Abstract
Background: The fetal stage is pivotal for growth and development, making it susceptible to the adverse effects of prenatal metal(loid)s exposure. This study evaluated the influence of gestational diabetes mellitus (GDM) on the placental transfer efficiency (PTE) of metal(loid)s and thus assessed the associated risks of prenatal metal(loid)s exposure.
Materials and method: Designed as a case-control study, it incorporated 114 pregnant participants: 65 without complications and 49 diagnosed with GDM. We utilized inductively coupled plasma mass spectrometry to quantify seven metal(loid)s - manganese (Mn), cobalt (Co), nickel (Ni), copper (Cu), gallium (Ga), arsenic (As), and cadmium (Cd) - in both maternal venous blood and umbilical cord blood.
Result: We compared metal(loid)s concentrations and their PTE in the maternal and cord blood between the two groups. Notably, Cu, Ga, As, and Co levels in the umbilical cord blood of the GDM group (657.9 ± 167.2 μg/L, 1.23 ± 0.34 μg/L, 5.19 ± 2.58 μg/L, 1.09 ± 2.03 μg/L) surpassed those of the control group, with PTE of Co showing a marked increase in GDM group (568.8 ± 150.4 μg/L, 1.05 ± 0.31 μg/L, 4.09 ± 2.54 μg/L, 0.47 ± 0.91 μg/L), with PTE of Co showing a marked increase in GDM group (p < 0.05). The PTE of Ni exhibited a reduction in the GDM group relative to the control group, yet this decrease did not reach statistical significance.
Conclusion: This study indicates that GDM can influence the placental transfer efficiency of certain metal(loid)s, leading to higher concentrations of Co, Cu, Ga, and As in the umbilical cord blood of the GDM group. The marked increase in the PTE of Co suggests a potential link to placental abnormal angiogenesis due to GDM.
1. Introduction
Heavy metal exposure during pregnancy has been associated with negative outcomes, including preterm birth and low birth weight [Citation1,Citation2]. Throughout gestation, metal(loid)s in the maternal blood are consistently transferred to the fetus. However, commonly, only the maternal metal(loid)s exposure levels are accessible for assessment [Citation3]. Placental transfer efficiency (PTE) refers to the efficiency of metal(loid)s transport between the mother and fetus through the placenta, typically represented by the ratio of metal(loid)s concentrations in umbilical cord blood to those in maternal blood. Current research suggests varying PTE for different metal(loid)s. For instance, metal(loid)s like mercury appear to accumulate more on the fetal side [Citation4]. Due to the heightened fetal sensitivity to metal(loid)s, even minuscule amounts can be detrimental to fetal health, even when these concentrations are deemed safe for mothers [Citation5]. Thus, understanding the PTE of metal(loid)s and the factors influencing them is essential in gauging fetal health risks.
Several factors modulate the metal(loid)s transfer between mother and fetus. Research suggests that biological sex can influence the PTE of certain metal(loid)s; for example, titanium and silver demonstrate higher PTE in male compared to female fetuses [Citation6]. Metal(loid)s interactions also play a role in their absorption and transfer. Notably, iron absorption and transfer have been found to be closely linked with copper levels [Citation7]. Additionally, for pregnant women with specific complications, metal(loid)s transfer efficiency might be altered. Such complications can cause a decrease in the expression of metallothionein (MT), which reduces Cd-MT complexes in the placenta. This weakens the placental barrier, potentially elevating the risk of cadmium (Cd) transfer and subsequent fetal exposure [Citation8]. Gestational diabetes mellitus (GDM) is a prevalent complication during pregnancy. It is defined as the onset of diabetes during the mid to late stages of pregnancy in women who did not have a previously diagnosed diabetes condition [Citation9]. Individuals diagnosed with GDM often present immune system abnormalities and experience chronic inflammatory responses [Citation10]. Furthermore, the elevated levels of blood glucose and insulin can adversely affect the placenta, prompting abnormal angiogenesis and potentially weakening the placental barrier function [Citation11–13].
