ABSTRACT
Introduction
Chronic Obstructive Pulmonary Disease (COPD) and cardiovascular diseases (CVD) commonly co-exist. Outcomes of people living with both conditions are poor in terms of symptom burden, receiving evidence-based treatment and mortality. Increased understanding of the underlying mechanisms may help to identify treatments to relieve this disease burden. This narrative review covers the overlap of COPD and CVD with a focus on clinical presentation, mechanisms, and interventions. Literature up to December 2023 are cited.
Areas Covered
1. What is COPD 2. The co-existence of COPD and cardiovascular disease 3. Mechanisms of cardiovascular disease in COPD. 4. Populations with COPD are at risk of CVD 5. Complexity in the co-diagnosis of COPD in those with cardiovascular disease. 6. Therapy for COPD and implications for cardiovascular events and risk. 7. Cardiovascular risk and exacerbations of COPD. 8. Pro-active identification and management of CV risk in COPD.
Expert Opinion
The prospective identification of co-morbid COPD in CVD patients and of CVD and CV risk in people with COPD is crucial for optimizing clinical outcomes. This includes the identification of novel treatment targets and the design of clinical trials specifically designed to reduce the cardiovascular burden and mortality associated with COPD. Databases searched: Pubmed, 2006–2023.
1. What is COPD?
The Global Initiative for Chronic Obstructive Lung Disease (GOLD) defines COPD as ‘a heterogeneous lung condition, characterized by chronic respiratory symptoms (dyspnoea, cough, expectoration and/or exacerbations) due to abnormalities of the airways (bronchitis, bronchiolitis) and/or alveoli (emphysema) that cause persistent, often progressive, airflow obstruction’ [Citation1]. This generally requires a significant long-term respiratory exposure, such as tobacco smoke, in a genetically susceptible individual. Despite this definition including emphysema – a radiological diagnosis – GOLD still specifies that the diagnosis of COPD must be based on spirometry and the demonstration of persistent airway obstruction after bronchodilatation, defined as the ratio between the volume of air exhaled in 1 second (FEV1) to the vital capacity (FVC) being < 0.7. Given that this ratio declines with age, a single cutoff may lead to over-diagnosing COPD in the elderly and other guidelines have recommended using the lower limit of normal for diagnosing airway obstruction [Citation2]. Breathlessness is a cardinal feature of COPD but also of many cardiac diseases, introducing immediate complexity for differential diagnosis and comorbid cardiorespiratory conditions that we discuss further below [Citation3].
There are limitations to the aforementioned approach to diagnosing COPD [Citation4]. Even in healthy individuals, trajectories of pulmonary function differ greatly over the lifespan. FEV1 typically peaks at age 25 years for males and 18 for females, followed by a plateau phase ahead of a gradual decline through physiological aging [Citation5]. People with COPD usually (but not always) demonstrate a more rapid decline in pulmonary function [Citation5]. As spirometry is relatively insensitive in the early stages of COPD, and a diagnosis of COPD is often made later in the disease trajectory, efforts are ongoing to support earlier diagnosis with the opportunity for earlier intervention. The term pre-COPD has been proposed to describe people at risk of developing COPD, defined as occurring in people with risk factors (such as smoking) and symptoms (sputum production, cough), with or without other abnormalities on CT or lung function but without airflow obstruction [Citation6]. The term PRISm (Preserved Ratio Impaired Spirometry) is used to describe patients with a reduced FEV1 and a preserved FEV1/FVC ratio, some of whom will progress to COPD [Citation7]. This heterogeneous group, previously referred to as having ‘restrictive’ spirometry, does not meet the diagnosis of COPD but does not have normal spirometry. People with PRISm have been largely excluded from therapeutic trials [Citation8]. A large population-based study showed that patients meeting the PRISm criteria are at significant risk of mortality, especially due to cardiovascular disease [Citation9], making this group of patients a further target for more detailed study and early intervention.
In those that meet the diagnostic criteria for COPD, GOLD advises that the severity of airflow obstruction is determined by examining the FEV1 from mild (>80%) to very severe (<30%). As there is a complex relationship between airflow obstruction and clinical impact, the burden of COPD is also defined in terms of symptoms (using questionnaires such as Modified Medical Research Council Scale (mMRC) and/or the COPD Assessment Test (CAT) and exacerbation risk, with patients at first diagnosis divided into three groups, termed A (low symptoms, low exacerbation risk), B (high symptoms but low exacerbation risk) and E (high exacerbation risk irrespective of symptoms) [Citation10]. This classification can be used to guide both initial non-pharmacological and pharmacological therapies in COPD to reduce symptoms and exacerbation risk.
2. The co-existence of COPD and cardiovascular disease: prevalence and challenges
COPD is the most common chronic respiratory condition, affecting around 10% of the global population [Citation11]. Many people living with COPD are diagnosed late or remain undiagnosed -especially in low- and middle-income countries (LMIC) [Citation12]. With an aging population and continued exposure to risk factors such as (indoor and outdoor) air pollution and smoking, this number will increase over the coming decades [Citation13]. An increase in the prevalence of COPD means an increase in the subsequent burden of the disease. According to the Global Burden of Disease study, COPD was responsible for 3.3 million deaths in 2019, accounting for 5% of all deaths and COPD is the third leading cause of death in the world [Citation8]. This burden is distributed unevenly, with 90% of COPD-related deaths occurring in LMIC [Citation14]. There are particular problems in LMIC with under-diagnosis [Citation12], the absence of guidelines [Citation15], challenges in guideline implementation [Citation16], and lack of access to effective and affordable interventions [Citation17]. COPD also causes a significant economic burden. In the European Union, the direct cost of COPD-associated healthcare in 2003 was estimated at 38.6 billion Euros, increasing to approximately 48.4 billion Euros in 2011 [Citation18]. Approximately 40–70% of the costs are due to treatment of acute exacerbations [Citation18]. The cost of COPD is estimated to increase to 4.3 trillion dollars globally by 2050 [Citation14].
Studies have shown that the prevalence of cardiovascular disease in patients with COPD is variable, ranging from 20–70% depending on the study population and cardiovascular conditions considered. Additionally, the risk of COPD is elevated in patients with established cardiovascular disease [Citation19]. The rate of major adverse cardiovascular events (MACE) in patients with COPD is 25% higher compared to those without COPD and thus is similar to the excess cardiovascular risk seen in individuals with diabetes [Citation20]. This can only be partially explained by shared risk factors such as aging, smoking and socioeconomic deprivation. Pathophysiological mechanisms including hypoxemia, hyperinflation, oxidative stress, and systemic inflammation likely play a major role [Citation21]. A systematic review by Chen et al. showed that compared to the general population, people living with COPD have an increased risk of cardiovascular disease. (OR 2·46, 95% CI 2·02–3·00) [Citation19,Citation21,Citation22]. This increase in risk persists even when corrected for shared risk factors including aging, smoking, and socioeconomic deprivation [Citation19,Citation23]. Many epidemiological studies have been performed with selected patient groups, typically those presenting in secondary care with advanced disease, making it likely that the actual prevalence of CVD in mild COPD is underestimated [Citation23]. This is of importance since cardiovascular mortality is highest in mild to moderate COPD [Citation9].
2.1. COPD and ischemic heart disease
Ischemic heart disease (IHD) is the most frequent cardiac comorbidity in people with COPD. Conversely, the prevalence of COPD ranges between 7 and 28% in patients presenting with an acute myocardial infarction. (24). Reciprocally, the relative risk of myocardial infarction was shown to be 1.4 times higher in people living with COPD compared to those without COPD. Patients with severe COPD are at an even higher risk of IHD, with a relative risk of 3.00 [Citation24]. CVD is a leading cause of death in people living with COPD, with up to a third of the deaths being attributable to ischemic heart disease, making it more likely for a person with COPD to die from cardiovascular disease than respiratory failure [Citation25,Citation26].
Despite this, tools to accurately assess cardiovascular risk in COPD, without the need for invasive diagnostics, are generally lacking. The GOLD strategy document recommends using the National Heart and Lung Institute global risk calculator [Citation10]. In 2015 a risk calculator specific for screening ischemic heart disease in COPD was developed. This COPDCoRi algorithm demonstrated an accuracy of 81% (95%CI: 77.78–84.71) in predicting the presence of coronary artery disease in COPD [Citation27]. Interestingly, it seems that (centrilobular) emphysema-phenotype COPD patients have a particularly high rate of coronary calcification, suggesting an important role of emphysema in the development of atherosclerosis, [Citation28] with recent studies suggesting that hyperinflation may be the main driver of this risk [Citation29].
2.2. COPD and heart failure
The prevalence of COPD ranges from 9 to 41% in patients with heart failure in studies from European cohorts [Citation30]. Large trials investigating heart failure with preserved ejection fraction (HFpEF) have often excluded people living with COPD, leading to a lower reported prevalence (11–19%) [Citation31]. The prevalence of heart failure increases in hospitalized COPD patients, rising to 70% in COPD patients on mechanical ventilation [Citation30].
Healthcare use in patients with concomitant COPD and heart failure is high. A retrospective study from Canada showed an annual rate of 12–14 visits, 39% of which were cardiorespiratory [Citation32]. These patients with combined COPD and heart failure are also more prone to hospitalizations and are at an increased risk of rehospitalization [Citation33]. For instance, patients with concomitant HFpEF and COPD have a 1.5-fold increased risk of (re)hospitalization and a 1.42 increased risk of cardiovascular death [Citation31]. Importantly, patients with COPD and concomitant heart failure remain less likely to receive adequate treatment for heart failure, such as with beta-blockers [Citation34].
The diagnosis of heart failure in patients with COPD is challenging [Citation35]. Symptoms of heart failure and COPD can be difficult to differentiate, with exertional dyspnea, nocturnal cough, and paroxysmal nocturnal dyspnea not specific to either. Findings on physical examination may also overlap. Additionally, chest radiography is not reliable in detecting features of heart failure in those patients with COPD with severe hyperinflation, disturbing the cardiothoracic ratio. Signs of pulmonary congestion on chest X-rays may be seen permanently in people with severe COPD [Citation36]. Transthoracic echocardiography windows in COPD can be impaired due to lung hyperinflation [Citation37]. BNP and NT-proBNP levels are normal to mildly elevated in stable patients with COPD but can be useful in excluding heart failure in patients with COPD when present at higher concentrations [Citation37].
2.3. COPD and pulmonary hypertension
Although a degree of pulmonary hypertension (PH) in patients with COPD is not uncommon, severe pulmonary hypertension is rare. A meta-analysis by Zhang et al. found a pooled prevalence of 39% (95% CI: 34–44.4I2 = 97.6%) for COPD-related pulmonary hypertension, with an increasing prevalence in patients with severe COPD [Citation38,Citation39]. The pathophysiology underlying PH associated with lung disease and/or hypoxia is characterized by the proliferation of endothelial and smooth muscle cells with rarefaction of blood vessels [Citation40]. This differs from classic pulmonary arterial hypertension (PAH) [Citation40]. This distinct vasculopathy in COPD has been linked with long-term exposure to tobacco smoke. Severe PH in COPD with a high pulmonary vascular resistance (more than 5 WU) may reflect severe underlying pulmonary vasculopathy [Citation40]. There are currently no PH-specific treatments registered for PH-associated with COPD, although PDE5 inhibitors could be considered in severe PH associated with COPD (PVR >5 WU) [Citation40], with some studies suggesting an improvement in exercise capacity [Citation41].
