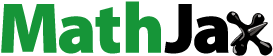
ABSTRACT
Passive solar still is a feasible and cost-effective option for the desalination of saline water in rural areas. However, its efficiency and daily yield are low. This research is carried out to improve the daily yield of the solar still by making design modifications and improve its efficiency by incorporating heat transfer enhancement mechanisms. The performance comparison of the modified and conventional solar still and its corresponding daily yield has been experimentally investigated by varying the basin water depth. The modified pyramid solar still has a unique combination of modifications such as a v-corrugated absorber surface, a nano-coated absorber surface, internal reflective mirrors and nano-embodied phase change material. The experimental test result of the system gives a daily yield of 2.95 and 5.73 l/m2 distilled water from conventional and modified solar still, respectively. This shows a yield improvement of 94.2%. Finally, the water quality is tested and drinkable as per the WHO standard.
Acronyms and nomenclatures
Symbols | = | Description |
a | = | Ambient |
CPSS | = | Conventional Pyramid Solar Still |
CuO | = | Copper Oxide |
Ex | = | Exergy rate |
g | = | Glass cover |
MPSS | = | Modified Pyramid Solar Still |
NPCM | = | Nano Phase Change Material |
p | = | Absorber plate |
PCM | = | Phase Change Material |
T | = | Temperature |
w | = | Basin water |
WHO | = | World Health Organization |
1. Introduction
Over 70% of the Earth’s surface is covered by water but most of it is not suitable for direct human consumption (Saravanan and Murugan Citation2020). However, some of these water bodies are polluted naturally due to their saline property and others are polluted by human activities. Desalination is a process for eliminating salt content from saline water (Abdelgaied et al. Citation2022). Passive solar stills are recognised as one of the most appropriate desalination techniques in rural areas. It uses solar energy to make saline water drinkable with simple thermodynamic processes (Mohsenzadeh, Aye, and Christopher Citation2021).
A single-basin single-slope solar still is the most basic type of passive solar still. This technology uses a closed device called a solar still in which water is evaporated using solar energy as a heat source and collects distilled water after condensation of the vapour while impurities are left on the base (Darbari and Rashidi Citation2022). However, the drawback of this technology is low efficiency and yield (Jobrane et al. Citation2022; Younes et al. Citation2022). A significant amount of heat energy is lost through the basin and sidewalls of the solar still to the atmosphere, which is the foremost reason behind its low performance in terms of daily yield and efficiency.
To solve this issue, many studies have been conducted to overcome the low efficiency and yield of passive solar still by making various types of design modifications. However, few works of literature have been reported on the impacts of nanoparticles and nano-embodied PCM in solar still applications. Attia et al. (Citation2022) tested the influence of top cover cooling and nanoparticle alterations on the performance of hemispherical solar stills. The study output showed that the diurnal yield and efficiency achieved by modified solar still compared to the conventional solar still was improved by 105.2% and 74.2%, respectively. Younes et al. (Citation2022) studied the impacts of rotating disks, CuO nano-enhanced paraffin wax mixed with emerging fins and reflectors on the performance of solar still. The study result shows that the daily accumulated freshwater produced from modified solar still was improved by 184%. Abdullah et al. (Citation2020) analysed the impact of using reflectors, nano-paint coating, and nano-enhanced phase change material on the performance of a single solar still. The study found that the diurnal yield of the modified solar still was improved by 108%.
Sharshir, Elkadeem, and Meng (Citation2020) studied the impacts of nanoparticles and pointed out that nanoparticles are one of the most effective methods for improving the thermal behaviour and yield of solar stills. Bait and Ameur (Citation2018) studied a comprehensive review of the enhancement of heat and mass transfer in solar stills by using nanofluids. The thermal conductivity of nanoparticles plays a significant role in the yield enrichment of solar still. Mahian et al. (Citation2017) reported that adding nanofluids to the basin of solar still can enhance the efficiency up to 50%.
