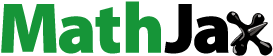
Abstract
Objectives
We have previously described gestational-age-independent sonographic indices to assess fetal lung size in the right and left lungs: The Quantitative Lung Index for the right lung (QLI-R) and for the left lung (QLI-L), respectively. The purpose of this study was to evaluate the clinical cutoff point of the QLI-R to predict pulmonary hypoplasia and neonatal death.
Materials and Methods
Retrospective assessment of the QLI-R in patients with left-sided congenital diaphragmatic hernia (CDH-L) and other fetal conditions at risk for fetal pulmonary hypoplasia. Cross-section and longitudinal assessment of the behavior of the QLI-R in untreated and treated patients. ROC curve analysis to determine the optimal cutoff point of the QLI-R in predicting neonatal death.
Results
One hundred eighteen patients with CDH-L and other fetal conditions at risk for pulmonary hypoplasia had QLI-R measurements done. Seventeen patients were excluded for various reasons. Eleven patients with conditions other than CDH-L but at risk for pulmonary hypoplasia were used for intraclass coefficient measurements of the QLI-R. Ninety patients had CDH-L, of which 78 did not undergo antenatal intervention and in which the cutoff point for pulmonary hypoplasia and neonatal demise was assessed. Stent tracheal occlusion was performed in the remaining 12 patients with CDH-L, in which the behavior of the QLI after surgery was assessed. Analysis of the ICC showed an overall intra-rater reliability of 0.985 (Cronbach’s Alpha-based). There was no correlation between gestational age and QLI-R (-0.73, Pearson correlation, p = .72). Twenty-six of the 78 patients (33%) with CDH-L managed expectantly had a neonatal demise. A QLI-R equal to or less than 0.45 was significantly predictive of neonatal demise (area under the curve 0.64, p = .046, sensitivity 77%). Nine of the 12 patients (75%) that underwent tracheal occlusion had neonatal survival. Of these, 10 had serial assessments of the QLI-R after surgery. An increase in the QLI-R of 0.11 was associated with a tendency for neonatal survival (p = .056).
Conclusion
Our study confirms that the QLI-R is a gestational-age-independent measurement of fetal lung size, with a high degree of reproducibility. In a population of expectantly managed CDH-L patients, a cutoff value of the QLI-R of 0.45 or lower is predictive of neonatal death from pulmonary hypoplasia. The QLI-R can be used to monitor fetal lung growth after tracheal occlusion, and an increase in the QLI-R is suggestive of neonatal survival. Further prospective studies are needed to confirm these findings and to explore the use of the QLI in other populations at risk for pulmonary hypoplasia and consequent neonatal demise.
Introduction
Neonatal pulmonary hypoplasia is characterized by severe respiratory distress resulting from a decrease in the number of pulmonary alveoli or bronchial branching [Citation1]. The reported incidence of neonatal pulmonary hypoplasia in the general population is between 9 and 11 per 10,000 live births, and it is present in 14–20% of neonatal autopsies [Citation2]. This is likely an underestimation of the true incidence of pulmonary hypoplasia, as infants with milder forms of hypoplasia may survive beyond the neonatal period [Citation2]. Pulmonary hypoplasia can be primary (idiopathic) or secondary to congenital anomalies. Fetal conditions that may result in pulmonary hypoplasia and neonatal death include space-occupying lesions such as congenital diaphragmatic hernia (CDH) [Citation3], congenital pulmonary airway malformation (CPAM) [Citation4], bronchopulmonary sequestration (BPS) [Citation5,Citation6], congenital lobar emphysema (CLE) [Citation7], pleural or pericardial effusions [Citation8,Citation9], or oligohydramnios/anhydramnios resulting from bilateral renal agenesis [Citation10], obstructive uropathy [Citation11] or premature rupture of membranes [Citation12], among others.