2.4. COPD and atrial fibrillation
Atrial fibrillation is the most common sustained arrhythmia. The prevalence of atrial fibrillation in patients with COPD is high and estimated to be around 25% [Citation42]. When both diseases are present, patients experience a higher symptom burden, poorer quality of life and worse cardiovascular and bleeding outcomes. Concomitant COPD and AF also increase the number of hospital admissions and are associated with an increase in all-cause mortality[Citation43,Citation44]. All-cause mortality in patients with combined COPD-AF is 50% at 5 years [Citation45] and data suggest a temporal relation such that patients diagnosed with COPD before AF show a worse prognosis than patients found to have COPD after an AF diagnosis [Citation46]. There are no specific treatment guidelines for the management of COPD-AF, and undertreatment of both diseases (inhaled pharmacotherapy and beta-blockers) is often seen, despite studies suggesting no increased risk of adverse events [Citation42].
2.5. COPD-OSA overlap syndrome and cardiovascular disease
Obstructive sleep apnea (OSA) is a prevalent and well-recognized comorbidity in cardiovascular disease. The combined presence of COPD and OSA is referred to as COPD-OSA overlap syndrome [Citation47]. This overlap syndrome is highly prevalent in patients diagnosed with OSA or COPD [Citation47], and the relationship is likely to be bidirectional [Citation48]. Overlap syndrome is more prevalent in moderate to severe COPD compared to milder COPD [Citation48].
The pathophysiology of COPD-OSA overlap syndrome is complex since there are both risk factors and protective factors in COPD that contribute to the co-occurrence. Risk factors for developing OSA in COPD include the use of corticosteroids, pharyngeal muscle weakness, smoking and fluid shifts when sleeping in the supine position [Citation49]. There are also protective factors for OSA in COPD. Upper airway patency is associated with lung volume and the hyperinflation seen in COPD may thus protect against OSA. Indeed, higher indices of air trapping and emphysema severity are associated with a lower apnea-hypopnea index. Hyperinflation, however, is associated with a lower arousal threshold which could contribute to the pathogenesis of overlap syndrome [Citation50]. Furthermore, COPD is associated with increased sleep fragmentation and lower REM sleep, which contributes to poor sleep quality in COPD, yet paradoxically protects from OSA. Other protective factors include older age and lower body mass index [Citation51]. Given this heterogeneity in risk factors, it is likely that different COPD phenotypes may drive OSA [Citation51], with COPD patients with airway-predominant COPD being at a particularly increased risk for developing OSA [Citation52].
Concomitant COPD and OSA have a profound impact on patients. Not only do patients with overlap have worse sleep quality, overlap syndrome is associated with an increased risk of exacerbations of COPD and increased medical costs [Citation53]. A recent meta-analysis showed that COPD-OSA overlap syndrome is also associated with increased cardiovascular risk [Citation47]. Patients with overlap syndrome had an increased risk of hypertension and peripheral arterial disease compared to patients with OSA alone. There are also studies suggesting an increased risk of ischemic heart disease, heart failure and stroke in COPD-OSA overlap.
It is thought that the increased hypoxemic burden seen in the overlap syndrome, compared to either disease alone [Citation54], is the most important contributor to the development of cardiovascular disease. This was recently confirmed using data from the European Sleep Apnea Database, showing that nocturnal hypoxia was strongly associated with hypertension, diabetes, and heart failure [Citation55]. Other contributing factors increasing the risk of cardiovascular disease are systemic inflammation, increased sympathetic nerve activation and endothelial dysfunction [Citation51].
Gender differences further exacerbate this issue. Women with OSA show elevated markers of systemic inflammation and hypoxemic burden, putatively translating to an increased risk of cardiovascular risk observed in women with OSA [Citation56]. Since both COPD and OSA are poorly recognized in women, there are important challenges remaining to COPD-OSA overlap diagnosis in women [Citation56]. Diagnosing COPD-OSA overlap is important since effective treatment may lower mortality and hospitalizations for exacerbations of COPD [Citation48]. Although the optimal type of positive airway pressure therapy (PAP) remains controversial, as does the impact of PAP on cardiovascular outcomes, recent studies suggest that correct treatment with PAP improves cardiovascular outcomes [Citation57]. Evidence of the benefit of chronic noninvasive ventilation is stronger in patients living with COPD and concomitant hypercapnia in terms of reducing mortality and hospitalizations and improving quality of life and symptoms. Furthermore, guidelines also suggest screening such patients for underlying obstructive sleep apnea [Citation58].
3. Mechanisms of cardiovascular disease in COPD ()
3.1. Shared risk factors and systemic inflammation
Figure 1. Mechanism of cardiovascular risk in COPD.
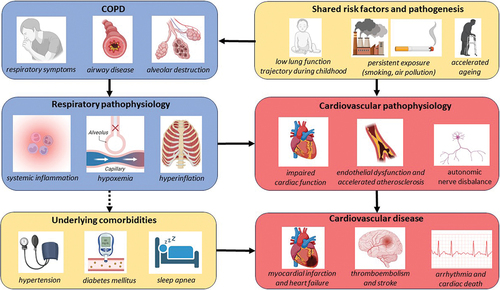
The most important shared risk factor between COPD and cardiovascular disease is tobacco smoking. Smoking is a known and significant risk factor for the development of atherosclerotic disease, including coronary artery disease, and is the major risk factor for the development of COPD [Citation59]. The mechanism by which smoking contributes to cardiovascular disease is not fully understood. It is thought to be a complex cascade of events leading to endothelial dysfunction and damage due to oxidative stress, the development of a hypercoagulative state, altered lipid status with increasing amounts of cholesterol and a hyperinflammatory state [Citation60]. This hyperinflammatory state is also thought to drive the development of COPD. Repeated exposure to irritants, such as cigarette smoke, leads to airway and alveolar epithelial inflammation and subsequent damage to the lung parenchyma in those individuals with a genetic susceptibility. This eventually leads to tissue damage and infiltration of macrophages and neutrophils into the lung tissue [Citation61].
Smoking cessation leads to a significant reduction in cardiovascular disease and is the primary CVD preventive strategy for tobacco smokers. A retrospective study by Duncan et al. showed that heavy smokers (>20 pack years) who quit smoking had a significant risk reduction for incident cardiovascular disease (HR > 0.61; 95% CI, 0.49–0.76) within 5 years [Citation62]. Similarly, ang Ho Doo et al. showed that patients with COPD who stopped smoking gained a 17% reduction in all-cause mortality (aHR, 0.83; 95% CI, 0.69–1.00) and a 44% reduction in cardiovascular mortality (aHR, 0.56; 95% CI, 0.33–0.95) when compared to continuing smokers [Citation59].
3.2. Accelerated ageing
Many of the characteristics seen in COPD, such as increased air trapping, loss of lung elastic recoil, and dilatation of the alveoli, can also be seen with healthy aging of the lung and there is evidence that COPD can be considered a disease of accelerated aging [Citation63]. This process of accelerated aging is complex but includes telomere shortening, cell senescence of endothelial cells and a reduction in cell proliferation [Citation64]. Similar aging pathways have also been identified in the cardiovascular system [Citation65], suggesting common molecular mechanisms of accelerated aging explaining the increased incidence of COPD and cardiovascular disease [Citation64].
3.3. Impaired lung function trajectories in early adulthood
As described above, there are different lung function trajectories that lead to the development of COPD. A cohort study by Agusti et al. showed that approximately 4% of the general population starts with impaired FEV1 (<80%) in early adulthood. People with lower-than-normal FEV 1 in adulthood may show a normal rate of decline in pulmonary function and still meet criteria for COPD. This group had an increased risk of cardiovascular and metabolic abnormalities in early childhood and developed comorbidities ten years earlier than patients starting with a normal FEV1 [Citation66].
3.4. Hypoxemia
Hypoxemia may be seen in severe COPD and is most often caused by ventilation-perfusion (V/Q) mismatch. Hypoxemia can be the result of either a high V/Q mismatch from alveolar destruction as seen in emphysema or a low V/Q mismatch due to poor ventilation in areas with normal blood flow. Exercise-induced desaturation and hypoxemia are not generally seen until diffusion capacity is around 50% and hypoxemia at rest when diffusion capacity is as low as 30% [Citation66]. When present, hypoxemia increases systemic inflammation, oxidative stress, foam cell production and upregulation of cellular adhesion in endothelial cells, which might contribute to the progression of atherosclerosis and coronary artery disease. [Citation22] Chronic hypoxia causes pulmonary vascular remodelling and pulmonary artery endothelial dysfunction, which may contribute to the development of pulmonary hypertension [Citation67].
3.5. Hyperinflation
COPD leads to a decrease in lung elastic recoil, shifting the equilibrium between lung and thoracic cage pressure and resulting in a change in lung volumes. Hyperinflation is defined as an increase in total lung capacity above the upper limit of normal together with an increase in RV/TLC or FRC/TLC ratio [Citation2]. This static hyperinflation is associated with greater intrathoracic pressure swings during respiration which may impact cardiac function. MRI studies show that emphysema is associated with lower right and left ventricular end-diastolic volumes [Citation68]. The latter study also suggested the mechanism underpinning this was low intrathoracic blood volume caused by depression of pulmonary vasculature, induced by increased intrathoracic pressure. Reducing hyperinflation either through lung volume reduction interventions or bronchodilatation improves cardiac preload and cardiac contractility [Citation69,Citation70]. Increased thoracic pressure swings also lead to increased transmural pressures as reflected by the association with emphysema and left ventricular mass in epidemiological studies [Citation71]. Greater left ventricular mass in patients with COPD is associated with a 1.5-fold increased risk of mortality compared to COPD patients with normal left ventricular dimensions [Citation72]. Intrathoracic pressure swings can be aggravated by exercise resulting in dynamic hyperinflation. COPD patients tend to increase their end-expiratory lung volume as a consequence of expiratory flow limitation. This dynamic hyperinflation results in an attenuated cardiac response in exercises [Citation73].
4. Which populations with COPD are at risk of CVD?
In the context of community primary prevention of cardiovascular disease, it is common to use risk-scoring tools. A number of these are available, used in different settings, and varying considerably in complexity [Citation74]. Direct evidence of benefit from implementation remains unclear [Citation75]. Interestingly, the QRISK3 algorithm in the UK includes some systemic inflammatory conditions such as rheumatoid arthritis, and systemic lupus (SLE), but not COPD [Citation76]. There is evidence to suggest that QRISK3 systematically underestimates cardiac risk in people living with COPD, as do other scores such as Framingham and ASSIGN [Citation77]. Similarly, there has been the suggestion that people with COPD should be considered for anticoagulation at lower CHA2DS2-VASc scores [Citation78]. Conversely, respiratory clinicians may not be using all the information available to them in assessing and managing cardiac risk. This is increasingly apparent with the wider use of CT imaging in COPD, and in lung cancer screening programs, permitting a qualitative or quantitative assessment of coronary artery calcification. COPD is a heterogeneous condition, which raises the important question of whether specific phenotypes of COPD may be associated with excess cardiovascular risk. There is some evidence, for example, that CV risk may be tracked with airway phenotypes including those with airway wall thickening on CT [Citation79].