Even though most of the previous research studies deal with quantitative ways of analysing the performance of solar still it is important to evaluate its performance in a qualitative way using exergy analysis to obtain the maximum possible work. Also, the effect of nanoparticles and nano-embodied PCM on the performance of pyramid solar still needs to be further investigated. Thus, the present study deals with both energy and exergy analyses of conventional and modified pyramid-type solar still with four proposed modifications (i.e. v-corrugated absorber surface, CuO nano-coating, internal reflectors and nano-enhanced phase change material) to improve the daily yield.
2. Material and methods
In comparison to carbon nanotubes and other nano-powders used to produce nanofluids, copper oxide nanoparticle is moderate in cost and also available in the local market. Even though the thermal conductivity of some nano-powders is high, they are not selected for nanofluid preparation due to their high cost. Thus, the selected CuO nano-particle is more reasonable in accordance with thermal conductivity and cost (Elango, Kannan, and Kalidasa Murugavel Citation2015). The thermophysical property of CuO nanoparticle is given in . The mass of the nanoparticle is measured and selected as per the total volume of the base fluid (Panchal et al. Citation2019). Since the nanoparticles are hydrophobic in nature, prone to agglomerate together and settle quickly (Mahian et al. Citation2017), the measured nano-powders are added to the base fluid to form the colloidal homogenous solution. The surfactant sodium dodecyl benzene sulphonate is used to maintain a stable and homogenous dispersion of the nanoparticles. The prepared samples are stirred by a magnetic stirrer for 30 min to avoid the agglomeration of nanoparticles. It is also sonicated for 1 h using an ultrasonicator for even distribution of particles in fluid base and better stability (Panchal et al. Citation2019; Thakur and Gaur Citation2022).
Table 1. Thermophysical properties of the CuO nanoparticle.
A paraffin wax, having an average melting temperature in the range of 58–59°C, has been chosen as a PCM for the thermal energy storage material due to its availability in the local market and low cost. shows the thermo-physical property of paraffin wax and nanoparticle-mixed PCM (Nano-PCM) (Rufuss et al. Citation2016). Here, CuO nanoparticle is used to enhance the thermal conductivity of the PCM.
Table 2. Thermophysical properties of paraffin wax and CuO-mixed Paraffin Wax (Nano-PCM).
2.1 Experimental set-up
The experimental investigation is carried out with two distinct pyramid-type solar stills. One is a modified pyramid solar still (MPSS) with a unique combination of effective modifications and the other is a conventional pyramid solar still (CPSS) that serves as the reference under Adama-Ethiopia climatic conditions.
An absorber plate of size 1*1 m2 is fabricated from galvanised iron sheet, and then coated with the black paint to increase the heat absorbing capacity of the absorber. The top glass cover of thickness 0.004 m is installed at the height of 0.3 m from the absorber plate. It serves as a solar radiation transmitter and a condensing surface for the water vapours generated in the basin of the still.
The glass cover is kept at the inclined angle of 30o on all four sides so that it forms a slope-like structure at all sides. Hence this is called a square pyramid-shaped solar still. Also, the glass and side walls interfaces are sealed with silicon to arrive at a leak-proof system. The sloping of the glass aids in the effective collection of the condensed water. Glass wool and wood are used to cover the outer boundary of the pyramid solar still which act as predominant thermal insulators.
2.1.1 Modified pyramid solar still
The modified solar still has incorporated four unique combinations of impactful modifications over the conventional pyramid solar still. The first modification is the use of a v-shaped corrugated absorber plate instead of the flat plate in CPSS. This is utilised to increase the surface area of the evaporation subjected to solar radiation. The basin is constructed in the form of a v-corrugated absorber surface made up of a galvanised iron sheet of size 1*1 m2 and with a height of 0.05 m. The v-corrugated square basin is mounted on a basin pyramid distiller’s main square area. The second modification is made by covering the basin with a blend of CuO nanoparticles and black paint so that the thermal conductivity and the solar radiation absorbance of the absorber surface increase. The magnetic stirring has produced a uniformly distributed mixture of nanoparticles and black enamel paint. The third modification is the addition of internal reflectors to the vertical walls of the solar still. These internal reflectors are used to direct solar radiation that passes through the transparent glass and strikes the wall of the solar still to the v-corrugated absorber plate. Thus, the evaporation rate of the saline water in the basin increases.
