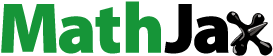
Abstract
Objective
To investigate the effects of chromosome polymorphism on the clinical outcomes of in vitro fertilization/embryo transfer (IVF/ET)-assisted reproductive technology.
Methods
The case data of 2740 patients treated between January 2018 and January 2019 were retrospectively analyzed. The patients were organized into two groups: a case group and a control group. In the case group (n = 81), one or both parents were characterized by chromosomal polymorphism; in the control group (n = 2659), both parents had normal chromosome karyotyping. The primary outcomes included clinical pregnancy rate (clinical pregnancy rate of fresh transfer cycles = number of clinical pregnancy cycles/number of fresh embryo transfer cycles × 100%) and live birth rate (live birth rate per fresh transfer cycles = number of live births/numbers of fresh embryo transfer cycles × 100%). The propensity score matching (PSM) method was used for statistical analysis.
Results
After PSM 1:2 matching for the patients in the two groups, 72 patients were successfully matched. The clinical pregnancy rate and live birth rate in the case group were lower than in the control group before PSM (clinical pregnancy rate: 33.30% case group vs. 46.60% control group, p = .020; live birth rate: 30.90% case group vs. 47.90% control group, p = .03). The differences were statistically significant (p < .05). The live birth rate in the case group was also significantly lower than in the control group after PSM (34.98% case group vs. 74.52% control group; p = .028). The correlation coefficient between clinical pregnancy and grouping (i.e. if there was a characteristic chromosome polymorphism) was −.045 (p = .02), while the correlation coefficient between live birth and grouping was −.046.
Conclusion
Chromosome polymorphism is weakly negatively correlated with live birth in IVF/ET-assisted reproduction and can significantly reduce the live birth rate of patients.
1. Introduction
Chromosomal polymorphism refers to small and constant non-pathological variations in the heterochromatic region of chromosomes, including variations in the structure, width of bands, and staining intensity that is widespread, follow Mendelian inheritance, and are considered to be a type of non-pathological chromosomal variation [Citation1]. It most commonly occurs in centromeres, entomirs, secondary constriction, and Y chromosomes, which are mainly composed of highly repetitive satellite DNA sequences that do not encode proteins; as such, chromosomal polymorphisms were initially considered normal karyotypic variations [Citation2]. Heterochromatin also plays an important role in spindle attachment, chromosome movement, meiotic pairing, and sister chromatid condensation [Citation3].
Polymorphic variation in non-proximal chromosomes usually occurs in heterochromatin beside the long arms of chromosomes 1, 9, and 16, in the short arm regions of group D and G chromosomes, and in the distal heterochromatin of the Y chromosome. The lengths of the increased heterochromatic regions in the long arm of these chromosomes are 1qh +, 9qh +, 16qh +, and Yqh + [Citation4].
It has been reported that the detection rate of chromosomal polymorphism in the normal population is 1.77% [Citation4]. Approximately 8%–12% of the reproductive-age population worldwide faces infertility problems. With the increasing incidence of infertility, assisted reproductive technology is becoming more widely used, and the relationship between chromosomal polymorphism and infertility has attracted much attention. Chromosomal polymorphism may be associated with infertility, recurrent early miscarriages, and neonatal malformations [Citation5]. In terms of gametogenesis, it may also affect homologous chromosome synapsis, which causes reproductive abnormalities like infertility, stillbirth, abortion, and offspring malformations [Citation4,Citation6]. However, the question of whether chromosomal polymorphism has an impact on the outcome of assisted pregnancy remains controversial, and how to scientifically and rationally elucidate its clinical significance and give appropriate advice to patients with the condition are problems that must be faced by reproductive genetics and obstetricians.
1.1. Objective
The present study aimed to investigate the effects of chromosome polymorphism on the clinical outcomes of in vitro fertilization/embryo transfer (IVF/ET)-assisted reproductive technology and provide a theoretical basis for the clinical application of this technology.
2. Subjects and methods
2.1. Clinical data
The data of 2740 patients who were treated in the Reproductive Medicine Center of the Shanxi Maternal and Child Health Hospital and the Reproductive Medicine Center of Tianjin Central Hospital of Gynecology and Obstetrics between January 2018 and January 2019 were retrospectively analyzed. The patients were organized into two groups: a case group and a control group. In the case group (n = 81), one or both of the parents were characterized by chromosomal polymorphism (confirmed by karyotype analysis) and met the inclusion criteria. In the control group (n = 2659), both parents had normal chromosome karyotyping and met the inclusion criteria.
