Abstract
Objective
The objective of this study is to explore the functions and mechanisms of the LncRNA-KCNQ1OT1/miR-29a-3p/SOCS3 molecular pathway in the context of unexplained recurrent spontaneous abortion (URSA).
Methods
We conducted qRT-PCR to assess the levels of LncRNA-KCNQ1OT1, miR-29a-3p, and SOCS3 in both abortion tissues from women who experienced URSA and healthy early pregnant women. A dual-luciferase assay was employed to investigate whether miR-29a-3p targets SOCS3. Furthermore, RNA IP and RNA Pull-Down assays were employed to confirm the interaction between KCNQ1OT1 and SOCS3 with miR-29a-3p. RNA FISH was used to determine the cellular localization of KCNQ1OT1. Additionally, trophoblast cells (HTR8/SVneo) were cultured and the CCK-8 assay was utilized to assess cell proliferation, while flow cytometry was employed to analyze cell apoptosis.
Results
Compared to abortion tissues obtained from healthy early pregnant individuals, those from women who experienced URSA displayed a notable downregulation of KCNQ1OT1 and SOCS3, accompanied by an upregulation of miR-29a-3p. Suppression of KCNQ1OT1 resulted in the inhibition of cell proliferation and the facilitation of apoptosis in HTR8/SVneo cells. Our findings suggest that KCNQ1OT1 may exert a regulatory influence on SOCS3 through a competitive binding mechanism with miR-29a-3p. Notably, KCNQ1OT1 exhibited expression in both the cytoplasm and nucleus, with a predominant localization in the cytoplasm. Furthermore, we observed a negative regulatory relationship between miR-29a-3p and SOCS3, as the miR-29a-3p mimic group demonstrated significantly reduced cell proliferation and an increased rate of apoptosis when compared to the negative control (NC mimic) group. Additionally, the SOCS3 Vector group exhibited a substantial improvement in proliferation capability and a marked reduction in the apoptosis rate in comparison to the NC Vector group. The miR-29a-3p mimic + SOCS3 Vector group demonstrated a remarkable enhancement in proliferation and a reduction in apoptosis when compared to the miR-29a-3p mimic group.
Conclusion
The competitive binding of miR-29a-3p to LncRNA-KCNQ1OT1 appears to result in the elevation of SOCS3 expression, consequently fostering the proliferation of trophoblast cells while concomitantly suppressing apoptosis.
1. Introduction
Recurrent spontaneous abortion (RSA) is an early pregnancy complication, which is defined as two or more spontaneous pregnancy loss with the same couple. The European Society of Human Reproduction and Embryology (ESHRE) considers it often occur continuously prior to 24 gestational weeks, which is a major challenge in the field of human reproduction. It is a deeply distressing event with profound consequences for those affected, particularly impacting women’s health and potentially resulting in pregnancy-related complications [Citation1,Citation2]. The etiology of RSA is intricate and multifaceted, encompassing factors such as uterine anatomical anomalies, chromosomal irregularities, infections, endocrine disorders, and autoimmune elements among established causal agents. Studies have shown, ANA + IgG injection in mice is able to induce fetal resorption and complement activation. The presence on placental tissues of immune complexes and complement fragments suggests the complement activation as a possible mechanism of placental damage [Citation3]. APLs with anti-2GPI specificity might bind their own antigenic target expressed on the trophoblast cell membranes, induce cell perturbation that ends in a defective maturation, but do not display a clear pro-apoptotic effect [Citation4]. The antibody binding, syncytiotrophoblast apoptosis, and consequently, inhibition of trophoblast gonadotropin secretion may represent key mechanisms by which anti-annexin V antibodies, once bound, can affect embryo implantation and pregnancy outcome [Citation5]. IL-3 is able to restore trophoblast invasiveness and differentiation, and it seems to be effective at physiologic and lower concentrations in the presence of antiphospholipid antibodies. This might represent an important role for IL-3 in the treatment ofantiphospholipid syndrome [Citation6]. Remarkably, approximately 50–60% of RSA cases remain unexplained, designated as unexplained recurrent spontaneous abortion (URSA) [Citation7,Citation8]. A pressing clinical imperative lies in the elucidation of the early pathogenesis of URSA, which can furnish a robust theoretical underpinning for the diagnosis and management of unexplained recurrent miscarriages.
There is no literature report on the relationship between SOCS3 and early embryonic development. Bioinformatics analysis found that the upstream regulation mechanism of SOCS3 may be reflected by lncRNA-KCNQ1OT1competitive binding of miR-29a-3p. Therefore, we targeted the molecular axis of lncRNA-KCNQ1OT1/miR-29a-3p/SOCS3. Based on the summary of the current research frontier development trend, this topic puts forward a scientific hypothesis, which has a certain innovation, and provides clues for the regulation mechanism of early embryonic development. This study delved into the molecular axis between LncRNA-KCNQ1OT1, miR-29a-3p, and SOCS3, revealing their role in unexplained recurrent spontaneous abortion. This helps to understand the potential mechanisms of natural abortion and provides new ideas for prevention and treatment. Simultaneously, the study involves the role of long-chain non coding RNA (LncRNA), indicating that non coding RNA may play a more important role in human physiology and diseases. This provides impetus for in-depth research on non-coding RNA in the future. Finally, by studying this molecular axis, new therapeutic targets may be discovered. The specific molecular axis has been proven to be a key factor in recurrent spontaneous abortion, so developing targeted treatment methods or drugs may become a reality.