5. Complexity in the co-diagnosis of COPD in those with cardiovascular disease
Diagnosing COPD in patients with cardiovascular disease requires the implementation of (post-bronchodilator) spirometry testing within cardiovascular care pathways. Despite the fact that COPD is highly prevalent in patients with cardiovascular disease, as described above, and has a significant impact on long-term survival in cardiovascular diseases, guideline recommendations on how and whom to screen for COPD in cardiovascular settings are scarce. The European Society of Cardiology (ESC) guideline on heart failure only states that screening ‘can be considered in patients with suspected COPD,’ proposing spirometry as the first-line screening tool. Analogous recommendations are lacking in guidelines on ischemic heart disease and atrial fibrillation [Citation80–82]. As a consequence, spirometry testing remains underused in people with cardiovascular diseases [Citation35]. This knowledge gap has recently been addressed by a European Heart Rhythm Association (EHRA)-led multidisciplinary consortium (EHRA-PATHS) aimed at developing new multi-morbidity care pathways in AF [Citation83]. In a recent survey, specific barriers to implementing such pathways included the lack of integrated care models, organizational or institutional issues and challenges with patient adherence [Citation84].
Deploying spirometry in the setting of integrated cardiovascular care pathways comes with specific challenges. First, it is not clear who should be screened. Because of shared risk factors (e.g. smoking) and symptomatology (dyspnea, exercise intolerance), commonly used case-finding questionnaires for COPD probably lack sufficient accuracy – although there is very little data in this area. This is also true for patients with heart failure, and patients with AF, 60% of whom present with dyspnea as their main symptom [Citation3]. This likely necessitates the upfront deployment of spirometry as the first-line case-finding tool in the setting of cardiovascular disease. Next, it is not yet established how and where such spirometry should take place in cardiovascular care pathways. The capacity to complete spirometry in lung function laboratories is likely not sufficient to allow mass screening of all people with cardiovascular diseases. Case-finding for underlying COPD using handheld micro-spirometers in primary care could be an alternative strategy but comes at the price of lower accuracy and performance [Citation85]if not associated with appropriate training and quality control systems [Citation86]. Third, correctly interpreting spirometry in the context of multimorbid cardiopulmonary disease is challenging since lung function abnormalities similar to COPD can be seen in bronchial wall edema as a feature of heart failure [Citation87]. Indeed, one study showed an obstructive ventilatory deficit (defined as FEV1/FVC <0.7) in 20% of heart failure patients admitted for acute cardiac decompensation that mostly ‘resolved’ six months after admission [Citation88]. This highlights the risk of over-diagnosing ‘COPD’ in heart failure patients who are not euvolemic [Citation89]. In general, restrictive ventilatory limitations (PRISm) are more frequently seen in patients with heart failure, ranging from mild to severe restrictive ventilatory limitations [Citation90], even in the absence of underlying lung disease [Citation91]. A fourth challenge is the interpretation of obstructive ventilatory limitations in the, often elderly, multimorbid patient presenting with dyspnea or exercise intolerance. Here both pulmonary and cardiac limitations may explain the symptomatology [Citation3] complicating the correct interpretation of spirometry and subsequent treatment initiation. Measuring NT pro-BNP has added value in these settings to discriminate between COPD and heart failure and some guidelines have addressed diagnosis in these patients [Citation92]. Trans-thoracic echocardiography may be challenging in hyper-inflated people with COPD. Though not available in every setting, cardiopulmonary exercise testing might add to identifying the limiting and contributing factors to reduced exercise intolerance3and one study has suggested that ventilatory efficiency during exercise, the so-called Ve-slope, can help to identify cardiac and pulmonary limitations in patients with concomitant cardiopulmonary diseases [Citation93].
There are particular challenges in correctly interpreting lung function in the context of cardiovascular diseases. First, there is accumulating evidence that lung function a priori predisposes people to develop cardiovascular disease. For instance, a reduced FVC has been associated with an increased risk for coronary artery disease [Citation94], heart failure [Citation95], and atrial fibrillation [Citation96]. Likewise, an obstructive ventilatory limitation (‘COPD’) is independently associated with the future risk of heart failure and atrial fibrillation [Citation97]. It is thought that subclinical lung function results in structural cardiac changes increasing the risk of overt cardiovascular disease. A low FEV1/FVC is associated with left ventricular (LV) underfilling and lower cardiac output and thus is a putative precursor for overt heart failure. Likewise, a low FVC is associated with higher LV mass, higher LV filling pressure, and higher pulmonary artery systolic pressure (PASP) in later life [Citation98]. Recent exercise studies have increased our understanding of the detrimental effect of increasing pulmonary capillary pressure on ventilatory mechanics and oxygen uptake during exercise [Citation99]. Exercise induces increases in pulmonary capillary pressure and triggers a cascade of pulmonary vascular dysfunction by increasing pulsatile loading while at the same time limiting distensibility [Citation100]. Simultaneous increases in pulmonary extravascular fluid lead to a decrease in pulmonary vascular compliance and an increase in resistance, which in turn impairs pulmonary gas exchange. This cascade ultimately leads to right ventricular (RV) impairment and RV to PC uncoupling increasing respiratory drive relative to CO2 production, shown as an increase in the VE/VCO2 slope during exercise [Citation100]. In addition, other mechanisms that contribute to the (restrictive) lung function impairment seen in cardiovascular disease are respiratory muscle fatigue, cardiac enlargement and reduced compliance due to the collapse of under-perfused alveoli as a consequence of low cardiac output [Citation91].
Novel developments in digital respiratory technologies can overcome at least some of the challenges of deploying spirometry for COPD case-finding. Newer handheld spirometry devices share some advantages which make upfront spirometry in cardiology services within reach. First, these devices can include an artificial intelligence-based quality assurance process which provides immediate feedback. This decreases the number of lung function measurement failures when performed by non-experts. Secondly, spirometry recordings may be directly uploaded to a cloud where they can be reviewed remotely by a physiologist or pulmonologist for quality and interpretation. We recently described the implementation of a COPD diagnostic work-up within the setting of an AF clinical care pathway and showed that COPD screening performed by cardiology nurses is both feasible and provides sufficient accuracy when using handheld (micro)spirometry in the cardiology clinic without direct supervision [Citation101]. This is one example where digital technologies can support the development and execution of integrated care models. Future studies should aim at investigating whether such opportunistic screening can overcome organizational or institutional barriers for upscaling and whether the management approaches based on the screening improve treatment outcomes in patients with cardiovascular diseases.
6. Therapy for COPD and implications for cardiovascular events and risk
There are many effective pharmacological and non-pharmacological interventions to mitigate symptoms and reduce the risk of COPD exacerbations [Citation10]. The major non-pharmacological interventions are exposure reduction (e.g. smoking cessation), vaccinations against respiratory pathogens, and an exercise and education class termed Pulmonary Rehabilitation. Aside from short-acting, as-needed beta-2-agonist, the mainstay of pharmacologic treatment is the combination of a long-acting beta-2-agonist with a long-acting muscarinic antagonist (LABA-LAMA) with or without the addition of inhaled corticosteroids (ICS) – ideally in a single device – for those in whom the risk-benefit profile is favorable [Citation10].
Exacerbation prevention is a major goal of COPD therapy. Importantly, as exacerbations are associated with periods of elevated cardiac risk, it follows that exacerbation reduction interventions may prevent cardiovascular events. Direct evidence of that, however, has been more challenging to demonstrate in clinical trials. The SUMMIT study [Citation102] tested the hypothesis that ICS-LABA therapy would reduce all-cause mortality and the incidence of cardiovascular events in people living with COPD at elevated risk of cardiovascular disease. Cardiovascular disease was defined as established cardiovascular disease or diabetes mellitus with end-organ disease, and increased cardiovascular risk was defined as aged >60 years and receiving medication for more than two of hypercholesterolemia, hypertension, diabetes mellitus, or peripheral arterial disease. Whilst both individual treatments (ICS and LABA) and the combination ICS-LABA reduced COPD exacerbations, this did not translate to a significant reduction in all-cause mortality or in cardiovascular endpoints.
Two large studies called ETHOS [Citation103] and IMPACT [Citation104] have subsequently included all-cause mortality as a key secondary endpoint when comparing exacerbation rates between triple ICS-LABA-LAMA and dual comparators (ICS-LABA, and LABA-LAMA). ICS-LAMA has not been examined. In both studies, triple therapy delivered in one inhaler was superior to both comparators at exacerbation reduction. In ETHOS, there was a greater reduction in mortality compared to LABA-LAMA (but not ICS-LABA) [Citation105] and the suggestion that the difference in deaths was driven by fewer cardiovascular events. A similar pattern was observed in IMPACT which used a different single inhaler triple therapy suggesting that there are class effects here with ICS-LABA-LAMA [Citation104]. Note that these data primarily suggest that it is the addition of ICS that is associated with mortality reduction. The number-needed-to-treat is lower than many established CVD interventions. ICS are anti-inflammatory, and long-acting bronchodilators reduce hyperinflation thus all components are active against the key mechanisms linking COPD with CVD and which are exaggerated during exacerbations.
Using endobronchial valves to reduce hyperinflation is also associated with improvements in cardiac preload and cardiac output [Citation69], providing mechanistic support for the concept of dual bronchodilator drugs improving cardiac function through the reduction of hyperinflation.
Whilst the focus of this review is on atherosclerotic cardiovascular disease and arrhythmias resulting in acute cardiovascular events, it is important to note that COPD can also be associated with (group 3) pulmonary hypertension as described above. This is likely due to chronic hypoxic vasoconstriction, and loss of the capillary bed in emphysema [Citation106]. The latter may explain the poor response of people with group 3 pulmonary hypertension to vasodilator drugs, and thus the poor prognosis of this group. For example, the phosphodiesterase-5 inhibitor tadalafil was not associated with either improved exercise capacity or quality of life in COPD [Citation107]. Detection of patients with significant pulmonary hypertension remains important, to guide the use of long-term oxygen therapy and assessment for transplantation. Whilst definitive assessment requires right-heart catheterization, the ratio of the pulmonary artery to aorta size on CT can provide an initial indication of likely pulmonary hypertension and risk of severe exacerbations [Citation108].
Regarding pulmonary rehabilitation, physical activity outside of the class was more closely related to improvements in aortic stiffness – as a marker of cardiovascular risk – than improvement in exercise capacity in people with COPD attending pulmonary rehabilitation [Citation109] such that responses in aortic stiffness during PR are variable [Citation110].
