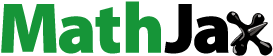
Abstract
Objective: Design an optimised auditory azimuth localisation training protocol by: (1) differing the number of training stimuli presentations to determine the highest angular accuracy achieved in the fewest training sessions, (2) evaluating the presence of training transfer from a broadband stimulus to untrained military-relevant stimuli and (3) measuring the effect of differing training strategies on localisation accuracy.
Design: Two pilot studies of 18 and six subjects, respectively, determined the number of presentations and type of stimuli to be included in a localisation training experiment that evaluated different training strategies. In the pilot studies, participants trained using eight Learning Units (LU) and then tested using untrained stimuli. In the main experiment, 40 participants completed eight LUs using four different learning strategies, with 10 participants randomly assigned to each condition.
Sample: All participants were ages 18–42 normal-hearing listeners with thresholds not exceeding 25 dB HL and asymmetry not exceeding 15 dB HL.
Results: Transfer of training occurred using three of the four untrained stimuli. The training strategy that resulted in the highest localisation accuracy involved active participation.
Conclusions: Training with a broadband stimulus transfers to untrained broadband stimuli. Training involving the active participation strategy resulted in the greatest localisation accuracy.
This article was based on a presentation at the 2019 Annual Conference of the National Hearing Conservation Association, and is part of the 10th dedicated supplement to hearing loss prevention
Introduction
Noise remains a ubiquitous threat to U.S. military service members. Noise and subsequent noise-induced hearing loss can disrupt verbal and nonverbal cues critical for understanding the operational environment. The rates of hearing loss within the military illustrate the adverse effects of hazardous noise. In 2016, the prevalence of significant hearing loss among soldiers in the U.S. Army was 24% (DOEHRS-DR Citation2016). The prevalence of noise-induced hearing loss in the civilian sector was 12.94% from 2003 to 2012, nearly half the rate reported in soldiers (Matterson et al. Citation2016). Those who served in the military were found to have a 30% greater likelihood of having severe hearing impairment compared to nonveteran counterparts (Groenwold, Tak, and Matterson Citation2011). The pervasive, and yet sometimes unpredictable, presence of noise in a service member’s environment may partially explain the discrepancy in civilian versus military hearing loss rates. In the midst of these varied noise sources, service members must maintain the ability to auditorily detect, recognise, localise, react and communicate (Tufts, Vasil, and Briggs Citation2009). In other words, service members must maintain auditory situation awareness. In fact, service members often encounter a trade-off between wearing hearing protection that can potentially disrupt auditory situation awareness (as many devices do) or not wear hearing protection at the expense of sudden and/or permanent hearing loss (Casali and Lee Citation2019). Both hearing loss and hearing protection use can present risks to the service member as they can diminish access to key situation awareness cues.
The effects of hearing protection devices on situation awareness have been studied extensively within the Virginia Tech Auditory Systems Laboratory. Certain types of hearing protection, including level-dependent passive and active (electronic battery-powered) systems, can impede detection and subsequent recognition of certain sounds, including those in civilian employment situations (Alali and Casali Citation2012; Clasing and Casali Citation2014). A larger body of literature exists on the many variables, including hearing protection, which have been investigated in spatial hearing experiments for humans; many of these can be found in Cave (Citation2019). A review of the various types and functionality of the level-dependent protectors that are discussed herein appears in Casali (Citation2010a, Citation2010b). In regards to localisation, listeners’ ability to localise back-up alarms in hazardous listening environments remained intact with certain passive hearing protectors (Alali and Casali Citation2011). However, in a weapon-related (i.e. gunfire) localisation task, both localisation accuracy and response time were significantly worse with certain active (electronic) protectors compared to the unprotected ear in varying levels of noise, with particularly poor performance when using a standard Army-issue pass-through earmuff (Talcott et al. Citation2012). Therefore, hearing protection can have differing effects on localisation according to its design, ambient noise levels and content of the signal (Alali and Casali Citation2011; Talcott et al. Citation2012). Promisingly, localisation accuracy using certain active hearing protectors can improve with training to achieve performance comparable to that of the open ear (Casali and Robinette Citation2015); however, training benefits do not necessarily cross over (i.e. transfer) between circumaural (earmuff style) and insert (earplug-style) devices. Furthermore, Casali and Lee (Citation2019), demonstrated that certain active protectors are not at all amenable to training benefits for localisation, i.e. trainee performance with a prototype device did not approach that of the open ear. Proper design and training of battery-powered level-dependent hearing protection can help maximise the benefits for protection and auditory situation awareness (Casali and Robinette Citation2015). Encouragingly, the improved detection that certain models of active protection offer may render more cues susceptible to training effects, although these benefits are very device- and situation-specific.