The prediction of pulmonary hypoplasia is based on the antenatal use of ultrasound, MRI or both [Citation13–17]. Predicting which fetuses are at risk for neonatal demise from pulmonary hypoplasia can help classify them into those that could benefit from some form of antenatal intervention. In 1996, Metkus et al. [Citation18] introduced the lung-to-head circumference ratio (LHR) as a sonographic measure to identify CDH fetuses with poor prognosis. The LHR was calculated by dividing the product of the largest perpendicular right lung diameters at the level of the 4-chamber view of the heart (lung area, in mm2), by the head circumference (in mm). The LHR was assumed to be independent of gestational age. Subsequent studies showed that the LHR was not independent of gestational age [Citation19] and that it was unable to predict neonatal demise [Citation18]. Failure of the LHR to predict neonatal demise was assumed to reflect lack of standardization of the ultrasound methodology [Citation20].
Peralta et al. [Citation21,Citation22] in a study on 650 normal fetuses showed that the LHR increases with gestational age and is thus not independent of gestational age, as previously assumed. Therefore, using a fixed value of the LHR to differentiate fetuses at risk for pulmonary hypoplasia is not possible, unless a specific gestational age is designated for the assessment of the LHR. The authors also suggested to use percentiles of the lung area (LA) instead [Citation21]. However, percentiles of LA are also dependent on gestational age, and do not account for differences in fetal size. Jani et al. [Citation23] proposed using the observed/expected (o/e) LHR concept to predict neonatal mortality in fetuses with CDH, with the assumption that the o/e LHR corrects for gestational age. Our group has shown that the o/e LHR is also not independent of gestational age [Citation22].
We have previously shown that the reason for the increase of the LHR with gestational age is mathematical, rather than biological or due to the lack of standardized ultrasound methodology [Citation24]. The rising value of the LHR with gestational age is due to the division of measures associated with geometric concepts of different dimensions. The LA is measured in mm2 (area), whereas the HC is measured in mm (length). This division of LA by HC results in a first-degree polynomial equation, which in the case of the LHR has a persistent positive slope [Citation23]. To address this problem, our group developed an alternative sonographic index for assessing fetal lung size, the Quantitative Lung Index (QLI) [Citation25], which is independent of the gestational age. We have generated formulas to assess the right lung (QLI-R) [Citation23] and the left lung (QLI-L) [Citation26].
The QLI-R involves the division of two second-degree polynomials:
where LA is the traced lung area (mm2) of the right lung at the level of the four-chamber view and HC is the head circumference (mm), squared [Citation27]. The formula for the QLI-L is similar, but the constant is 144, instead of 100:
The QLI-R and the QLI-L can be calculated using mm or cm. The LHR can only be calculated using mm, reflecting also the mathematical flaw of this index.
Although the QLI is currently the only gestational-age independent sonographic index to assess fetal lung size, the cutoff value associated with a small lung and thus predictive of pulmonary hypoplasia and neonatal death is unknown. This is because the QLI was derived mathematically from the existing formulas for the head circumference and the lung area, rather than from a clinical sample. In our original publication, we proposed a QLI-R value of <0.6, which corresponds to the 0.1st percentile, to suggest the presence of a small lung. The purpose of the current study was to estimate the cutoff value of the QLI-R predictive of neonatal demise in a clinical setting and to evaluate the role of the QLI-R in predicting outcome after tracheal occlusion.
Materials and methods
Data for this study was collected retrospectively from three separate cohorts in Kiev, Ukraine, Mexico City, Mexico, and Miami, USA, using deidentified data. Patients underwent a comprehensive ultrasound examination that confirmed the presence of left-sided congenital diaphragmatic hernia (CDH-L) or any other condition that could result in pulmonary hypoplasia and consequent neonatal demise. A karyotype was obtained by genetic amniocentesis in all subjects. The presence of intrathoracic liver was determined by ultrasound. Fetal right lung area was assessed in mm by the tracing method using the ultrasound machine calipers. The traced area was also calculated offline using commercially available digital tracing software (Screen Tracing Paper, Iconico.com). The Quantitative Lung Index (QLI) for the right lung (QLI-R) was calculated using the traced area of the right lung at the level of the 4-chamber view and the head circumference, as previously reported [Citation23]. The lung-to-head ratio (LHR) and the observed vs. expected lung-to-head ratio (o/e LHR) were calculated using both the longest diameter methods (LDM) and traced area methods (TAM). Antenatal intervention in selected cases of CDH-L (QLI <0.6) was offered using a modified Z-stent [Citation24]. Neonatal management was conducted according to internationally accepted protocols [Citation25], which included intubation, stabilization, conventional mechanical ventilation or high-frequency oscillatory ventilation, nitric oxide administration and control of pulmonary hypertension as needed. Postnatal surgical correction of the diaphragmatic defect was performed when suitable. ECMO was offered where available. Neonatal outcome was categorized as alive to discharge.