6.1. Using cardiovascular drugs to treat COPD
Given the high prevalence of cardiovascular disease and risk factors in patients living with COPD, including smoking, hypertension and hypercholesterolemia, primary prevention through risk factor modification is an important treatment goal [Citation22]. This includes regular cardiovascular assessment (blood pressure measurements) and adequate lifestyle advice (smoking cessation and regular exercise). Interestingly, influenza vaccination might offer both respiratory and cardiac benefits to patients with COPD and should be promoted accordingly [Citation111]. Patients with clinically manifest cardiovascular disease and COPD should receive guideline-recommended treatment of cardiovascular disease irrespective of COPD diagnosis. Of note, this includes beta-blockers, for instance, in patients with heart failure or atrial fibrillation. Current practices show the underuse of such medications, even when indicated using conventional criteria and despite the good safety profile of cardio-selective beta-blockers in COPD [Citation112].
Real-world evidence suggested better COPD outcomes in people with COPD receiving treatment with cardiovascular drugs. [Citation113] And incentivized randomized controlled trials of cardiovascular drugs in COPD, outside the primary cardiovascular indication, have evaluated if such drugs can ameliorate COPD outcomes including exacerbations. However, many of these interventions showed negative results when assessed in RCTs. For instance, the BLOCK-COPD, a study in people with moderate to severe COPD and no primary indication for β-blocker, was prematurely stopped because of an increased risk of hospitalization in those randomized to metoprolol [Citation114]. Likewise, simvastatin did not reduce the risk of COPD exacerbations in a randomized controlled trial [Citation115]. More recently, observational evidence has emerged suggesting that GLP-1 receptor agonists and SGLT-2 inhibitors (but not DPP-4 inhibitors) are associated with fewer severe exacerbations compared with sulfonylureas in people with COPD and type 2 diabetes [Citation116]. Observational data also exist for benefits on mortality and exacerbations from drugs affecting the renin-angiotensin system [Citation117]. The same is true for antiplatelet therapies, where observational studies have been associated with reduced exacerbation risk and mortality [Citation118] and mechanistic studies support altered responses to aspirin in COPD [Citation119], which could contribute to thrombotic complications. However, given the discrepancy between real-world and RCT evidence for β-blockers and statins, such results with other drug classes urgently require confirmation in RCTs. Such trials are additionally complex to conduct and interpret because of high screen-fail rates given the high prevalence of (previously undiagnosed) cardiovascular disease and CVD risk factors in clinical trial populations with COPD.
7. Cardiovascular risk and exacerbations of COPD
The increased vulnerability to cardiovascular events in patients with COPD is even more pronounced around the time of and following an exacerbation of COPD. In a post hoc analysis of the SUMMIT trial, described above, the hazard ratio (HR) for cardiovascular events was 4 times higher in the first 30 days after an exacerbation [Citation120]. Similar results have been published from registry studies. In a national Danish study, the odds for a cardiovascular event was 3.7 times higher within 4 weeks after an exacerbation compared to exacerbation-free time periods [Citation121]. This risk was consistent throughout each of the major cardiovascular outcomes studied; cardiovascular death OR 4.3, acute MI OR 3.6 and stroke OR 2.8 [Citation121]. Recently, the results from a multi-country registry study were published investigating the risk of cardiovascular events after an exacerbation, the EXACOS-CV study [Citation122]. This study showed that CV risk peaked around the onset of the exacerbation (day 1–7) with the odds ratios for cardiovascular events or death ranging between 1.2–3.9 and 15.8–49.6 after a moderate or severe (hospitalized) exacerbation respectively [Citation123]. This and other studies have shown that CV risk is most pronounced when an exacerbation is more severe [Citation120,Citation124]. Importantly, the increased risk for cardiovascular events following an exacerbation persists beyond this short-term period. In the previously mentioned SUMMIT trial, the hazard risk of cardiovascular events remained doubled for up to one year after a moderate or severe exacerbation [Citation120].
Several mechanisms may explain the increased CV risk after an exacerbation. Central to the pathophysiology of an exacerbation is a worsening of airway inflammation evoked by viruses, bacteria and environmental pollutants [Citation125]. These pro-inflammatory cytokines and inflammatory mediators, such as interleukin-6 and tumor necrosis factor-alpha, can ‘leak’ into the systemic circulation causing systemic inflammation, which in turn can activate inflammatory cells in atherosclerotic plaques [Citation126]. This exacerbation stimulus-associated activity in atherosclerotic plaques contributes to plaque destabilization and increases the risk of thrombotic events such as myocardial infarction and stroke [Citation126]. The risk of such ischemic vascular events may also be increased as a result of the accompanying hypoxemia seen during an exacerbation and the increased oxygen demand due to inflammatory- and medication-induced tachycardia [Citation127]. Other pathogen-specific mechanisms could be the direct invasion of viruses into the heart causing local inflammation such as is seen in influenza [Citation127].
This inflammatory response simultaneously activates coagulation and thrombosis pathways that further increase the risk of thrombosis at sites of plaque disruption causing cardiovascular events [Citation128]. COPD exacerbations are associated with increased platelet activation, which can result in the formation of vascular thrombosis [Citation129]. Plasma coagulation is further increased during an exacerbation of COPD, also predisposing patients to venous thromboembolisms [Citation129]. The inflammatory response, combined with the increased oxidative stress seen with an exacerbation, can impair endothelial function. Indeed, markers of endothelial dysfunction, such as activated von Willebrand Factor, are increased during an exacerbation [Citation42,Citation129]. Such endothelial dysfunction can lead to the progression and rupture of atherosclerotic lesions and decreased blood flow due to vasospasm and thrombosis [Citation130].
Changes in lung mechanics and accompanying VQ mismatch during an exacerbation also make COPD patients vulnerable to cardiovascular events [Citation131]. Bronchospasm and mucus impaction lead to an increase in airway resistance and subsequent worsening of expiratory flow limitation [Citation131]. Expiratory flow limitation in turn leads to (dynamic) hyperinflation and increased positive intrathoracic pressures [Citation131]. This increase in dynamic hyperinflation (DH) impairs venous return and reduces right ventricular preload [Citation132]. Right ventricular end-diastolic pressure increases as a result of increased pulmonary vascular resistance (PVR) caused by the positive intrathoracic pressure and PVR is further increased as a result of hypoxemic vasoconstriction due to increased VQ mismatch seen in exacerbations [Citation131]. The resulting reduced right ventricular function impairs left ventricular diastolic function as a consequence of interventricular dependency [Citation133]. Left ventricular function is further depressed by increased transmural pressure and left ventricular afterload resulting from negative thoracic pressure as a consequence of increased airway resistance [Citation132]. Tachycardia, hypoxemia and hypercapnia further contribute to a compromised left ventricular function and can induce myocardial infarction, (supra)ventricular arrhythmia, heart failure and cardiovascular death.
A fourth mechanism that could trigger cardiovascular events is increased sympathetic nerve activation in COPD [Citation134]. An exacerbation promotes a milieu for sympathetic nerve overactivation: systemic inflammation, increased respiratory effort, increased thoracic pressure swings, secondary pulmonary hypertension, concomitant hypoxemia and hypercapnia can all trigger sympathetic nerve activity [Citation135]. Though beta-agonist use has not been shown to be associated with sympathetic nerve overactivity [Citation136], (over)use of beta-agonists is common during an exacerbation [Citation137] and might therefore trigger the sympathetic nerve system. Overactivity of the sympathetic nerve system leads to vasoconstriction, hypertension and increased afterload contributing to cardiovascular events [Citation135]. Increased sympathetic nerve activity also increases automaticity and action potential shortening which may lead to (supra)ventricular arrhythmias, such as atrial fibrillation [Citation42]. Indeed, the risk of an AF-related hospitalization is indeed increased in the first 90 days after a severe exacerbation [Citation42]. Other arrhythmogenic triggers during an exacerbation are the increased right atrial pressure as a consequence of the increased right ventricular end-diastolic pressure (RVEDP) and the transient shortening of the right atrial effective refractory period resulting from the increased intrathoracic pressure swings [Citation42]. Interestingly, it has been shown that the transition from hypercapnia to normo-ventilation, rather than prolonged hypoxemia or hypercapnia itself, increases right atrial vulnerability leading to conducting disturbances [Citation138].
Some of the above-mentioned mechanisms might also explain the extended risk (beyond the first 30 days) of cardiovascular events around the time of a COPD exacerbation although this is still poorly understood. The mechanisms may be similar to the prolonged risk of cardiovascular events observed after pneumonia and include persistent inflammatory activity and a prolonged procoagulant state [Citation139]. Long-term studies on inflammatory trajectories in COPD are scarce. Two small studies show full resolution of systemic inflammation after 14 days, however [Citation140]. Even less is known about whether the hypercoagulable state persists after an exacerbation [Citation141]. Exacerbations lead to a decline in lung function. In around 7–8% of COPD patients, lung function does not fully recover >90 days after an exacerbation [Citation142]. As mentioned previously such patients will have a sustained worsening of expiratory flow which depresses left ventricular function and could put them at risk for heart failure and ischemic heart disease. Other proposed mechanisms for the prolonged cardiovascular risk after an exacerbation are accelerated atherosclerosis induced either by endothelial dysfunction or oxidative stress, or reduced physical activity after an exacerbation [Citation78]. In favor of the latter hypothesis is the prolonged and slow recovery of arterial stiffness that is seen after an exacerbation [Citation143]. Another interesting hypothesis is that an exacerbation induces changes in the airway or gastrointestinal microbiome that could be more atherogenic [Citation144]. There is indeed a link between changes in gut microbiota and the occurrence of cardiovascular disease [Citation145]. A final putative mechanism explaining the extended cardiovascular risk after an exacerbation of COPD is the observation that exacerbations may induce myocardial fibrosis as seen in studies using MRI [Citation146], which could subsequently be an ongoing substrate for left ventricular dysfunction or arrhythmias.
8. Practical advice: pro-active identification and management of CV risk in COPD
The best current advice is to carefully consider and manage cardiovascular risk factors in people living with COPD, , and to consider the presence of undiagnosed COPD in people with established cardiovascular disease with institution of appropriate COPD management. Active steps include regular cardiovascular risk management in every person living with COPD allowing prevention of, or early detection of cardiovascular comorbidities. Likewise, COPD should be actively sought (or excluded) early in people with cardiovascular disease through active case-finding and detection of abnormal lung function (COPD and PRISm). The presence of abnormal spirometry should prompt the cardiovascular clinician to optimize respiratory health. Given the overlapping symptoms and intertwined pathophysiology, patients with combined cardiovascular and respiratory disease will likely benefit from a multidisciplinary and integrated care approach [Citation3]. People living with COPD and clinically manifest cardiovascular disease should receive cardiovascular treatment according to existing guidelines, mitigating current evidence suggesting undertreatment of CV disease in people with COPD. However, there is no current evidence to support the wider use of cardiovascular therapies beyond established cardiovascular indications in COPD to improve COPD outcomes. Optimizing COPD treatment, particularly with regards to preventing exacerbations, reduces premature, avoidable cardiopulmonary mortality and that seems, at least in part, to be driven by a reduced risk of cardiovascular events with ICS-LABA-LAMA. Those working in cardiovascular medicine should be alert to undiagnosed or inadequately treated COPD and recognize that appropriate diagnosis and management of COPD may provide additional benefit on cardiovascular outcomes.
Figure 2. Cardiovascular risk assessment in COPD.