Long non-coding RNAs (lncRNAs), transcript molecules exceeding 200 base pairs in length, are recognized as integral components of the regulatory framework governing intricate biological processes in the human body, including cellular invasion and migration [Citation9]. Subsequent to extensive research, it has come to light that lncRNAs are also influential in reproductive processes. For instance, Mi et al. reported aberrant upregulation of LncRNA HZ05 in tissues from recurrent miscarriage cases, demonstrating that overexpression of LncRNA HZ05 can impede the proliferation of trophoblast cells, resulting in functional impairment and recurrent miscarriages [Citation10]. Huang et al. revealed that lnc-SLC4A1-1 contributes to trophoblast cell apoptosis, engages the transcription factor NF-κB, thereby exacerbating the inflammatory response in the trophoblastic layer, and culminates in recurrent miscarriages [Citation11]. Nevertheless, the expression and regulatory mechanisms of LncRNA-KCNQ1OT1, a member of the lncRNA family, remain unexplored in the context of URSA.
MicroRNAs (miRNAs), small non-coding molecules approximately 22 nucleotides in length, are pervasive players in processes related to cellular development, differentiation, proliferation, apoptosis, gene regulation, and the onset and progression of tumors [Citation12]. Current research has begun to unveil the regulatory roles of miRNAs in the pathogenesis of URSA. Notably, miR-29a-3p, extensively investigated in oncology, is known for its downregulation in various malignancies and its role as a tumor suppressor gene, capable of hindering tumor cell proliferation, migration, and invasion [Citation13,Citation14]. Prior studies have indicated elevated expression levels of miR-29a-3p in the chorionic villi and decidua of women experiencing recurrent miscarriages, potentially linking it to the phenomenon of recurrent spontaneous abortion [Citation15]. However, a dearth of contemporary research exists concerning the precise regulatory mechanisms of miR-29a-3p in the context of recurrent miscarriages.
Suppressor of Cytokine Signaling 3 (SOCS3) represents one of the principal negative regulators involved in cytokine signal transduction and significantly influences cellular immunity modulation [Citation16]. Studies have indicated substantial SOCS3 expression in the decidua of mice at a gestational age of 9.5–10.5 days, suggesting its pivotal role in regulating immunity at the maternal-fetal interface [Citation17].
In the present study, we explored the expression of the LncRNA-KCNQ1OT1/miR-29a-3p/SOCS3 molecular axis within abortive tissues, with the objective of unraveling the biological connections between this molecular axis and the occurrence of URSA. Concurrently, through the cultivation of normal human villous trophoblast cells during early pregnancy, we assessed the regulatory mechanisms of the LncRNA-KCNQ1OT1/miR-29a-3p/SOCS3 molecular axis in the context of URSA. These study provide a novel theoretical framework for understanding the pathogenesis of URSA.
2. Materials and methods
2.1. Study participants
We selected a group of 15 patients who experienced URSA and were admitted to our hospital between August 2021 and January 2022. These patients had ages ranging from 20 to 34 years, with an average age of 26.94 ± 4.08 years, and their gestational ages varied between 54 and 68 days. They presented clinical symptoms such as vaginal bleeding or abdominal pain. Ultrasound examinations revealed the absence of primary cardiac activity, leading to a clinical diagnosis of inevitable abortion.
In the same time frame, we also chose a control group consisting of healthy early pregnant women who required artificial abortion. These women were aged between 20 and 34 years, with an average age of 27.34 ± 4.69 years, and their gestational ages ranged from 49 to 67 days. We collected chorionic villi tissues from all patients after performing dilation and curettage. Inclusion Criteria: Female patients ranging in age from 20 to 34. Previous instances of spontaneous abortion within 13 weeks, except for biochemical pregnancy, with a history of two or more successive miscarriages with the same partner. Both peripheral blood karyotype and miscarriage chromosomes displayed normalcy. Consistent maintenance of regular menstrual cycles lasting 21–37 days. Absence of medication use during pregnancy. The body mass index (BMI) of the women fell within the range of 18–25 kg/m2.
2.1.1. Exclusion criteria
Chromosomal abnormalities in the karyotypes of peripheral blood and miscarriage chromosomes for both partners. The presence of related endocrine disorders (such as adrenal diseases, diabetes, polycystic ovary syndrome, etc.). Identification of severe infectious factors in previous miscarriages. Autoimmune diseases. Patients with exposure to adverse lifestyles or environmental factors. Multiple pregnancies. Patients who missed abortion and received pre-treatment for abortion (such as mifepristone). Reproductive abnormalities detected during gynecological examinations, gynecological ultrasounds, and hysteroscopy (such as cervical adhesion, intrauterine adhesion, cervical insufficiency, uterine malformations, uterine fibroids, adenomyosis, chocolate cysts, etc.).
2.2. Cell culture and treatment
The HTR8/SVneo cell line originates from early human placental villous trophoblast cells (HTR/SVneo cells have ready- made cell lines that can be used, and the trophoblast cells obtained by D&C are more difficult and limited to obtain that way). These cells were maintained in DMEM medium, supplemented with 10% fetal bovine serum (FBS) and 105 U/L of streptomycin, and incubated at 37 °C in a 5% CO2 incubator. The medium was refreshed every 12–24 hours as needed based on cell growth. When the cell confluence reached 70–80%, they were passaged with 0.25% Trypsin-EDTA solution, sub-cultured every 2–3 days, and preserved by freezing in liquid nitrogen.
Cultured HTR8/SVneo cells were organized into two main groups. In Group 1, we had the following subgroups: a control (blank) group, an Si-NC group (serving as a negative control), and an Si-KCNQ1OT1 group (targeting KCNQ1OT1 for silencing). Group 2 consisted of subgroups, including a negative control mimic group (NC Mimic), a miR-29a-3p mimic group (for overexpressing miR-29a-3p), an NC Vector group (for silencing miR-29a-3p), a SOCS3 Vector group (for overexpressing SOCS3), and a miR-29a-3p Mimic + SOCS3 Vector group. The steps for cell transfection were as follows: (1) Cells were initially seeded in 50 mL culture flasks and allowed to grow in a complete culture medium until they reached 50–60% confluence. (2) Lipofectamine 2000 (purchased from GIBCO) and the desired RNA or DNA for transfection were prepared as follows: a mixture of 5 µL lipofectamine 2000 and 100 µL serum-free culture medium was prepared and placed in a sterile EP tube at room temperature for 5 minutes. (3) RNA (50 nmoL) or DNA (2 µg) was combined with 100 µL serum-free culture medium and left at room temperature for 20 minutes to allow the formation of complexes with liposomes. (4) Cells in the culture flask were washed with serum-free culture medium, and the prepared complex was added to serum-free culture medium (without antibiotics). The mixture was gently mixed and then added to the 10 mL culture flask for transfection. (5) Cells were incubated in a 37 °C incubator with 5% CO2. After 6–8 hours, the solution was replaced with complete culture medium.