2.2. Inclusion and exclusion criteria
Inclusion criteria: (1) age of parents ≤40 years; (2) in vitro fertilization (IVF) or intracytoplasmic sperm injection (ICSI) used for fertilization; (3) treatment protocols included long or antagonist options; (4) first egg retrieval cycle selected based on laboratory-related index statistics; (5) fresh embryo transfer cycle selected for clinical indices and statistics; (6) complete follow-up data were available.
Exclusion criteria: (1) uterine abnormalities, including uterine deformities, endometrial polyps, adenomyosis, uterine leiomyoma, scarred uterus, and cervical conization; (2) ovarian abnormalities, including unilateral ovarian absence, ovarian cysts, and polycystic ovary syndrome; (3) abnormal chromosomes in either parent; (4) endocrine diseases, including thyroid disease, hyperprolactinemia, and diabetes; (5) immunological diseases; (6) cases with habits like smoking or alcohol or history of the drugs that may affect.
2.3. Methodology
2.3.1. Karyotype analysis
All patients underwent routine karyotype analysis in the Reproductive Medicine Center of the Shanxi Maternal and Child Health Hospital and the Reproductive Medicine Center of Tianjin Central Hospital of Gynecology and Obstetrics. In each case, the peripheral blood lymphocytes of both parents were cultured for 68–72 h. After the administration of colchicine and hypotonic treatment, the collected cells were treated with G-banding. Karyotype analysis [Citation7] was performed for the results based on the international naming system of human cytology.
2.3.2. IVF/ET-assisted reproductive treatment
Ovulation induction was performed using a standard long protocol or antagonist protocol. A vaginal ultrasound was performed to detect follicular development. When two or three dominant follicles with a diameter ≥18 mm were found, an intramuscular injection of human chorionic gonadotropin (hCG) was given as a trigger. A transvaginal puncture was conducted for egg retrieval under a B-ultrasound 36 h after the injection. The IVF/ICSI fertilization was performed based on the semen test results. All patients underwent IVF/ET on the third day after egg extraction, following an assessment of their general condition, ovulation induction, and laboratory embryo development. Regular luteal support was given from the day of egg retrieval.
2.3.3. Follow-up clinical outcomes
Biochemical pregnancy was determined by a urine pregnancy test or hCG test14 days after IVF/ET. If a gestational sac could be identified by transvaginal ultrasonography four weeks after transplantation, it was classed as clinical pregnancy, and the patient was followed up to a pregnancy outcome.
2.3.4. Observational indexes
The general data of patients in the case group and control group included the type, causes, and years of infertility, age, body mass index (BMI), and basic hormone levels of the gestational parent, GN-related indexes of ovulation induction, and endometrial thickness on trigger days. The primary outcomes included clinical pregnancy rate (clinical pregnancy rate of fresh transfer cycles = number of clinical pregnancy cycles/number of fresh embryo transfer cycles × 100%) and live birth rate (live birth rate per fresh transfer cycles = number of live births/number of fresh embryo transfer cycles × 100%). The secondary outcomes included laboratory-related indexes (the number of egg retrials, mature eggs, fertilized eggs, normal fertilized eggs, normal cleavage, available embryos, superior embryos, blastocysts, transplanted embryos, and implantation embryos) and pregnancy-related indexes (biochemical pregnancy rate, early abortion rate [early abortion rate = number of cycles of spontaneous abortion within 12 weeks of pregnancy/number of clinical pregnancy cycles × 100%], late abortion rate [late abortion rate = number of abortion cycles from 12 weeks to less than 28 weeks/number of clinical pregnancy cycles × 100%], and ectopic pregnancy rate [ectopic pregnancy rate = number of ectopic pregnancy cycles/number of clinical pregnancy cycles × 100%]).
