Abstract
Purpose: This review aims to overview the use of echocardiography in diagnosing neonatal pulmonary hypertension, assessing cardiac function, and understanding the significance and limitations of various parameters in clinical practice.Materials and methods: Advancements in echocardiography for diagnosing and assessing neonatal pulmonary hypertension, evaluating cardiac function, monitoring treatment effectiveness, and predicting prognosis are discussed.Results: Echocardiography is a pivotal tool for diagnosing and managing neonatal pulmonary hypertension. It should be used with other ultrasound parameters to confirm findings and provide comprehensive analysis for improved accuracy.Conclusion: Understanding the value of echocardiography in neonatal pulmonary hypertension diagnosis and management is crucial. Its integration with other imaging modalities enhances diagnostic accuracy and improves patient outcomes.
The definition of neonatal pulmonary hypertension has been updated in recent years. To highlight the role of different pulmonary diseases in the development of pulmonary hypertension in the neonatal period, the 6th World Forum on Pulmonary Hypertension (WSPH), Nice, France, 2018, divided neonatal pulmonary hypertension into two categories (1) persistent pulmonary hypertension of the newborn (PPHN), which is the most common cause of pulmonary hypertension (PH) in the neonatal period and indicates a persistent increase in pulmonary vascular resistance (PVR) after birth, with severe clinical symptoms such as hypoxemia [Citation1], the incidence of which accounts for about 0.2% of live births and about 10% of all newborns with respiratory failure, with a high mortality rate [Citation2]; (2) Pulmonary hypertension based on lung disease and/or hypoxia belongs to the category of developmental lung diseases, such as prenatal and postnatal injuries to the alveoli, pulmonary vasculature, and connective tissue, and is commonly associated with bronchopulmonary dysplasia (BPD) [Citation3].
In PPHN, elevated pulmonary artery pressure (PAP) is usually secondary to elevated PVR and has the following etiologies: (1) maladaptation: the structure and number of pulmonary vessels are normal but vasoreactivity of them is abnormal; (2) maldevelopment: increased smooth muscle cell thickness and increased distal extension of muscle to vessels that are usually not muscularized; and (3) underdevelopment: lung hypoplasia associated with decreased pulmonary artery number [Citation4,Citation5].
Echocardiography (ECHO) has been widely used in the diagnosis of neonatal pulmonary hypertension to exclude PH due to congenital heart disease or other related underlying conditions, as well as to assess the degree of PAP, evaluate cardiac function therapeutic management and assess risk prognosis, but its accuracy is still controversial. Therefore, it is important to objectively understand the advantages and disadvantages of echocardiography in the application of PH in neonates. This article reviews the progress of echocardiography in the diagnosis and evaluation of cardiac function in neonatal pulmonary hypertension.
1. Echocardiographic diagnosis of PH and extent assessment
The right heart catheterization, an invasive test, is still the "gold standard” for the determination of PAP and PVR. It carries certain risks that cannot be tolerated by critically ill children, especially neonates. The indication for any cardiac catheterization in neonates has to be carefully evaluated [Citation6]. On the other hand, clinical practice guidelines recommend the use of ECHO for the diagnosis of PH. For some experts, ECHO is considered as the reference standard to confirm PPHN [Citation7]. The expert consensus on neonatal pulmonary hypertension developed by the Neonatology Group of the Pediatrics Branch of the Chinese Medical Association in 2017 states the criteria for the ultrasound diagnosis of neonatal pulmonary hypertension as follows: systolic pulmonary artery pressure (sPAP) >35 mmHg or >2/3 of the systolic pressure of the body circulation, or the presence of a right-to-left shunt at the level of the atria or the ductus arteriosus. ECHO can be used to estimate PAP and to assess the direction of shunts in the patent ductus arteriosus (PDA) or patent foramen ovale (PFO) (), but there is a lack of reliable noninvasive tests for the determination of PVR. Therefore, the ECHO assessment of PAP and the shunt direction of PDA and PFO are mainly described here.
Table 1. Overview of echocardiographic parameters for the evaluation of pulmonary artery pressure, pulmonary vascular resistance, and shunts in persistent pulmonary hypertension of the newborn.
