Abstract
Objective
In recent years, several studies have reported an association between unsaturated fatty acids (UFAs) and the risk of developing preeclampsia; however, its exact causal effect is unclear. This study assessed the causal association between circulating UFAs and preeclampsia.
Methods
A two-sample Mendelian randomization (MR) study using publicly available genome-wide association study (GWAS) summary data for circulating UFA s (N = 114,999) and preeclampsia (N = 118,291) was performed. Single nucleotide polymorphisms (SNPs) significantly associated with exposure was selected as instrumental variables (IVs). The inverse variance weighted (IVW) test was used as the primary method for estimating causality in MR analysis, while MR pleiotropy residual sum and outlier (MR-PRESSO) and MR-Egger regression methods were used to assess horizontal pleiotropy. Cochran’s Q test was used to evaluate heterogeneity among SNPs, and leave-one-out sensitivity analysis was used to determine the effect of individual SNPs on the results of the MR analysis. Bonferroni correction was used as a correction for multiple corrections.
Results
Two-sample MR analysis suggested that the ratio of monounsaturated fatty acids (MUFAs) to total fatty acids (OR 1.150, 95% CI 1.006–1.315, p = 0.041), the ratio of polyunsaturated fatty acids (PUFAs) to total fatty acids (OR 0.805, 95% CI 0.658–0.986, p = 0.036) and the ratio of PUFAs to MUFAs (OR 0.807, 95% CI 0.694–0.938, p = 0.005) were causally associated with preeclampsia. After Bonferroni correction, the causal association between the ratio of polyunsaturated to MUFAs and preeclampsia remained statistically different.
Conclusions
This MR analysis provides evidence for a genetic causal association between circulating UFAs and preeclampsia.
Introduction
Preeclampsia (PE) is a condition specific to pregnancy and a severe manifestation of hypertensive disorders of pregnancy. It is defined as new-onset hypertension after 20 weeks of gestation with features such as urinary protein, acute kidney injury, and liver dysfunction [Citation1,Citation2]. PE affects 3–5% of pregnancies worldwide and is a significant contributor to global maternal morbidity (including serious outcomes such as liver rupture, kidney failure, seizures (eclampsia and stroke), and mortality) [Citation3]. In addition to the maternal disease burden, fetal morbidity and mortality can result from medically induced preterm birth, fetal growth restriction, and placental abruption [Citation4]. Maternal deaths due to hypertension were 26% in Latin America and the Caribbean and 9% in Africa and Asia in 2020 [Citation5].
Recent studies have shown that pregnant women with PE have higher circulating levels of palmitoleic acid and that subjects with circulating levels of total omega-3 within the reference range have an 8.5-fold higher risk of developing PE than those above the reference range [Citation6,Citation7]. Observational studies have suggested that PE is associated with low intake of omega-3 long-chain polyunsaturated fatty acids (PUFAs) [Citation8]. In addition, several studies have examined fatty acid supplementation for the prevention of PE, and a meta-analysis of 14 studies showed a protective effect of omega-3 fatty acid supplementation on the risk of PE (RR 0.82; 95% CI 0.70–0.97, p = 0. 024, I2 = 19.0%)[Citation9]. A prospective study showed that dietary intake of long-chain PUFAs (EPA, DHA, and AA) might protect pregnant women from developing PE [Citation10].
In recent years, Mendelian randomization (MR) analysis has been widely used to assess the causal impact of clinical factors on a disease [Citation11]. MR explores the causal association between risk factors and disease using instrumental variables (IVs)[Citation12]. Due to the stochastic nature of the inheritance of genetic variation, MR analysis can provide more reliable results by reducing the likelihood of being influenced by potential confounders, reversing causal bias, and genetically inferring correlations between exposure and outcome [Citation13,Citation14]. Studies have shown that single nucleotide polymorphisms (SNPs) in genes fat mass and obesity-associated [Citation15], insulin-like growth factor binding protein 1 [Citation16], and angiotensinogen [Citation17] appear to be associated with the onset of PE. However, the causal association between unsaturated fatty acids (UFAs) and PE is unclear. Therefore, we performed a two-sample MR analysis to investigate the causal effect of the UFA on PE.