2.3.5. Statistical methods
SPSS Statistics software version 23.0 was used for statistical analysis. First, the proportion and incidence of different polymorphic karyotypes detected in patients were analyzed in the case group. The dosage and time of the controlled ovarian hyperstimulation medications were then matched with the patients of both groups under a ratio of 1:2 using a propensity score matching (PSM) method according to the patient’s general characteristics and laboratory indexes, including the gestational parent’s age, type of infertility, years of infertility, BMI, and basic endocrine hormone levels. The caliper value was set as .001. The results were demonstrated and analyzed before and after matching. The categorical data were expressed as a rate or a percentage. A χ2 test was then used for comparison. Continuous variables were expressed as the mean ± standard deviation, ± s, while non-normally distributed measurement data were expressed as the median (P25, P75). The normality of continuous variables was tested by the Shapiro–Wilk test as well as the graphical illustration of histograms and Q–Q plots. A t-test was used for continuous variables that conformed to the normal distribution, and the Mann–Whitney test was used for the comparison of continuous variables that did not conform to the normal distribution. Spearman’s correlation analysis was used to analyze the association of PSM pre-chromosomal polymorphism with clinical pregnancy and live birth. All hypothesis test levels were set to α = .05.
3. Results
3.1. Subject data
A total of 81 patients with chromosomal polymorphism met the inclusion criteria and were enrolled, while 2659 patients were included in the control group based on the inclusion and exclusion criteria. All patients had complete follow-up data and were followed up with to the end of the pregnancy.
3.2. Type and incidence of chromosomal polymorphism
The 81 patients with chromosomal polymorphism were divided into four groups based on the results of the karyotype analysis: a chromosome 1, 9, and 16 abnormalities group (n = 45), a D/G abnormalities group (n = 29), a Y chromosome abnormalities group (n = 5), and a complex polymorphism group (two types of chromosomal polymorphism in the same case) (n = 2). The incidence of chromosome polymorphism in the cases treated at the two centers was 2.95%. The karyotype with the highest incidence was chromosome 9 pericentric inversion (inv [Citation3]) (.72%), while the karyotypes with the lowest incidence were an increase in the chromosome short arm in the heterochromatin region (.07%) of the D/G abnormalities group, an increase and decrease in the long-arm secondary constriction region of chromosome 1 (.07%), and complex polymorphism (.07%). The number and proportion of the various types of chromosomal polymorphism detected are shown in .
Table 1. Chromosome polymorphism and its incidence.
3.3. Comparison of baseline characteristics before and after PSM
Before PSM, there were 81 patients in the case group and 2659 patients in the control group. The differences in the type of infertility (p = .029), factors of infertility (p = .021), basal luteinizing hormone (p = 0), basal prolactin (p = 0), and basal T (p = 0) were statistically significant at the baseline before matching (p < .05; see ). After PSM 1:2 matching for the patients in the two groups, 72 patients were successfully matched. The differences between the two groups in baseline characteristics were not statistically significant after PSM 1:2 matching (p > .05; see ).
Table 2. Comparison of general baseline characteristics of patients.
3.4. Comparison of laboratory-related indexes before and after PSM
The differences between the two groups in number of superior embryos (p = .036), transplanted embryos (p = .005), and implanted embryos (p = .004) were statistically significant (p < .05). The differences in other indexes, such as the number of egg retrials, mature eggs, fertilized eggs, normal fertilized eggs, normal cleavage, available embryos, and blastocysts, were not statistically significant (p > .05; see ). The differences between the two groups in each laboratory index after PSM were not statistically significant (p > .05; see ).
Table 3. Comparison of relevant indicators in the laboratory.
3.5. Comparison of pregnancy outcome-related indexes before and after PSM
Before PSM, the clinical pregnancy rate was 33.30% in the case group and 46.60% in the control group, and the difference was statistically significant (p = .02). The live birth rate was 24.70% in the case group and 28.00% in the control group, and the difference was statistically significant (p = .015). The differences between the two groups in other indexes, such as biochemical pregnancy rate, early abortion rate, late abortion rate, and ectopic pregnancy rate, were not statistically significant (p > .05; see ).
Table 4. Comparison of pregnancy outcomes.
After PSM, the clinical pregnancy rate was 39.63% in the case group and 40.80% in the control group, and the difference was not statistically significant (p = .922). The live birth rate was 34.98% in the case group and 74.52% in the control group, with the live birth rate of the control group being significantly higher than that of the case group (p < .001; see ).
3.6. Correlation analysis of chromosomal polymorphism with clinical pregnancy and live birth after PSM
The control group was defined as 0 and the case group as 1. Clinical pregnancy and live birth were regarded as the grade variables: no pregnancy or biochemical pregnancy were defined as 0, clinical pregnancy was defined as 1, cases without live birth were defined as 0, and cases with live birth were defined as 1. After PSM, a correlation analysis of chromosomal polymorphism with clinical pregnancy and live birth was performed. The correlation coefficient between clinical pregnancy and grouping (i.e. if there was a characteristic chromosome polymorphism) was −.045 (p = .02), while the correlation coefficient between live birth and grouping was −.046 (p = .015; see ).
