same level and (2) had a well-defined base and top. Only recordings fulfilling these two quality-criteria were included in the analysis. A peak height was calculated for each strain peak (Sδ) ().
The force data was imported and processed in a Python script (Python 3.8) and described in detail elsewhere [Citation6]. In short, the force data was calibrated and expressed in Newton (N), baseline correction was performed by linear regression, and subsequently, the area of a force peak (AF) was calculated by numerical integration (as shown in ).
Figure 3. The force data plotted against the time with the area of a force peak (AF), marked with green.

Based on AF and Sδ, we calculated the cervical elastography indexGE (CEIGE given in N), which expresses the cervical biomechanical strength given as,
The mean of the three CEIGE per woman was reported. However, if one CEIGE measurement differed ≥15% from this mean (|100% - (CEIGE/mean)100%| ≥15%), the measurement was considered unreliable and therefore excluded. The mean of the remaining two recordings was then calculated, and if the CEIGE differed ≥15% from this mean, both recordings were excluded, i.e. the woman was excluded from the study. Finally, a cervical strength indexGE (CSIGE given in N mm) was defined as CEIGE multiplied by the cervical length.
Inter and intraobserver reliability
To assess the interobserver reliability of the CEIGE, two certified examiners (CRT and MSSJ) independently performed the scans, placed the ROI within these scans, and subsequently selected the strain peaks for further data-processing. Interobserver reliability included eight women. For intraobserver reliability, the selection of the strain peaks and the data processing were repeated after four to six weeks to avoid recall bias. Intraobserver reliability included the two examiners evaluating 33 and eight women, respectively.
Statistical analysis
For the four gestational age groups, the means and the 95% confidence intervals (CI) were estimated and compared using linear regression with robust variance estimation to account for unequal variances. In addition, a test for trend was performed without dividing the gestational age into groups.
The measurement variations were analyzed by the 95% limits of agreement (LoA)and intraclass correlation coefficients (ICC). The mixed-effects linear regression model was used to estimate the intraobserver reliability for the two observers even though significant differences in the standard deviation (SD) in the compared groups, as well as the intra-observer difference, were not normally distributed.
The overall ability to discriminate between women with a cervical dilatation time >7 h as well as women with a duration of the latent phase >24 h was measured by the area under the ROC curve (AUC) with 95% CI. From the ROC curves, the cutoff for the variables of interest were determined. Statistical significance was defined as a two-tailed P-value < 0.05. Statistical analyses were conducted using STATA/IC 16.0.
Ethical approval
The study was approved by the Danish Regional Committee on Health Research Ethics (1-10-72-138-16) and the Danish Data Protection Agency (2012-58-006). All women gave written informed consent, and the study was conducted according to the Declaration of Helsinki II. Data were collected and managed using REDCap [Citation18].
Results
Cervical strain elastography at different gestational ages: Of the 66 women with different gestational ages, we excluded 11 as the elastography scans did not meet the set quality criteria and an additional eight as the CEIGE differed ≥15% from the mean. Thus, the study population comprised 47 women of whom 27 (57%) were nulliparous. Background characteristics are presented in .
Table 1. Background characteristics, women with different gestational ages.
With advancing gestational age, the CEIGE decreased before the cervical length decreased (). When comparing the means in the first trimester of pregnancy (weeks 12–14) with the means in the third trimester (weeks 30–34), the cervical length decreased by 6% whereas the CEIGE decreased by 38%. The combined variable CSIGE (CEIGE multiplied by cervical length) decreased by 43%.
Table 2. Cervical length, CEIGE and CSIGE in relation to the gestational age where cervical elastography indexGE (CEIGE) and cervical strength indexGE (CSIGE), gestational age (GA), number of pregnant women (n).
Cervical strain elastography in women undergoing labor induction: Of the 38 women admitted for labor induction, we excluded seven as the elastography scans did not meet the set quality criteria: two because the CEIGE differed ≥15% from the mean, and another two because of the cesarean section before active labor. This resulted in a study population of 27, including 19 (70%) nulliparous women. None of the included women had a cesarean section performed during the active part of the labor, i.e. from 4 to 10 cm cervical dilatation. Background characteristics are presented in .
Table 3. Background characteristics, women admitted for labor induction.