In order to standardise testing of auditory situation awareness, Virginia Tech Auditory Systems Laboratory personnel developed a battery of tests known as Detection, Recognition/Identification, Localisation, and Communication (DRILCOM) (Casali and Lee Citation2019). The test battery and apparatus previously demonstrated its sensitivity to differences among several active and passive level-dependent hearing protectors on various aspects of auditory situation awareness (Casali and Lee Citation2019; Casali and Robinette Citation2015). This same instrumentation shows potential as a means to deliver training to improve localisation accuracy, as demonstrated by Casali and Robinette (Citation2015). As such, the Office of Naval Research (ONR) provided a contract to develop and validate a horizontal auditory localisation training regimen for later incorporation into a portable auditory localisation training system that would be sensitive to design-imposed differences among hearing protection devices. The eventual goal of the training regimen and portable system will be to improve auditory localisation in service members, both with and without hearing protection devices. The focus of this study was the development of effective stimuli and presentation protocols for use in a training regimen for later incorporation into the portable system’s design that will be covered in a follow-on study. Therefore, the purpose of this study was to develop an optimised azimuth training protocol for eventual use in a field-validated portable localisation training system.
The localisation protocol used in the DRILCOM auditory training regimen employs a stimulus that incorporates spectral cues needed for localisation. Specifically, the DRILCOM apparatus emits a dissonant, non-harmonically related, tonal signal that contained 104, 295, 450, 737, 2967, 4959, 7025 and 7880 Hz (Casali and Lee Citation2019). These frequencies render the predominant horizontal localisation cues accessible using the following mechanisms: interaural timing differences (ITDs), interaural level differences (ILDs) and pinnae spectral cues. ITD cues dominate localisation of sounds emanating from the sides of the head below 1500 Hz as the wavelength must be able to “bend around” the diameter of the head to render timing cues (Moore Citation1997). ILDs occur when the near ear receives a more intense signal than the far ear (Emanuel, Maroonroge, and Letowski Citation2009). ILD cues are dominated by higher frequencies with frequencies above 2000–3000 Hz providing the most information, again for sounds from the sides of the head (Moore Citation1997). Lastly, the highly contoured surface of the pinnae and successive funnelling into the ear canal resonates higher frequencies (Emanuel, Maroonroge, and Letowski Citation2009). This contouring creates spectral changes in the signal even with small changes in sound location, particularly in the 3000–8000 Hz region for sounds that emanate generally from the front or back of the head. (Pickles Citation1988). While the dissonant signal is “trainable”, whether this signal transfers to localisation accuracy using military-relevant signals remains unknown.
Training principles developed by Wolfle (Citation1946) offer guidance to improving the DRILCOM training procedure. Wolfle’s (Citation1946) training guidelines were as follows: (1) distribute practice in order to pace training so as not to overload the trainee or unnecessarily extend session length; (2) have trainees participate versus only having them observe; (3) vary material to prevent monotony and improve generalisation of the skill; (4) maintain accurate records of progress in order to monitor and tailor individual training; (5) furnish immediate feedback to reinforce correct training procedures and extinguish incorrect ones and (6) provide clear and systematic lesson plans with simple logical steps that builds into successively more complicated material. As such, this study evaluated the efficacy of adding each of the aforementioned principles not already included in the DRILCOM protocol.