Finally, the fourth modification over the CPSS is made by installing energy storage under the basin to minimise the heat loss in the solar still. The paraffin wax is used as a phase change material (PCM) for latent heat storage. It is placed beneath the v-corrugated absorber to extend the evaporation time after sunset. The paraffin wax modules are first crushed into smaller pieces and based on the recommendations from Rufuss et al. (Citation2016) regarding the blending of nano-composites, the paraffin wax is heated to a temperature above the melting point followed by the addition of the nanoparticles. The mixtures are then sonicated for 1 h at 40 kHz. Thus, aggregation and settling of nanoparticles in the PCM is avoided. Finally, the liquid PCM mixture is poured into the storage beneath the v-corrugated absorber. A photographic view of the experimental set-up is shown in .
2.2 Measuring instruments
The solar radiation data are taken from the National Metrological Agency, Adama-Ethiopia due to the lack of radiation measuring instruments on the site. Ambient temperature, absorber plate temperature, basin water temperature, glass cover temperature and the PCM temperature are measured using a K-type thermocouple and digital thermometer. The instruments are calibrated using primary instruments available in the Mechanical Engineering department laboratory of Adama Science and Technology University, Adama-Ethiopia. The associated percentage errors, accuracies, and working ranges of the instruments are summarised in .
Table 3. Accuracy and error for various measuring instruments.
3. System performance
The performance of the solar still is analysed by assessing the thermal efficiency of the system. The total incident solar radiation on an inclined surface (It) is the sum of the beam (Ib), diffuse (Id) and reflected solar radiation from a horizontal surface and surrounding it (Bekele, Alemu, and Mishra Citation2013; Mahian et al. Citation2017).
where Zenith angle,
The angle of incidence,
is the surface slope angle, and
g is the ground reflectivity (0.2 for bare earth and 0.7 for snow).
3.1 Energy efficiency
The thermal efficiency of solar still can be computed as the ratio of evaporative heat transfer to the incident solar irradiation on the absorber plate and it is given by Shoeibi, Kargarsharifabad, and Rahbar (Citation2021)
where the latent heat of vaporisation (hfg) of water can be estimated as (Yousef, Hassan, and Sekiguchi Citation2019) follows:
for
for
3.2 Exergy efficiency
The exergy efficiency of the solar still is defined as the ratio of the evaporative exergy to the net input sun exergy. It can be written as (Shoeibi, Kargarsharifabad, and Rahbar Citation2021; Yousef, Hassan, and Sekiguchi Citation2019)
Exergy evaporation is calculated as follows (Abdelgaied et al. Citation2022)
In a solar still, exergy input can be analysed based on the radiation entering the basin (Sharshir et al. Citation2018).
where Ta is the ambient temperature and Ts is the temperature of the sun.
4. Results and discussions
A series of experimental observations have been conducted on solar stills using CPSS and MPSS at Adama Science and Technology University, Adama-Ethiopia in May 2022. The experiments are carried out for seven consecutive days starting from 9:00 AM to 4:00 PM. The ambient temperature and monthly average hourly incident global solar radiation for May corresponding to the local time is plotted, as shown in . It is observed that the ambient temperature has varied from about 25.3°C to 34.8°C during the experimental observations. The observed average maximum and minimum intensity of the solar insolation obtained is 770 and 300 W/m2 at 12:00 and 16:00 h, respectively.
4.1 Varying the levels of the water
The experimental analysis of conventional and modified pyramid solar still is carried out at 1, 2, and 3 cm depth levels of the water. The obtained results are recorded at every 30-minute interval.
4.1.1 Hourly variation of absorber plate temperatures
The absorber plate temperature of CPSS and MPSS observed during experimental analyses is shown in . It is observed that for 1 cm saline water depth, the absorber plate temperature of CPSS has increased from about 32°C to 64°C with an increase in solar irradiation from morning to 12:30 h. For 3 cm saline water depth, the temperature of CPSS has increased from 28°C to 61°C till 13:30 h. Whereas, for a 1 cm saline water depth, the absorber plate temperature of MPSS increased from about 33°C to 73°C till 13:00 h and for a 3 cm saline water depth, the temperature of MPSS increased from about 29°C to 68°C till 13:30 h. Once the basin reached the peak, then the temperature of the basin in CPSS falls rapidly. While the MPSS has relatively controlled the temperature drop in the afternoon and maintained a higher temperature in the basin.