Statistical analysis was performed using IBM SPSS Statistics for Windows, version 28 (IBM Corp., Armonk, N.Y., USA). Continuous variables were assessed for normality, using the Kolmogorov-Smirnov test. Binary multiple logistic regression was used to determine the significant variables associated with neonatal outcome. A ROC curve was used to determine the optimal QLI cutoff point predictive of survival. Continuous and discrete variables were compared using parametric or non-parametric tests, or Chi-square with Fisher’s correction as needed. A p-value <.05 was considered statistically significant. The study was approved by the Western Institutional Review Board, and was considered exempted, as it qualified as posing no risk or minimal risk to the subjects.
Results
shows the patient cohort flow. A total of 118 patients at risk for pulmonary hypoplasia were identified. Seventeen patients were excluded: four had a right-sided CDH, one had diaphragmatic agenesis and bilateral CDH, three patients for genetic reasons (one with tetrasomy 12p, one with partial Turner syndrome, one with twins and trisomy 18), one with congenital heart disease, one with multiple anomalies, three patients elected pregnancy termination, and four had missing ultrasound data.
Of the remaining patients (101), 11 with conditions other than CDH-L but at risk for pulmonary hypoplasia, were used to determine the intraclass correlation coefficient (ICC) of the QLI-R: 7 had pleural effusions, 2 had congenital pulmonary airway malformation (CPAM), and 2 had oligohydramnios or anhydramnios from premature rupture of membranes or fetal urinary tract obstruction. The ICC was assessed by measuring the head circumference once and by tracing the area of the right lung in three separate measurements in three separate images at the level of the 4-chamber view during the same visit by the same operator, blinded to the results of the measurements. Analysis of the ICC of the QLI in the 11 patients with non-CDH conditions showed an overall intra-rater reliability of 0.985 (Cronbach’s Alpha-based).
Of the 90 patients with left-sided CHD, 78 had no fetal intervention and 12 had tracheal occlusion with a modified Z-stent. Neonatal outcome was unknown in one of the 78 CDH-L patients, leaving 77 expectantly managed patients for analysis.
Comparison of ultrasound methodology
shows the results of measuring the LHR using the longest diameter method (LDM) vs. the tracing method (TAM) in the 77 patients with CDH-L managed expectantly. The LHR was significantly larger (41.6%) if obtained by the LDM method compared to the TAM, whereas the o/e LHR did not differ by measurement method.
Table 1. LHR and o/e LHR by longest diameter or tracing methods.
Since the QLI-R only uses the tracing method, it was only derived that way.
Gestational age and QLI-R
To assess the relationship between the QLI-R and gestational age, 25 patients with CDH-L who did not undergo antenatal treatment and in whom serial QLI measurements were done at least twice at different gestational ages, were selected. The mean gestational age at the first ultrasound measurement was 29 ± 4.7 weeks, with a mean QLI-R of 0.6 ± 0.26, and the mean gestational age at the second measurement was 33.5 ± 4.2 weeks, with a mean QLI-R of 0.61 ± 0.2. The mean gestational age for both measurements was 32 ± 3.69 weeks, with a mean QLI of 0.59 ± 0.18. There was no correlation between gestational age and QLI-R (-0.73, Pearson correlation, p = .72).
QLI-R cutoff in predicting neonatal death
To establish the cutoff of the QLI in predicting pulmonary hypoplasia and neonatal demise, the analysis was limited to the 77 CDH-L patients without antenatal treatment. shows the demographics of this population.