1.1. Pulmonary artery pressure assessment
In clinical practice, parameters used to estimate PAP on ECHO include tricuspid regurgitation peak velocity (VmaxTR) (), peak pulmonary regurgitation flow velocity (VmaxPR) (), ratio of pulmonary artery acceleration time (PAAT) to right ventricular ejection time (RVET) (), and end-systolic interventricular septum (IVS) shape. The sPAP, mean pulmonary artery pressure (mPAP), and end-diastolic pulmonary artery pressure (EDPAP) obtained directly or indirectly by ECHO measurements have been used to diagnose and monitor PH. It has been reported that cardiac catheterization was highly correlated with sPAP obtained by ECHO assessment [4V2 (VmaxTR) + right atrial pressure] (r = 0.917.p < 0.001) in pediatric PH [Citation8]; furthermore, Jain et al. [Citation9] confirmed that PAAT was inversely correlated with sPAP and mPAP, and PAAT was shortened in patients with PH. They also considered that PAAT/RVET <0.23 in neonates suggested increased PAP. Neonatal normative values for the PAAT are available, providing physicians the opportunity to compare neonates with suspected PH to normal neonates [Citation10]; the left ventricular systolic eccentricity index was obtained at the level of the parasternal left ventricular (LV) short-axis papillary muscle (LVsEI), LVsEI = D1 (LV internal diameter parallel to the IVS)/D2 (LV internal diameter perpendicular to the IVS), suggesting an increased RV afterload when LVsEI > 1.0 [Citation11] (). The above study confirms that ECHO plays an irreplaceable role in the diagnosis of PH.
Figure 1. Tricuspid regurgitation peak velocity (Vmax TR). The estimation of systolic pulmonary artery pressure (sPAP) can be derived from the tricuspid regurgitation jet. The peak velocity of the tricuspid regurgitation jet measures 3.95 m/s, which corresponds to a maximum pressure gradient of 62.41 mmHg as per the modified Bernoulli equation.
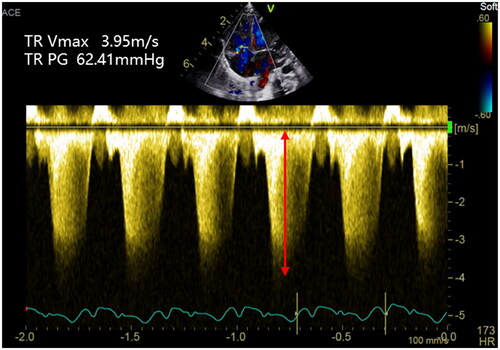
Figure 2. Peak pulmonary regurgitation flow velocity (VmaxPR). The estimation of mean pulmonary artery pressure (mPAP) can be derived from the pulmonary regurgitation jet. The peak velocity of the pulmonary regurgitation jet measures 1.95 m/s, which corresponds to a maximum pressure gradient of 15.21 mmHg as per the modified Bernoulli equation.
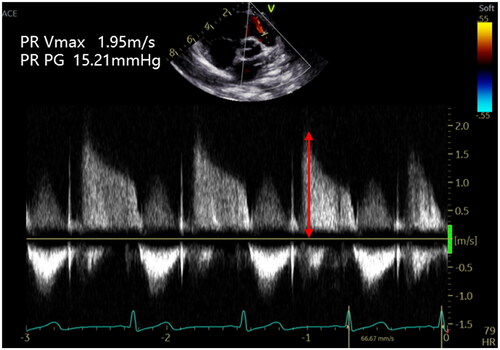
1.2. Diversion direction of PDA and PFO
When the flow direction of the neonatal PDA or PFO is a right-to-left or bidirectional shunt, it suggests that the PAP exceeds or is equal to the systemic circulatory pressure (physiologic bidirectional shunts that occur within the first 12h of life in healthy neonates should be excluded) [Citation7]. The change in flow direction, although alleviating the right heart pressure burden in pediatric patients with PH, is accompanied by a decrease in systemic oxygen saturation. Breinig et al. [Citation12] discovered that persistent right-to-left shunts in patent ductus arteriosus were linked to mortality outcomes, suggesting that such shunts serve as an indicator of disease severity.
However, due to the limitations of ECHO measurements such as angle dependence, susceptibility to neonatal heart rate and unavailability [Citation4, Citation13], it is often impossible to obtain the aforementioned ECHO data or to make the accuracy of the data measurements low. Since no single ECHO parameter can be used to diagnose PH alone, a comprehensive evaluation should be performed in cases of suspected PH to objectively interpret ECHO results.
2. Echocardiographic assessment of cardiac function
Persistently elevated PVR with neonatal PH can lead to increased RV afterload, RV dilatation, RV wall hypertrophy, and RV insufficiency. Due to the interaction of the right and left ventricles, right ventricular enlargement can lead to a shift of the septum from right to left, causing a decrease in left ventricular filling and compliance, and finally triggering hypoxemia and hypotension [Citation14]. Some kinds of neonatal pulmonary hypertension (e.g., caused by neonatal asphyxia) can also directly lead to myocardial injury, which in turn can abnormal left ventricular systolic and diastolic function, leading to pulmonary venous hypertension and aggravating PH. Taking together, a vicious circle is created and makes cardiac function worse.