The prenatal exposure to varying levels of metal(loid)s can induce distinct effects on fetal health. For instance, Cd exposure during pregnancy has been linked to negative implications for fetal neurodevelopment [Citation14]. A deficiency in cobalt can alter oxidative stress levels, which is believed to correlate with preterm births [Citation15]. Essential trace elements, such as copper (Cu) and manganese (Mn), play pivotal roles in fostering normal fetal growth and development. A deficit in these elements has been associated with negative pregnancy outcomes, including low birth weight and miscarriages [Citation16,Citation17]. Arsenic (As) exposure during pregnancy can lead to genetic anomalies in newborns and may predispose them to diseases, including cancer, later in life [Citation18].
Past studies focusing on trace elements and heavy metal concentrations in maternal and fetal blood have predominantly centered on their quantification and potential correlations with birth outcomes. There has been limited investigation into the determinants influencing the PTE of metal(loid)s. In our current research, we analyzed 114 pairs of maternal and umbilical cord blood samples sourced from Changsha, China. This sample set encompassed 65 samples from women with normal pregnancies and 49 from those diagnosed with GDM. Our core objective was to discern the distribution and transfer efficiency of seven distinct elements—manganese (Mn), cobalt (Co), nickel (Ni), copper (Cu), gallium (Ga), arsenic (As), and cadmium (Cd)—within the maternal-fetal bloodstream. Additionally, we aimed to probe the association between the PTE of these elements and the presence of gestational diabetes mellitus.
2. Materials and methods
2.1. Recruitment and sample collection
Between August and October 2021, 300 pregnant women were recruited from the Hunan Province Maternal and Child Health Hospital. Women registered in the hospital were invited to participate after being informed of the objectives and methods of the study. The trial protocol was approved by the research ethics committees of all participating institutions, and informed consent was obtained from all participating subjects. The length and birth weight of the newborns were measured after delivery. The venous blood of the pregnant women was collected before delivery, and the cord blood was collected after delivery. Blood samples were stored in uncontaminated lithium heparin anticoagulant tubes. A portion of the whole blood samples were centrifuged at 3000 rpm for 5 min to separate plasma and blood cells. After plasma separation, blood cell samples were resuspended to their original volume with an equal volume of phosphate-buffered saline (PBS), and all samples were stored at −80 °C until analysis. Routine hematological parameters of maternal blood were determined before delivery using an Automated Hematology Analyzer (Sysmex XN9000), and lipid profiles were measured during late pregnancy examinations. Participants had resided locally for at least three years, had no other diseases or history of heavy metal exposure, and all were single-pregnancy with vaginal delivery. The diagnosis of GDM was as follows: fasting plasma glucose (FPG) screening at 24–28 weeks of pregnancy, with FPG ≥ 5.1 mmol/L diagnosed as GDM. If FPG < 5.1 mmol/L, a 75 g oral glucose tolerance test (OGTT) was performed. Pregnant women with blood glucose levels exceeding 5.1 mmol/L fasting, 10.0 mmol/L at 1 h, and 8.5 mmol/L at 2 h after glucose ingestion were diagnosed with GDM. The study was designed as a case-control study, and samples were screened according to the complications of the pregnant women. The study was divided into GDM group and healthy pregnant women group. There was a total of 65 pairs in the control group and 49 pairs in the GDM group.
2.2. Chemicals and reagents
Multi-element standard solution (Agilent), mixed internal standard solution (Agilent). HNO3 and H2O2 were purchased from Merck (Darmstadt, Germany). All chemicals used were of analytical reagent grade. Ultrapure water (18.2 MΩCM; Millpore, USA) was used throughout the experiment.
2.3. Sample processing and metal(loid)s element determination
0.2 ml of blood cell samples were mixed with 0.2 ml HNO3 and 0.06 ml H2O2, and heated on an electric heating plate at 95 °C for 2 h until the solution clarified. After digestion, the solution was brought to 10 ml with deionized water. Subsequently, Mn, Co, Ni, Cu, Ga, As, and Cd were analyzed by Inductively Coupled Plasma Mass Spectrometry (ICP-MS, Agilent 8800; CA, USA). Multi-element standard solutions were used for the calibration and verification of the standard curve. Germanium (Ge), indium (In), and rhenium (Re) were used as internal references for calibrating the ICP-MS system. A standard sample was added for quality control every 30 samples. The limit of detection (LOD) for Mn, Co, Ni, Cu, Ga, As, Cd were 0.21, 0.04, 0.13, 1.15, 2.2 × 10−3, 8.0 × 10−3, and 2.9 × 10−3 μg/L, respectively, with limit of quantitation (LOQ) of 0.72, 0.12, 0.43, 3.83, 7.4 × 10−3, 2.7 × 10−2, 3.9 × 10−2, 9.7 × 10−3 μg/L. The recovery rates for the spiked samples of each element ranged between 88.6 and 107.8%, with relative standard deviations (RSDs) less than 5%.