Table 2. Demographics of patients with CDH-L and no antenatal intervention N = 77.
The median QLI associated with neonatal demise was 0.38 vs. 0.51 for survivors (p = .004, Mann-Whitney U test). A ROC curve analysis was performed in the 77 patients with CDH-L who did not undergo treatment to determine the optimal cutoff of the QLI-R predictive of neonatal demise (). Twenty-six patients (33%) had a neonatal demise. Using the first QLI-R obtained at the time of diagnosis, the QLI-R cutoff predictive of neonatal demise was 0.45 (area under the curve 0.7, p = .001). In patients with serial QLI-R measurements (N = 25), if the last QLI was used, a QLI-R equal to or less than 0.45 was also significantly predictive of neonatal demise (area under the curve 0.64, p = .046).
A ROC was constructed to assess the cutoff point of the LHR using the longest diameter method (LHR-LDM). The area under the curve was 0.725, p = .001. An LHR of 1.8 was the optimal cutoff point, with a sensitivity of 77% ().
Similarly, a ROC curve was constructed to assess the cutoff point of the LHR using the tracing method (LHR-TAM). The area under the curve was 0.71, p = .03. An LHR-TAM of 1.2 was the cutoff point, with a sensitivity of 77% ().
The optimal cutoff point for the o/e LHR was also assessed by constructing a ROC curve. Since the longest diameter or the tracing method were no different, only a single ROC curve was needed, and we chose to show the one generated with the tracing method. The area under the curve was 0.71, p = .002. An o/e LHR of 45% was the optimal cutoff point, with a sensitivity of 80% ().
Binary multiple logistic regression was performed to assess which antenatal factors were predictive of neonatal demise. The variables assessed included liver-up, QLI-R, LHR-TAM and o/e LHR, and included possible interaction between the variables. A value for the LHR of 1 or less and a value for the o/e LHR of 25% or less was used in the calculation, as used in the literature [Citation27,Citation28]. Analysis showed that a value of the QLI-R < 0.45 and liver-up were both predictive of neonatal demise (p = .006 and 0.012, respectively, odds ratios and (95% CI) 5.4 (1.6–18) and 4.2 (1.3–13.1), respectively), whereas an LHR <1 was not. None of the patients had an o/e LHR less than 25% and therefore this parameter could not be assessed. Because an interaction between liver-up and QLI-R was noted, each risk factor was assessed separately, controlling for the other. In patients with liver-down, a QLI <0.45 was predictive of neonatal demise (p = .045, odds ratio 4.9, 95% CI 1.04–23.2). For patients with liver up, there was a tendency for a QLI-R < 0.45 to predict neonatal demise (p = .058, odds ratio 6.2, 95% CI 0.94–41.5).
Only 3 patients underwent ECMO, one managed expectantly and the other two with the tracheal stent. All three of these babies died.
QLI-R and tracheal occlusion
Effect of treatment on the QLI-R was assessed in 12 patients with CDH-L who underwent tracheal occlusion using a modified Z-stent. The modified Z-stent occludes the trachea for up to 4 weeks, after which time the occluding material dissolves spontaneously. Nine of the 12 patients (75%) had neonatal survival. The mean gestational age at diagnosis was 24.6 weeks (SD = 3.8), with a mean QLI-R of 0.48 =/- 0.15. The mean gestational age at the time of surgery was 31.2 ± 1.6 weeks. The mean gestational age at delivery was 35.2 weeks (35.8 vs. 34.9, neonatal demise vs. alive, respectively, p > .05). There was a statistically significant increase in the QLI-R after surgery to 0.52 (p = .01, paired samples t-test). However, the maximum QLI-R was not predictive of survival (p = .4, Student’s t-test). In ten of these 12 patients, serial QLI-R measurements were obtained after surgery. Because the tracheal occluding device (modified Z-stent) ceases to occlude the trachea after 3-4 weeks spontaneously, the QLI-R is expected to return to baseline value after 4 weeks. Fetal lung growth was assessed by subtracting the mean pre-operative QLI-R from the mean post-operative QLI-R 1-3 weeks after surgery. An increase in the QLI-R by 0.11 points 1–3 weeks after surgery was associated with a tendency for neonatal survival (p = .056, two-sided Student’s t-test) ().