Objectives
Determine if training with DRILCOM’s broadband signal transfers to localising untrained military-relevant stimuli
Evaluate the impact of decreasing training duration by decreasing the number of stimulus presentations
Evaluate the effects of using active participation in the training regimen, as opposed to passive techniques in the DRILCOM protocol (Casali and Lee Citation2019)
Evaluate the effects of adding an adaptive strategy that individually tailors training
Materials and methods
In order to describe the training methodologies manipulated in this study, a recapitulation of the methods on which they were based is warranted. The DRILCOM training strategy used by Casali and Lee (Citation2019) incorporated 12 Learning Units (LU), with each LU lasting approximately 20 minutes for a total of about four hours. An LU is a complete block of auditory localisation training. A training protocol is considered complete after a participant completes a preset number of iterations of these LUs. The basic experimental task involved the participant selecting on the display via mouse, “click to sound”, a sound then played from one of 12 locations, and the participant would respond by selecting the speaker location on the display via mouse click. Prior to the training, participants were given a familiarisation task in which they practiced initiating and responding to the experimental signal. The participants were also shown how the system would display feedback for a correct and incorrect response. Participants were instructed to respond as accurately and quickly as possible once they initiated signal delivery. In Casali and Lee’s (Citation2019) DRILCOM study, each LU was comprised of the following steps:
Sequential- The dissonant signal would play around a 12-speaker “clock face” array, separated by 30° (i.e. a speaker located at each “hour”), in order, for a total of five “laps” around the 12-speaker array. The speaker array was hidden by an acoustically transparent curtain and pictured in . The participant had prior knowledge of which loudspeaker location would play the sound, the participant would respond, and the display would indicate the actual sound location versus participant response, as shown in .
Figure 1. DRILCOM testing facility and participant interface demonstrating the 12 response location options and system-generated feedback for an incorrect response. Adapted from Casali and Lee (Citation2019), Figure 1, pS67 and Figure 3, pS69.
Random- The participants would have no prior knowledge of which speaker would play the signal, but received immediate feedback by displaying the answer given and the actual location of the signal. The signal was played from each speaker location five times in random order.
Test- A signal would play from each location three times in random order for a total of 36 presentations. The participant would not know the signal location ahead of time and would not receive feedback regarding the response.
In order to optimise Casali and Lee’s (Citation2019) DRILCOM protocol, two pilot studies and one main experiment were conducted in order to converge on a localisation training protocol. The investigators hypothesised that training with a broadband stimulus, reducing the number of presentations, and implementing more user-engaged training techniques would result in training transfer, or learning. Randomisation was conducted using a Microsoft® Excel random order generator by assigning the order by which the participant walked through the door to a subject number. Then, random assignment to a presentation condition was stratified according to the number of conditions (e.g. stratified every third participant for the three conditions in Pilot 1). Pilot 2 was conducted after Pilot 1 and served to add another condition to the experiment. Therefore, all participants in Pilot 2 underwent the same conditions and their assignment was not randomised.
In the original Casali and Lee (Citation2019) DRILCOM protocol, one training block, or LU, was comprised of sequential, random and test subunits. The dissonant stimulus played randomly from each speaker location five times in the sequential and random subunits. In the test subunit, the signal was randomly presented three times from each location. Pilot Experiment 1 sought to shorten training time by manipulating the number of presentations only in the sequential and random subunits. Three presentations in the test subunit remained constant across conditions. Instead of five presentations of the dissonant signal from each speaker location, as in the original DRILCOM protocol, participants heard only two, three or four presentations from each location for the sequential and random subunits. All participants trained and tested on the broadband stimulus then tested on four untrained, military-relevant stimuli. In Pilot Experiment 2, the experimenters evaluated the effects of eliminating sequential presentations from LU2–LU8. The four-presentation group from Pilot 1 served as the comparison group. The experimental group in Pilot 2 had almost identical subunit components with the exception of no sequential presentations after LU1. All participants underwent eight LUs and then underwent four tests with the untrained, military-relevant stimuli. In both pilot studies, the investigators assessed the presence of training transfer, or learning, according to the number of presentations and type of test stimuli (dissonant and military-relevant). For randomisation for each condition, participants were assigned to a two-, three- or four-presentation condition and randomly matched to the presentation condition.