2.2.1. qRT-PCR
Total RNA were extracted from both tissue samples and logarithmic cells (b1802, Harbin Beiguo Haiji Biotechnology Co., Ltd., China), followed by assessment of the concentration and purity of the RNA. A reverse transcription reaction using a PCR amplification instrument was performed, as per the instructions of the reverse transcription kit (TransGen Biotech), to create cDNA templates. Shanghai Saint Work designed and synthesized primers for LncRNA-KCNQ1OT1, miR-29a-3p, SOCS, Bax, Bcl-2, Caspase3, caspase9, U6, and GAPDH. We set up a 20 µL reverse transcription experimental system following the guidelines of easyscript first-strand cDNA synthesis Supermix (AE301-02, TransGen Biotech, Beijing, China). Mixed reagent (5 µL), total RNA (5 µL), random primers (1 µL), and RNase-free H2O (9 µL) were added to the EP tubes. These tubes were then centrifuged and thoroughly mixed before being placed in a PCR instrument (Model 9700, Dingguo Changsheng Biotechnology) for reverse transcription following these reaction conditions: 37 °C for 15 minutes; 85 °C for 5 seconds, and termination at 4 °C. The synthesized cDNA was stored at −20 °C. Subsequently, the cDNA was utilized for Real-Time Quantitative PCR, with reference to SYBR® Fluorescent Quantification PCR and the pre-mixed ex taqtm II reagent kit (Takara, Dalian, China). The 20 µL reaction system comprised 10 µL of SYBR pre-mixture, 2 µL of cDNA template, 0.6 µL of upstream and downstream primers, and 6.8 µL of sterile water. The experiment was conducted using the ABI 7500 Fluorescent Quantification PCR system from USA, with GAPDH and U6 as internal parameters. The reaction conditions involved pre-denaturation at 95 °C for 30 seconds, denaturation at 95 °C for 30 seconds, annealing for 20 seconds, extension at 72 °C for 30 seconds, and 45 cycles. This allowed the detection of the relative expressions of LncRNA-KCNQ1OT1, miR-29a-3p, SOCS, Bax, Bcl-2, Caspase3, and caspase9.
2.2.2. Western blotting
Proteins were extracted from actively growing tissues and cells during the logarithmic phase. Following tissue or cell lysis, the resulting mixture was centrifuged at 14,000 g for 10 minutes to separate the supernatant. The protein concentrations were determined using the BCA assay and then stored at −20 °C for future use.
Subsequently, we prepared a 10% separating gel and a 5% stacking gel using the SDS-PAGE Gel kit. After separating the proteins through polyacrylamide gel electrophoresis, they were transferred onto an NC membrane using the wet spinning method and subsequently sealed at room temperature with 5% BSA for 1 hour.
Primary antibodies, including anti-rabbit monoclonal antibodies SOCS (ab280884, Abcam, Cambridge, MA, USA) and GAPDH (ab181602, Abcam, Cambridge, MA, USA), were diluted and applied, incubating overnight at 4 °C. The following day, the membrane underwent three washes with PBST, each lasting 10 minutes. The secondary antibody (ab7312, Abcam, Cambridge, MA, USA) was diluted with 5% skim milk, applied to the membrane, and incubated at room temperature on a shaker for 1 hour. The membrane was again washed three times with PBST, each time for 15 minutes.
A developer solution was added, and the IPP7 Biological Gel Imaging System (MG8600, Morgan Biotechnology Co., Ltd., China Ltd.) was used for the development process. For quantitative analysis, Starbase (Media Cybernetics, Singapore) was employed.
2.2.3. CCK-8
Cells were placed in a 96-well plate and left to incubate at 37 °C with 5% CO2 overnight. Following this incubation period, the initial culture medium was removed, and cells from different experimental groups were transfected. They were then allowed to continue incubating for 48 hours. Subsequently, the culture medium was replaced with 100 µL of medium containing 10% CCK8 solution, and the cells were incubated again at 37 °C with 5% CO2 for an additional 3 hours. The absorbance at OD450 was measured using a spectrophotometer to determine cell proliferation activity.
2.2.4. Flow cytometry
Annexin V-FITC/PI double staining was employed to assess cell apoptosis. At 48 hours post-transfection, cells were harvested, and their concentration was adjusted to 1 × 106 cells/mL. Subsequently, a 70% ethanol solution (precooled) was used to fix the cells, and they were left overnight at 4 °C. Following two PBS washes, a 100 µL aliquot of cell suspension (containing no less than 106 cells/mL) was taken. These cells were washed again with PBS and then centrifuged. The cells were then resuspended in 200 µL of binding buffer, and 10 µL of Annexin V-FITC and 5 µL of PI were added. After gently mixing, the cells were shielded from light and allowed to incubate at room temperature for 15 minutes. Subsequently, 300 µL of binding buffer was added, and cell apoptosis was analyzed using flow cytometry (Tunin NXT, Thermo Fisher, USA).
2.2.5. RNA fluorescence in situ hybridization (FISH)
The KCNQ1OT1 expression in HTR8/SVneo Cells was analyzed through in situ hybridization using a FISH Kit (c10910, Ribobio, Guangzhou). To perform this, cell climbing slices were positioned in the bottom of a 24-well plate, and HTR8/SVneo Cells in the logarithmic growth phase were digested and added to the climbing slices (approximately 6 × 104 cells/well). The cell confluence was maintained at 60–70% before the experiment.