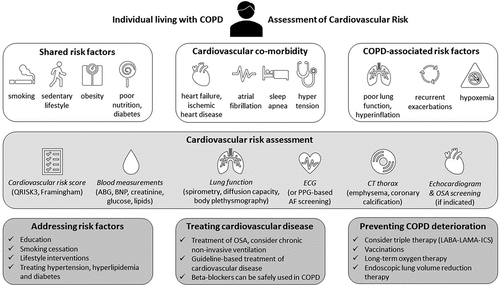
9. Conclusion
COPD and CVD commonly coexist but the overlap is often not recognized. Combined COPD-CVD has a severe impact on quality of life and results in premature, avoidable mortality throughout the spectrum of cardiovascular diseases. Key mechanisms include inflammation, hyperinflation, and hypoxemia. Proactive identification of CVD and CV risk in people with COPD, and COPD in people with CVD is essential to reduce cardiopulmonary risk and improve clinical outcomes.
10. Expert opinion
Given the high prevalence of co-existing COPD and CVD, and the elevated CV risk in COPD, not adopting a pro-active approach to the identification of COPD in those with CVD, and not managing CV risk in those with COPD will lead to preventable ill-health and avoidable, premature mortality. The challenge here is primarily one of health policy and policy implementation: the tools to diagnose COPD are not complex, yet access to spirometry remains limited in many settings. Similarly, there is a lack of awareness that COPD (including undiagnosed COPD) is a major risk factor for cardiovascular disease. Addressing both is key to making progress toward reducing premature cardiopulmonary mortality, including meeting WHO Sustainable Development Goals. A deeper understanding of the complex mechanisms underlying the interactions between COPD and CVD could assist development of novel interventions. Meanwhile, effective treatment of both conditions according to up-to-date guidance is the current best practical approach. Closer integration of care between cardiologists, pulmonologists and primary care teams – in partnership with patients can best support this. Given that COPD exacerbations are a major driver of CV risk in COPD, a renewed focus on preventing exacerbations is an important approach. This includes a ‘zero tolerance’ of exacerbations by patients and clinicians: having frequent exacerbations in COPD should not be seen as an inevitable consequence of living with COPD, in the same way, that myocardial infarction is not an inevitable consequence of living with cardiovascular risk. Future opportunities include the ability of artificial intelligence to support the interpretation and quality assurance of spirometry, and the wider roll-out of CT-based lung cancer screening, and CT-based cardiac imaging, the images from both of which can support the identification of those with emphysema, and those with elevated cardiovascular risk through coronary artery calcification assessment. As a result of these developments, and greater awareness of the importance of co-existent COPD and CVD, we advocate for and anticipate better identification of co-existent COPD and CVD, and management of CV risk in COPD over the coming years.
Given that patients with COPD are at an increased risk both for future pulmonary (i.e. exacerbations) as well as for cardiovascular (i.e. MACE) adverse events, the term cardiopulmonary risk has been introduced [Citation147]. Cardiopulmonary risk can be understood as the chance of a patient with COPD experiencing a major adverse cardiovascular or pulmonary event, or both. Introducing this term conveys two powerful messages that could change the lives of people living with COPD. First, it emphasizes the need to treat patients with COPD in such a way that both cardiovascular and pulmonary adverse outcomes are minimized. Second, it steers treatment approaches away from the current reactive and symptom-based treatment algorithms toward a more proactive and preventive approach, mitigating future risks and -putatively- improving survival. Fundamental gaps in knowledge exist to realize this risk-reducing potential of COPD treatments and future studies should focus on unravelling the mechanisms driving this increased cardiovascular risk, as well as on exploring different treatment approaches to reduce cardiopulmonary events in people living with COPD. For instance, further evidence is required to understand the mechanisms of all-cause and CVD-related mortality reduction observed with ‘triple therapy’ (ICS-LABA-LAMA) in COPD. Second, current trials on cardiovascular drugs have so far failed to show any cardiopulmonary benefit in people living with COPD, which begs the question of whether specific endotypes of phenotypes of COPD exist that show favorable cardiopulmonary outcomes. Other unresolved research questions surround the timing of such cardiovascular drugs in COPD. The existing prothrombotic milieu of an exacerbation of COPD for instance suggests that anticoagulant therapy after an exacerbation might be helpful to decrease cardiovascular risk. These and other researchable questions that have been addressed [Citation147], open a new research area with exciting possibilities for people living with COPD.
Article highlights
The new definition and classification of Chronic Obstructive Pulmonary Disease (COPD) and the term Preserved Ratio Impaired Spirometry (PRISm) to identify individuals with an increased risk for cardiovascular disease (CVD).
The high disease burden of co-morbid COPD and CVD and importance of recognizing and treating both COPD and cardiovascular disease in people living with both conditions.
Mechanisms underlying the increased prevalence of CVD in COPD, such as shared risk factors, systemic inflammation, accelerated aging, impaired lung function trajectories, hypoxemia and hyperinflation
The challenges of diagnosing COPD in people with cardiovascular disease using questionnaires and pulmonary function tests.
The pivotal role of exacerbations of COPD in the increased risk of CVD
Potential therapies that decrease the cardiovascular risk in people with COPD including combined (‘triple’) inhaler drugs
A pro-active identification of CVD risk in COPD and propose a treatment strategy to mitigate this increased cardiovascular risk
The challenges of implementing cardiovascular risk reduction in a population with COPD and put forward a research agenda of unresolved questions that should be addressed in future studies
Declaration of interests
S.O. Simons has received grants from AstraZeneca, Boehringer Ingelheim, Chiesi, GlaxoSmithKline and Roche; Consulting fees from AstraZeneca, Boehringer Ingelheim, Chiesi and GlaxoSmithKline; payment or honoraria for lectures and presentations from AstraZeneca and Chiesi; Support for attending meetings and/or travel from AstraZeneca and Chiesi; leadership role as chair to Dutch COPD working group of the Dutch Thoracic Society; Oximeters from the Dutch Thoracic Society. All payments were to his institution. J.R. Hurst has received grants from AstraZeneca; consulting fees from AstraZeneca and GlaxoSmithKline; payment or honoraria for presentations and lectures from AstraZeneca, Boehringer Ingelheim, Chiesi, Sanofi and Takeda; support for attending meeting and/or travel from AstraZeneca; participation in advisory board from AstraZeneca: Donation of Nonin oximeters. The authors have no other relevant affiliations or financial involvement with any organization or entity with a financial interest in or financial conflict with the subject matter or materials discussed in the manuscript apart from those disclosed.
Reviewer disclosures
Peer reviewers on this manuscript have no relevant financial or other relationships to disclose.
Additional information
Funding
References
- Alvar Agusti MC, Richard Beasley M, Bartolome R, et al. Global strategy for the diagnosis, management, and prevention of chronic obstructive pulmonary disease. 2023.
- Stanojevic S, Kaminsky DA, Miller MR, et al. ERS/ATS technical standard on interpretive strategies for routine lung function tests. Eur Respir J. 2022;60(1):2101499. doi: 10.1183/13993003.01499-2021
- van der Velden RMJ, Hermans ANL, Pluymaekers NAHA, et al. Dyspnea in patients with atrial fibrillation: mechanisms, assessment and an interdisciplinary and integrated care approach. Int J Cardiol Heart Vasc. 2022;42:101086. doi: 10.1016/j.ijcha.2022.101086
- Ananth S, Hurst JR. ERJ advances: state of the art in definitions and diagnosis of COPD. Eur Respir J. 2023;61(4):2202318. doi: 10.1183/13993003.02318-2022
- Agusti A, Faner R. Lung function trajectories in health and disease. Lancet Respir Med. 2019;7(4):358–364. doi: 10.1016/S2213-2600(18)30529-0
- Han MK, Agusti A, Celli BR, et al. From GOLD 0 to Pre-COPD. Am J Respir Crit Care Med. 2021;203(4):414–423. doi: 10.1164/rccm.202008-3328PP
- Higbee DH, Granell R, Davey Smith G, et al. Prevalence, risk factors, and clinical implications of preserved ratio impaired spirometry: a UK biobank cohort analysis. Lancet Respir Med. 2022;10(2):149–157. doi: 10.1016/S2213-2600(21)00369-6
- Rabe KF, Hurst JR, Suissa S. Cardiovascular disease and COPD: dangerous liaisons? Eur Respir Rev. 2018;27(149):180057. doi: 10.1183/16000617.0057-2018
- Labaki WW, Gu T, Murray S, et al. Causes of and clinical features associated with death in tobacco cigarette users by lung function impairment. Am J Respir Crit Care Med. 2023;208(4):451–460. doi: 10.1164/rccm.202210-1887OC
- Agustí A, Celli BR, Criner GJ, et al. Global initiative for chronic obstructive lung disease 2023 report: GOLD executive summary. Eur Respir J. 2023;61(4):2300239. doi: 10.1183/13993003.00239-2023
- Adeloye D, Song P, Zhu Y, et al. Global, regional, and national prevalence of, and risk factors for, chronic obstructive pulmonary disease (COPD) in 2019: a systematic review and modelling analysis. Lancet Respir Med. 2022;10(5):447–458. doi: 10.1016/S2213-2600(21)00511-7
- Siddharthan T, Pollard SL, Quaderi SA, et al. Discriminative accuracy of chronic obstructive pulmonary disease screening instruments in 3 low- and middle-income country settings. JAMA. 2022;327(2):151–160. doi: 10.1001/jama.2021.23065
- Sievi NA, Sepin J, Roeder M, et al. Are predictors for overall Mortality in COPD patients robust over Time? J Clin Med. 2023;12(4):1587. doi: 10.3390/jcm12041587
- Chen S, Kuhn M, Prettner K, et al. The global economic burden of chronic obstructive pulmonary disease for 204 countries and territories in 2020-50: a health-augmented macroeconomic modelling study. Lancet Glob Health. 2023;11(8):e1183–e1193.
- Tabyshova A, Hurst JR, Soriano JB, et al. Gaps in COPD guidelines of low- and middle-income countries: a systematic scoping review. Chest. 2021;159(2):575–584. doi: 10.1016/j.chest.2020.09.260
- Hurst JR, Buist AS, Gaga M, et al. Challenges in the Implementation of chronic obstructive pulmonary disease Guidelines in low- and middle-income countries: an official American Thoracic Society Workshop Report. Ann Am Thorac Soc. 2021;18(8):1269–1277. doi: 10.1513/AnnalsATS.202103-284ST
- Florman KEH, Siddharthan T, Pollard SL, et al. Unmet diagnostic and therapeutic opportunities for chronic obstructive pulmonary disease in low- and middle-income countries. Am J Respir Crit Care Med. 2023;208(4):442–450. doi: 10.1164/rccm.202302-0289OC
- Agarwal D. COPD generates substantial cost for health systems. Lancet Glob Health. 2023;11(8):e1138–e1139. doi: 10.1016/S2214-109X(23)00304-2
- Onishi K. Total management of chronic obstructive pulmonary disease (COPD) as an independent risk factor for cardiovascular disease. J Cardiol. 2017;70(2):128–134. doi: 10.1016/j.jjcc.2017.03.001
- Maclagan LC, Croxford R, Chu A, et al. Quantifying COPD as a risk factor for cardiac disease in a primary prevention cohort. Eur Respir J. 2023;62(2):2202364. doi: 10.1183/13993003.02364-2022
- Chen W, Thomas J, Sadatsafavi M, et al. Risk of cardiovascular comorbidity in patients with chronic obstructive pulmonary disease: a systematic review and meta-analysis. Lancet Respir Med. 2015;3(8):631–639. doi: 10.1016/S2213-2600(15)00241-6
- Morgan AD, Zakeri R, Quint JK. Defining the relationship between COPD and CVD: what are the implications for clinical practice? Ther Adv Respir Dis. 2018;12:1753465817750524. doi: 10.1177/1753465817750524
- Kibbler J, Wade C, Mussell G, et al. Systematic review and meta-analysis of prevalence of undiagnosed major cardiac comorbidities in COPD. ERJ Open Res. 2023;9(6):00548–02023. Published online October 12. doi: 10.1183/23120541.00548-2023.