With the input of optimised presentations and signal stimulus, the main experiment assessed four different training strategies. In all training conditions, the participants underwent eight LUs. The training conditions were known as: DRILCOM, adaptive, choose, and adaptive + choose. The DRILCOM condition maintained most of the strategies from the Casali and Lee (Citation2019) study with each LU comprised of sequential, random and test subunits. The adaptive condition was comprised of all of the conditions of DRILCOM with the addition of a subunit, known as adaptive, that immediately followed the random subunit. The adaptive subunit presented the participant with four presentations from each of the speaker locations answered incorrectly in the preceding random subunit. The choose condition followed the same format as DRILCOM, except with the addition of a subunit following the random one. In the choose subunit, the participants employed a more active training strategy compared to the more passive and system-generated ones in the aforementioned strategies. The participant had 18 trials to choose from which speaker location they wished to practice. The adaptive + choose condition was comprised of the following subunits: sequential, random, adaptive, choose and test. The composition of each LU is provided in . The number of presentations within each subunit was manipulated in order to total the same approximate total number of presentations among conditions. However, the adaptive condition varied according to individual performance in the preceding random session. The same instrumentation used in Casali and Lee (Citation2019), shown in , was used in both pilots and the main experiment. The diameter of the 12-speaker array was slightly greater than 3 metres, with each loudspeaker mounted at 1.14 metres from the ground mounted on a steel ring (Casali and Lee Citation2015). The speaker model used in DRILCOM was 30-Watt, 6.25 inch Behritone C50A powered speakers with a 5.25 inch round driver with a frequency response of 90–17,000 Hz, flat within ± 3 dB (Casali and Lee Citation2015). For background noise generation, the system used a QSC CX1102 TM power amplifier and 4 JBL SoundPower SP215-6™ speakers (Casali and Lee Citation2015). The DRILCOM system PC equipped was equipped with Windows 7, LabVIEW™ (2013 version), MatLab™ and used to record localisation performance. The computer monitor and mouse in front of the participant recorded the response. An automated 15-position switch, of which 12 were used in this experiment, were routed into the patch bay that were routed to the speakers.
Table 1. Subunit composition for each training condition in the main experiment.
Participants
All participants were recruited from the Virginia Tech community in accordance with a protocol approved by the university Institutional Review Board for human subjects. In the pilot studies, general trends, versus experimental power, were sought to assist in developing a training protocol. With the exception of the second pilot study, the target gender ratios of participants represented those in the U.S. military of 75–85% male and 15–25% female (DMDC, 2019). The first pilot study included 14 males and four females (ages 19–42, mean age of 27). The second pilot included one male and five females (ages 18–28 with a mean age of 24). In the main experiment, 31 males and nine females participated (ages 19–38, with a mean age of 24). Participants had no prior experience with electronic or other level-dependent hearing protection or localisation training from previous research participation or video games. However, all testing was conducted without the use of hearing protection. All participants were otoscopically inspected to ensure their ear canals were generally free from obstructions. Participants were also pre-screened for hearing thresholds not to exceed 25 dB HL from 500–6000 Hz, with no threshold difference between each ear to exceed 15 dB.
Pilot experiment 1
The first pilot sought to shorten the number of presentations within each LU. Casali and Lee’s (Citation2019) DRILCOM protocol incorporated 12 LUs. Each LU contained two training sessions and one test session. First, the software presented five sequential “laps” around the “clock” occurring in clock-wise and counter-clockwise order. The next training subunit was comprised of five random presentations from each of the 12 speaker locations. Lastly, in the test subunit the test stimulus was played three times from each location in random order.