Methods
Study design
In this study, we performed a two-sample MR analysis to assess the causal association between UFA and PE. SNPs were used as IVs [Citation18]. We explored the causal association between circulating monounsaturated fatty acids (MUFAs), the ratio of MUFAs to total fatty acids (rMUFA), PUFAs, the ratio of PUFAs to total fatty acids (rPUFA). and the ratio of PUFAs to MUFAs (rP/MUFA) and PE respectively. Three important assumptions must be confirmed throughout the process to maximize the results’ accuracy [Citation12]. First, the selected IV should be directly related to UFAs. Second, the IV should not be influenced by potential confounding factors affecting exposure and outcome. Third, the IV affects PE only through UFAs. A directed acyclic graph assessed the causal association between UFA and PE (). All data for this study were obtained from publicly available databases, and all original studies were approved by ethical review and informed consent.
Figure 1. A directed acyclic graph for Mendelian randomization analysis. Single nucleotide polymorphisms associated with unsaturated fatty acids were screened as IVs. SNPs associated with body mass index, type 2 diabetes, hypertension, and systolic blood pressure were removed to reduce the bias caused by confounders.
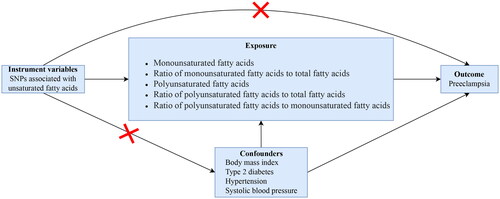
Data sources
The data on UFA and PE (ID: finn-b-O15_PREECLAMPS) used in our study were obtained from the genome-wide association study (GWAS) summary data (https://gwas.mrcieu.ac.uk/). The UFA in this study included MUFA (GWAS ID: met-d-MUFA), rMUFA (GWAS ID: met-d-MUFA_pct), PUFA (GWAS ID: met-d-PUFA), rPUFA (GWAS ID: met-d-PUFA_pct), and rP/MUFA (GWAS ID: met-d-PUFA_by_MUFA). A total of 114,999 participants of European ancestry were included in the data related to UFA, and 118,291 participants of European ancestry were included in the data for PE, which contained 3556 patients with PE and 114,735 controls.
IVs selection
The selection threshold for SNP was p < 5e-8. We tested the selected SNPs for linkage disequilibrium (LD) to ensure the validity of the data, and a clumping procedure was performed to filter independent SNPs within a window size of 1,0000 kb and a threshold of r2 < 0. 01. In addition, PhenoScanner (http://www.phenoscanner.medschl.cam.ac.uk/) [Citation19] was used to search for genome-wide features significantly associated (p < 5e-8) with these SNPs to remove which SNPs were associated with confounders of UFA and PE. PhenoScanner is a curated database of publicly available results from large-scale human genetic association studies.
Next, we assessed the strength of each IV and excluded weakly correlated IVs by calculating the F statistic, with R2 denoting the exposure variance explained by each IV, N denoting the GWAS sample size of exposure factors (N = 114,999). F > 10 indicates that the IVs were sufficiently strong, which means that the IVs had a sufficient estimation effect for subsequent MR analysis without weak instrumental bias [Citation20]. The F statistic was calculated as F = R2(N-2)/(1-R2)[Citation21].
Statistical analyses
The inverse variance weighted (IVW) method was mainly used in the current study to infer a potential causal association between UFAs and PE. In addition, three validated methods, MR-Egger, weighted median, and weighted mode, were applied to evaluate the possible association comprehensively. Bonferroni correction of the evidential threshold was based on the number of exposures (p value < 0.05/5 exposures). The association with a two-sided p value < 0.01 (0.05/5 exposures) was deemed statistically significant, and a two-sided p value < 0.05 was thought suggestive [Citation22]. The Cochrane’s Q statistic calculated the heterogeneity of SNPs. In addition, leave-one-out analysis was used to identify individual SNPs that strongly influenced the outcome. Directional pleiotropy was assessed by estimating the deviation of the MR-Egger intercept [Citation23], with p < 0.05 indicating mean directional pleiotropy [Citation24]. The MR-PRESSO method was applied to check the pleiotropy outliers SNP [Citation25]. The MR-Egger intercept and MR pleiotropy residuals and MR-PRESSO methods were used to test that all IVs met the third assumption.