- Goedemans L, Bax JJ, Delgado V. COPD and acute myocardial infarction. Eur Respir Rev. 2020;29(156):190139. doi: 10.1183/16000617.0139-2019
- Campo G, Pavasini R, Malagù M, et al. Chronic obstructive pulmonary disease and ischemic heart disease comorbidity: overview of mechanisms and clinical management. Cardiovasc Drugs Ther. 2015;29(2):147–157. doi: 10.1007/s10557-014-6569-y
- Divo M, Cote C, de Torres JP, et al. Comorbidities and risk of mortality in patients with chronic obstructive pulmonary disease. Am J Respir Crit Care Med. 2012;186(2):155–161. doi: 10.1164/rccm.201201-0034OC
- Cazzola M, Calzetta L, Matera MG, et al. Chronic obstructive pulmonary disease and coronary disease: COPDCoRi, a simple and effective algorithm for predicting the risk of coronary artery disease in COPD patients. Respir med. 2015;109(8):1019–1025. doi: 10.1016/j.rmed.2015.05.021
- Bhatt SP, Nath HP, Kim YI, et al. Centrilobular emphysema and coronary artery calcification: mediation analysis in the SPIROMICS cohort. Respir Res. 2018;19(1):257. doi: 10.1186/s12931-018-0946-1
- Chandra D, Gupta A, Kinney GL, et al. The association between lung hyperinflation and coronary artery disease in smokers. Chest. 2021;160(3):858–871. doi: 10.1016/j.chest.2021.04.066
- Papaporfyriou A, Bartziokas K, Gompelmann D, et al. Cardiovascular diseases in COPD: from diagnosis and prevalence to therapy. Life (Basel). 2023;13(6):1299. doi: 10.3390/life13061299
- Mooney L, Hawkins NM, Jhund PS, et al. Impact of chronic obstructive pulmonary disease in patients with Heart failure with preserved ejection fraction: insights from PARAGON-HF. J Am Heart Assoc. 2021;10(23):e021494. doi: 10.1161/JAHA.121.021494
- Hawkins NM, Peterson S, Salimian S, et al. Epidemiology and treatment of heart failure with chronic obstructive pulmonary disease in Canadian primary care. ESC Heart Fail. 2023;10(6):3612–3621.
- Axson EL, Ragutheeswaran K, Sundaram V, et al. Hospitalisation and mortality in patients with comorbid COPD and heart failure: a systematic review and meta-analysis. Respir Res. 2020;21(1):54. doi: 10.1186/s12931-020-1312-7
- Ehteshami-Afshar S, Mooney L, Dewan P, et al. Clinical characteristics and outcomes of patients with Heart failure with Reduced ejection fraction and chronic obstructive pulmonary disease: insights from PARADIGM-HF. J Am Heart Assoc. 2021;10(4):e019238. doi: 10.1161/JAHA.120.019238
- Canepa M, Franssen FME, Olschewski H, et al. Diagnostic and therapeutic gaps in patients with Heart failure and chronic obstructive pulmonary disease. JACC Heart Fail. 2019;7(10):823–833. doi: 10.1016/j.jchf.2019.05.009
- Rutten FH, Cramer MJM, Lammers JWJ, et al. Heart failure and chronic obstructive pulmonary disease: an ignored combination? Eur J Heart Fail. 2006;8(7):706–711. doi: 10.1016/j.ejheart.2006.01.010
- Hawkins NM, Petrie MC, Jhund PS, et al. Heart failure and chronic obstructive pulmonary disease: diagnostic pitfalls and epidemiology. Eur J Heart Fail. 2009;11(2):130–139. doi: 10.1093/eurjhf/hfn013
- Zhang L, Liu Y, Zhao S, et al. The incidence and prevalence of pulmonary hypertension in the COPD population: a systematic review and meta-analysis. Int J Chron Obstruct Pulmon Dis. 2022;17:1365–1379. doi: 10.2147/COPD.S359873
- Blanco I, Tura-Ceide O, Peinado V VI. Updated perspectives on pulmonary hypertension in COPD. Int J Chron Obstruct Pulmon Dis. 2020;15:1315–1324. doi:10.2147/COPD.S211841.
- Olsson KM, Corte TJ, Kamp JC, et al. Pulmonary hypertension associated with lung disease: new insights into pathomechanisms, diagnosis, and management. Lancet Respir Med. 2023;11(9):820–835. doi: 10.1016/S2213-2600(23)00259-X
- Barnes H, Brown Z, Burns A, et al. Phosphodiesterase 5 inhibitors for pulmonary hypertension. Cochrane Database Syst Rev. 2019;2019(3):CD012621. doi: 10.1002/14651858.CD012621.pub2
- Simons SO, Elliott A, Sastry M, et al. Chronic obstructive pulmonary disease and atrial fibrillation: an interdisciplinary perspective. Eur Heart J. 2021;42(5):532–540. doi: 10.1093/eurheartj/ehaa822
- Proietti M, Laroche C, Drozd M, et al. Impact of chronic obstructive pulmonary disease on prognosis in atrial fibrillation: A report from the EURObservational Research Programme Pilot Survey on Atrial Fibrillation (EORP-AF) General Registry. Am Heart J. 2016;181:83–91. doi: 10.1016/j.ahj.2016.08.011
- Durheim MT, Holmes DN, Blanco RG, et al. Characteristics and outcomes of adults with chronic obstructive pulmonary disease and atrial fibrillation. Heart. 2018;104(22):1850–1858. doi: 10.1136/heartjnl-2017-312735
- Emil Warming P, Garcia R, Johann Hansen C, et al. Atrial fibrillation and chronic obstructive pulmonary disease: diagnostic sequence and mortality risk. Eur Heart J Qual Care Clin Outcomes. 2023;9(2): 128–134. doi: 10.1093/ehjqcco/qcac059
- Warming PE, Garcia GR, Hansen CJ, et al. Atrial fibrillation and chronic obstructive pulmonary disease: diagnostic sequence and mortality risk. Eur Heart J Qual Care Clin Outcomes. Published online Feb 2023;9(2):128–134. doi: 10.1093/ehjqcco/qcac059
- Shah AJ, Quek E, Alqahtani JS, et al. Cardiovascular outcomes in patients with COPD-OSA overlap syndrome: A systematic review and meta-analysis. Sleep Med Rev. 2022;63:101627. doi: 10.1016/j.smrv.2022.101627
- Gleeson M, McNicholas WT. Bidirectional relationships of comorbidity with obstructive sleep apnoea. Eur Respir Rev. 2022;31(164):210256. doi: 10.1183/16000617.0256-2021
- Bouloukaki I, Fanaridis M, Testelmans D, et al. Overlaps between obstructive sleep apnoea and other respiratory diseases, including COPD, asthma and interstitial lung disease. Breathe. 2022;18(3):220073. doi: 10.1183/20734735.0073-2022
- Messineo L, Lonni S, Magri R, et al. Lung air trapping lowers respiratory arousal threshold and contributes to sleep apnea pathogenesis in COPD patients with overlap syndrome. Respir Physiol Neurobiol. 2020;271:103315. doi: 10.1016/j.resp.2019.103315
- McNicholas WT. COPD-OSA Overlap Syndrome: Evolving Evidence Regarding Epidemiology, Clinical Consequences, and Management. Chest. 2017;152(6):1318–1326. doi: 10.1016/j.chest.2017.04.160
- Kim V, Han MK, Vance GB, et al. The chronic bronchitic phenotype of COPD: an analysis of the COPDGene Study. Chest. 2011;140(3):626–633. doi: 10.1378/chest.10-2948
- Shawon MSR, Perret JL, Senaratna CV, et al. Current evidence on prevalence and clinical outcomes of co-morbid obstructive sleep apnea and chronic obstructive pulmonary disease: A systematic review. Sleep Med Rev. 2017;32:58–68. doi: 10.1016/j.smrv.2016.02.007
- McNicholas WT. Chronic obstructive pulmonary disease and obstructive sleep apnoea-the overlap syndrome. J Thorac Dis. 2016;8(2):236–242. doi: 10.21037/jtd.2016.12.36
- van Zeller M, Basoglu OK, Verbraecken J, et al. Sleep and cardiometabolic comorbidities in the obstructive sleep apnoea-COPD overlap syndrome: data from the European Sleep Apnoea Database. ERJ Open Res. 2023;9(3). doi: 10.1183/23120541.00676-2022
- Sodhi A, Pisani M, Glassberg MK, et al. Sex and Gender in Lung Disease and Sleep Disorders: A State-of-the-Art Review. Chest. 2022;162(3):647–658. doi: 10.1016/j.chest.2022.03.006
- Sánchez-de-la-Torre M, Gracia-Lavedan E, Benitez ID, et al. Adherence to CPAP Treatment and the risk of recurrent cardiovascular events: a meta-analysis. JAMA. 2023;330(13):1255–1265. doi: 10.1001/jama.2023.17465
- Macrea M, Oczkowski S, Rochwerg B, et al. Long-term noninvasive ventilation in chronic stable hypercapnic chronic obstructive pulmonary disease. an official American thoracic society clinical practice guideline. Am J Respir Crit Care Med. 2020;202(4):e74–e87. doi: 10.1164/rccm.202006-2382ST
- Almagro P, Boixeda R, Diez-Manglano J, et al. Insights into chronic obstructive pulmonary disease as critical risk factor for cardiovascular disease. Int J Chron Obstruct Pulmon Dis. 2020;15:755–764. doi:10.2147/COPD.S238214.