Among these 27 women (), the Bishop score (AUC 0.81) and the cervical length (AUC = 0.78) were relatively good predictors of a latency phase >24 h (from induction to 4 cm cervical dilatation), whereas the CEIGE was not (AUC 0.53). Opposite the prediction of a relatively long active phase (>7 h); where CEIGE had an AUC of 0.86 (0.94 for nulliparous women). When selecting a CEIGE cutoff of 0.13 (), the sensitivity was 100% and the specificity 92% (). Notice, this outcome (relatively long active phase) was not associated with a long cervix but rather with a short cervix (AUC of 0.75).
Figure 4. Receiver operating characteristic (ROC) curves showing the cervical elastography indexGE (triangle) as predictor of cervical dilatation time >7 h for (A) all included women, (n = 27), and (B) nullipara (n = 19). CO (0.13) indicates the cut off giving the maximum Youden value (enlarged black triangle) [Citation30]. (C) Cervical dilatation time from 4 to 10 cm (hours) in relation to the cervical elastography indexGE for nullipara.
![Figure 4. Receiver operating characteristic (ROC) curves showing the cervical elastography indexGE (triangle) as predictor of cervical dilatation time >7 h for (A) all included women, (n = 27), and (B) nullipara (n = 19). CO (0.13) indicates the cut off giving the maximum Youden value (enlarged black triangle) [Citation30]. (C) Cervical dilatation time from 4 to 10 cm (hours) in relation to the cervical elastography indexGE for nullipara.](/cms/asset/aade988c-0024-499f-823f-2b8b70382d9a/ijmf_a_2213797_f0004_c.jpg)
Table 4. Among women undergoing induction of labor, the associations between cervical length, CEIGE and Bishop Score and the outcomes durations of latency phase and active phase are expressed by the area under the ROC curve (AUC) with 95% CI.
Reproducibility: The reproducibility of the CEIGE assessments was evaluated by two examiners (CRT, MSSJ), revealing an intraobserver LoA of ±0.056 (95% CI; ±0.045 to ±0.069) and an ICC of 0.93 (95% CI; 0.88–0.96), whereas the interobserver LoA was −0.135 (95% CI; −0.246 to −0.023) to 0.217 (95% CI; 0.106–0.329) and the ICC 0.49 (95% CI;−0.22 to 0.87).
Discussion
This feasibility study led to two main findings. First, among women with uncomplicated pregnancies, the CSIGE decreased at a lower gestational age than the cervical length. Second, among women undergoing labor induction at term, CEIGE is superior to the Bishop score as well as to the ultrasound-measured cervical length regarding the prediction of a cervical dilatation time from 4 to 10 cm exceeding 7 h.
The study is limited by the small number of women included. Furthermore, we excluded a high number of scans (30% in both study groups). Most probably this fraction could be reduced considerably by increasing the number of elastography recordings per scan (2–3 in this study). This would allow us to exclude more elastography recordings without compromising the scan of a specific woman. As each elastography recording takes less than 20 s, the number could be doubled without unacceptable discomfort for the woman.
Our results indicate that quantitative strain elastography may give important information about a uterine cervix with normal or near-normal length. Thus, the CSIGE decreased at a much lower gestational age than the cervical length (). In fact, the cervical length is almost unchanged until a few weeks before the start of labor [Citation19–22], whereas we found that the CSIGE already decreases at approximately gestational week 32 + 0 (). This indicates that the cervix does not shorten until the normal stress of the pregnancy exceeds the biomechanical strength of the cervix [Citation1]. Thus, CSIGE may constitute an additional biomarker for risk of preterm cervical softening; i.e. a biomarker which is informative even when the length of the cervix is still normal [Citation23].
The potential clinical perspective of quantitative strain elastography regarding the evaluation of the cervix with normal length is also illustrated by the results from women undergoing labor induction. Among these women, the CEIGE was not a good predictor of a relatively long timespan (>24 h) from labor induction to a cervical dilatation of 4 cm. Regarding this outcome, the Bishop score and the cervical length assessment performed much better. The reason for this is probably that these women had rather different cervical lengths; some had longer cervices and some had shorter cervices; likewise, some had closed cervical canals whereas some had initiated cervical dilatation (the most informative assessment included in the Bishop score) [Citation24]. The CEIGE may perform much better on women undergoing labor induction in spite of a low Bishop score and a normal cervical length, i.e. with similar cervical lengths. If so, that may be clinically relevant as these women are challenging regarding the planning of labor induction.