All statistical analyses were performed using R software (R Foundation for Statistical Computing, Vienna, Austria, version 4.2.2), TwoSampleMR, and MR-PRESSO packages.
Results
MR analysis
The screening process for IVs is shown in Supplementary Table 1. The F statistic of each SNP was >10, and no SNP was excluded. Results of IVW analysis showed that there was no causal association between MUFA (OR 1.086, 95% CI 0.935–1.262, p = 0.279) and PUFA (OR 1.039, 95% CI 0.906–1.191, p = 0.585) and PE (). There was a positive causal association between rMUFA and PE (OR 1.115, 95% CI 1.006–1.315, p = 0.041). In addition, there was a negative causal association between rPUFA (OR 0.805, 95% CI 0.658–0.986, p = 0.036) and rP/MUFA (OR 0.807, 95% CI 0.694–0.938, p = 0.005) and PE. After Bonferroni correction, the causal association between rP/MUFA and PE remained statistically significant, whereas rMUFA and rPUFA were suggestively statistically significant.
Figure 2. Results of MR analyses conducted to estimate potential associations between circulating unsaturated fatty acids and preeclampsia.
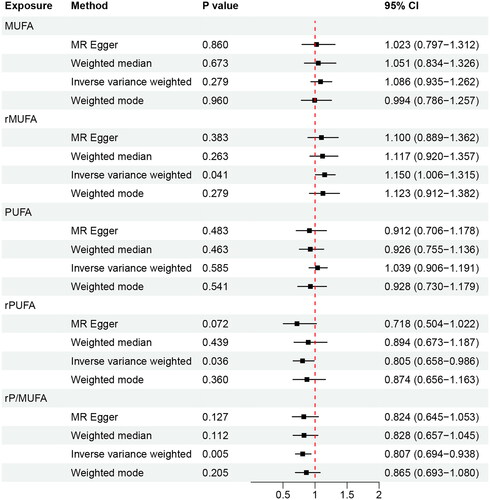
The MR-Egger, weighted median, and weighted mode methods showed that the MR analysis results for MUFA and PUFA were unstable. The MR analyses of rMUFA, rPUFA, and rP/MUFA were stable.
Sensitivity analysis
Cochrane’s Q statistic showed no heterogeneity in IVW or MR Egger methods for the five exposure factors (all p > 0.05, ). Leave-one-out analysis () results suggest that a single SNP strongly influences the MR analysis results of rMUFA and rPUFA, and the MR results for rP/MUFA were not affected by a single SNP.
Figure 3. Leave-one-out sensitivity analysis of the causal effect of circulating UFA on PE. (A) MUFA; (B) rMUFA; (C) PUFA; (D) rPUFA; (E) rP/MUFA.
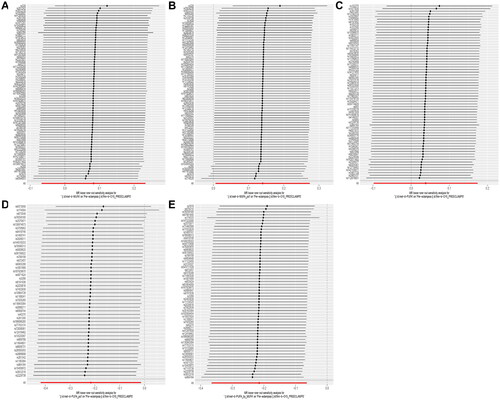
Table 1. Heterogeneity testing using the Cochrane Q statistic.
MR-Egger intercept analysis (, ) and MR-PRESSO () showed no horizontal pleiotropy in any IVs used in the MR analyses for the five exposure factors.