Discussion
Our data suggest that a value of the QLI-R of 0.45 or less in patients with CDH-L managed expectantly can differentiate fetuses likely to survive the neonatal period. Our analysis confirms the gestational-age independence of the QLI, with no correlation between QLI-R and gestational age. As a corollary, any increase in the QLI after antenatal treatment, such as tracheal occlusion, reflects actual lung growth. This also allows the use of the QLI-R to monitor the effects of antenatal therapy.
The QLI-R was derived mathematically from the formulas for the head circumference and the right lung area [Citation23]. Essentially, dividing the right lung area by the square of the head circumference is associated with a relatively constant relationship throughout gestation (QLI-R), which is virtually independent of gestational age [Citation21]. We also showed that dividing polynomials of different order, such as it is done for the calculation of the LHR (lung area in mm2 and head circumference in mm), is associated with a value that increases with advancing gestational age [Citation21]. The increase in the value of the LHR with gestational age not only negates its potential use to categorize pregnancies at risk for poor outcome when using a fixed cutoff point (e.g. LHR <1.4 [Citation29] or LHR <0.9 [Citation30], but also as a monitoring tool to assess fetal lung growth after tracheal occlusion or any other form of fetal therapy. We have also previously shown that the o/e LHR is not gestational-age independent [Citation22], which does not allow the use of a fixed value as predictor of neonatal outcome. Despite the demonstrated mathematical shortcomings of the LHR and the o/e LHR, these indices continue to be used clinically to categorize fetuses at risk for pulmonary hypoplasia [Citation27,Citation28, Citation31–33].
Our previous study estimated mathematically that a small right lung would have a QLI-R of <.6, which corresponds to the .1st percentile [Citation21]. For the left lung a similar analysis suggested a value for the QLI-L of <.5, which is at the .1st percentile between 22–32 weeks. Our current study suggests that a value of the QLI-R of 0.45 or less could be used to predict pulmonary hypoplasia and neonatal demise in fetuses with CDH-L, which is close to our previous mathematical estimates. Different than the LHR or the o/e LHR, the gestational-age independence of the QLI-R confirmed in the current study, allows this sonographic index to be used at any point during pregnancy, particularly during the second trimester, when counseling of the patients can be crucial. The correct identification of patients at risk for pulmonary hypoplasia may allow for better patient selection in determining whether antenatal therapy is warranted. The advantage of the QLI over the use of percentiles is that percentiles are gestational age dependent and do not account for differences in fetal growth. Therefore, a small-for-gestational age fetus may have a small lung by percentile, yet an adequate lung by QLI-R. Similarly, a large-for-gestational age fetus may have an adequate lung by percentile, but a small lung by QLI-R.
Since the LHR increases with gestational age, monitoring the effect of antenatal treatment is not possible with the LHR or the o/e LHR, despite other reports [Citation34]. Indeed, a higher value of the LHR at a later post-operative date can be the result of therapy or from the natural increase of the LHR with gestational age. This also applies to the o/e LHR, as the o/e LHR is simply a percent of the LHR. In contrast, since the QLI-R does not increase with advancing gestational age and given the reliability of the measurement by the high intraclass correlation coefficient found in the current study, any increase in the value of the QLI, particularly above the 0.45 cutoff, indicates lung growth. In this regard, although our study did not find a correlation between the highest value of the QLI-R and neonatal survival in patients in whom antenatal treatment was performed, but it did reflect the expected rise in fetal lung growth after tracheal occlusion and a tendency to predict neonatal survival based on the 1–3 week delta compared to the pre-op QLI-R.