The cells were first rinsed with 1 × PBS for 5 minutes and subsequently fixed with 4% paraformaldehyde at room temperature for 10 minutes. After fixation, the cells were washed with 1 × PBS for 5 minutes, and this step was repeated three times. To prepare for hybridization, 1 mL of pre-cooled osmotic solution was added to each well and allowed to stand for 5 minutes at 4 °C. Following this, the osmotic solution was removed, and the cells were washed with 1 × PBS for 5 minutes, and this step was repeated three times.
Next, 200 µL of pre-hybridization solution was added to each well and sealed at 37 °C for 30 minutes. In a dark environment, 2.5 µL of FISH Probe mixed storage solution was combined with the hybridization solution. The pre-hybridization solution in each cell well was removed, and an appropriate amount of hybridization solution containing the KCNQ1OT1 probe was added. Hybridization was conducted overnight at 37 °C.
To reduce background signals, cells in each well were washed three times with Wash Solution I, each time for 5 minutes. They were then washed once with Wash Solution II and once with Wash Solution III. Subsequently, the cells were washed at room temperature with 1 × PBS for 5 minutes, followed by staining with DAPI Staining Solution for 10 minutes. Under dark conditions, the cell climbing slices were carefully detached from the wells, affixed onto cover slips using a sealing agent and subjected to fluorescence detection. The specificity probe for KCNQ1OT1 was custom-synthesized by Ribobio.
2.2.6. RNA pull-down expression
The HTR8/SVneo Cells were transfected with 50 nm biotinylated wt-bio-miR-29a-3p and mut-miR-29a-3p. After 48 hours, the cells were harvested and rinsed with PBS. Subsequently, the cells were immersed in a specific lysis buffer (Ambion, Austin, Texas, USA) for a duration of 10 minutes. The lysates were mixed with M-280 streptavidin magnetic beads (s3762, Sigma, USA), which had been pre-coated with BSA and yeast tRNA to prevent RNase activity (Trnabak ro, Sigma). These beads were left to incubate at 4 °C for 3 hours, followed by two washes with pre-chilled lysis buffer, three washes with low-salt buffer, and a final wash with high-salt buffer. RNA that had adhered to the beads was purified using Trizol, and subsequently, the expression of KCNQ1OT1 and SOCS3 was assessed using qPCR.
2.2.7. RNA immunoprecipitation
Cells were disrupted in a lysis buffer that contained rnasin (Takara) and a mixture of protease inhibitors (b14001a, Roche, USA). This mixture consisted of 25 mM Tris-HCl at pH 7.4, 150 mM NaCl, 0.5% NP-40, 2 mM EDTA, 1 mM NaF, and 0.5 mM dithiothreitol. The lysates were then subjected to centrifugation at 12,000 g for 30 minutes, and the resulting supernatant was harvested. Subsequently, the supernatant was combined with anti-Go-2 magnetic beads (BMFA-1, Biomark Technologies, China), while the control group received anti-IgG magnetic beads. Following a 4-hour incubation at 4 °C, the beads were thoroughly washed three times with a wash buffer (composed of 50 mM Tris-HCl, 300 mM NaCl at pH 7.4, 1 mM MgCl2, and 0.1% NP-40). RNA was then extracted from the magnetic beads using Trizol, and the expression levels of KCNQ1OT1 and SOCS3 were determined through qRT-PCR.
2.2.8. Dual-luciferase assay
We obtained fragments that contain the binding sites for miR-29a-3p and SOCS3. The full-length 3’UTR region of SOCS3 were cloned and amplified into the pmirGLO Luciferase Vector (E1330, Promega, USA), and named as SOCS3-Wt. Additionally, the SOCS3 Mut Vector was created through site-directed mutagenesis. The internal reference used was the PRL TK Vector, which expresses Renilla luciferase (e2241, Promega, USA). Next, the miR-29a-3p mimic and the NC mimic were separately co-transfected with the luciferase reporter vector into HTR8/SVneo cells (CRL-1415, ATCC, USA). Fluorescence intensity was measured using a fluorometer (Glomax20/20, Promega, USA).
3. Statistical analysis
SPSS version 26.0 software was used to analyze the data. Measurement data were expressed as mean ± standard deviation (x ± SD), which was consistent with the homogeneity of variance of normal distribution. All data in this study were compared between groups using F-test and LSD method. The distribution of the data was examined, and p < 0.05 was considered statistically significant.
4. Results
4.1. Differential expression of LncRNA-KCNQ1OT1, miR-29a-3p, and SOCS3 in URSA
Abortion tissues were gathered from both women who experienced URSA and healthy early pregnant women. The levels of LncRNA-KCNQ1OT1, miR-29a-3p, and SOCS3 mRNA were measured using qRT-PCR. When compared to the control group, the URSA group exhibited a notable increase in miR-29a-3p expression and a significant decrease in the expression of KCNQ1OT1 and SOCS3 mRNA in the abortion tissues (as depicted in ). To determine the levels of SOCS3 protein in the tissues, western blot analysis was performed. It was observed that the abortion tissues of the URSA group had a considerable reduction in the expression of SOCS3 protein when compared to the control group (as illustrated in ).
Figure 1. The levels of LncRNA-KCNQ1OT1, miR-29a-3p, SOCS3 mRNA, and SOCS3 protein expression in the abortion tissues of women with URSA. Panel A denotes the expression of KCNQ1OT1, Panel B shows the expression of miR-29a-3p, Panel C displays the expression of SOCS3 mRNA, and Panel D represents the expression of SOCS3 protein. *Compared with the control group, Wilcoxon rank-sum test, p < 0.05.