2.1. Assessment of right heart function
Right heart function is a key determinant of prognosis in patients with PH and is associated with mortality and the degree of right heart failure in patients [Citation15].
Parameters of right ventricular systolic function including: (1) Fraction of right ventricular area change (RV-FAC): FAC (%) = (EDA - ESA)/EDA (ESA: end-systolic area, EDA: end-diastolic area). Using 2D ultrasound images, Levy [Citation16] et al. reported that the normal value of RV-FAC in healthy term neonates was 33 ± 5% at birth, increasing to 39 ± 4% by 1 month of age. The limitation of this study is that this parameter was measured in one plane and did not reflect the overall right ventricular systolic function in the presence of right ventricular segmental wall motion abnormalities. Bulbul et al. [Citation17] used three-dimensional ultrasound speckle tracking imaging (3D-STI) to overcome this limitation. They revealed that RV-FAV was 46 ± 3.4% in 50 normal full-term neonates. However, their method requires high image quality and its application is limited. (2) Tricuspid annular plane systolic excursion (TAPSE): Longitudinal systolic displacement of the base of the right ventricular free wall (at the level of the tricuspid annulus) was measured at the apical four-chamber view (). The TAPSE ranged from 0.44 cm (Z-score ±2: 0.30–0.59 cm) in preterm neonates in the 26th week of gestation to 1.03 cm (Z-score ±2: 0.85–1.21 cm) in term neonates in the 41st week of gestation. The TAPSE values increased in a linear way from the 26th to 41st week of gestation [Citation18]. Chikkabyrappa et al. [Citation19] showed a significant reduction in TAPSE in neonates with PH, suggesting reduced RV longitudinal systolic function. The limitation of this study is the angular dependence of the measurement. (3) Right ventricular global longitudinal strain (RV GLS) and strain rate: Strain reflects the degree of myocardial deformation, and strain rate represents the ability of the myocardium to produce deformation. Longitudinal contraction is the main mode of deformation for right ventricle, therefore longitudinal strain is a reliable parameter to reflect right ventricular systolic function (). In a study by Jain et al. [Citation20] it was found that RV GLS was reduced in PPHN patients compared to controls (−21.6%±4.6% vs −16.9%±5.4%, p < 0.01). RV GLS can be obtained by the speckle tracking technique and is independent of the angle of measurement [Citation21]. However, studies about RV GLS in neonatal PH are scarce and further clinical validation is needed.
Figure 5. Tricuspid annular plane systolic excursion (TAPSE). TAPSE is a measure of RV longitudinal function and obtained from the 4-chamber view using the M-Mode with the cursor aligned along the direction of the lateral annulus.
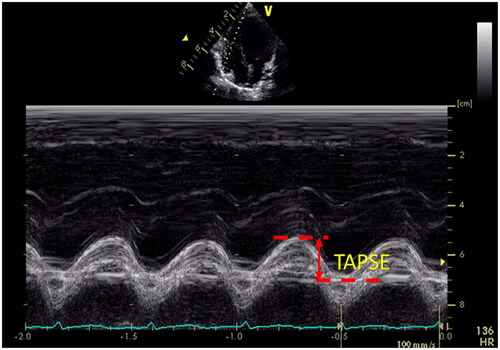
Figure 6. Right ventricular global longitudinal strain (RV GLS). The endocardial boundary of the right ventricle was automatically delineated using two-dimensional speckle tracking echocardiography on the apical 4-chamber view, with a specific focus on the RV. This included the RV apical segment, RV free wall, and RV interventricular septum to demonstrate the maximum diameter of the RV. The myocardial segments visualized by two-dimensional speckle tracking echocardiography comprised the basal, middle, and apical segments of the RV free wall, as well as the apical, middle, and basal segments of the RV interventricular septum. The RV GLS value in the figure is −19.7%.