2.4. Statistical analysis
Statistical analyses were performed using IBM SPSS Statistics 20 software (IBM, NY, USA). The distribution of heavy metal(loid)s was described by arithmetic mean (AM), median, and quartile. Data above the limit of detection (LOD) were considered quantifiable, and metal(loid)s concentrations below the LOD were replaced by the LOD divided by 2. The placental transfer efficiency of metal(loid)s is defined as the percentage ratio of metal(loid)s concentration in umbilical cord blood to that in maternal blood. Spearman rank correlation test was used to describe the correlation between each continuous variable. The comparison between the GDM group and the normal group was made using the Mann-Whitney U test. p < 0.05 was considered statistically significant.
3. Results
A total of 114 pairs of pregnant women were included in this study, with 49 in the GDM group and 65 in the control group. As shown in , there were significant differences between the two groups in terms of age, gestational age, white blood cells count (WBC), neutrophils count (NEUT) and monocytes count (MONO). The age in the GDM group were higher than those in the control group, while gestational age, WBC, NEUT, and MONO count were lower than those in the control group. The levels of heavy metal(loid)s in maternal and cord blood are shown in and . The levels of Cu, Ga, and As in the maternal blood of the GDM group were higher than those in the control group. In cord blood, the levels of Co, Cu, Ga, and As in the GDM group were higher. The relationship between the metal(loid)s levels in the blood of pregnant women and the parameters of the mother is shown in Tables S1 and S2. In the GDM group, the level of Co was negatively correlated with the number of red blood cell count (RBC) and eosinophils count (EOS) (r = −0.427, p < 0.05) (r = −0.357, p < 0.05), and positively correlated with the mean corpuscular hemoglobin concentration (MCHC) (r = 0.492, p < 0.005). The level of Ni was positively correlated with the age of the pregnant women (r = 0.457, p < 0.05), and the level of As was negatively correlated with the mother’s systolic blood pressure (SBP)and diastolic blood pressure (DBP) (r = −0.424, p < 0.005) (r = −0.388, p < 0.01). In the control group, the level of Mn was negatively correlated with the mean corpuscular volume (MCV) and mean corpuscular hemoglobin (MCH) (r = −0.290, p < 0.05) (r = −0.337, p < 0.01), the level of Ni was negatively correlated with plasma triglyceride concentration (TG), MCHC, and basophils count (BASO) (r = −0.429, p < 0.01) (r = −0.309, p < 0.05) (r = −0.326, p < 0.05), the level of Cu was positively correlated with TG and platelet (PLT) (r = 0.348, p < 0.01) (r = 0.368, p < 0.005), the level of Ga was positively correlated with TG and PLT (r = 0.287, p < 0.05) (r = 0.321, p < 0.01), the level of As was positively correlated with lymphocyte count (LY) and PLT (r = 0.315, p < 0.05) (r = 0.335, p < 0.01).
Table 1. Comparison of clinical, routine blood, and lipid parameters between GDM and control groups.
Table 2. Distribution of metal(loid)s concentration (μg/L) in maternal blood.
Table 3. Distribution of metal(loid)s concentration (μg/L) in cord blood.