4.1.2 Hourly variation of basin water temperature
The hourly variations of saline water temperature operating under CPSS and MPSS are shown in . The saline water temperatures of CPSS at 1 and 3 cm water depth varied from 29°C to 61°C and 26°C to 57°C till 13:00 and 14:00 h, respectively. Whereas in the case of MPSS, the maximum basin water temperatures at 1 and 3 cm saline water depth are found at 68°C and 63°C, at 12:30 and 14:00 h, respectively. Hence, the temperature of saline water in the basin of modified pyramid solar still is higher than the conventional one, this is due to the increase of heat transfer surface area as a result of v-corrugation of the absorber plate and the enhanced thermal conductivity of the absorber plate due to the effect of nanoparticle coated black painting. Also, there is an effect of internal reflective surfaces in enhancing the solar radiation striking the absorber plate. In the afternoon when the solar radiation continues to decrease, the temperature of the saline water in MPSS maintained a higher water temperature in the basin due to the migration of thermal energy from the PCM to the absorber surface. This enhances the daily yield of the solar still. It is clearly noticed that the lowest basin water depth attains the highest water temperature and it decreases with an increase in basin water depth. This is due to the high thermal inertia requirement of the higher depth of water level i.e. since the thermal energy is distributed over a larger amount of mass of water, the evaporation rate decreases with an increase in water depth.
4.1.3 Hourly variation of glass cover temperatures
The glass cover temperatures of CPSS and MPSS have been compared, as shown in . In the case of CPSS, the maximum values of glass cover temperature of 48°C and 44°C are obtained at 1 and 3 cm depth of saline water at 13:00 and 14:00 h, respectively. In the case of MPSS, the maximum values of glass cover temperatures are obtained as 52°C and 48°C at 1 and 3 cm depth of saline water at 13:00 and 13:30 h, respectively. In the morning time, a steep increase in glass temperature has been observed. However, the glass cover has attained the maximum steady-state temperature at 14:00 h. After that, the glass cover temperature falls quickly up to 17:00 h. This is due to the decrease in the solar radiation intensity on the absorber surface.
4.1.4 Hourly variation of PCM temperatures
The average variation of the PCM temperature of MPSS is shown in . As the solar intensity and ambient temperature increase, the temperature of phase change material also increases gradually up to noon time. At the initial time of the experiment, the phase change material absorbs heat from the absorber plate of the solar still (i.e. charging phase), and its temperature is increased slowly. During this process, the absorbed thermal energy from the absorber plate is stored as sensible heat.
The charging process of the PCM begins with an increase in PCM temperature and attains its melting point between 10:30 and 13:00, 11:00 and 13:30 and 11:30 and 14:00 h at a water depth of 1, 2 and 3 cm, respectively. This variation of time is because of the effect of basin water depth. During this process, the solid phase of PCM is altered to the liquid phase. Furthermore, when the phase change material temperature attains its melting temperature, the melting process is completed. Later the discharging process begins when the solar intensity and ambient temperature start to decline which causes the absorber plate temperature to drop below the PCM temperature and heat flows from the PCM to the basin water through the absorber plate. Consequently, the temperature of PCM declines with time up to achieving the solidification temperature at around 14:00 h and the solidification rate is low. After 17:00 h, the temperature of the phase change material continues to decline up to the ambient temperature by losing the sensible heat until it reaches thermal equilibrium with the environment.
The most important observation is that the average rise in temperatures of basin water for both CPSS and MPSS is nearly similar during the charging time, but there is a significant difference during the discharging time. This is due to the heat released to the saline water from the PCM during the discharging time as a result the basin water temperature of the MPSS is higher than that of the CPSS.