Figure 4. MR-Egger intercept analysis to analyze horizontal pleiotropy of SNPs. (A) MUFA; (B) rMUFA; (C) PUFA; (D) rPUFA; (E) rP/MUFA.
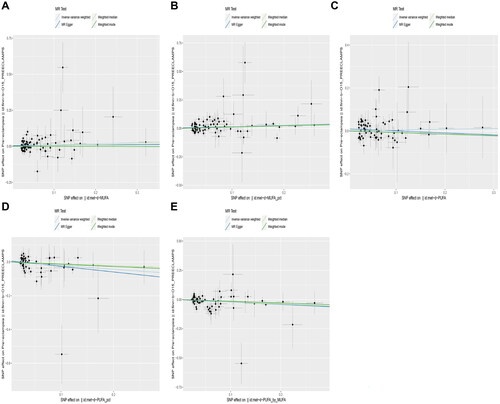
Table 2. MR-Egger intercept analysis to analyze horizontal pleiotropy of SNPs.
Table 3. Pleiotropy testing using MR-PRESSO.
Discussion
By analyzing the summary data from GWAS, our study shows no evidence of a causal association between circulating MUFA, PUFA, and PE. However, there was a significant causal association between rMUFA, rPUFA and rP/MUFA, and PE. Moreover, the causal association between rP/MUFA and PE was statistically significant even after Bonferroni correction.
Studies have shown PE patients have higher circulating total UFA and MUFA [Citation26,Citation27]. Among them, the circulating palmitoleic acid content is higher [Citation26], and palmitate increases syncytial trophoblast cell death, especially through caspase-mediated apoptosis [Citation28]. However, our MR analysis of the causal association between MUFA and PE does not support these characteristics.
PUFA mainly includes omega-3 and omega-6 fatty acids. Omega-3 fatty acids can prevent inflammation, while omega-6 fatty acids are involved in inflammatory response. Analysis of UFAs in the placenta of PE found that the concentration of omega-3 and omega-6 fatty acids decreased [Citation29]. Circulating total omega-3 fatty acids were associated with a reduced risk of PE [Citation30], with each 1% increase associated with a 24% reduction in the chance of pregnancy-related hypertension (OR 0.76; 95% CI 0.60–0.97)[Citation31]. PE is associated with altered maternal omega-3 fatty acids status, altered placental omega-3 fatty acids metabolism, reduced cord blood omega-3 fatty acids levels, and impacts on infant neurodevelopment and brain health later in life [Citation30,Citation32]. In a meta-analysis that included 24 studies, circulating omega-3 fatty acids was protective against the risk of PE (RR = 0.84, 95% CI 0.74–0.96, p = 0.008; I2 = 24%) [Citation33]. In addition, the concentration of omega-3 fatty acids in red blood cells decreased in women with PE [Citation34]. For total omega-6 fatty acids, the odds ratio was 0.46 (95% CI 0.23–0.92), although there were indications of a slightly increased risk of PE associated with high levels of arachidonic acid [Citation35]. PE can be avoided by consuming long-chain PUFA during pregnancy; however, there is no significant correlation between MUFA intake and PE [Citation36].
PUFA play a vital role in placental angiogenesis and are significantly negatively correlated with soluble tyrosine kinase receptor 1 in PE circulation [Citation37]. Mathew et al. improved the angiogenesis in placental mesenchymal stem cells using omega-3 fatty acids [Citation38]. In addition, omega-3 fatty acids can reduce the severity of late-onset PE by increasing the levels of transcription factors [Citation39]. In addition, arachidonic acid and its metabolites are closely related to the inflammatory response in PE, and a moderate inflammatory response can improve maternal-fetal tolerance [Citation40]. PUFA diet can reduce the incidence of PE by upregulating the expression of lipoxygenase 12 and lipoxygenase 15 and reducing the expression of cyclooxygenase-2, tumor necrosis factor, and endoglin [Citation41].