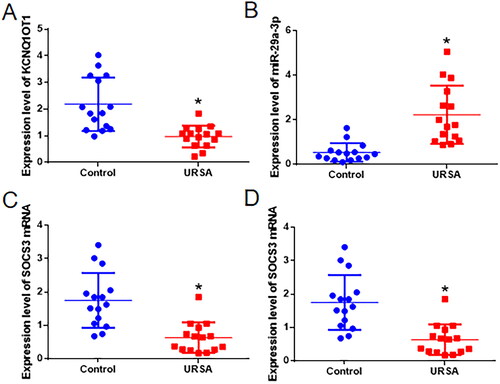
4.2. The influence of KCNQ1OT1 expression on human villous trophoblast cells
In the cellular groups where KCNQ1OT1 expression was suppressed, qRT-PCR analysis revealed a noteworthy reduction in the expression of KCNQ1OT1 in the si-KCNQ1OT1 group as compared to the Si-NC group (). Cell proliferation and apoptosis were assessed using the CCK-8 Assay () and flow cytometry (). The results demonstrated that, in contrast to the Si-NC group, the Si-KCNQ1OT1 group exhibited a significant decrease in cell proliferation and a marked increase in cell apoptosis. Furthermore, qRT-PCR was employed to measure the expression of apoptosis-related genes, including Bax, Bcl-2, caspase-3, and caspase-9. The Si-KCNQ1OT1 group displayed a significant increase in Bcl-2 expression and a substantial decrease in the expressions of Bax, Bcl-2, Caspase3, and caspase9 when compared to the Si-NC group ().
Figure 2. Influence of inhibiting KCNQ1OT1 on the growth and programmed cell death of HTR8/SVneo cells. In A, KCNQ1OT1 expression is measured using qRT-PCR. In B, the CCK-8 assay is employed to evaluate cell growth in both sets of cells. C depicts the evaluation of cell apoptosis via flow cytometry, while D depicts the determination of cell apoptosis-related markers through qRT-PCR.* Compared with the Si-NC group, p < 0.05.
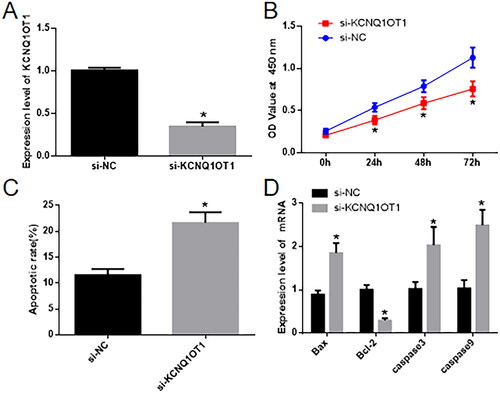
4.3. KCNQ1OT1 promotes SOCS3 expression by adsorbing miR-29a-3p
The FISH findings revealed that KCNQ1OT1 is expressed in both the nucleus and cytoplasm, and was mainly localized in the cytoplasm (depicted in ). In comparison to the NC group, miR-29a-3p inhibited luciferase activity in the SOCS3 promoter binding region, while the mutated promoter was unaffected. This suggests that miR-29a-3p can target and suppress SOCS3 expression (as illustrated in ). The RNA pull-down assay demonstrated a significant increase in binding between KCNQ1OT1 and the SOCS3 promoter by wt-miR-29a-3p, as opposed to mut-miR-29a-3p. This points to a direct interaction between miR-29a-3p and SOCS3 (as seen in ). These results imply that KCNQ1OT1 may be involved in regulating SOCS3 by competitively binding with miR-29a-3p. In the RNA-IP test results, it was observed that the binding of KCNQ1OT1 and miR-29a-3p to anti-AGO2 significantly increased when compared to anti-IgG. This suggests a direct binding between miR-29a-3p and KCNQ1UT1 ().
Figure 3. KCNQ1OT1 enhances SOCS3 expression by acting as a sponge to absorb miR-29a-3p. (A) Displays the outcomes of RNA FISH, revealing the presence of KCNQ1OT1 in both the cellular cytoplasm and nucleus. (B) Displays the statistical assessment of the dual-luciferase reporter assay, while (C) displays the statistical analysis of the RNA pull-down assay. (D) Displays the statistical analysis of the RNA-IP experiment.*Compared with the NC mimic group, p < 0.05; #compared with Mut-miR-29a-3p, p < 0.05; & compared with anti-IgG, p < 0.05.
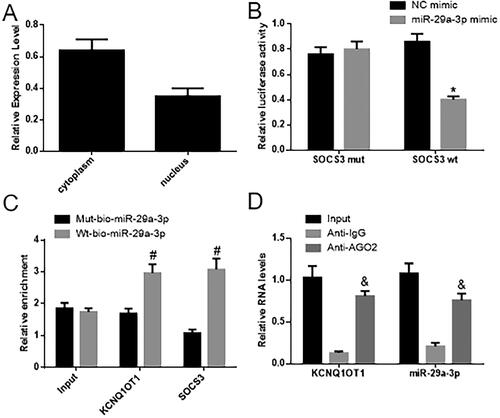
4.4. MiR-29a-3p inhibits the proliferation of human chorionic villi membrane trophoblast cells by inhibiting the expression of SOCS3
In the cell groups where the expression of miR-29a-3p was manipulated, the qRT-PCR and western blot results revealed notable differences. Specifically, in the miR-29a-3p mimic group, the expression of miR-29a-3p was significantly higher than in the NC mimic group. Additionally, the expression of SOCS3 mRNA and protein was markedly lower in the miR-29a-3p mimic group compared to the NC mimic group. In contrast, when comparing the SOCS3 Vector group to the NC Vector group, the expression of miR-29a-3p did not show a significant change, but the levels of SOCS3 mRNA and protein notably increased. Furthermore, in the miR-29a-3p-mimic + SOCS3 Vector group, there was no significant alteration in miR-29a-3p expression compared to the miR-29a-3p mimic group, but the expression of SOCS3 mRNA and protein significantly increased (as shown in ).