While the biometries of the cervices of the women undergoing labor induction were rather similar, i.e. when they had reached a cervical dilatation of 4 cm or more, the CEIGE performed better than the Bishop score and the cervical length regarding the prediction of a relatively long timespan (>7 h) from 4 to 10 cm cervical dilatation. For nulliparous women, the AUC was 0.94. This is even better than what has been obtained with two other approaches on quantitative strain elastography [Citation6,Citation25]. However, the outcome “relatively long duration of the active phase” is only a potential proxy measurement for failed labor induction. Therefore, larger cohort studies are needed to evaluate the clinical implication of this finding.
It is remarkable that women with low Bishop scores and long cervices had a shorter instead of a longer timespan from 4 to 10 cm cervical dilatation. A likely explanation for this is that these women have stronger labor contractions when they reach 4 cm cervical dilatation. Notably, these findings confirm a detailed analysis from 1982 of the elements included in the Bishop score [Citation24]. This study showed that the cervical length (digitally assessed as 0, 1, 2 or 3 cm) predicted “the period of latency” much better than the “duration of labor”, whereas the cervical consistency (digitally assessed as soft, medium or firm) predicted “duration of labor” much better than “the period of latency”.
A strong alternative to quantitative strain elastography is shear wave elastography, which is already implemented at more ultrasound platforms and has been studied regarding the evaluation of adenomyosis, leiomyoma, polyps, endometrial cancer, cervical cancer, cervical ripening and risk of preterm birth [Citation26–29]. In spite of promising results, much larger studies are needed to compare the performances of the two approaches. However, future studies on quantitative strain elastography must await improvement of the method, including adjustment of the protocol, the applicability to other ultrasound platforms, the access to the algorithms in the ultrasound machines including automation of the strain curve selections, the incorporation of the force-measuring device in the ultrasound probe, and eventually the development of a feature ensuring standardized compressions and decompressions during the scans, which may improve the interobserver reliability. Also the performance of shear wave elastography may benefit from the application of a force measuring device as it remains unclarified whether the pressure applied to the tissue affects also these results. This needs to be studied. Furthermore, other alternatives including biomechanical devices should also be considered [Citation3,Citation4].
Conclusion
This feasibility study demonstrates that quantitative strain elastography based on the algorithm of the ultrasound machine performs better than the Bishop score and the cervical length; thus, it deserves further study.
Acknowledgement
We would like to thank photographer Helle Brandstrup Larsen, Randers Regional Hospital, for providing us with the photos of the force measuring device.
Disclosure statement of interest
All authors declare: (1) no support from companies for the submitted work; (2) no relationships with companies that might have an interest in the submitted work in the previous 3 years; (3) no financial relationship of their spouses, partners, or children that may be relevant to the submitted work; and (4) no financial interests that may be relevant to the submitted work.
Additional information
Funding
References
- Word RA, Li XH, Hnat M, et al. Dynamics of cervical remodeling during pregnancy and parturition: mechanisms and current concepts. Semin Reprod Med. 2007;25(1):69–79. Jan
- Kolkman DG, Verhoeven CJ, Brinkhorst SJ, et al. The bishop score as a predictor of labor induction success: a systematic review. Am J Perinatol. 2013;30(8):625–630. Sep
- Hee L. Overview of the methods available for biomechanical testing of the uterine cervix in vivo. Acta Obstet Gynecol Scand. 2014;93(12):1219–1237.
- Feltovich H, Carlson L. New techniques in evaluation of the cervix. Semin Perinatol. 2017;41(8):477–484.
- Fruscalzo A, Mazza E, Feltovich H, et al. Cervical elastography during pregnancy: a critical review of current approaches with a focus on controversies and limitations. J Med Ultrason. 2016;43(4):493–504.
- Thomsen CR, Jensen MSS, Leonhard AK, et al. A force-measuring device combined with ultrasound-based elastography for assessment of the uterine cervix. Acta Obstet Gynecol Scand. 2022;101(2):241–247.
- Kuusela P, Jacobsson B, Hagberg H, et al. Second trimester transvaginal ultrasound measurement of cervical length for prediction of preterm birth: a blinded prospective multicentre diagnostic accuracy study. BJOG. 2021;128(2):195–206.
- Taipale P, Hiilesmaa V. Sonographic measurement of uterine cervix at 18–22 weeks’ gestation and the risk of preterm delivery. Obstet Gynecol. 1998;92(6):902–907.
- Davies G, Ottenhof C, Woodman M, et al. Cervix length and relaxin as predictors of preterm birth. J Obstet Gynaecol Can. 2008;30(12):1124–1131.