Based on the results of this study, the role of UFA in the pathogenesis of PE may not act through changes in its concentration but rather result from changing the relationship between the ratio of MUFA and PUFA.
Limitations
The limitations of our study should be noted as well. First, in the MR analysis, the results of MR Egger, weighted median, and weighted mode are not entirely consistent with the results of IVW, which may be related to SNP pleiotropy and other reasons. Second, the included population only included European ancestry. Although the consistency of the participating population can reduce the bias of results caused by different populations, whether this result applies to the whole population remains to be further proved. Finally, since MUFA and PUFA are not a single UFA but a mixture of UFA, it has not been possible to determine which UFA produced the main effect in the results of this study.
Conclusion
In conclusion, we assessed for the first time the causal association between circulating UFA and PE using MR analysis. We found that circulating levels of MUFA and PUFA were not causally related to PE, but rMUPA, rPUFA, and rP/MUFA were significantly causally associated with PE. After using Bonferroni correction, the causal association between rP/MUFA and PE remained statistically significant, whereas it was suggestive significant between rMUPA, rPUFA, and PE.
Authors’ contributions
W.Y. was responsible for study design, data extraction and analysis, and article writing. L.S. and W.C. were responsible for the study design and direction. Y.H. and J.X. were responsible for study design, revision, paper review, guidance, and funding.
Ethics approval and consent to participate
Our study did not collect original data but used publicly available GWAS summary data; therefore, ethics committee approval was not required.
Consent for publication
Not applicable.
Supplemental Material
Download MS Excel (228.5 KB)Acknowledgments
We thank Professor Ding Hongjuan for her support in this research.
Disclosure statement
The authors declare that they have no competing interests.
Availability of data and materials
The datasets used and/or analyzed during this study are available from the corresponding author upon reasonable request.
Additional information
Funding
References
- Brown MA, Magee LA, Kenny LC, et al. Hypertensive disorders of pregnancy: ISSHP classification, diagnosis, and management recommendations for international practice. Hypertension. 2018;72(1):24–43. doi:10.1161/HYPERTENSIONAHA.117.10803.
- Webster K, Fishburn S, Maresh M, et al. Diagnosis and management of hypertension in pregnancy: summary of updated NICE guidance. BMJ. 2019;366:l5119. doi:10.1136/bmj.l5119.
- MacDonald TM, Walker SP, Hannan NJ, et al. Clinical tools and biomarkers to predict preeclampsia. EBioMedicine. 2022;75:103780. doi:10.1016/j.ebiom.2021.103780.
- Abalos E, Cuesta C, Grosso AL, et al. Global and regional estimates of preeclampsia and eclampsia: a systematic review. Eur J Obstet Gynecol Reprod Biol. 2013;170(1):1–7. doi:10.1016/j.ejogrb.2013.05.005.
- Gestational hypertension and preeclampsia: ACOG practice bulletin summary, number 222. Obstet Gynecol. 2020;135(6):1492–1495.
- Wadhwani NS, Narang AS, Mehendale SS, et al. Reduced maternal erythrocyte long chain polyunsaturated fatty acids exist in early pregnancy in preeclampsia. Lipids. 2016;51(1):85–94. doi:10.1007/s11745-015-4098-5.
- Herlambang H, Puspasari A, Maharani C, et al. Comprehensive fatty acid fractionation profilling in preeclampsia: a case control study with multivariable analysis. BMC Pregnancy Childbirth. 2022;22(1):8. doi:10.1186/s12884-021-04313-3.
- Burchakov DI, Kuznetsova IV, Uspenskaya YB. Omega-3 long-chain polyunsaturated fatty acids and preeclampsia: trials say “no,” but is it the final word? Nutrients. 2017;9(12):1364. doi:10.3390/nu9121364.
- Bakouei F, Delavar MA, Mashayekh-Amiri S, et al. Efficacy of n-3 fatty acids supplementation on the prevention of pregnancy induced-hypertension or preeclampsia: a systematic review and meta-analysis. Taiwan J Obstet Gynecol. 2020;59(1):8–15. doi:10.1016/j.tjog.2019.11.002.