Figure 4. miR-29a-3p hinders the growth of human villous trophoblast cells and encourages their programmed cell death by suppressing the activity of SOCS3. (A) Displays the measurement of miR-29a-3p and SOCS3 expression using qRT-PCR. (B) Displays the utilization of a CCK-8 assay to assess cell proliferation. (C) Displays the application of flow cytometry to observe cell apoptosis. (D) Displays the evaluation of cell apoptosis-related markers through qRT-PCR. Tukey HSD* Compared with the NC mimic group, p < 0.05; #compared with NC vector, p < 0.05; &compared with the miR-29a-3p mimic, n = 3, p < 0.05.
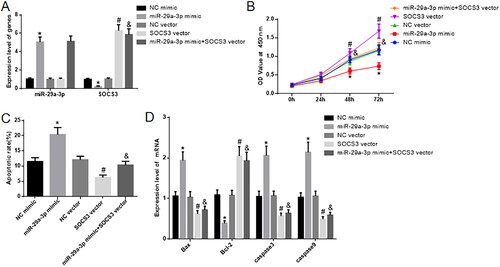
To assess cell proliferation and apoptosis, the CCK-8 assay () and flow cytometry () were employed. When comparing the miR-29a-3p mimic group to the NC mimic group, there was a substantial decrease in cell proliferation, and the apoptosis ratio significantly increased. Conversely, when comparing the SOCS3 Vector group to the NC Vector group, there was a significant increase in cell proliferation and a decrease in the cell apoptosis ratio.
Compared to the miR-29a-3p-mimic group, the miR-29a-3p-mimic + SOCS3 Vector group exhibited a marked increase in cell proliferation and a reduction in the cell apoptosis ratio. We used qRT-PCR to analyze the expression of apoptosis-related genes, namely Bax, Bcl-2, Caspase3, and Caspase9 (). When compared to the NC mimic group, the miR-29a-3p mimic group showed a significant increase in Bcl-2 expression and a notable decrease in the expression of Bax, Bcl-2, Caspase3, and Caspase9. On the other hand, in comparison to the NC Vector group, the SOCS3 Vector group exhibited a significant decrease in Bcl-2 expression and a substantial increase in the expression of Bax, Bcl-2, Caspase3, and Caspase9. Notably, when compared with the miR-29a-3p-mimic group, the miR-29a-3p-mimic + SOCS3 Vector group displayed a significant decrease in Bcl-2 expression and a considerable increase in the expression of Bax, Bcl-2, Caspase3, and Caspase9. Additional data are provided in the Supplementary Materials.
5. Discussion
The successful development and maintenance of a typical pregnancy are closely tied to the proper functioning of the placenta. The key factor in placental function is the healthy growth of the trophoblastic layer within the placenta. In the early stages of a normal pregnancy, the villous trophoblast layer is highly active and robust in its growth and division. The villous trophoblast cells rapidly multiply, divide, and mature. As the pregnancy progresses, they shift toward apoptosis, especially in the later stages of pregnancy [Citation18,Citation19]. This delicate equilibrium involves the careful regulation of cell apoptosis and proliferation in pregnancy trophoblast cells.
The development of a typical pregnancy placenta involves the differentiation of embryonic trophoblast cells, decidual invasion, vascular remodeling, and tissue restructuring, among other processes. Apoptosis plays a role in this dynamic, contributing to the controlled elimination of cells in the nourishing layer [Citation20]. When this balance is disrupted, and cell apoptosis becomes dominant, it can affect placental function negatively, potentially leading to irregular embryo and fetal development and adverse pregnancy outcomes. Studies on chorionic villi and placentas at different stages of pregnancy reveal that trophoblast cells are actively proliferating in early pregnancy but shift towards apoptosis as the pregnancy progresses. Cell proliferation decreases while apoptosis increases with gestational age [Citation21,Citation22].
In cases of recurrent miscarriage, there are abnormalities in the expression of genes related to apoptosis. The pro-apoptotic gene Bax shows a significant increase in protein expression, while the anti-apoptotic Bcl-2 protein significantly decreases. This shift towards apoptosis predominates and contributes to recurrent miscarriages [Citation23]. The level of apoptosis in the decidua during recurrent miscarriages is notably higher than in normal pregnancies. Apoptosis increases the number of apoptotic cells through the caspase-dependent signaling pathway, leading to recurrent miscarriages [Citation24]. Moreover, an increase in apoptosis of endometrial cells is also associated with recurrent miscarriages, and both pro-apoptotic and anti-apoptotic genes are involved in the process [Citation25].
The results of this study revealed that LncRNA-KCNQ1OT1 is present in the miscarriage tissue of normal healthy early pregnant women, but its expression is notably low in the miscarriage tissue of women with URSA. Upon silencing KCNQ1OT1, the growth of HTR8/SVneo cells was significantly hampered, and the rate of apoptosis considerably increased. These findings indicate that KCNQ1OT1 has the potential to enhance the proliferation of HTR8/SVneo cells while impeding cell apoptosis. Multiple studies have validated the role of lncRNA as a competitive endogenous RNA, where they can compete by "sponging" common miRNAs through shared miRNA response elements (MREs), thereby mitigating the influence of miRNAs on the mRNA of target genes [Citation26]. Furthermore, it was demonstrated that KCNQ1OT1 can serve as a sponge for absorbing miR-29a-3p.
MiR-29a-3p, a member of the miRNA family, has been extensively investigated in the field of oncology [Citation27,Citation28]. Dong et al. [Citation29] conducted a microarray analysis on chorionic villi and decidua from both normal terminated pregnancies and recurrent miscarriages, revealing significant increases in the expression of miR-184, miR-187, and miR-125b-2p, while miR-520f, miR-3175, and miR-4672 showed significant decreases. These genes are associated with processes like adhesion, apoptosis, and angiogenesis, suggesting that miRNA-regulated target genes may play a role in the molecular mechanism of recurrent miscarriages. Recent research has shown elevated miR-29a-3p expression in chorionic villi and decidua of patients with RM and NP [Citation30]. However, the precise regulatory mechanism of miR-29a-3p in this context remains unclear.