The PTE of different metal(loid)s between mother and fetus is shown in . In the GDM group, the percentages of PTE for Mn, Co, Ni, Cu, Ga, As, and Cd were 231%, 531%, 143%, 106%, 101%, 91%, and 14%, respectively. In contrast, the PTE values in the control group were 213%, 175%, 113%, 111%, 104%, 99%, and 11% for the same metal(loid)s, respectively. Compared to the control group, the PTE of Co in the GDM group is higher. There were no significant differences in the PTE of other elements. As shown in Figure S1, there is a correlation between the PTE of different elements, the PTE of Cu and Ga are positively correlated with Mn (r = 0.197, p < 0.05) (r = 0.418, p < 0.001), the PTE of Cu and Ga are positively correlated (r = 0.368, p < 0.001) and the PTE of As, Cd, and Ni are positively correlated (r = 0.216, p < 0.05) (r = 0.199, p < 0.05).
Figure 1. Distribution of metal(loid)s placental transfer efficiency (%) in control and GDM group (G GDM group, N control group).
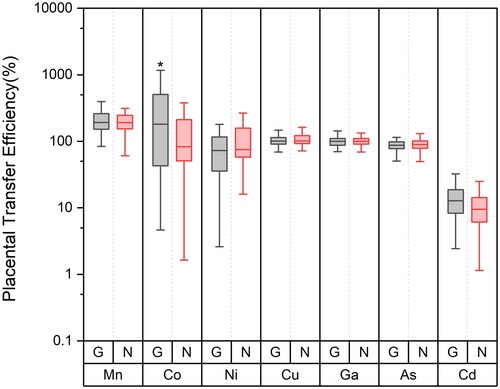
The relationship between metal(loid)s PTE and maternal parameters is shown in and . In the GDM group, the PTE of Co is positively correlated with the mother’s perinatal body mass index (BMI) (r = 0.471, p < 0.01), the PTE of Ni is negatively correlated with the mother’s age (r = −0.495, p < 0.05), and positively correlated with the mother’s hemoglobin (HGB), WBC, NEUT, and MONO (r = 0.427, p < 0.05) (r = 0.737, p < 0.001) (r = 0.57, p < 0.01) (r = 0.44, p < 0.05), the PTE of Cu is negatively correlated with the mother’s SBP (r = −0.327, p < 0.05), the PTE of Ga is negatively correlated with EOS (r = −0.338, p < 0.05), the PTE of Cd is negatively correlated with TG and BASO (r = −0.414, p < 0.01) (r = −0.321, p < 0.05). In the control group, the PTE of Mn is negatively correlated with the mother’s perinatal BMI (r = −0.262, p < 0.05), the PTE of Cu is negatively correlated with the mother’s perinatal BMI and TG (r = −0.311, p < 0.05) (r = −0.374, p < 0.005), the PTE of Ga is negatively correlated with the mother’s perinatal BMI and TG (r = −0.28, p < 0.05) (r = −0.393, p < 0.001), the PTE of Cd is negatively correlated with LY (r = −0.327, p < 0.05).
Table 4. Spearman correlation between parameters and metal(loid)s placental transport efficiency in pregnant women with GDM.
Table 5. Spearman correlation between parameters and metal(loid)s placental transport efficiency in normal pregnant women.
4. Discussion
The aim of this study is to unveil the correlations between the PTE of metal(loid)s and GDM. Our findings indicate that the PTE of Mn and Co exceed 200%, suggesting that these metal(loid)s are readily transferred to the fetal side and tend to accumulate there. Ni, Cu, Ga, and As have transfer efficiencies around 100%, indicating their capability to cross the placental barrier and reach the fetus. Cd, with a transfer efficiency of only 13%, demonstrates its difficulty in penetrating the placental barrier. Above all, a significantly higher PTE of Co in the GDM group compared to the control group, while no similar trends were observed for other metal(loid)s. This suggests that the increased PTE of Co in the GDM cohort may represent a specific phenomenon, likely unrelated to general changes in placental permeability, and more associated with abnormalities in placental angiogenesis. Cobalt is linked to oxidative stress and inflammatory responses and plays a role in antioxidant defense; its concentration increment may be beneficial within a certain normal range for enhancing placental blood supply [Citation19]. Co2+ facilitates the synthesis of erythropoietin, activating the differentiation of erythrocytes and the hematopoietic process [Citation20]. Cobalt in the bloodstream irreversibly enters erythrocytes and binds to hemoglobin [Citation21]. Although insulin cannot directly cross the placenta, it can activate receptors on the surface of the trophoblast cells, triggering pathways essential for endothelial cell survival and angiogenesis [Citation22]. Under the GDM condition, these pathways may lead to anomalies in angiogenesis [Citation23]. Additionally, the diabetic state of the mother may affect the development and function of placental vasculature, thereby influencing placental permeability and endothelial cell selectivity [Citation24]. Insulin may also alter placental permeability by regulating the expression of aquaporins (AQPs) [Citation25]. Our data show that the umbilical cord blood Co concentration in GDM pregnant women is significantly higher than in normal pregnancies, which could be linked to the aberrant insulin levels in GDM women affecting placental receptor activation and fetal-side angiogenesis, leading to the fetal accumulation of Co. Regarding the increased Co transfer efficiency in GDM patients, we speculate that the altered biological availability of Co may result from the abnormal milieu of GDM.