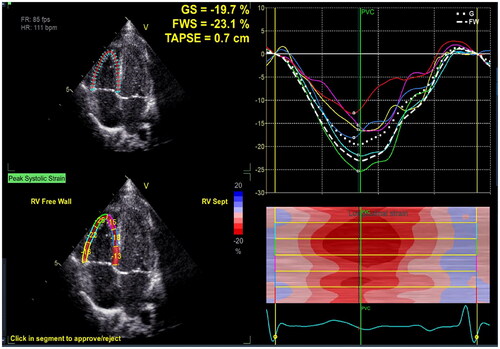
Overall right ventricular function: (1) Myocardial performance index (MPI, also referred to as Tei index): Diastolic function is traditionally studied by measuring the tricuspid valve inflow velocities (ratio (E/A)) during diastole by Pulsed Doppler (PW) from the apical 4-chamber view. Tei index = (ventricular isovolumic ejection time + ventricular isovolumic relaxation time)/ventricular ejection time, which can be obtained by both PW and Tissue Doppler imaging (TDI) methods, with the TDI method being more sensitive than the PW method for assessing myocardial function (). Normal values of Tei index in healthy neonates measured by TDI are 0.25–0.38 [Citation22]. The Tei index was increased in right heart insufficiency, and the Tei index obtained by the TDI method and the PW method were greater than 0.55 and 0.40, respectively. Limitations of this method include: angular dependence of Tei index measurement; shortened isovolumic diastolic time when right atrial pressure is elevated due to tricuspid regurgitation, which can lead to underestimation of Tei index. (2) Right ventricular systolic to diastolic duration ratio (RV S/D ratio): Reflects right ventricular load and contractility. Elevated S/D ratio indicates increased right ventricular afterload and right ventricular insufficiency. Aggarwal S et al. [Citation23] compared ECHO measurements between normal controls and 117 PPHN patients, and found that RV S/D was significantly higher in the PPHN group compared to normal controls and significantly higher in those with poor prognosis compared to those with good prognosis, suggesting that an elevated RV S/D ratio is a marker of RV dysfunction and is associated with poor outcomes in PPHN. However, certain therapeutic measures (e.g. mechanical ventilation, rehydration, and positive inotropic drugs) may affect the accuracy of this parameter measurement.
Figure 7. Myocardial Performance Index—Tei index. The Tei index obtained by Tissue Doppler is illustrated in the figure. Tei index = the sum of isovolumic contraction and relaxation time divided by ejection time (a − b/a).
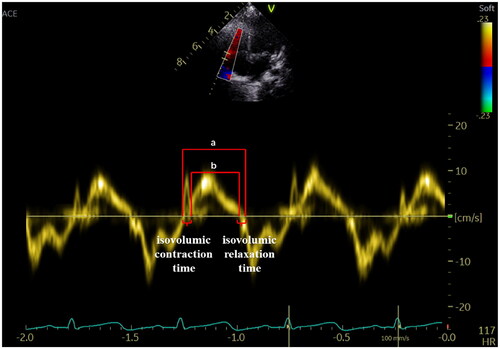
Right atrial function: Previous studies have mostly focused on the evaluation of the right ventricle in patients with PH, but the RV geometry is complex and each parameter has limitations. The right atrium (RA) is an integral part of the right heart and plays an important role in maintaining right ventricular filling. Studies [Citation15, Citation24–27] have been performed to evaluate right atrial function in adult patients with PH associated with chronic obstructive pulmonary disease, primary pulmonary hypertension, and left heart disease by applying two-dimensional ultrasound speckle tracking imaging (2D-STI) and 3D-STI. Additionally, these techniques were used to evaluate the relationship between RA function and PH in adult and pediatric patients, the changes in RA function during different phases with the degree of PH, as well as predict the potential risk of adverse cardiovascular events among patients with PH. Previous studies have shown that RA volume and function are indispensable indicators for assessing the prognosis of patients with PH [Citation15, Citation28–30].
Right atrial function can be well-evaluated in the following three phases: the reservoir phase during atrial filling (ventricular systole), the conduit phase during passive emptying into the ventricle (early ventricular diastole), and the active pump phase during atrial systole (late ventricular diastole) (). Jone PN [Citation31], Querejeta Roca [Citation32] et al. showed that RA reservoir and conduit function were impaired in PH patients, and the degree of decline was related to RV dysfunction, but not to the degree of PH. A study [Citation33] assessed the alterations in right heart function among children with PPHN using 2D-STE and revealed that RA function was impaired in all three stages compared to the normal control group (all p < 0.05). The combination of right atrial storage strain and RV GLS proved to be the most effective indicator for early detection of right heart function injury in PPHN, exhibiting a sensitivity and specificity of 0.905 and 1.000, respectively, with an AUC of 0.985. The above studies confirmed that RA function and RV function were both impaired in PH patients, and there was a correlation between some of the parameters, and RA function parameters are expected to provide more basis for our evaluation of right heart function. However, there are few studies on neonates; whether RA strain is altered before RV strain during the development of PH remains to be further investigated. The RA function in neonates with PH, and its correlation with RV function, the degree of PH and the prognosis of the neonates also deserve further investigation.