- Gallucci G, Tartarone A, Lerose R, et al. Cardiovascular risk of smoking and benefits of smoking cessation. J Thorac Dis. 2020;12(7):3866–3876. doi: 10.21037/jtd.2020.02.47
- Kotlyarov S. The role of smoking in the mechanisms of development of chronic obstructive pulmonary disease and atherosclerosis. Int J Mol Sci. 2023;24(10):8725. doi: 10.3390/ijms24108725
- Doo JH, Kim SM, Park YJ, et al. Smoking cessation after diagnosis of COPD is associated with lower all-cause and cause-specific mortality: a nationwide population-based cohort study of South Korean men. BMC Pulm Med. 2023;23(1):237. doi: 10.1186/s12890-023-02533-1
- MacNee W. Is chronic obstructive pulmonary disease an accelerated aging disease? Ann Am Thorac Soc. 2016;13(Suppl 5):S429–S437. doi: 10.1513/AnnalsATS.201602-124AW
- Barnes PJ. Senescence in COPD and its comorbidities. Annu Rev Physiol. 2017;79(1):517–539. doi: 10.1146/annurev-physiol-022516-034314
- North BJ, Sinclair DA. The intersection between aging and cardiovascular disease. Circ Res. 2012;110(8):1097–1108. doi: 10.1161/CIRCRESAHA.111.246876
- Agustí A, Noell G, Brugada J, et al. Lung function in early adulthood and health in later life: a transgenerational cohort analysis. Lancet Respir Med. 2017;5(12):935–945. doi: 10.1016/S2213-2600(17)30434-4
- Gredic M, Blanco I, Kovacs G, et al. Pulmonary hypertension in chronic obstructive pulmonary disease. Br J Pharmacol. 2021;178(1):132–151. doi: 10.1111/bph.14979
- Jörgensen K, Müller MF, Nel J, et al. Reduced intrathoracic blood volume and left and right ventricular dimensions in patients with severe emphysema: an MRI study. Chest. 2007;131(4):1050–1057. doi: 10.1378/chest.06-2245
- van der Molen MC, Hartman JE, Vanfleteren LEGW, et al. Reduction of Lung Hyperinflation Improves Cardiac Preload, Contractility, and Output in Emphysema: A Clinical Trial in Patients Who Received Endobronchial Valves. Am J Respir Crit Care Med. 2022;206(6):704–711. doi: 10.1164/rccm.202201-0214OC
- Hohlfeld JM, Vogel-Claussen J, Biller H, et al. Effect of lung deflation with indacaterol plus glycopyrronium on ventricular filling in patients with hyperinflation and COPD (CLAIM): a double-blind, randomised, crossover, placebo-controlled, single-centre trial. Lancet Respir Med. 2018;6(5):368–378. doi: 10.1016/S2213-2600(18)30054-7
- Smith BM, Kawut SM, Bluemke DA, et al. Pulmonary hyperinflation and left ventricular mass: the Multi-Ethnic Study of Atherosclerosis COPD Study. Circulation. 2013;127(14):1503–1511, 1511e1–6. doi: 10.1161/CIRCULATIONAHA.113.001653
- Short PM, Anderson WJ, Elder DHJ, et al. Impact of left ventricular hypertrophy on survival in chronic obstructive pulmonary disease. Lung. 2015;193(4):487–495. doi: 10.1007/s00408-015-9724-8
- Smith JR, Johnson BD, Olson TP. Impaired central hemodynamics in chronic obstructive pulmonary disease during submaximal exercise. J Appl Physiol (1985). 2019;127(3):691–697. doi: 10.1152/japplphysiol.00877.2018
- Sacramento-Pacheco J, Duarte-Clíments G, Gómez-Salgado J, et al. Cardiovascular risk assessment tools: A scoping review. Aust Crit Care. 2019;32(6):540–559. doi: 10.1016/j.aucc.2018.09.008
- Karmali KN, Persell SD, Perel P, et al. Risk scoring for the primary prevention of cardiovascular disease. Cochrane Database Of Systematic Reviews. 2017;2021(6):CD006887. doi: 10.1002/14651858.CD006887.pub4
- Hippisley-Cox J, Coupland C, Brindle P. Development and validation of QRISK3 risk prediction algorithms to estimate future risk of cardiovascular disease: prospective cohort study. BMJ. 2017;357:j2099. doi: 10.1136/bmj.j2099
- van Staa TP, Gulliford M, Ng ES-W, et al. Prediction of cardiovascular risk using Framingham, ASSIGN and QRISK2: how well do they predict individual rather than population risk? PLoS One. 2014;9(10):e106455. doi: 10.1371/journal.pone.0106455
- Balbirsingh V, Mohammed AS, Turner AM, et al. Cardiovascular disease in chronic obstructive pulmonary disease: a narrative review. Thorax. Published online Jun 30, 2022;77(9):939–945. doi: 10.1136/thoraxjnl-2021-218333
- Young KA, Regan EA, Han MK, et al. Subtypes of COPD have unique distributions and differential risk of mortality. Chronic Obstr Pulm Dis. 2019;6(5):400–413. doi: 10.15326/jcopdf.6.5.2019.0150
- Hindricks G, Potpara T, Dagres N, et al. 2020 ESC Guidelines for the diagnosis and management of atrial fibrillation developed in collaboration with the European Association for Cardio-Thoracic Surgery (EACTS): The Task Force for the diagnosis and management of atrial fibrillation of the European Society of Cardiology (ESC) Developed with the special contribution of the European Heart Rhythm Association (EHRA) of the ESC. Eur Heart J. 2021;42(5):373–498.
- Knuuti J, Wijns W, Saraste A, et al. 2019 ESC Guidelines for the diagnosis and management of chronic coronary syndromes. Eur Heart J. 2020;41(3):407–477. doi: 10.1093/eurheartj/ehz425
- McDonagh TA, Metra M, Adamo M, et al. 2021 ESC Guidelines for the diagnosis and treatment of acute and chronic heart failure. Eur Heart J. 2021;42(36):3599–3726. doi: 10.1093/eurheartj/ehab368
- Heidbuchel H, Van Gelder IC, Desteghe L, et al. ESC and EHRA lead a path towards integrated care for multimorbid atrial fibrillation patients: the Horizon 2020 EHRA-PATHS project. European Heart Journal. 2022;43(15):1450–1452. doi: 10.1093/eurheartj/ehab672
- Lee G, Baker E, Collins R, et al. The challenge of managing multimorbid atrial fibrillation: a pan-European European Heart Rhythm Association (EHRA) member survey of current management practices and clinical priorities. EP Europace. 2022;24(12):2004–2014. doi: 10.1093/europace/euac136
- Zhou J, Li X, Wang X, et al. Accuracy of portable spirometers in the diagnosis of chronic obstructive pulmonary disease a meta-analysis. NPJ Prim Care Respir Med. 2022;32(1):15. doi: 10.1038/s41533-022-00275-x
- Ioannides AE, Tayal U, Quint JK. Spirometry in atrial fibrillation: What’s the catch? Expert Rev Respir Med. Published online Nov 8, 2023;17(10):937–950. doi: 10.1080/17476348.2023.2279236
- Chase SC, Fermoyle CC, Wheatley CM, et al. The effect of diuresis on extravascular lung water and pulmonary function in acute decompensated heart failure. ESC Heart Fail. 2018;5(2):364–371. doi: 10.1002/ehf2.12253
- Iversen KK, Kjaergaard J, Akkan D, et al. Chronic obstructive pulmonary disease in patients admitted with heart failure. J Intern Med. 2008;264(4):361–369. doi: 10.1111/j.1365-2796.2008.01975.x
- Güder G, Brenner S, Störk S, et al. Chronic obstructive pulmonary disease in heart failure: accurate diagnosis and treatment. Eur J Heart Fail. 2014;16(12):1273–1282. doi: 10.1002/ejhf.183
- Magnussen H, Canepa M, Zambito PE, et al. What can we learn from pulmonary function testing in heart failure? Eur J Heart Fail. 2017;19(10):1222–1229. doi: 10.1002/ejhf.946
- Contini M, Conte E, Agostoni P. Lung function evaluation in heart failure: possible pitfalls. Breathe. 2020;16(1):190316. doi: 10.1183/20734735.0316-2019
- Roversi S, Fabbri LM, Sin DD, et al. Chronic obstructive pulmonary disease and cardiac diseases. An urgent need for Integrated Care. Am J Respir Crit Care Med. 2016;194(11):1319–1336. doi: 10.1164/rccm.201604-0690SO
- Smith JR, Van Iterson EH, Johnson BD, et al. Exercise ventilatory inefficiency in heart failure and chronic obstructive pulmonary disease. Int J Cardiol. 2019;274:232–236. doi: 10.1016/j.ijcard.2018.09.007
- Au Yeung SL, Borges MC, Lawlor DA, et al. Impact of lung function on cardiovascular diseases and cardiovascular risk factors: a two sample bidirectional Mendelian randomisation study. Thorax. 2022;77(2):164–171. doi: 10.1136/thoraxjnl-2020-215600
- Ramalho SHR, Claggett BL, Washko GR, et al. Association of pulmonary function with late-life cardiac function and Heart failure risk: the ARIC study. J Am Heart Assoc. 2022;11(14):e023990. doi: 10.1161/JAHA.121.023990
- Johnson LSB, Juhlin T, Engström G, et al. Reduced forced expiratory volume is associated with increased incidence of atrial fibrillation: the Malmo Preventive Project. Europace. 2014;16(2):182–188. doi: 10.1093/europace/eut255
- Noubiap JJ, Tu SJ, Emami M, et al. Incident atrial fibrillation in relation to ventilatory parameters: a prospective cohort study. Can J Cardiol. 2023;39(5):614–622. doi: 10.1016/j.cjca.2023.02.004
- Cuttica MJ, Colangelo LA, Shah SJ, et al. Loss of lung health from young adulthood and cardiac phenotypes in middle age. Am J Respir Crit Care Med. 2015;192(1):76–85. doi: 10.1164/rccm.201501-0116OC
- Verbrugge FH, Guazzi M, Testani JM, et al. Altered hemodynamics and End-Organ Damage in Heart failure: impact on the lung and kidney. Circulation. 2020;142(10):998–1012. doi: 10.1161/CIRCULATIONAHA.119.045409
- Guazzi M, Wilhelm M, Halle M, et al. Exercise testing in heart failure with preserved ejection fraction: an appraisal through diagnosis, pathophysiology and therapy - a clinical consensus statement of the Heart Failure Association and European Association of Preventive Cardiology of the European Society of Cardiology. Eur J Heart Fail. 2022;24(8):1327–1345. doi: 10.1002/ejhf.2601
- van der Velden RMJ, Hereijgers MJM, Arman N, et al. Implementation of a screening and management pathway for chronic obstructive pulmonary disease in patients with atrial fibrillation. Europace. 2023;25(7). doi: 10.1093/europace/euad193
- Vestbo J, Anderson JA, Brook RD, et al. Fluticasone furoate and vilanterol and survival in chronic obstructive pulmonary disease with heightened cardiovascular risk (SUMMIT): a double-blind randomised controlled trial. Lancet. 2016;387(10030):1817–1826. doi: 10.1016/S0140-6736(16)30069-1
- Rabe KF, Martinez FJ, Ferguson GT, et al. Triple Inhaled Therapy at Two Glucocorticoid Doses in Moderate-to-Very-Severe COPD. N Engl J Med. 2020;383(1):35–48. doi: 10.1056/NEJMoa1916046
- Lipson DA, Barnhart F, Brealey N, et al. Once-Daily Single-Inhaler Triple versus Dual Therapy in Patients with COPD. N Engl J Med. 2018;378(18):1671–1680. doi: 10.1056/NEJMoa1713901
- Martinez FJ, Rabe KF, Ferguson GT, et al. Reduced all-cause mortality in the ETHOS trial of budesonide/glycopyrrolate/formoterol for chronic obstructive pulmonary disease. a randomized, double-blind, multicenter, parallel-group study. Am J Respir Crit Care Med. 2021;203(5):553–564. doi: 10.1164/rccm.202006-2618OC
- Singh N, Dorfmüller P, Shlobin OA, et al. Group 3 pulmonary hypertension: from bench to bedside. Circ Res. 2022;130(9):1404–1422. doi: 10.1161/CIRCRESAHA.121.319970
- Goudie AR, Lipworth BJ, Hopkinson PJ, et al. Tadalafil in patients with chronic obstructive pulmonary disease: a randomised, double-blind, parallel-group, placebo-controlled trial. Lancet Respir Med. 2014;2(4):293–300. doi: 10.1016/S2213-2600(14)70013-X
- Wells JM, Washko GR, Han MK, et al. Pulmonary arterial enlargement and acute exacerbations of COPD. N Engl J Med. 2012;367(10):913–921. doi: 10.1056/NEJMoa1203830
- Aldabayan YS, Ridsdale HA, Alrajeh AM, et al. Pulmonary rehabilitation, physical activity and aortic stiffness in COPD. Respir Res. 2019;20(1):166. doi: 10.1186/s12931-019-1135-6
- Vanfleteren LEGW, Spruit MA, Groenen MTJ, et al. Arterial stiffness in patients with COPD: the role of systemic inflammation and the effects of pulmonary rehabilitation. Eur Respir J. 2014;43(5):1306–1315. doi: 10.1183/09031936.00169313
- Yedlapati SH, Khan SU, Talluri S, et al. Effects of influenza vaccine on Mortality and cardiovascular outcomes in patients with cardiovascular disease: a systematic review and meta-analysis. J Am Heart Assoc. 2021;10(6):e019636. doi: 10.1161/JAHA.120.019636
- Alter P, Mayerhofer BA, Kahnert K, et al. Prevalence of cardiac comorbidities, and their underdetection and contribution to exertional symptoms in COPD: results from the COSYCONET cohort. Int J Chron Obstruct Pulmon Dis. 2019;14:2163–2172. doi:10.2147/COPD.S209343.