Our research results indicate that in healthy pregnant women, blood Cu levels are positively correlated with plasma TG levels, but this correlation is not observed in GDM patients. Similarly, the PTE of Cu and Ga in healthy pregnant women is negatively correlated with plasma TG levels, a phenomenon not present in GDM patients. This may be attributed to changes in the binding affinity of proteins in the plasma of GDM patients for metal(loid)s such as Cu, thereby affecting the transfer of metal(loid)s to the fetus. It is known that overweight and obesity significantly increase the risk of GDM [Citation26], with perinatal BMI being an indicator of metabolic and nutritional status during pregnancy and adiposity being the main contributor to gestational weight gain [Citation27]. Blood lipid levels are considered to be related to metal(loid)s content in the blood, especially copper and iron [Citation28,Citation29]. A cross-sectional study found that the concentration of copper in umbilical cord serum was positively correlated with triglycerides [Citation30], consistent with our observations. The bioactivity and bioavailability of copper may change in GDM patients [Citation31]. GDM is closely related to abnormalities in the maternal immune system [Citation32], with chronic inflammation playing a significant role in the onset and progression of GDM [Citation33]. Notably, significant changes in blood cell parameters and immune cell counts have been observed in GDM patients [Citation34,Citation35]. Importantly, we found that the transfer efficiency of Ni in the GDM group was positively correlated with hemoglobin concentration, white blood cells, monocytes, and neutrophil counts, while no such relationship was observed in normal pregnancies. Nickel, as a metal(loid)s element in the active center of enzymes, can penetrate the placental barrier to the fetus [Citation36]. Albumin in serum is the primary binding protein for nickel [Citation37] and can rapidly bind to trophoblast cells [Citation38]. The changes in maternal-fetal transfer of Ni in GDM patients may be associated with its interactions with immune cells.
Our study points out that in normal pregnancies, fetal concentrations of Co are typically higher than in the mother, and this phenomenon is even more pronounced in GDM patients. Excessive intake of cobalt is associated with developmental toxic effects, including increased levels of lipid peroxidation in embryos [Citation39]. Exposure to cobalt, such as that observed in laboratory rats exposed to cobalt sulfate, can result in reduced fetal weight and delayed skeletal development, as well as increased risk of embryonic death [Citation40]. Furthermore, studies have found that elevated concentrations of cobalt in maternal and placental tissues are significantly correlated with an increased risk of coronary heart disease in offspring [Citation41]. Even though our results show that, in populations with GDM, the cobalt concentrations in umbilical cord blood are comparable to levels reported in other studies [Citation42], the increased transfer efficiency of cobalt in individuals with high cobalt concentrations and concurrent GDM should not be overlooked. This finding underscores the need to pay closer attention to the potential implications of elevated cobalt transfer in the context of GDM. Therefore, we advise that GDM patients should be particularly cautious to avoid excessive cobalt accumulation on the fetal side, to prevent potential adverse effects on the fetus, even at levels of Co concentration considered safe within the mother. Metal(loid)s isotope exposure is a commonly used method to investigate the transfer of metal(loid)s between mother and offspring [Citation43]. Mouse models of GDM and normal pregnancy can be separately constructed, exposing them to stable isotopes of metal(loid)s during pregnancy. By measuring the metal(loid)s concentrations and isotope ratios in the blood or tissues of the offspring and mothers from both groups, the impact of GDM on the efficiency of placental transfer of these metal(loid)s can be determined. This experimental approach can also be applied to validate other pregnancy complications that are easily modeled. Due to experimental constraints, this study did not conduct related animal experiments. Further evidence and mechanistic investigations into changes in the PTE of cobalt and other metal(loid)s in GDM patients may benefit from considering this approach.