Table 5. Correlation analysis (N = 2740).
4. Discussion
In recent years, studies have found that the incidence of chromosome polymorphism in infertile patients is higher than in fertile patients and that the overall incidence of chromosome heterogeneity is higher in infertile patients [Citation3]. Hong et al. compared the outcomes of assisted reproduction between a female polymorphic group, a male polymorphic group, and a non-polymorphic group, finding no difference in implantation rate or clinical pregnancy rate [Citation1]. Xiao et al. studied Yqh − polymorphism, finding that the reproductive outcomes of couples carrying Yqh − are significantly worse and that their cycle cancelation rate is significantly higher [Citation8].
Dong et al. found that the frequency of chromosome polymorphism in the infertile population was 2.74% [Citation9], while Liang et al. reported the frequency in infertile patients was 3.17% [Citation10]. In the present study, the total frequency of chromosome polymorphism was 2.95%, which is consistent with the results of the correlational studies. However, a seven-year retrospective study by Cheng et al. found that the incidence of chromosome polymorphism was 5.53% in infertile patients, which is significantly higher than in the normal population. The same study also found that the variant with the highest frequency of overall polymorphism was 9 qh+, followed by inv (9), which is the most common type of chromosome polymorphism in patients with endometriosis [Citation11]. In the present study, the detection rate of chromosome polymorphism in the infertile population is higher than in normal population, and the most common variant is inv (9). Chromosome polymorphism is likely to be correlated with infertility.
Ou et al. conducted a meta-analysis of eight case-controlled studies, finding that only male chromosome polymorphism negatively affects fertilization, cleavage, and high-quality embryo rate in assisted reproduction [Citation12]. Li et al. discovered that the variation frequency of Yqh in infertile and fertile patients may increase with the decrease of sperm count and that the frequency of Yqh in azoospermia patients increases significantly [Citation13]. Some studies have also found that couples carrying Yqh − have lower cycle cancelation rates, fertilization rates, implantation rates, and high-quality embryo rates [Citation8]. This suggests that Y-chromosome polymorphism may affect sperm quality and quantity and potentially adversely affect fertilization and embryo development.
Previous studies have shown that the use of ICSI fertilization can improve the clinical outcomes of male patients with chromosomal polymorphisms [Citation8,Citation14]. This is because male Y-chromosome polymorphism is the main factor affecting the fertilization process in partial patients.
Ni et al. found that the pregnancy outcome after IVF treatment was poor in couples with chromosomal polymorphism in the sperm-giving parent, with the cumulative live birth rate per cycle being significantly reduced and the cumulative early spontaneous abortion rate being high [Citation11, Citation15]. This is consistent with the results of the present study, in which the difference in the live birth rate after PSM was still present. This suggests that the chromosome polymorphism of parents may have an effect on embryo quality and fetal development and that the possibility of fetal malformation and abnormal development is increased, thereby leading to a decrease in the live birth rate.
A study by Schmid et al. showed that 1, 9, 16, and Y variations in the heterochromatin regions are associated with embryonic developmental disorders [Citation16]. A retrospective study by Sun et al. found that chromosome polymorphism is independently associated with the multinucleated embryogenesis of the blastomere and with poor-quality multinucleated embryos and dysontogenesis [Citation17,Citation18]. Accordingly, chromosome polymorphism can be considered to be related to an increase in embryo aneuploidy rate and the impairment of embryonic development potential, which leads to the embryo being unable to enter the normal development pathway, making it prone to early pregnancy abortion and complications for the gestational parent during pregnancy. This eventually causes a decline in the live birth rate. The results of the present study’s correlation analysis show that all correlation coefficients between polymorphism and clinical pregnancy and live birth are negative, indicating that chromosome polymorphism has adverse effects on live birth. This is consistent with previous findings [Citation15,Citation19].
Structural heterochromatin is mainly located in the telomeres, centromeres, and peripheral regions of chromosomes, and it does not encode proteins. It is very important for genome stability and chromosome separation [Citation20]. A polymorphic variation in heterochromatin may therefore cause meiosis abnormalities and impair gamete formation [Citation20,Citation21]. Some studies have found that heterochromatin associated with chromosomal polymorphism can inhibit or even silence the gene expression of euchromatin [Citation22,Citation23].