The findings demonstrate a significant increase in miR-29a-3p expression in women with URSA. Overexpressing miR-29a-3p led to decreased trophoblast cell proliferation and an increased rate of apoptosis, suggesting that abnormal miR-29a-3p expression might contribute to the development of URSA. KCNQ1OT1 could potentially act as a competing endogenous RNA (ceRNA) and influence the regulation of miR-29a-3p in URSA.
Mature miRNAs execute their functions in mammalian cells by binding to the 3’ non-transcribed regions of target gene mRNAs, resulting in the inhibition of mRNA translation or direct degradation. This process, in turn, silences genes and modulates gene expression at the post-transcriptional level [Citation15]. Previous studies have identified a binding site between miR-29a-3p and SOCS3, a regulator of proliferation and immunity. Our research has further revealed that miR-29a-3p can target and suppress SOCS3 expression. Simultaneously, the overexpression of SOCS3 can mitigate the inhibitory effects of miR-29a-3p overexpression on trophoblast cell proliferation and promote cell apoptosis.
6. Conclusion
In summary, this research uncovers that miR-29a-3p expression is elevated in abortion tissues of women with URSA, while the levels of LncRNA-KCNQ1OT1 and SOCS3 are notably diminished. MiR-29a-3p can hinder the expression of SOCS3, suppress trophoblast cell proliferation, and facilitate cell apoptosis. Through the competing endogenous RNA (ceRNA) mechanism, LncRNA-KCNQ1OT1 can act as a sponge for miR-29a-3p, thereby increasing SOCS3 expression, promoting trophoblast cell proliferation, and inhibiting cell atrophy and death. Our discoveries offer a fresh theoretical foundation for understanding the development of URSA.
This study has various strengths and limitations. Firstly, its strengths lie in the in-depth investigation of molecular mechanisms, revealing the interactions among LncRNA-KCNQ1OT1, miR-29a-3p, and SOCS3, and their roles in recurrent miscarriage. This contributes to a better understanding of the potential HTR/svr mechanisms of spontaneous abortion and provides new insights for prevention and treatment. The study involving the role of non-coding RNAs suggests that non-coding RNAs may play a more significant role in human physiology and diseases, offering new directions for future research. Additionally, this study integrates clinical and basic scientific research, particularly in the fields of molecular biology, genetics, and gynecology, facilitating a comprehensive understanding of the complexity of recurrent spontaneous abortion and promoting collaboration and communication across different disciplines.
However, this study also has some limitations: the relatively small number of cases of recurrent miscarriage may limit the sample size of the study, thereby affecting the reliability and generalizability of the results; given the involvement of complex molecular mechanisms and interactions among multiple gene expressions, experimental design may face challenges that require careful planning and control to ensure result accuracy; although the study has revealed the role of molecular axes, their prospects in clinical application remain uncertain. Further research is needed to validate whether these findings can be translated into effective therapeutic methods or diagnostic tools.
Ethical approval
The study was conducted in accordance with the Declaration of Helsinki. The study was approved by Ethics Committee of the Affiliated Hospital of Guizhou Medical University (No. 2021-236). Written informed consent was obtained from all participants.
Supplemental Material
Download MS Word (415.6 KB)Acknowledgements
We are particularly grateful to all the people who have given us help on our article.
Disclosure statement
No potential conflict of interest was reported by the author(s).
Data availability statement
All data generated or analyzed during this study are included in this article. Further enquiries can be directed to the corresponding author.
Additional information
Funding
References
- Practice Committee of the American Society for Reproductive Medicine. Electronic address: [email protected]. Definitions of infertility and recurrent pregnancy loss: a committee opinion. Fertil Steril. 2020;113(3):1–13. doi: 10.1016/j.fertnstert.2019.11.025.
- Bender AR, Christiansen OB, Elson J, et al. ESHRE guideline: recurrent pregnancy loss. Hum Reprod Open. 2018;2018:y4. doi: 10.1093/hropen/hoy004.
- Di Simone N, Castellani R, Caliandro D, Caruso A. Monoclonal anti-annexin V antibody inhibits trophoblast gonadotropin secretion and induces syncytiotrophoblast apoptosis. Biol Reprod. 2001;65(6):1766–1770. doi: 10.1095/biolreprod65.6.1766.
- Veglia M, D’Ippolito S, Marana R, et al. Human IgG antinuclear antibodies induce pregnancy loss in mice by increasing immune complex deposition in placental tissue: in vivo study. Am J Reprod Immunol. 2015;74(6):542–552. doi: 10.1111/aji.12429.
- Di Simone N, Castellani R, Raschi E, et al. Anti-beta-2 glycoprotein I antibodies affect Bcl-2 and Bax trophoblast expression without evidence of apoptosis. Ann N Y Acad Sci. 2006;1069:364–376. doi: 10.1196/annals.1351.034.
- Di Simone N, Caliandro D, Castellani R, et al. Interleukin-3 and human trophoblast: in vitro explanations for the effect of interleukin in patients with antiphospholipid antibody syndrome. Fertil Steril. 2000;73(6):1194–200. doi: 10.1016/s0015-0282(00)00533-1.
- Zhang X, Wang H, Feng T, et al. The relationship between semen factors and unexplained recurrent spontaneous abortion. Clin Chim Acta. 2020;510:605–612. doi: 10.1016/j.cca.2020.08.022.
- Li YY, Chen SW, Zhao F, et al. Association of arsenic with unexplained recurrent spontaneous abortion: a case-control study. Zhonghua Yu Fang Yi Xue Za Zhi. 2019;53(5):470–474.
- Zhao L, Li J, Huang S. Patients with unexplained recurrent spontaneous abortion show decreased levels of microRNA-146a-5p in the Deciduae. Ann Clin Lab Sci. 2018;48(2):177–182.