4.1.5 Comparison of the cumulative yield for different depths of basin water
shows the cumulative yield of CPSS and MPSS at basin water depths of 1, 2 and 3 cm. For the case of CPSS, the total daily cumulative yield obtained per m2 absorber plate area at the basin water depth of 1, 2 and 3 cm are 2.95, 2.763 and 2.443 l/m2, respectively. There is an increase of 21% daily yield for the basin water depth of 1 cm and a 13% increase for the basin water depth of 2 cm, compared with the basin water depth of 3 cm.
For the case of MPSS, the total daily cumulative yield obtained per m2 absorber plate area for the basin water depth of 1, 2 and 3 cm are 5.728, 4.905 and 4.389 l/m2, respectively. That is, there is an increase of 31% yield for the basin water depth of 1 cm and a 12% increase for the basin water depth of 2 cm, compared with the basin water depth of 3 cm.
From it is observed that the daily cumulative yield is higher for the basin water depth of 1 cm and the yield is lesser for the basin water depth of 3 cm. This is because the higher rate of evaporation of the water in the basin varies with the depth of the saline water in the still, which is similar to the earlier work reported by Saravanan and Murugan (Citation2020).
4.2 Energy and exergy efficiency
shows the comparison of the daily average thermal efficiency of CPSS and MPSS. The daily thermal efficiency of CPSS obtained at 1, 2 and 3 cm basin water depth is 43.90%, 41.29% and 36.67%, respectively. While, the average daily thermal efficiencies of the MPSS at 1, 2 and 3 cm basin water depth are 63.72%, 54.53% and 47.65%, respectively. In both cases the maximum and minimum values of daily thermal efficiency are obtained at 1 and 3 cm basin water depth, respectively. This also proves that the daily thermal efficiency decreases as the basin water depth increases. The reason behind this is that the water temperature quickly rises for a lower basin water depth due to the minimum volume of water and gives a higher amount of yield. The proposed modifications have improved the thermal efficiency of a solar still, which is similar to the earlier research works reported by Sharshir, Elkadeem, and Meng et al. (Citation2020) and Younes et al. (Citation2022).
4.3 Exergy efficiency
The exergy efficiency of CPSS and MPSS observed during experimental observations is shown in . The exergy performance is estimated to quantify the losses in solar still. The average daily exergy efficiencies of a CPSS at 1, 2 and 3 cm basin water depth are about 2.2%, 2.0% and 1.8%, respectively. The average daily exergy efficiencies of an MPSS obtained are 3.7%, 3.6% and 3.4% at 1, 2 and 3 cm basin water depth, respectively. The maximum daily exergy is observed at 1 cm basin water depth, which is an optimal water depth to produce maximum yield. The daily exergy efficiency obtained is similar to the earlier research study reported by Sharshir et al. (Citation2018).
4.4 Comparison of the CPSS and MPSS
is a summary of the average values of the daily cumulative yield and daily thermal and exergy efficiency of the CPSS and MPSS. The average daily cumulative distillate water yield during the experimental observations achieved by the MPSS reached 5.73 l/m2/day compared to 2.95 l/m2/day achieved by CPSS representing a 94.2% average enhancement in accumulative yield.
Table 4. Daily average yield, thermal and exergy efficiency of CPSS and MPSS.
The average daily thermal efficiency during the experimental observations of CPSS reached 43.90% while adding the incorporated effective four modifications on the design of MPSS has enhanced the average daily thermal efficiency to 63.72% which is an improvement of 45.1%. Also, the average daily exergy efficiency during the experimental observations of CPSS reached 2.2%, while adding the incorporated effective four modifications on the design of MPSS has enhanced the average daily exergy efficiency to 3.7% which is a 68.2% improvement in daily exergy efficiency.