- Blamoun AI, Batty GN, DeBari VA, et al. Statins may reduce episodes of exacerbation and the requirement for intubation in patients with COPD: evidence from a retrospective cohort study. Int J Clin Pract. 2008;62(9):1373–1378. doi: 10.1111/j.1742-1241.2008.01731.x
- Dransfield MT, Voelker H, Bhatt SP, et al. Metoprolol for the Prevention of Acute Exacerbations of COPD. N Engl J Med. 2019;381(24):2304–2314. doi: 10.1056/NEJMoa1908142
- Criner GJ, Connett JE, Aaron SD, et al. Simvastatin for the prevention of exacerbations in moderate-to-severe COPD. N Engl J Med. 2014;370(23):2201–2210. doi: 10.1056/NEJMoa1403086
- Pradhan R, Lu S, Yin H, et al. Novel antihyperglycaemic drugs and prevention of chronic obstructive pulmonary disease exacerbations among patients with type 2 diabetes: population based cohort study. BMJ. 2022;379:e071380. doi: 10.1136/bmj-2022-071380
- Mancini GBJ, Etminan M, Zhang B, et al. Reduction of morbidity and mortality by statins, angiotensin-converting enzyme inhibitors, and angiotensin receptor blockers in patients with chronic obstructive pulmonary disease. J Am Coll Cardiol. 2006;47(12):2554–2560. doi: 10.1016/j.jacc.2006.04.039
- Fawzy A, Putcha N, Aaron CP, et al. Aspirin use and respiratory morbidity in COPD: a propensity score-matched analysis in subpopulations and intermediate outcome measures in COPD study. Chest. 2019;155(3):519–527. doi: 10.1016/j.chest.2018.11.028
- Kunadian V, Wilson N, Stocken DD, et al. Antiplatelet therapy in the primary prevention of cardiovascular disease in patients with chronic obstructive pulmonary disease: a randomised controlled proof-of-concept trial. ERJ Open Res. 2019;5(3):00110–2019. doi: 10.1183/23120541.00110-2019
- Kunisaki KM, Dransfield MT, Anderson JA, et al. Exacerbations of chronic obstructive pulmonary disease and cardiac events. A post hoc cohort analysis from the SUMMIT Randomized Clinical Trial. Am J Respir Crit Care Med. 2018;198(1):51–57. doi: 10.1164/rccm.201711-2239OC
- Reilev M, Pottegård A, Lykkegaard J, et al. Increased risk of major adverse cardiac events following the onset of acute exacerbations of COPD. Respirology. 2019;24(12):1183–1190. doi: 10.1111/resp.13620
- Nordon C, Rhodes K, Quint JK, et al. Exacerbations of COPD and their OutcomeS on CardioVascular diseases (EXACOS-CV) Programme: protocol of multicountry observational cohort studies. BMJ Open. 2023;13(4):e070022. doi: 10.1136/bmjopen-2022-070022
- Vogelmeier C Increased risk of severe cardiovascular events following exacerbations of COPD: a multi-database cohort study. Poster PA3013. Published online 2023.
- Wells JM, Criner GJ, Halpin DMG, et al. Mortality risk and serious cardiopulmonary events in moderate-to-severe COPD: Post Hoc analysis of the IMPACT trial. Chronic Obstr Pulm Dis. 2023;10(1):33–45. doi: 10.15326/jcopdf.2022.0332
- Papi A. Pathophysiology of exacerbations of chronic obstructive pulmonary disease. Proc Am Thorac Soc. 2006;3(3):245–251. doi: 10.1513/pats.200512-125SF
- Musher DM, Abers MS, Corrales-Medina VF, et al. Acute Infection and Myocardial Infarction. N Engl J Med. 2019;380(2):171–176. doi: 10.1056/NEJMra1808137
- Skaarup KG, Modin D, Nielsen L, et al. Influenza and cardiovascular disease pathophysiology: strings attached. Eur Heart J Suppl. 2023;25(Suppl A):A5–A11. doi: 10.1093/eurheartjsupp/suac117
- Polosa R, Malerba M, Cacciola RR, et al. Effect of acute exacerbations on circulating endothelial, clotting and fibrinolytic markers in COPD patients. Intern Emerg Med. 2013;8(7):567–574. doi: 10.1007/s11739-011-0636-1
- van der Vorm LN, Li L, Huskens D, et al. Acute exacerbations of COPD are associated with a prothrombotic state through platelet-monocyte complexes, endothelial activation and increased thrombin generation. Respir med. 2020;171:106094. doi: 10.1016/j.rmed.2020.106094
- Widlansky ME, Gokce N, Keaney JF, et al. The clinical implications of endothelial dysfunction. J Am Coll Cardiol. 2003;42(7):1149–1160. doi: 10.1016/s0735-1097(03)00994-x
- O’Donnell DE. COPD exacerbations. 3: Pathophysiology. Thorax. 2006;61(4):354–361. doi: 10.1136/thx.2005.041830
- Verhoeff K, Mitchell JR. Cardiopulmonary physiology: why the heart and lungs are inextricably linked. Adv Physiol Educ. 2017;41(3):348–353. doi: 10.1152/advan.00190.2016
- Cheyne WS, Williams AM, Harper MI, et al. Heart-lung interaction in a model of COPD: importance of lung volume and direct ventricular interaction. Am J Physiol Heart Circ Physiol. 2016;311(6):H1367–H1374. doi: 10.1152/ajpheart.00458.2016
- Spiesshoefer J, Regmi B, Ottaviani MM, et al. Sympathetic and vagal nerve activity in COPD: pathophysiology, presumed determinants and underappreciated therapeutic potential. Front Physiol. 2022;13:919422. doi: 10.3389/fphys.2022.919422
- Viglino D, Maltais F, Tamisier R. Common pathophysiological pathways of the autonomic nervous system. In: Cardiovascular Complications of Respiratory DisordersVol. 2020. European Respiratory Society; p. 12–30. 10.1183/2312508X.10027119
- Elhage S, Laurent L, Diallo K, et al. Effects of long-acting bronchodilators on cardiac autonomic control in COPD. Respir Med Res. 2022;82:100968. doi: 10.1016/j.resmer.2022.100968
- Ferguson GT, Skärby T, Nordenmark LH, et al. Unreported and overlooked: a post hoc analysis of COPD symptom-related attacks from the RISE study. Int J Chron Obstruct Pulmon Dis. 2020;15:3123–3134. doi:10.2147/COPD.S277147.
- Stevenson IH, Roberts-Thomson KC, Kistler PM, et al. Atrial electrophysiology is altered by acute hypercapnia but not hypoxemia: implications for promotion of atrial fibrillation in pulmonary disease and sleep apnea. Heart Rhythm. 2010;7(9):1263–1270. doi: 10.1016/j.hrthm.2010.03.020
- Corrales-Medina VF, Alvarez KN, Weissfeld LA, et al. Association between hospitalization for pneumonia and subsequent risk of cardiovascular disease. JAMA. 2015;313(3):264–274. doi: 10.1001/jama.2014.18229
- Chang C, Guo Z, Shen N, et al. Dynamics of inflammation resolution and symptom recovery during AECOPD treatment. Sci Rep. 2014;4(1):5516. doi: 10.1038/srep05516
- Kyriakopoulos C, Gogali A, Kostikas K, et al. Hypercoagulable State in COPD-A Comprehensive Literature Review. Diagn (Basel). 2021;11(8):1447. doi: 10.3390/diagnostics11081447
- Wageck B, Cox NS, Holland AE. Recovery Following Acute Exacerbations of Chronic Obstructive Pulmonary Disease - a Review. COPD: J Chronic Obstructive Pulmonary Dis. 2019;16(1):93–103. doi: 10.1080/15412555.2019.1598965
- Patel ARC, Kowlessar BS, Donaldson GC, et al. Cardiovascular risk, myocardial injury, and exacerbations of chronic obstructive pulmonary disease. Am J Respir Crit Care Med. 2013;188(9):1091–1099. doi: 10.1164/rccm.201306-1170OC
- Khedoe PPSJ, Rensen PCN, Berbée JFP, et al. Murine models of cardiovascular comorbidity in chronic obstructive pulmonary disease. Am J Physiol Lung Cell Mol Physiol. 2016;310(11):L1011–27. doi: 10.1152/ajplung.00013.2016
- Gabriel CL, Ferguson JF. Gut microbiota and microbial metabolism in early risk of cardiometabolic disease. Circ Res. 2023;132(12):1674–1691. doi: 10.1161/CIRCRESAHA.123.322055
- Lagan J, Schelbert EB, Naish JH, et al. Mechanisms Underlying the Association of Chronic Obstructive Pulmonary Disease with Heart Failure. JACC Cardiovasc Imaging. 2021;14(10):1963–1973. doi: 10.1016/j.jcmg.2021.03.026
- Hurst JR, Gale CP, Global Working Group on Cardiopulmonary Risk. MACE in COPD: addressing cardiopulmonary risk. Lancet Respir Med. 2024 Mar 1. 10.1016/S2213-2600(24)00038-9