Although not the focus of the current article, we assessed the performance of the LHR (longest diameter or tracing method) and the o/e LHR in our population, as these indices continue to be used by other investigators. Our analysis shows that, although both the LHR using either methodology (longest diameter or tracing method) or the o/e LHR can predict neonatal demise, the cutoff values generated in our population are very different and much higher than those commonly used to recommend antenatal treatment. This is a direct reflection of the dependance of both indices on gestational age. In our cohort, an LHR of 1.8 (longest diameter) or 1.2 (tracing method) or an o/e LHR of 45% would have to had to be used to classify patients at risk for neonatal demise. The current definition of high-risk CDH patients requires an LHR of 1.0 or less or an o/e LHR of 25% or less. In our cohort, an LHR of 1.0 or less would have had a sensitivity of only 50% and an o/e LHR of 25% or less would have only had a sensitivity of 11%. Instead, a QLI of <0.45 had a sensitivity of 77% in predicting neonatal demise.
Our study has significant limitations. The retrospective nature of the study did not allow the use of a standardized protocol to assess the QLI serially in all patients and at specific gestational age intervals. The gestational age at diagnosis, approximately 29 weeks, albeit still in time to offer antenatal intervention, is higher than in other cohorts, where the diagnosis is typically made before 26 weeks. However, since the QLI is independent of gestational age, it could still be used to categorize patients at high risk for neonatal demise at this gestational agea. As can be seen in , at this gestational age, the median LHR of 1.4 (longest diameter method) or 1.01 (tracing method), would not have correctly identified these patients as candidates for antenatal treatment who in fact had a neonatal demise. Similarly, a median o/e LHR of 35–37% (longest diameter or tracing method) would not have qualified these patients for tracheal occlusion and yet they were associated with neonatal demise. Because the Z-stent has been discontinued, we could not have a larger number of treated patients. However, we were still able to show a 75% survival and the value of the QLI-R to monitor fetal lung growth after treatment. Strengths of our study include a significant number of CDH-L patients managed expectantly, which allows knowledge of the natural history and predictive value of the sonographic indices. In addition, the results of the QLI were not used in patient management in patients managed expectantly, which also allows for a better understanding of the natural history of the QLI-R in predicting pulmonary hypoplasia and neonatal death.
In summary, our data suggest that the measurement of the QLI-R is reliable and that a QLI-R of 0.45 or less may be useful in predicting neonatal demise from pulmonary hypoplasia in patients with CDH-L. The gestational age-independence of the QLI-R is clinically confirmed. The independence of the QLI-R with gestational age also allows the use of this sonographic index in evaluating lung growth after antenatal intervention. Further prospective studies are needed to refine the cutoff of the QLI-R in this patient population as well as in other fetal conditions associated with risk of pulmonary hypoplasia and consequent neonatal demise. Similarly, studies to determine the clinical cutoff point of the QLI-L in patients at risk for pulmonary hypoplasia and neonatal demise from conditions affecting the right lung are warranted.
Author contribution
All authors have participated in the writing and editing of this manuscript.
Disclosure statement
The authors report there are no competing interests to declare.
Data availability
The data that support the findings of this study are available from the corresponding author, RQ, upon reasonable request.
Additional information
Funding
References
- Winn HN, Chen M, Amon E, et al. Neonatal pulmonary hypoplasia and perinatal mortality in patients with midtrimester rupture of amniotic membranes–a critical analysis. Am J Obstet Gynecol. 2000;182(6):1638–1644. doi: 10.1067/mob.2000.107435.
- Cotten CM. Pulmonary hypoplasia. Semin Fetal Neonatal Med. 2017;22(4):250–255. doi: 10.1016/j.siny.2017.06.004.
- Kluth D, Tenbrinck R, von Ekesparre M, et al. The natural history of congenital diaphragmatic hernia and pulmonary hypoplasia in the embryo. J Pediatr Surg. 1993;28(3):456–463. doi: 10.1016/0022-3468(93)90248-j.
- Delacourt C, Bertille N, Salomon LJ, et al. Prenatal natural history of congenital pulmonary malformations: MALFPULM population-based cohort study. Ultrasound Obstet Gynecol. 2019;54(3):381–388. doi: 10.1002/uog.20130.