In Pilot Experiment 1, the number of presentations in the sequential and random subunits were reduced. Participants were randomly assigned to conditions consisting of either two, three or four-presentations from each speaker location in the sequential and random subunits. Regardless of presentation condition, three presentations from each location were maintained for the test subunit. All participants underwent eight LUs with the dissonant signal. Participants were trained using the dissonant signal presented at 55 dBA with 40 dBA of pink noise in order to mask ambient sounds. The accuracy performance measure was the number of speaker locations correctly identified out of 36 presentations for each test subunit, totalling eight measures. From this measure, the mean score according to presentation condition was computed. Specifically, this pilot was used to determine which number of presentations resulted in 90% accuracy in the least amount of time. The 90% criterion, or 32.5 correct out of 36, was established due to the approximately 88% absolute localisation accuracy achieved in Casali and Lee (Citation2019) that involved a more difficult task of choosing from 24 options (12 actual speaker locations and 12 distractors on the display) versus the 12 in this pilot study. The investigators determined from the Casali and Lee (Citation2019) study that performance reached asymptote at eight LUs. Therefore, the number of LUs were reduced from 12 to eight in the pilot study.
After completion of eight LUs with the dissonant signal, participants underwent a test using four military-relevant stimuli. The purpose of the testing with the untrained stimuli was to evaluate if training using the dissonant signal generalised to untrained stimuli. A subject matter expert panel was assembled to assist in determining which sounds were important for ground combat service members to localise. Seven soldiers with at least one deployment, and with as many as six, with direct combat experience were queried. Ten infantry marines collectively responded from the Gruntworks programme. Gruntworks is a U.S. Marine Corps organisation tasked with research, design, acquisition and equipping Marine rifle squads. Via email, panel members were asked to provide sounds that they felt were important for service members to localise. Respondents were asked to rank order their selection with 1 being the most important and 5 being less important. From the eight responses, the most commonly ranked items in order of importance were: (1) small arms fire, namely the AK-47 rifle, (2) indirect fire (e.g. mortar and rocket-propelled grenade), (3) speech (e.g. shouted commands or local nationals in the conflict zone) and (4) aircraft. From the aforementioned responses, the untrained test signals were recorded in one second durations each, and consisted of the following: (1) an AK-47 three round burst, (2) a whistle tube simulator to emulate an incoming rocket-propelled grenade, (3) a sentence in Arabic, (4) an Apache helicopter. Each signal was presented with an overall (broadband) amplitude of 55 dBA at the subject’s ear position. The order of presentation of the test signal was randomised using a a Microsoft® Excel random order generator. The number of presentations (two, three and four) in each training subunit (i.e. sequential and random), served as the between-subjects factor. The untrained test stimuli (i.e. AK-47, Apache, Arabic and whistle tube) served as the within-subjects factor. The spectral content of each stimuli is depicted in .
Pilot experiment 2
In the second pilot, the purpose was to determine the impact of eliminating sequential testing from LU2–LU8 on localisation accuracy. Participants often remarked that the sequential subunit seemed the least beneficial and the most monotonous. As a result, the investigators evaluated the effects of maintaining four sequential presentations in LU1 only. All experimental procedures were replicated from the first pilot, with exception of removing the sequential subunit from LU2–LU8. Results from Pilot Experiment 1 demonstrated that four stimulus presentations from each speaker location for the random subunit yielded the greatest training transfer. Therefore, Pilot Experiment 2 consisted of the following subunit components: sequential (LU1 only, four presentations), random (four presentations, LU1–LU8), test (three presentations, LU1–LU8), and four tests using untrained, military-relevant stimuli (three presentations following LU8). The objective was to compare the scaled-down sequential condition to the four-presentation condition in Pilot Experiment 1. Therefore, the between-subjects variable in the second pilot was the number of presentations from each speaker location for the sequential subunit for LU2–LU8. The within-subjects factor was untrained test stimuli i.e. AK-47, Apache, Arabic and whistle tube). The dependent measure was consistent with the first pilot where number correct out of 36 for each test was computed. From this measure, the slopes of the simple linear regression lines across LUs were calculated and compared between the four-presentation condition in the first pilot and the results from the second pilot.