Figure 8. Right atrial strain (RA LS). The endocardial boundary of the right atrial was automatically delineated using two-dimensional speckle tracking echocardiography on the apical 4-chamber view, with a specific focus on the RA. RA LS consists of three phases: the reservoir phase, the conduit phase, and the active pump phase.
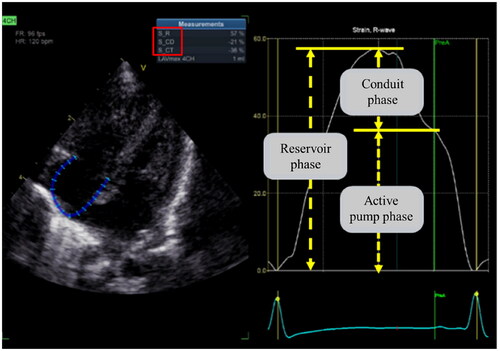
2.2. Assessment of left ventricular function
PPHN patients can develop right-to-left shunts (reduced pulmonary venous return) and ventricular-ventricular interactions (flattening of the septum), resulting in lower LV preload, lower output, and normal or mildly decreased LV ejection fraction (LVEF). Jain et al. [Citation20] used ECHO to assess left and right ventricular size and function in 49 PPHN patients and found that PPHN patients had biventricular cardiac dysfunction, and there was a correlation between right and left ventricular function. Recent studies have found that LV function was impaired in patients with PH despite normal LVEF and that LV dysfunction was strongly associated with poor clinical outcomes [Citation34]. The above-mentioned studies suggest that the assessment of LV function in patients with PH cannot be neglected. Moreover, when LV systolic or diastolic dysfunction is present it can lead to LV filling restriction, which in turn can cause neonatal PH, and care should be taken to exclude this condition.
Left ventricular systolic function: (1) LV fractional shortening (LV FS): The FS is calculated as the difference between LV end-systolic thickness diameter and LV end-diastolic thickness diameter, divided by LV end-diastolic thickness diameter. In healthy neonates, the normal range for FS is 25% to 45%. However, it should be noted that the FS may be limited due to septal flattening or paradoxical septal motion caused by myocardial hypertrophy and increased right ventricular pressure; (2) Pressure-strain loop (PSL) is an noninvasive method to assess ventricular systolic function by quantifying the work by the myocardium. PSL parameters are as follows: global constructive work (GCW), positive work done by systolic myocardial shortening or diastolic myocardial lengthening that contributes to LV ejection; global wasted work (GWW), negative work done by systolic myocardial lengthening or diastolic myocardial shortening that counteracts LV ejection; global work efficiency (GWE), which is GWE = GCW/(GCW + GWW); global work index (GWI), which refers to the total work done from mitral valve closure to mitral valve opening, isovolumic systole, and isovolumic diastole during one cardiac cycle, is often expressed as the area enclosed by the LV PSL. Yanase et al. [Citation35] used PSL to measure myocardial work in 113 healthy neonates and obtained reference ranges for myocardial work in neonates: 1008.5 ± 200.1 mmHg%, 58.1 ± 28.1 mmHg%, 93.1 ± 2.9%, and 698.7 ± 147.9 mmHg% for GCW, GWW, GWE, and GWI, respectively. Wang et al. [Citation36], after excluding PH caused by left heart disease and hypoxia, studied 52 adult patients with PH and found that LVGWE, LVGWI and LVGCW were lower in patients with PH than in healthy controls, while LVGWW was abnormally increased in patients with PH. PSL parameters correlated well with clinical outcomes and other ECHO parameters for assessing cardiac function (all p < 0.05). It is suggested that PSL can be used as a new parameter to assess cardiac function in patients with PH. However, this method is not yet widely used in clinical practice, and there are few studies in neonatal PH.
Overall left ventricular function: AbdelMassih et al. [Citation37] included 30 full-term neonates diagnosed with PPHN by ECHO and found that the LV Tei index (LV Myocardial performance index) was a better predictor for adverse outcomes than the RV Tei index in patients with PPHN, suggesting that neonatal PH patients also have impaired left heart function. However, the Tei index reflects both LV systolic and diastolic function, is not specific, and is influenced by cardiac preload and afterload. Moreover, the reproducibility of the Tei index is yet to be further demonstrated because of the faster heart rate in neonates.