- Lee FL, Said N, Grikscheit TC, et al. Treatment of congenital pulmonary airway malformation induced hydrops fetalis via percutaneous sclerotherapy. Fetal Diagn Ther. 2012;31(4):264–268. doi: 10.1159/000336226.
- Hellmund A, Berg C, Geipel A, et al. Prenatal diagnosis and evaluation of sonographic predictors for intervention and adverse outcome in congenital pulmonary airway malformation. PLoS One. 2016;11(3):e0150474. doi: 10.1371/journal.pone.0150474.
- Peters NCJ, Hijkoop A, Hermelijn SM, et al. Prediction of postnatal outcome in fetuses with congenital lung malformation: 2-year follow-up study. Ultrasound Obstet Gynecol. 2021;58(3):428–438. doi: 10.1002/uog.23542.
- Castillo RA, Devoe LD, Falls G, et al. Pleural effusions and pulmonary hypoplasia. Am J Obstet Gynecol. 1987;157(5):1252–1255. doi: 10.1016/s0002-9378(87)80304-6.
- Grandt J, Gottschalk I, Geipel A, et al. Intrauterine thoracoamniotic shunting of fetal hydrothorax with the somatex intrauterine shunt: intrauterine course and postnatal outcome. J Clin Med. 2022;11(9):2312.
- Bienstock JL, Birsner ML, Coleman F, et al. Successful in utero intervention for bilateral renal agenesis. Obstet Gynecol. 2014;124(2 Pt 2 Suppl 1):413–415. doi: 10.1097/AOG.0000000000000339.
- Cheung KW, Morris RK, Kilby MD. Congenital urinary tract obstruction. Best Pract Res Clin Obstet Gynaecol. 2019;58:78–92. doi: 10.1016/j.bpobgyn.2019.01.003.
- Yang LC, Taylor DR, Kaufman HH, et al. Maternal and fetal outcomes of spontaneous preterm premature rupture of membranes. J Am Osteopath Assoc. 2004;104(12):537–542.
- Kalache KD, Espinoza J, Chaiworapongsa T, et al. Three-dimensional ultrasound fetal lung volume measurement: a systematic study comparing the multiplanar method with the rotational (VOCAL) technique. Ultrasound Obstet Gynecol. 2003;21(2):111–118. doi: 10.1002/uog.39.
- Coleman A, Phithakwatchara N, Shaaban A, et al. Fetal lung growth represented by longitudinal changes in MRI-derived fetal lung volume parameters predicts survival in isolated left-sided congenital diaphragmatic hernia. Prenat Diagn. 2015;35(2):160–166. doi: 10.1002/pd.4510.
- Tsuda H, Kotani T, Miura M, et al. Observed-to-expected MRI fetal lung volume can predict long-term lung morbidity in infants with congenital diaphragmatic hernia. J Matern Fetal Neonatal Med. 2017;30(13):1509–1513. doi: 10.1080/14767058.2017.1299126.
- Jani JC, Cannie M, Peralta CF, et al. Lung volumes in fetuses with congenital diaphragmatic hernia: comparison of 3D US and MR imaging assessments. Radiology. 2007;244(2):575–582. doi: 10.1148/radiol.2442061158.
- Songster GS, Gray DL, Crane JP. Prenatal prediction of lethal pulmonary hypoplasia using ultrasonic fetal chest circumference. Obstet Gynecol. 1989;73(2):261–266.
- Ba’ath ME, Jesudason EC, Losty PD. How useful is the lung-to-head ratio in predicting outcome in the fetus with congenital diaphragmatic hernia? A systematic review and meta-analysis. Ultrasound Obstet Gynecol. 2007;30(6):897–906. doi: 10.1002/uog.5164.
- Peralta CF, Cavoretto P, Csapo B, et al. Assessment of lung area in normal fetuses at 12-32 weeks. Ultrasound Obstet Gynecol. 2005;26(7):718–724. doi: 10.1002/uog.2651.