5. Conclusion
This study has illuminated significant differences in the levels of Co, Cu, Ga, and As in the umbilical cord blood of the GDM group compared to the control group, with a marked elevation in the transfer efficiency of Co. In GDM patients, we observed correlations between the transfer efficiency of specific metal(loid)s and maternal blood lipid concentrations, as well as immune cell counts. Our findings provide a comprehensive overview of the determinants influencing the PTE of metal(loid)s. Importantly, the study underscores the potential risks posed by elevated Co levels in the fetuses of GDM patients, even at concentrations deemed safe for the expectant mother. Overall, our results pave the way for a deeper comprehension of the dynamics of maternal-infant metal(loid) transfer and highlight the need for further research to elucidate the underlying mechanisms of this phenomenon.
Supplemental Material
Download MS Word (73.1 KB)Disclosure statement
No potential conflict of interest was reported by the author(s).
Data availability statement
The authors confirm that the data supporting the findings of this study are available within the article and its supplementary materials.
Additional information
Funding
References
- Ashrap P, Watkins DJ, Mukherjee B, et al. Maternal blood metal and metalloid concentrations in association with birth outcomes in Northern Puerto Rico. Environ Int. 2020;138:1. doi: 10.1016/j.envint.2020.105606.
- Tian L-L, Zhao Y-C, Wang X-C, et al. Effects of gestational cadmium exposure on pregnancy outcome and development in the offspring at age 4.5 years. Biol Trace Elem Res. 2009;132(1–3):51–10. doi: 10.1007/s12011-009-8391-0.
- Okubo H, Nakayama SF. Periconceptional maternal diet quality influences blood heavy metal concentrations and their effect on low birth weight: the Japan environment and children’s study. Environ Int. 2023;173:107808. doi: 10.1016/j.envint.2023.107808.
- Li A, Zhuang T, Shi J, et al. Heavy metals in maternal and cord blood in Beijing and their efficiency of placental transfer. J Environ Sci. 2019;80:99–106. doi: 10.1016/j.jes.2018.11.004.
- Wells PG, Lee CJJ, McCallum GP, et al. Receptor- and reactive intermediate-mediated mechanisms of teratogenesis. Handb Exp Pharmacol. 2010;196:131–162. doi: 10.1007/978-3-642-00663-0_6.
- Li X, Li A, Zhang W, et al. A pilot study of mothers and infants reveals fetal sex differences in the placental transfer efficiency of heavy metals. Ecotoxicol Environ Saf. 2019;186:109755. doi: 10.1016/j.ecoenv.2019.109755.
- McArdle HJ, Andersen HS, Jones H, et al. Copper and iron transport across the placenta: regulation and interactions. J Neuroendocrinol. 2008;20(4):427–431. doi: 10.1111/j.1365-2826.2008.01658.x.
- Dong X, Ding A, Hu H, et al. Placental barrier on cadmium transfer from mother to fetus in related to pregnancy complications. Int J Women’s Health. 2023;15:179–190. doi: 10.2147/ijwh.S393067.
- American Diabetes Association. Classification and diagnosis of diabetes: standards of medical care in diabetes-2020. Diabetes Care. 2020;43:S14–S31. doi: 10.2337/dc20-S002.
- Pendeloski KPT, Mattar R, Torloni MR, et al. Immunoregulatory molecules in patients with gestational diabetes mellitus. Endocrine. 2015;50(1):99–109. doi: 10.1007/s12020-015-0567-0.
- Jadhav A, Khaire A, Gundu S, et al. Placental neurotrophin levels in gestational diabetes mellitus. Int J Dev Neurosci. 2021;81(4):352–363. doi: 10.1002/jdn.10107.