Pericentric inv (9) is considered one of the most common mutations in the human karyotype [Citation11,Citation24], which is consistent with the results of this study. The occurrence of recombinant heteroploidy due to certain types of pericentric inversions has been described in previous literature [Citation25]. It has been suggested that inv (9) has an inter-chromosomal effect that leads to mitotic disorders and an increased risk of aneuploidy. Furthermore, during meiosis, inv (9) may interfere with the pairing of homologous chromosomes [Citation26]. Some reports have suggested that genes located at 9p13, such as Talin1 and MELK, may be associated with early embryo implantation and endometrial receptivity [Citation27]. In the present study, the highest incidence of inv (9) was found in the case group (24.69%). This polymorphism may lead to a decrease in gamete and/or embryo quality by affecting cell division. It may also damage the gene expression related to endometrial receptivity in chromosome 9, ultimately affecting live birth. However, the molecular mechanism still needs to be validated by scientific molecular biological experiments.
The advantage of the current study is that the PSM method was used to make the baseline characteristics of patients comparable, avoid confounding factors, reduce bias, and make the conclusion more reliable. The correlation analysis was also used to confirm the effect of chromosomal polymorphism on the clinical outcome of assisted reproduction. The disadvantage of this study is that it was a single-center retrospective cohort study, and the number of case group is little in comparison with control group, and analysis of each chromosome polymorphism subgroup was not undertaken due to the limited sample size. More multi-center, large-sample prospective studies are needed to further confirm the conclusion.
5. Conclusion
The incidence of chromosomal polymorphism in infertile patients is higher than in the normal population. Chromosomal polymorphism is weakly negatively associated with live births in couples receiving IVF/ET-assisted reproductive technology. Therefore, for couples who intend to undergo IVF/ET-assisted reproduction, a preoperative examination of the karyotype is necessary. For couples in whom chromosomal polymorphism is detected, targeted genetic counseling and pregnancy guidance are recommended.
Acknowledgements
We would like to acknowledge the hard and dedicated work of all the staff that implemented the intervention and evaluation components of the study.
Disclosure statement
No potential conflict of interest was reported by the author(s).
Additional information
Funding
References
- Hong Y, Zhou YW, Tao J, et al. Do polymorphic variants of chromosomes affect the outcome of in vitro fertilization and embryo transfer treatment? Hum Reprod. 2011;26(4):933–940. doi: 10.1093/humrep/deq333.
- Mierla D, Stoian V. Chromosomal polymorphisms involved in reproductive failure in the romanian population. Balkan J Med Genet. 2012;15(2):23–28. doi: 10.2478/bjmg-2013-0003.
- Madon PF, Athalye AS, Parikh FR. Polymorphic variants on chromosomes probably play a significant role in infertility. Reprod Biomed Online. 2005;11(6):726–732. doi: 10.1016/s1472-6483(10)61691-4.
- Wilson A, Watt K, Ma S. The incidence of long heterochromatic polymorphism variants in infants conceived through assisted reproductive technologies. Reprod Biomed Online. 2017;35(2):219–224. doi: 10.1016/j.rbmo.2017.04.008.
- Sahin FI, Yilmaz Z, Yuregir OO, et al. Chromosome heteromorphisms: an impact on infertility. J Assist Reprod Genet. 2008;25(5):191–195. doi: 10.1007/s10815-008-9216-3.
- Zickler D, Kleckner N. Recombination, pairing, and synapsis of homologs during meiosis. Cold Spring Harb Perspect Biol. 2015;7:a016626. doi: 10.1101/cshperspect.a016626.
- Shaffer LG, Mcgowanjordan J, Schmid M, et al. ISCN: an international system for human cytogenetic nomenclature (2013). Cytogenet Genome Res. 2012;
- Xiao Z, Zhou X, Xu W, et al. A preliminary study of the relationship between the long arm of the Y chromosome (Yqh+) and reproductive outcomes in IVF/ICSI-ET. Eur J Obstet Gynecol Reprod Biol. 2012;165(1):57–60. doi: 10.1016/j.ejogrb.2012.07.004.
- Dong Y, Jiang YT, Du RC, et al. Impact of chromosomal heteromorphisms on reproductive failure and analysis of 38 heteromorphic pedigrees in Northeast China. J Assist Reprod Genet. 2013;30(2):275–281. doi: 10.1007/s10815-012-9910-z.