3. Echocardiographic assessment of treatment outcome and prognosis
Neonates with PH are often severely ill and require ventilator and vasoactive drug support, such as positive inotropes and pulmonary vasodilators inhaled nitric oxide (iNO) [Citation38]. ECHO can be used to diagnose PH, quantify disease severity by estimating sPAP and assess biventricular function in the course of the disease in pediatric patients. This enables identification of potential pathophysiological changes and possible adverse clinical outcomes, such as cardiac insufficiency, leading to accurate risk assessment, long-term follow-up, and monitoring of the child’s response to therapy [Citation20]. Patients with severe cardiopulmonary failure also require extracorporeal membrane pulmonary oxygenation (ECMO) support. The ECHO plays a fundamental role in the management of children who potentially need ECMO support, including selecting appropriate patients, guiding intubation positioning, monitoring complications, and providing indications for termination of ECMO. Malowitz et al. [Citation39] showed that changes in RV-FAC, TAPSE, and RV GLS in PH neonates predicted the prognosis of the children: When the neonate’s RV-FAC was less than 19% or TAPSE <4 mm, it predicted severe disease and the need for ECMO treatment, the sensitivity and specificity of the latter were 56% and 85%, respectively; when the RV GLS was greater than or equal to −9%, it also predicted the need for ECMO treatment, with sensitivity 52% and specificity 77% respectively. Aggarwal et al. [Citation23] showed that the sensitivity of RV S/D > 1.3 predicted mortality or the need for ECMO treatment with a sensitivity of 93%. Persistence of right to left shunts can also be considered as a predictor for poor outcomes [Citation12]. The results of all the above studies indicate that ECHO has a greater significance in guiding treatment and assessing prognosis. However, the main limitation of ECHO is that the interobserver agreement is not very good in qualitative assessment [Citation40].
4. Summary and outlook
In conclusion, ECHO is safe, noninvasive, and convenient, and has become an important tool for the diagnosis and management of PH in neonates. It can be used to work up etiologic exclusionary diagnosis, evaluate PAP and assess cardiac function, as well as predict treatment outcome. However, ECHO can only perform indirect measurements of PAP and cardiac function, and its accuracy in estimating PAP and cardiac function in neonatal applications needs further validation. Although the application of ECHO for the diagnosis of PH in neonates is as popular as that in adults, the reference ranges of relevant parameters in neonates and children differ from those in adults. Different measurement methods and evaluation indicators have their own constraints, and none of them can be independently used for the evaluation. Therefore, it is necessary to take several parameters together and analyze them comprehensively. Afterwards, the accuracy of echocardiographic evaluation in neonates with PH might be improved.
Consent for publication
Informed consent has been obtained from participants to publish information in online open access publications.
Authors’ contributions
Zehang Hu: writing the article, reviewing and analyzing the literature; Shumin Fan: guiding the conception of the article, reviewing and analyzing the literature, suggesting revisions, critically reviewing the intellectual content of the article, and finalizing the published version. All authors contributed to editorial changes in the manuscript. All authors read and approved the final manuscript.
Disclosure statement
No potential conflict of interest was reported by the author(s).
Data Availability statement
Data availability is not applicable to this article as no new data were created or analyzed in this study.
Additional information
Funding
References
- Jain A, McNamara PJ. Persistent pulmonary hypertension of the newborn: advances in diagnosis and treatment. Semin Fetal Neonatal Med. 2015;20(4):1–11. doi:10.1016/j.siny.2015.03.001.
- Sankaran D, Lakshminrusimha S. Pulmonary hypertension in the newborn-etiology and pathogenesis. Semin Fetal Neonatal Med. 2022;27(4):101381. doi:10.1016/j.siny.2022.101381.
- Hansmann G, Sallmon H, Roehr CC, et al. Pulmonary hypertension in bronchopulmonary dysplasia. Pediatr Res. 2021;89(3):446–455. doi:10.1038/s41390-020-0993-4.
- More K, Soni R, Gupta S. The role of bedside functional echocardiography in the assessment and management of pulmonary hypertension. Semin Fetal Neonatal Med. 2022;27(4):101366. doi:10.1016/j.siny.2022.101366.
- Mandell E, Kinsella JP, Abman SH. Persistent pulmonary hypertension of the newborn. Pediatr Pulmonol. 2021;56(3):661–669. doi:10.1002/ppul.25073.
- Sallmon H, Meinel K, Nordmeyer J, et al. Cardiac catheterization in infants with bronchopulmonary Dysplasia-Beyond economic considerations. J Pediatr. 2022;250:110. doi:10.1016/j.jpeds.2022.07.055.