- Lei J, Zhao M, Li L, et al. Research progress of placental vascular pathophysiological changes in pregnancy-induced hypertension and gestational diabetes mellitus. Front Physiol. 2022;13:954636. doi: 10.3389/fphys.2022.954636.
- Sobrevia L, Valero P, Grismaldo A, et al. Mitochondrial dysfunction in the fetoplacental unit in gestational diabetes mellitus. Biochim Biophys Acta Mol Basis Dis. 2020;1866(12):165948. doi: 10.1016/j.bbadis.2020.165948.
- Wang Y, Chen L, Gao Y, et al. Effects of prenatal exposure to cadmium on neurodevelopment of infants in Shandong, China. Environ Pollut. 2016;211:67–73. doi: 10.1016/j.envpol.2015.12.038.
- Li Z-J, Liang C-M, Xia X, et al. Association between maternal and umbilical cord serum cobalt concentration during pregnancy and the risk of preterm birth: the Ma’anshan birth cohort (MABC) study. Chemosphere. 2019c;218:487–492. doi: 10.1016/j.chemosphere.2018.11.122.
- Atazadegan MA, Heidari-Beni M, Riahi R, et al. Association of selenium, zinc and copper concentrations during pregnancy with birth weight: a systematic review and meta-analysis. J Trace Elem Med Biol. 2022;69:126903. doi: 10.1016/j.jtemb.2021.126903.
- Wood RJ. Manganese and birth outcome. Nutr Rev. 2009;67(7):416–420. doi: 10.1111/j.1753-4887.2009.00214.x.
- Navasumrit P, Chaisatra K, Promvijit J, et al. Exposure to arsenic in utero is associated with various types of DNA damage and micronuclei in newborns: a birth cohort study. Environ Health. 2019;18(1):51. doi: 10.1186/s12940-019-0481-7.
- Liang C, Wang J, Xia X, et al. Serum cobalt status during pregnancy and the risks of pregnancy-induced hypertension syndrome: a prospective birth cohort study. J Trace Elem Med Biol. 2018;46:39–45. doi: 10.1016/j.jtemb.2017.11.009.
- Simonsen LO, Harbak H, Bennekou P. Cobalt metabolism and toxicology—a brief update. Sci Total Environ. 2012;432:210–215. doi: 10.1016/j.scitotenv.2012.06.009.
- Simonsen LO, Brown AM, Harbak H, et al. Cobalt uptake and binding in human red blood cells. Blood Cells Mol Dis. 2011;46(4):266–276. doi: 10.1016/j.bcmd.2011.02.009.
- Desoye G, Hofmann HH, Weiss PA. Insulin binding to trophoblast plasma membranes and placental glycogen content in well-controlled gestational diabetic women treated with diet or insulin, in well-controlled overt diabetic patients and in healthy control subjects. Diabetologia. 1992;35(1):45–55. doi: 10.1007/BF00400851.
- Alwhaibi A, Verma A, Adil MS, et al. The unconventional role of Akt1 in the advanced cancers and in diabetes-promoted carcinogenesis. Pharmacol Res. 2019;145:104270. doi: 10.1016/j.phrs.2019.104270.
- Leach L, Taylor A, Sciota F. Vascular dysfunction in the diabetic placenta: causes and consequences. J Anat. 2009;215(1):69–76. doi: 10.1111/j.1469-7580.2009.01098.x.
- Damiano AE. Review: water channel proteins in the human placenta and fetal membranes. Placenta. 2011;32(Suppl 2):S207–S211. doi: 10.1016/j.placenta.2010.12.012.
- Alwash SM, McIntyre HD, Mamun A. The association of general obesity, Central obesity and visceral body fat with the risk of gestational diabetes mellitus: evidence from a systematic review and meta-analysis. Obes Res Clin Pract. 2021;15(5):425–430. doi: 10.1016/j.orcp.2021.07.005.
- Lederman SA, Paxton A, Heymsfield SB, et al. Body fat and water changes during pregnancy in women with different body weight and weight gain. Obstet Gynecol. 1997;90(4):483–488. doi: 10.1016/s0029-7844(97)00355-4.