- Li SN, Liu YH, Luo ZY, et al. The association between dietary fatty acid intake and the risk of developing preeclampsia: a matched case-control study. Sci Rep. 2021;11(1):4048. doi:10.1038/s41598-021-83674-3.
- Emdin CA, Khera AV, Kathiresan S. Mendelian randomization. JAMA. 2017;318(19):1925–1926. doi:10.1001/jama.2017.17219.
- Davies NM, Holmes MV, Davey Smith G. Reading mendelian randomisation studies: a guide, glossary, and checklist for clinicians. BMJ. 2018;362:k601. doi:10.1136/bmj.k601.
- Richmond RC, Davey Smith G. Mendelian randomization: concepts and scope. Cold Spring Harb Perspect Med. 2022;12(1):a040501. doi:10.1101/cshperspect.a040501.
- Larsson SC, Bäck M, Rees JMB, et al. Body mass index and body composition in relation to 14 cardiovascular conditions in UK biobank: a Mendelian randomization study. Eur Heart J. 2020;41(2):221–226. doi:10.1093/eurheartj/ehz388.
- Azizi-Tabesh G, Sadeghi H, Farhadi A, et al. The obesity associated FTO gene polymorphism and the risk of preeclampsia in Iranian women: a case-control study. Hypertens Pregnancy. 2023;42(1):2210685.
- Peng X, He D, Peng R, et al. Associations between IGFBP1 gene polymorphisms and the risk of preeclampsia and fetal growth restriction. Hypertens Res. 2023;46(9):2070–2084. doi:10.1038/s41440-023-01309-8.
- He D, Peng X, Xie H, et al. Genetic variations in angiotensinogen gene and risk of preeclampsia: a pilot study. J Clin Med. 2023;12(4):1509. doi:10.1038/s41440-023-01309-8.
- Lawlor DA, Harbord RM, Sterne JA, et al. Mendelian randomization: using genes as instruments for making causal inferences in epidemiology. Stat Med. 2008;27(8):1133–1163. doi:10.1002/sim.3034.
- Staley JR, Blackshaw J, Kamat MA, et al. PhenoScanner: a database of human genotype-phenotype associations. Bioinformatics. 2016;32(20):3207–3209. doi:10.1093/bioinformatics/btw373.
- Burgess S, Thompson SG, CRP CHD Genetics Collaboration. Avoiding bias from weak instruments in Mendelian randomization studies. Int J Epidemiol. 2011;40(3):755–764. doi:10.1093/ije/dyr036.
- Papadimitriou N, Dimou N, Tsilidis KK, et al. Physical activity and risks of breast and colorectal cancer: a mendelian randomisation analysis. Nat Commun. 2020;11(1):597. doi:10.1038/s41467-020-14389-8.
- Chen Y, Lyu S, Xiao W, et al. Sleep traits causally affect the brain cortical structure: a mendelian randomization study. Biomedicines. 2023;11(8):2296. doi:10.3390/biomedicines11082296.
- Bowden J, Davey Smith G, Burgess S. Mendelian randomization with invalid instruments: effect estimation and bias detection through egger regression. Int J Epidemiol. 2015;44(2):512–525. doi:10.1093/ije/dyv080.
- Burgess S, Thompson SG. Erratum to: interpreting findings from Mendelian randomization using the MR-egger method. Eur J Epidemiol. 2017;32(5):391–392. doi:10.1007/s10654-017-0276-5.
- Verbanck M, Chen CY, Neale B, et al. Publisher correction: detection of widespread horizontal pleiotropy in causal relationships inferred from Mendelian randomization between complex traits and diseases. Nat Genet. 2018;50(8):1196–1196. doi:10.1038/s41588-018-0164-2.
- Skytte HN, Christensen JJ, Gunnes N, et al. Metabolic profiling of pregnancies complicated by preeclampsia: a longitudinal study. Acta Obstet Gynecol Scand. 2023;102(3):334–343. doi:10.1111/aogs.14505.