- Iams JD, Goldenberg RL, Meis PJ, et al. The length of the cervix and the risk of spontaneous premature delivery. National institute of child health and human development maternal fetal medicine unit network. N Engl J Med. 1996;334(9):567–572.
- Carvalho MH, Bittar RE, Brizot ML, et al. Cervical length at 11–14 weeks’ and 22-24 weeks’ gestation evaluated by transvaginal sonography, and gestational age at delivery. Ultrasound Obstet Gynecol. 2003;21(2):135–139.
- Leung TN, Pang MW, Leung TY, et al. Cervical length at 18–22 weeks of gestation for prediction of spontaneous preterm delivery in Hong Kong Chinese women. Ultrasound Obstet Gynecol. 2005;26(7):713–717.
- Verhoeven CJ, Opmeer BC, Oei SG, et al. Transvaginal sonographic assessment of cervical length and wedging for predicting outcome of labor induction at term: a systematic review and meta-analysis. Ultrasound Obstet Gynecol. 2013;42(5):500–508.
- Uldbjerg N, Ekman G, Malmstrom A, et al. Ripening of the human uterine cervix related to changes in collagen, glycosaminoglycans, and collagenolytic activity. Am J Obstet Gynecol. 1983;147(6):662–666.
- Bishop EH. Pelvic scoring for elective induction. Obstet Gynecol. 1964;24:266–268.
- The Fetal Medicine Foundation. Cervical assessment–protocol for measurement. Available from: https://fetalmedicine.org/cervical-assessment-1
- Myers K, Socrate S, Tzeranis D, et al. Changes in the biochemical constituents and morphologic appearance of the human cervical stroma during pregnancy. Eur J Obstet Gynecol Reprod Biol. 2009;144(Suppl 1):S82–S9.
- Harris PA, Taylor R, Minor BL, et al. The REDCap consortium: building an international community of software platform partners. J Biomed Inform. 2019;95:103208.
- Wulff CB, Rode L, Rosthoj S, et al. Transvaginal sonographic cervical length in first and second trimesters in a low-risk population: a prospective study. Ultrasound Obstet Gynecol. 2018;51(5):604–613.
- Iams JD, Cebrik D, Lynch C, et al. The rate of cervical change and the phenotype of spontaneous preterm birth. Am J Obstet Gynecol. 2011;205(2):130.e1–130.e6.
- Papastefanou I, Pilalis A, Kappou D, et al. Cervical length at 11–40 weeks: unconditional and conditional longitudinal reference ranges. Acta Obstet Gynecol Scand. 2016;95(12):1376–1382.
- Salomon LJ, Diaz-Garcia C, Bernard JP, et al. Reference range for cervical length throughout pregnancy: non-parametric LMS-based model applied to a large sample. Ultrasound Obstet Gynecol. 2009;33(4):459–464.
- Sundtoft I, Langhoff-Roos J, Sandager P, et al. Cervical collagen is reduced in non-pregnant women with a history of cervical insufficiency and a short cervix. Acta Obstet Gynecol Scand. 2017;96(8):984–990.
- Lange AP, Secher NJ, Westergaard JG, et al. Prelabor evaluation of inducibility. Obstet Gynecol. 1982;60(2):137–147.
- Hee L, Rasmussen CK, Schlutter JM, et al. Quantitative sonoelastography of the uterine cervix prior to induction of labor as a predictor of cervical dilation time. Acta Obstet Gynecol Scand. 2014;93(7):684–690.
- Carlson LC, Hall TJ, Rosado-Mendez IM, et al. Quantitative assessment of cervical softening during pregnancy with shear wave elasticity imaging: an in vivo longitudinal study. Interface Focus. 2019;9(5):20190030.
- Masso P, Callejas A, Melchor J, et al. In vivo measurement of cervical elasticity on pregnant women by torsional wave technique: a preliminary study. Sensors. 2019;19(15):3249.
- Manchanda S, Vora Z, Sharma R, et al. Quantitative sonoelastographic assessment of the normal uterus using shear wave elastography: an initial experience. J Ultrasound Med. 2019;38(12):3183–3189.
- Agarwal S, Agarwal A, Joon P, et al. Fetal adrenal gland biometry and cervical elastography as predictors of preterm birth: a comparative study. Ultrasound. 2018;26(1):54–62.
- Schisterman EF, Perkins NJ, Liu A, et al. Optimal cut-point and its corresponding youden index to discriminate individuals using pooled blood samples. Epidemiology. 2005;16(1):73–81.