- Pavão ML, Figueiredo T, Santos V, et al. Whole blood glutathione peroxidase and erythrocyte superoxide dismutase activities, serum trace elements (Se, Cu, Zn) and cardiovascular risk factors in subjects from the city of Ponta Delgada, Island of San Miguel, The Azores Archipelago, Portugal. Biomarkers. 2006;11(5):460–471. doi: 10.1080/13547500600625828.
- Zhou J, Liu C, Francis M, et al. The causal effects of blood iron and copper on lipid metabolism diseases: evidence from phenome-wide Mendelian randomization study. Nutrients. 2020;12(10):3174. doi: 10.3390/nu12103174.
- Wells EM, Navas-Acien A, Apelberg BJ, et al. Association of selenium and copper with lipids in umbilical cord blood. J Dev Origins Health Dis. 2014;5(4):281–287. doi: 10.1017/s2040174414000233.
- Valero P, Fuentes G, Cornejo M, et al. Exposome and foetoplacental vascular dysfunction in gestational diabetes mellitus. Mol Aspects Med. 2022;87 doi: 10.1016/j.mam.2021.:.101019.
- Sheu A, Chan Y, Ferguson A, et al. A proinflammatory CD4+ T cell phenotype in gestational diabetes mellitus. Diabetologia. 2018;61(7):1633–1643. doi: 10.1007/s00125-018-4615-1.
- Fashami MA, Hajian S, Afrakhteh M, et al. Is there an association between platelet and blood inflammatory indices and the risk of gestational diabetes mellitus? Obstet Gynecol Sci. 2020;63(2):133–140. doi: 10.5468/ogs.2020.63.2.133.
- Sun T, Meng F, Zhao H, et al. Elevated First-Trimester neutrophil count is closely associated with the development of maternal gestational diabetes mellitus and adverse pregnancy outcomes. Diabetes. 2020;69(7):1401–1410. doi: 10.2337/db19-0976.
- Yang H, Zhu C, Ma Q, et al. Variations of blood cells in prediction of gestational diabetes mellitus. J Perinat Med. 2015;43(1):89–93. doi: 10.1515/jpm-2014-0007.
- Iyengar G, Rapp A. Human placenta as a ‘dual’ biomarker for monitoring fetal and maternal environment with special reference to potentially toxic trace elements. Part 3: toxic trace elements in placenta and placenta as a biomarker for these elements. Sci Total Environ. 2001;280(1–3):221–238. doi: 10.1016/s0048-9697(01)00827-0.
- Laurie SH, Pratt DE. A spectroscopie study of nickel(II)-bovine serum albumin binding and reactivity. J Inorg Biochem. 1986;28(4):431–439. doi: 10.1016/0162-0134(86)80028-9.
- Mas A, Yeger H, Sarkar B. Nickel binding by isolated human trophoblast cells. Res Commun Chem Pathol Pharmacol. 1990;67(2):271–278.
- Cai G, Zhu J, Shen C, et al. The effects of cobalt on the development, oxidative stress, and apoptosis in zebrafish embryos. Biol Trace Elem Res. 2012;150(1–3):200–207. doi: 10.1007/s12011-012-9506-6.
- Szakmáry E, Ungváry G, Hudák A, et al. Effects of cobalt sulfate on prenatal development of mice, rats, and rabbits, and on early postnatal development of rats. J Toxicol Environ Health A. 2001;62(5):367–386. doi: 10.1080/152873901300018110.
- Zhang N, Yang S, Yang J, et al. Association between metal cobalt exposure and the risk of congenital heart defect occurrence in offspring: a multi-hospital case-control study. Environ Health Prev Med. 2020;25(1):38. doi: 10.1186/s12199-020-00877-2.
- Cottrell JN, Thomas DS, Mitchell BL, et al. Rural and urban differences in prenatal exposure to essential and toxic elements. J Toxicol Environ Health A. 2018;81(23):1214–1223. doi: 10.1080/15287394.2018.1547890.
- Rinderknecht AL, Kleinman MT, Ericson JE. Pb enamel biomarker: deposition of pre- and postnatal Pb isotope injection in reconstructed time points along rat enamel transect. Environ Res. 2005;99(2):169–176. doi: 10.1016/j.envres.2005.01.005.