- Singh Y, Lakshminrusimha S. Pathophysiology and management of persistent pulmonary hypertension of the newborn. Clin Perinatol. 2021;48(3):595–618. doi:10.1016/j.clp.2021.05.009.
- Sohail A, Korejo HB, Shaikh AS, et al. Correlation between echocardiography and cardiac catheterization for the assessment of pulmonary hypertension in pediatric patients. Cureus. 2019;11(8):e5511. doi:10.7759/cureus.5511.
- Jain A, Mohamed A, Kavanagh B, et al. Cardiopulmonary adaptation during first day of life in human neonates. J Pediatr. 2018;200:50–57 e2. doi:10.1016/j.jpeds.2018.04.051.
- Koestenberger M, Grangl G, Avian A, et al. Normal reference values and z scores of the pulmonary artery acceleration time in children and its importance for the assessment of pulmonary hypertension. Circ Cardiovasc Imaging. 2017;10(1):e005336. doi:10.1161/CIRCIMAGING.116.005336.
- Bhattacharya S, Sen S, Levy PT, et al. Comprehensive evaluation of right heart performance and pulmonary hemodynamics in neonatal pulmonary hypertension: evaluation of cardiopulmonary performance in neonatal pulmonary hypertension. Curr Treat Options Cardiovasc Med. 2019;21(2):10. doi:10.1007/s11936-019-0713-8.
- Breinig S, Dicky O, Ehlinger V, et al. Echocardiographic parameters predictive of poor outcome in persistent pulmonary hypertension of the newborn (PPHN): preliminary results. Pediatr Cardiol. 2021;42(8):1848–1853. doi:10.1007/s00246-021-02677-z.
- Sanchez Mejia AA, Rodgers NJ. Evaluation and monitoring of pulmonary hypertension in neonates with congenital diaphragmatic hernia. Curr Treat Options Cardiovasc Med. 2019;21(2):11. doi:10.1007/s11936-019-0711-x.
- Martinho S, Adão R, Leite-Moreira AF, et al. Persistent pulmonary hypertension of the newborn: pathophysiological mechanisms and novel therapeutic approaches. Front Pediatr. 2020;8:342. doi:10.3389/fped.2020.00342.
- Alenezi F, Mandawat A, Il’Giovine ZJ, et al. Clinical utility and prognostic value of right atrial function in pulmonary hypertension. Circ Cardiovasc Imaging. 2018;11(11):e006984. doi:10.1161/CIRCIMAGING.117.006984.
- Levy PT, Dioneda B, Holland MR, et al. Right ventricular function in preterm and term neonates: reference values for right ventricle areas and fractional area of change. J Am Soc Echocardiogr. 2015;28(5):559–569. doi:10.1016/j.echo.2015.01.024.
- Bulbul Z, Siblini G, Tamim H, et al. Right ventricular volumes, ejection fraction, and systolic function indices in normal neonates by three-dimensional speckle-tracking echocardiography. Pediatr Cardiol. 2022;43(1):181–190. doi:10.1007/s00246-021-02716-9.
- Koestenberger M, Nagel B, Ravekes W, et al. Systolic right ventricular function in preterm and term neonates: reference values of the tricuspid annular plane systolic excursion (TAPSE) in 258 patients and calculation of Z-score values. Neonatology. 2011;100(1):85–92. doi:10.1159/000322006.
- Chikkabyrappa SM, Critser P, Roane J, et al. Tripartite assessment of right ventricular systolic function in persistent pulmonary hypertension of the newborn. Pediatr Cardiol. 2020;41(6):1206–1211. doi:10.1007/s00246-020-02376-1.
- Jain A, El-Khuffash AF, van Herpen CH, et al. Cardiac function and ventricular interactions in persistent pulmonary hypertension of the newborn. Pediatr Crit Care Med. 2021;22(2):e145–e157. doi:10.1097/PCC.0000000000002579.
- Voigt JU, Cvijic M. 2- and 3-dimensional myocardial strain in cardiac health and disease. JACC Cardiovasc Imaging. 2019;12(9):1849–1863. doi:10.1016/j.jcmg.2019.01.044.
- Tissot C, Singh Y. Neonatal functional echocardiography. Curr Opin Pediatr. 2020;32(2):235–244. doi:10.1097/MOP.0000000000000887.
- Aggarwal S, Natarajan G. Echocardiographic correlates of persistent pulmonary hypertension of the newborn. Early Hum Dev. 2015;91(4):285–289. doi:10.1016/j.earlhumdev.2015.02.008.