- Mi C, Chen W, Liang T, et al. Lnc-HZ05 regulates BPDE-inhibited human trophoblast cell proliferation and affects the occurrence of miscarriage by directly binding with miR-hz05. Cell Biol Toxicol. 2022;38(6):1137–1157. doi: 10.1007/s10565-021-09687-w.
- Huang Z, Du G, Huang X, et al. The enhancer RNA lnc-SLC4A1-1 epigenetically regulates unexplained recurrent pregnancy loss (URPL) by activating CXCL8 and NF-kB pathway. EBioMedicine. 2018;38:162–170. doi: 10.1016/j.ebiom.2018.11.015.
- Tang M, Bai L, Wan Z, et al. circRNA-DURSA regulates trophoblast apoptosis via miR-760-HIST1H2BE axis in unexplained recurrent spontaneous abortion. Mol Ther Nucleic Acids. 2021;26:1433–1445. doi: 10.1016/j.omtn.2021.06.012.
- Qin W, Tang Y, Yang N, et al. Potential role of circulating microRNAs as a biomarker for unexplained recurrent spontaneous abortion. Fertil Steril. 2016;105(5):1247–1254.e3. doi: 10.1016/j.fertnstert.2016.01.028.
- Li D, Li J, Jia B, et al. Genome-wide identification of microRNAs in decidual natural killer cells from patients with unexplained recurrent spontaneous abortion. Am J Reprod Immunol. 2018;80(5):e13052.
- Yang Q, Gu WW, Gu Y, et al. Association of the peripheral blood levels of circulating microRNAs with both recurrent miscarriage and the outcomes of embryo transfer in an in vitro fertilization process. J Transl Med. 2018;16(1):186. doi: 10.1186/s12967-018-1556-x.
- Ying X, Jin X, Zhu Y,et al. Exosomes released from decidual macrophages deliver miR-153-3p, which inhibits trophoblastic biological behavior in unexplained recurrent spontaneous abortion. Int Immunopharmacol. 2020;88:106981. doi: 10.1016/j.intimp.2020.106981.
- Chen H, Cheng S, Liu C, et al. Bioinformatics analysis of differentially expressed genes, methylated genes, and miRNAs in unexplained recurrent spontaneous abortion. J Comput Biol. 2019;26(12):1418–1426. doi: 10.1089/cmb.2019.0158.
- Liu S, Gu X, Weng R. Clinical effect of lymphocyte immunotherapy on patients with unexplained recurrent spontaneous abortion. Immun Inflamm Dis. 2021;9(4):1272–1278. doi: 10.1002/iid3.474.
- Dean DD, Agarwal S, Muthuswamy S. Defining the role of FMR1 gene in unexplained recurrent spontaneous abortion. J Assist Reprod Genet. 2019;36(11):2245–2250. doi: 10.1007/s10815-019-01591-x.
- Somigliana E, Viganò P, Vignali M. Endometriosis and unexplained recurrent spontaneous abortion: pathological states resulting from aberrant modulation of natural killer cell function? Hum Reprod Update. 1999;5(1):40–51. doi: 10.1093/humupd/5.1.40.
- Fan T, Zhong XM, Wei XC, et al. The alteration and potential relationship of vaginal microbiota and chemokines for unexplained recurrent spontaneous abortion. Medicine. 2020;99(51):e23558. doi: 10.1097/MD.0000000000023558.
- Fainboim L, Belén S, González V, et al. Evaluation of paternal lymphocyte immunotherapy and potential biomarker mixed lymphocyte reaction-blocking factor in an Argentinian cohort of women with unexplained recurrent spontaneous abortion and unexplained infertility. Am J Reprod Immunol. 2021;86(2):e13422.
- Zhu L, Liu M, Zhang S, et al. Foxp3 TSDR hypermethylation is correlated with decreased Tregs in patients with unexplained recurrent spontaneous abortion. Reprod Sci. 2021;28(2):470–478. doi: 10.1007/s43032-020-00299-z.
- Zhu D, Zou H, Liu J, et al. Inhibition of HMGB1 ameliorates the maternal-fetal interface destruction in unexplained recurrent spontaneous abortion by suppressing pyroptosis activation. Front Immunol. 2021;12:782792. doi: 10.3389/fimmu.2021.782792.
- Zhang J, Liu X, Gao Y. Abnormal H3K27 histone methylation of RASA1 gene leads to unexplained recurrent spontaneous abortion by regulating Ras-MAPK pathway in trophoblast cells. Mol Biol Rep. 2021;48(6):5109–5119. doi: 10.1007/s11033-021-06507-6.
- Wang XQ, Li Y, Su X, et al. Haplotype-based association of two SNPs in miR-323b with unexplained recurrent spontaneous abortion in a Chinese Han population. J Cell Physiol. 2018;233(8):6001–6017. doi: 10.1002/jcp.26415.
- Li D, Li J. Association of miR-34a-3p/5p, miR-141-3p/5p, and miR-24 in decidual natural killer cells with unexplained recurrent spontaneous abortion. Med Sci Monit. 2016;22:922–929. doi: 10.12659/msm.895459.
- Xiao B, Xue X, Hu F, et al. Expression and regulatory mechanism of microRNA-155 in the villi of patients with unexplained recurrent spontaneous abortion patients. Zhonghua Fu Chan Ke Za Zhi. 2014;49(2):130–134.
- Dong F, Zhang Y, Xia F, et al. Genome-wide miRNA profiling of villus and decidua of recurrent spontaneous abortion patients. Reproduction. 2014;148(1):33–41. doi: 10.1530/REP-14-0095.
- Wang XQ, Wang H, Zhang L, et al. Haplotype-based association of two SNPs in miR-423 with unexplained recurrent pregnancy loss in a Chinese Han population. Exp Cell Res. 2019;374(1):210–220. doi: 10.1016/j.yexcr.2018.11.026.