Based on the above results, utilisation of the four hybrid design modifications is effective in achieving the highest performance of a modified solar distiller. The results obtained by incorporation of modifications in the present study are in good agreement with earlier research work reported by Abdullah et al. (Citation2020).
shows a comparison between daily yield values obtained by other researchers and the present work. Here, it is observed that the present work provides a better daily distilled water yield from the solar still than previous research works. shows the physio-chemical analysis of saline and distilled water. The solar still reduces most of the impurities well below the standards set by WHO. The water quality test done for distilled water shows it is in good agreement with WHO limits and the obtained purified water is suitable for drinking purposes and other applications. Likewise, the water quality test results of the present study are in good agreement with an earlier research work (Jobrane et al. Citation2022).
Table 5. Comparison of the present work with earlier research works.
Table 6. Water quality analysis of saline and distilled water.
5. Conclusions
This study has experimentally investigated the performance of modified passive solar still using nanoparticle-coated absorber plate and nanoparticle-mixed PCM for desalinating saline water. The experimentation is carried out with two distinct pyramid-type solar stills: one is a modified pyramid solar still and the other is a conventional pyramid solar still that serves as the reference for comparison. The following conclusions are drawn from the experimental test result of the pyramid-type solar still:
▪ The daily average yield of distilled water obtained from CPSS is 2.95 l/m2 and from that of MPSS is 5.73 l/m2. This shows a daily average yield improvement of 94.2% due to the incorporation of modifications made.
▪ The daily average thermal efficiency of the CPSS and MPSS is 43.9 and 63.72%, respectively. This shows a thermal efficiency enhancement of 45.1%. Similarly, the daily average exergy efficiency of the CPSS and MPSS is 2.2 and 3.7%, respectively. This shows an exergy efficiency improvement of 68.2%.
▪ The use of nano-particle mixed with PCM as thermal energy storage in the pyramid-type solar still has favoured the evaporation to sustain in the afternoon when the solar radiation intensity has decreased. Hence it has a positive contribution to the reduction of useful energy loss and the improvement of daily yield.
▪ The distilled water quality test has been done and it shows a good agreement with WHO limits hence, the obtained distilled water is suitable for drinking.
Disclosure statement
No potential conflict of interest was reported by the author(s).
References
- Abdelgaied, M., A. S. Abdulla, G. B. Abdelaziz, and A. E. Kabeel. 2022. “Performance Improvement of Modified Stepped Solar Distillers Using Three Effective Hybrid Optimization Modifications.” Sustainable Energy Technologies and Assessments 51: 101942. doi:10.1016/j.seta.2021.101936
- Abdullah, A. S., F. A. Essa, H. Ben Bacha, and Z. M. Omara. 2020. “Improving the Trays Solar Still Performance Using Reflectors and Phase Change Material With Nanoparticles.” Journal of Energy Storage 31: 101744. doi:10.1016/j.est.2020.101744
- Attia, M. E. H., A. E. Kabeel, E. Elaloui, M. Abdelgaied, and A. Abdullah. 2022. “Experimental Study on Improving the Yield of Hemispherical Distillers Using CuO Nanoparticles and Cooling the Glass Cover.” Solar Energy Materials and Solar Cells 235: 111482. doi:10.1016/j.solmat.2021.111482
- Bait, O., and S. M. Ameur. 2018. “Enhanced Heat and Mass Transfer in Solar Stills Using Nanofluids: A Review.” Solar Energy 170: 694–722. doi:10.1016/j.solener.2018.06.020