- Richter MJ, Zedler D, Berliner D, et al. Clinical relevance of right atrial functional response to treatment in pulmonary arterial hypertension. Front Cardiovasc Med. 2021;8:775039. doi:10.3389/fcvm.2021.775039.
- Wessels JN, Mouratoglou SA, van Wezenbeek J, et al. Right atrial function is associated with right venticular diastolic stiffness: RA-RV interaction in pulmonary arterial hypertension. Eur Respir J. 2022;59(6):2101454. doi:10.1183/13993003.01454-2021.
- Lichtblau M, Bader PR, Saxer S, et al. Right atrial pressure during exercise predicts survival in patients with pulmonary hypertension. J Am Heart Assoc. 2020;9(22):e018123. doi:10.1161/JAHA.120.018123.
- Richter MJ, Fortuni F, Wiegand MA, et al. Association of right atrial conduit phase with right ventricular lusitropic function in pulmonary hypertension. Int J Cardiovasc Imaging. 2020;36(4):633–642. doi:10.1007/s10554-019-01763-x.
- Hasselberg NE, Kagiyama N, Soyama Y, et al. The prognostic value of right atrial strain imaging in patients with precapillary pulmonary hypertension. J Am Soc Echocardiogr. 2021;34(8):851–861.e1. doi:10.1016/j.echo.2021.03.007.
- Frank BS, Schäfer M, Thomas TM, et al. Longitudinal assessment of right atrial conduit fraction provides additional insight to predict adverse events in pediatric pulmonary hypertension. Int J Cardiol. 2021;329:242–245. doi:10.1016/j.ijcard.2020.12.073.
- Frank BS, Schafer M, Thomas TM, et al. Right atrial conduit phase emptying predicts risk of adverse events in pediatric pulmonary arterial hypertension. J Am Soc Echocardiogr. 2020;33(8):1006–1013. doi:10.1016/j.echo.2020.02.015.
- Jone PN, Schafer M, Li L, et al. Right atrial deformation in predicting outcomes in pediatric pulmonary hypertension. Circ Cardiovasc Imaging. 2017;10(12):e006250. doi:10.1161/CIRCIMAGING.117.006250.
- Querejeta Roca G, Campbell P, Claggett B, et al. Right atrial function in pulmonary arterial hypertension. Circ Cardiovasc Imaging. 2015;8(11):e003521. doi:10.1161/CIRCIMAGING.115.003521.
- Hu ZH, Xia B, Jiang JB, et al. Evaluation of right heart function in persistent pulmonary hypertension of the newborn by two-dimensional speckle-tracking imaging. Chinese Journal of Ultrasonography. 2023;32(11):968–976. doi:10.3760/cma.j.cn131148-20230507-00260.
- Padervinskienė L, Krivickienė A, Hoppenot D, et al. Prognostic value of left ventricular function and mechanics in pulmonary hypertension: a pilot cardiovascular magnetic resonance feature tracking study. Medicina (Kaunas). 2019;55(3) doi:10.3390/medicina55030073.
- Yanase Y, Iwashima S, Takahashi K. Echocardiographic reference ranges of Non-Invasive myocardial work indices in newborns. Circ Rep. 2022;4(9):429–438. doi:10.1253/circrep.CR-22-0045.
- Wang J, Ni C, Yang M, et al. Apply pressure-strain loop to quantify myocardial work in pulmonary hypertension: a prospective cohort study. Front Cardiovasc Med. 2022;9:1022987. doi:10.3389/fcvm.2022.1022987.
- AbdelMassih AF, Al Zahraa Hassan F, El-Gammal A, et al. The overlooked left ventricle in persistent pulmonary hypertension of the newborn. J Matern Fetal Neonatal Med. 2021;34(1):72–76. doi:10.1080/14767058.2019.1598363.
- Nandula PS, Shah SD. Persistent Pulmonary Hypertension of the Newborn. Treasure island (FL): ineligible companies. Disclosure: sanket Shah Declares No Relevant Financial Relationships with Ineligible Companies; 2023.
- Malowitz JR, Forsha DE, Smith PB, et al. Right ventricular echocardiographic indices predict poor outcomes in infants with persistent pulmonary hypertension of the newborn. Eur Heart J Cardiovasc Imaging. 2015;16(11):1224–1231. doi:10.1093/ehjci/jev071.
- Smith A, Purna JR, Castaldo MP, et al. Accuracy and reliability of qualitative echocardiography assessment of right ventricular size and function in neonates. Echocardiography. 2019;36(7):1346–1352. doi:10.1111/echo.14409.