- Liang J, Zhang Y, Yu Y, et al. Effect of chromosomal polymorphisms of different genders on fertilization rate of fresh IVF–ICSI embryo transfer cycles. Reprod Biomed Online. 2014;29(4):436–444. doi: 10.1016/j.rbmo.2014.06.011.
- Cheng R, Ma Y, Nie Y, et al. Chromosomal polymorphisms are associated with female infertility and adverse reproductive outcomes after infertility treatment: a 7-year retrospective study. Reprod Biomed Online. 2017;35(1):72–80. doi: 10.1016/j.rbmo.2017.03.022.
- Ou Z, Yin M, Chen Z, et al. Meta-analysis of the association between chromosomal polymorphisms and outcomes of embryo transfer following in vitro fertilization and/or intracytoplasmic sperm injection. Int J Gynaecol Obstet. 2019;144(2):135–142. doi: 10.1002/ijgo.12702.
- Li LL, Peng D, Wang RX, et al. Correlation between chromosomal polymorphisms and male infertility in a Northeast Chinese population. Genet Mol Res. 2015;14(4):15435–15443. doi: 10.4238/2015.November.30.21.
- Li SJ, Cheng YX, Ye-Shang , Zhou DN, Zhang Y, Yin TL, Yang J. Chromosomal polymorphisms associated with reproductive outcomes after IVF-ET. J Assist Reprod Genet. 2020;37 (7): 1703–1710. doi: 10.1007/s10815-020-01793-8.
- Ni T, Li J, Chen H, et al. Male chromosomal polymorphisms reduce cumulative live birth rate for IVF couples. J Assist Reprod Genet. 2017;34(8):1017–1025. doi: 10.1007/s10815-017-0951-1.
- Schmid M, Vogel W, Krone W. Heterochromatic regions of chromosomes 1, 9, 16 and Y in children with signs of embryonic development disorder. Genetika. 1982;18(12):2052–2054.
- Sun L, Chen ZH, Yang L, et al. Chromosomal polymorphisms are independently associated with multinucleated embryo formation. J Assist Reprod Genet. 2018;35(1):149–156. doi: 10.1007/s10815-017-1037-9.
- Li S, Zhou D, Zhang Y, et al. Polymorphic variants of chromosomes decrease cleaved embryos rate in vitro fertilization and embryo transfer treatment. Fertil Steril. 2018;110(4):e143. doi: 10.1016/j.fertnstert.2018.07.422.
- Xu X, Zhang R, Wang W, et al. The effect of chromosomal polymorphisms on the outcomes of fresh IVF/ICSI–ET cycles in a chinese population. J Assist Reprod Genet. 2016;33(11):1481–1486. doi: 10.1007/s10815-016-0793-2.
- Janssen A, Colmenares SU, Karpen GH. Heterochromatin: guardian of the genome. Annu Rev Cell Dev Biol. 2018;34:265–288. doi: 10.1146/annurev-cellbio-100617-062653.
- Sullivan LL, Chew K, Sullivan BA. α satellite DNA variation and function of the human centromere. Nucleus. 2017;8(4):331–339. doi: 10.1080/19491034.2017.1308989.
- Ho JW, Jung YL, Liu T, et al. Comparative analysis of metazoan chromatin organization. Nature. 2014;512(7515):449–452. doi: 10.1038/nature13415.
- van de Werken C, van der Heijden GW, Eleveld C, et al. Paternal heterochromatin formation in human embryos is H3K9/HP1 directed and primed by sperm-derived histone modifications. Nat Commun. 2014;5:5868. doi: 10.1038/ncomms6868.
- Šípek A, Jr, Panczak A, Mihalová R, et al. Pericentric inversion of human chromosome 9 epidemiology study in Czech males and females. Olia Biol. 2015;61 (4):140–146.
- Anton E, Blanco J, Egozcue J, et al. Sperm studies in heterozygote inversion carriers: a review. Cytogenet Genome Res. 2005;111(3–4):297–304. doi: 10.1159/000086903.
- Murthy SK, Prabhakara K. Mitotic disturbances associated with inversion 9qh. A case report. Annales De Génétique. 1990;33(3):169–172. doi: 10.1002/ajmg.1320350131.
- Lerer I, Sagi M, Meiner V, et al. Deletion of the ANKRD15 gene at 9p24.3 causes parent-of-origin-dependent inheritance of familial cerebral palsy. Hum Mol Genet. 2005;14(24):3911–3920. doi: 10.1093/hmg/ddi415.