- Bekele, A., D. Alemu, and M. Mishra. 2013. “Large-Scale Solar Water Heating Systems Analysis in Ethiopia: A Case Study.” International Journal of Sustainable Energy 32 (4): 207–228. doi:10.1080/14786451.2011.605951
- Darbari, B., and S. Rashidi. 2022. “Performance Analysis for Single Slope Solar Still Enhanced with Multi-Shaped Floating Porous Absorber.” Sustainable Energy Technologies and Assessments 50: 101854. doi:10.1016/j.seta.2021.101854
- Elango, T., A. Kannan, and K. Kalidasa Murugavel. 2015. “Performance Study on Single Basin Single Slope Solar Still with Different Water Nanofluids.” Desalination 360: 45–51. doi:10.1016/j.desal.2015.01.004
- Jobrane, M., A. Kopmeier, A. Kahn, H. M. Cauchie, A. Kharroubi, and C. Penny. 2022. “Theoretical and Experimental Investigation on a Novel Design of Wick Type Solar Still for Sustainable Freshwater Production.” Applied Thermal Engineering 200: 117648–117662. doi:10.1016/j.applthermaleng.2021.117648
- Kianifar, A., S. Zeinali Heris, and O. Mahian. 2012. “Exergy and Economic Analysis of a Pyramid-Shaped Solar Water Purification System: Active and Passive Cases.” Energy 38 (1): 31–36. doi:10.1016/j.energy.2011.12.046
- Mahian, O., A. Kianifar, S. Z. Heris, D. Wen, A. Z. Sahin, and S. Wongwises. 2017. “Nanofluids Effects on the Evaporation Rate in a Solar Still Equipped with a Heat Exchanger.” Nano Energy 36: 134–155. doi:10.1016/j.nanoen.2017.04.025
- Mohsenzadeh, M., L. Aye, and P. Christopher. 2021. “A Review on Various Designs for Performance Improvement of Passive Solar Stills for Remote Areas.” Solar Energy 228: 594–611. doi:10.1016/j.solener.2021.09.086
- Panchal, H., R. Sathyamurthy, A. E. Kabeel, S. A. El-Agouz, Ds.S. Rufus, T. Arunkumar, M. A. Manokar, et al. 2019. “Annual Performance Analysis of Adding Different Nanofluids in Stepped Solar Still.” Journal of Thermal Analysis and Calorimetry 138 (5): 3175–3182. doi:10.1007/s10973-019-08346-x
- Rufuss, D. D. W., S. Iniyan, L. Suganthi, and P. A. Davies. 2016. “Solar Stills: A Comprehensive Review of Designs, Performance and Material Advances.” Renewable and Sustainable Energy Reviews 63: 464–496. doi:10.1016/j.rser.2016.05.068
- Saravanan, A., and M. Murugan. 2020. “Performance Evaluation of Square Pyramid Solar Still with Various Vertical Wick Materials: An Experimental Approach.” Thermal Science and Engineering Progress 19: 1–9. doi:10.1016/j.tsep.2020.100581
- Sharshir, S. W., M. R. Elkadeem, and A. Meng. 2020. “Performance Enhancement of Pyramid Solar Distiller Using Nanofluid Integrated With v-Corrugated Absorber and Wick: An Experimental Study.” Applied Thermal Engineering 168: 114848. doi:10.1016/j.applthermaleng.2019.114848
- Sharshir, S. W., G. Peng, A. H. Elsheikh, E. M. A. Edreis, M. A. Eltawil, T. Abdelhamid, A. E. Kabeel, J. Zang, and N. Yang. 2018. “Energy and Exergy Analysis of Solar Stills With Micro/Nano Particles: A Comparative Study.” Energy Conversion and Management 177: 363–375. doi:10.1016/j.enconman.2018.09.074
- Shoeibi, S., H. Kargarsharifabad, and N. Rahbar. 2021. “Effects of Nano-Enhanced Phase Change Material and Nano-Coated on the Performance of Solar Stills.” Journal of Energy Storage 42: 103061(1–17). doi:10.1016/j.est.2021.103061
- Thakur, V. K., and M. K. Gaur. 2022. “Study the Effect of CuO Nanoparticles on the Performance of Passive Solar Still in Winter and Summer Season.” Materials Today: Proceedings 57 (5): 2009–2017. doi:10.1016/j.matpr.2021.11.119
- Younes, M. M., A. S. Abdullah, Z. M. Omara, and F. A. Essa. 2022. “Enhancement of Discs’ Solar Still Performance Using Thermal Energy Storage Unit and Reflectors: An Experimental Approach.” Alexandria Engineering Journal 61 (10): 7477–7487. doi:10.1016/j.aej.2022.01.001
- Yousef, M. S., H. Hassan, and H. Sekiguchi. 2019. “Energy, Exergy, Economic and Enviro-Economic Analyses of Solar Distillation System Using Different Absorbing Materials.” Applied Thermal Engineering 150: 30–41. doi:10.1016/j.applthermaleng.2019.01.005