- Nandi A, Wadhwani N, Randhir K, et al. Association of vitamin D with fatty acids in pregnancy. Prostaglandins Leukot Essent Fatty Acids. 2020;157:102030. doi:10.1016/j.plefa.2019.102030.
- Colvin BN, Longtine MS, Chen B, et al. Oleate attenuates palmitate-induced endoplasmic reticulum stress and apoptosis in placental trophoblasts. Reproduction. 2017;153(4):369–380. doi:10.1530/REP-16-0576.
- Wang Y, Walsh SW, Kay HH. Placental tissue levels of nonesterified polyunsaturated fatty acids in normal and preeclamptic pregnancies. Hypertens Pregnancy. 2005;24(3):235–245. doi:10.1080/10641950500281118.
- Firouzabadi FD, Shab-Bidar S, Jayedi A. The effects of omega-3 polyunsaturated fatty acids supplementation in pregnancy, lactation, and infancy: an umbrella review of meta-analyses of randomized trials. Pharmacol Res. 2022;177:106100. doi:10.1016/j.phrs.2022.106100.
- Lim WY, Chong M, Calder PC, et al. Relations of plasma polyunsaturated fatty acids with blood pressures during the 26th and 28th week of gestation in women of Chinese, Malay, and Indian ethnicity. Medicine (Baltimore). 2015;94(9):e571.). doi:10.1097/MD.0000000000000571.
- Devarshi PP, Grant RW, Ikonte CJ, et al. Maternal omega-3 nutrition, placental transfer and fetal brain development in gestational diabetes and preeclampsia. Nutrients. 2019;11(5):1107. doi:10.3390/nu11051107.
- Abdelrahman MA, Osama H, Saeed H, et al. Impact of n-3 polyunsaturated fatty acid intake in pregnancy on maternal health and birth outcomes: systematic review and meta-analysis from randomized controlled trails. Arch Gynecol Obstet. 2023;307(1):249–262. doi:10.1007/s00404-022-06533-0.
- Qiu C, Sanchez SE, Larrabure G, et al. Erythrocyte omega-3 and omega-6 polyunsaturated fatty acids and preeclampsia risk in Peruvian women. Arch Gynecol Obstet. 2006;274(2):97–103. doi:10.1007/s00404-006-0140-4.
- Mahomed K, Williams MA, King IB, et al. Erythrocyte omega-3, omega-6 and trans fatty acids in relation to risk of preeclampsia among women delivering at Harare maternity hospital, Zimbabwe. Physiol Res. 2007;56(1):37–50. doi:10.33549/physiolres.930859.
- Achamrah N, Ditisheim A. Nutritional approach to preeclampsia prevention. Curr Opin Clin Nutr Metab Care. 2018;21(3):168–173. doi:10.1097/MCO.0000000000000462.
- Kulkarni AV, Mehendale SS, Yadav HR, et al. Reduced placental docosahexaenoic acid levels associated with increased levels of sFlt-1 in preeclampsia. Prostaglandins Leukot Essent Fatty Acids. 2011;84(1–2):51–55. doi:10.1016/j.plefa.2010.09.005.
- Mathew SA, Bhonde RR. Omega-3 polyunsaturated fatty acids promote angiogenesis in placenta derived mesenchymal stromal cells. Pharmacol Res. 2018;132:90–98. doi:10.1016/j.phrs.2018.04.002.
- Khaire AA, Thakar SR, Wagh GN, et al. Placental lipid metabolism in preeclampsia. J Hypertens. 2021;39(1):127–134. doi:10.1097/HJH.0000000000002596.
- Yang Y, Wang Y, Lv Y, et al. Dissecting the roles of lipids in preeclampsia. Metabolites. 2022;12(7):590. doi:10.3390/metabo12070590.
- Ou M, Zhang Q, Zhao H, et al. Polyunsaturated fatty acid diet and upregulation of lipoxin A4 reduce the inflammatory response of preeclampsia. J Proteome Res. 2021;20(1):357–368. doi:10.1021/acs.jproteome.0c00439.