- Jani JC, Peralta CF, Nicolaides KH. Lung-to-head ratio: a need to unify the technique. Ultrasound Obstet Gynecol. 2012;39(1):2–6. doi: 10.1002/uog.11065.
- Jani J, Peralta CF, Benachi A, et al. Assessment of lung area in fetuses with congenital diaphragmatic hernia. Ultrasound Obstet Gynecol. 2007;30(1):72–76. doi: 10.1002/uog.4051.
- Quintero RA, Kontopoulos EV, Quintero LF, et al. The observed vs. expected lung-to-head ratio does not correct for the effect of gestational age on the lung-to-head ratio. J Matern Fetal Neonatal Med. 2013;26(6):552–557. doi: 10.3109/14767058.2012.736000.
- Quintero RA, Quintero LF, Chmait R, et al. The quantitative lung index (QLI): a gestational age-independent sonographic predictor of fetal lung growth. Am J Obstet Gynecol. 2011;205(6):544 e1-8. doi: 10.1016/j.ajog.2011.07.031.
- Quintero R, Morales W, Bornick P, et al. Minimally-invasive intraluminal tracheal occlusion in a human fetus with left congenital diaphragmatic hernia at 27 weeks’ gestation via direct fetal laryngoscopy. Prenat Neonat Med. 2000;5:134–140.
- Snoek KG, Reiss IK, Greenough A, et al. Standardized postnatal management of infants with congenital diaphragmatic hernia in Europe: the CDH EURO consortium consensus - 2015 update. Neonatology. 2016;110(1):66–74. doi: 10.1159/000444210.
- Kontopoulos EV, Quintero LF, Chmait R, et al. The quantitative lung index: the left lung. J Matern Fetal Neonatal Med. 2022;35(21):4142–4148. doi: 10.1080/14767058.2020.1847076.
- Deprest JA, Nicolaides KH, Benachi A, et al. Randomized trial of fetal surgery for severe left diaphragmatic hernia. N Engl J Med. 2021;385(2):107–118. doi: 10.1056/NEJMoa2027030.
- Deprest JA, Benachi A, Gratacos E, et al. Randomized trial of fetal surgery for moderate left diaphragmatic hernia. N Engl J Med. 2021;385(2):119–129. doi: 10.1056/NEJMoa2026983.
- Harrison MR, Keller RL, Hawgood SB, et al. A randomized trial of fetal endoscopic tracheal occlusion for severe fetal congenital diaphragmatic hernia. N Engl J Med. 2003;349(20):1916–1924. doi: 10.1056/NEJMoa035005.
- Yang SH, Nobuhara KK, Keller RL, et al. Reliability of the lung-to-head ratio as a predictor of outcome in fetuses with isolated left congenital diaphragmatic hernia at gestation outside 24-26 weeks. Am J Obstet Gynecol. 2007;197(1):30 e1-7. doi: 10.1016/j.ajog.2007.01.016.
- Abbasi N, Ryan G, Johnson A, et al. Reproducibility of fetal lung-to-head ratio in left diaphragmatic hernia across the North American fetal therapy network (NAFTNet). Prenat Diagn. 2019;39(3):188–194. doi: 10.1002/pd.5413.
- Senat MV, Bouchghoul H, Stirnemann J, et al. Prognosis of isolated congenital diaphragmatic hernia using lung-area-to-head-circumference ratio: variability across centers in a national perinatal network. Ultrasound Obstet Gynecol. 2018;51(2):208–213. doi: 10.1002/uog.17463.
- Novoa YNVA, Sutton LF, Neis AE, et al. Reproducibility of lung-to-Head ratio ultrasound measurements in congenital diaphragmatic hernia. J Ultrasound Med. 2018;37(8):2037–2041. doi: 10.1002/jum.14557.
- Partridge EA, Peranteau WH, Herkert L, et al. Rate of increase of lung-to-head ratio over the course of gestation is predictive of survival in left-sided congenital diaphragmatic hernia. J Pediatr Surg. 2016;51(5):703–705. doi: 10.1016/j.jpedsurg.2016.02.006.