Main experiment
The main experiment evaluated the effect of training strategy on localisation accuracy across LUs. Therefore, the experiment used a pre-test-posttest control group, mixed-factors design. The training strategy served as the between-subjects factor. The LU served as the within-subjects factor. After the familiarisation task, a pre-test was administered. Then, eight LUs were administered. The test sub-unit in LU8 served as the post-test. The training strategies, or conditions, were: DRILCOM, adaptive, choose, and adaptive + choose. displays the number of presentations from each speaker location in each LU. The results from the two pilot experiments determined the type and number of stimuli used in the main experiment. Pilot data showed that training with the dissonant signal generalised to localisation performance using all of the untrained test stimuli except the whistle tube simulator. Additionally, due to the presence of a possible ceiling effect, the main experiment displayed 24 possible response locations versus 12 used in the pilot and Casali and Lee (Citation2019) DRILCOM study. However, only 12 active speakers were used in this study and 12 additional speakers on the display served as distractors. The change in response options resulted in accuracy within 15°. Four sequential presentations were maintained in the LU1. One sequential presentation was incorporated in LUs 2–8, with a randomised starting position and direction (clockwise or counter-clockwise). The subunits within each experimental condition are listed in . The measurement variables were mean raw score that were converted to absolute percent correct (i.e. number correct out of 36).
The dissonant signal and pink noise were presented at 70 dBA and 55 dBA. respectively. The follow-on research on the portable localisation training system that will incorporate the training strategy as a result of this experiment will use at least two active sound-transmission hearing protection devices in the form of military Tactical Communications and Protective Systems (TCAPS) (Casali Citation2010b). The 70 dBA presentation level was used in order to simulate a typical operational situation with signals that need to be localised, but are supra-threshold and easily detected, and also which, depending upon the active protector’s design, trigger the amplifier in the electronic pass-through circuitry without putting the devices into full compression.
Results
In Pilot 1, the mean raw score at each LU was computed for each presentation condition and are shown in . A linear regression approach was applied to the data, where LU served as the predictor variable and raw score served as the response variable. The slope of the line for each presentation condition was computed from LU1 through LU8 (two presentation, score = 30.36 + 0.28 × LU; three presentation, score = 29.46 + 0.63 × LU; four presentation, score = 32.51 + 0.18 × LU). All tests used p < 0.05 as the threshold for significance. Slope comparison using independent t-tests showed no significant difference among slopes (two versus three, t[94] = 1.13, p = 0.261, SE = 0.31; two versus four, t[94] = 0.34, p = 0.732, SE = 0.31; three versus four, t[94] = 1.40, p = 0.164, SE = 0.33). Using the cut-off value of less than 10% degradation in percent correct from LU8 to the untrained stimuli, all untrained signals showed transfer-of-training benefit except the whistle tube simulator. The highest mean score was obtained in the three-presentation condition at LU5 (M = 34.7, SD = 1.94). The four-presentation condition resulted in the highest mean scores for AK-47, Apache, Arabic and whistle tube stimuli. Four one-way between-subjects ANOVAs for each untrained stimulus were conducted in order to evaluate the effect of number of presentations on localisation accuracy. The number of presentations served as the independent variable and the number of correct responses out of 36, or raw score, served as the dependent variable. Of note, one participant did not complete testing using the Arabic stimulus. No significant differences existed among presentation conditions in any of the four ANOVAs (AK-47, F[2,15] = 0.78, p = 0.48, MSE = 23.04; Apache, F[2,15] = 0.83, p=.46, MSE = 16.3; Arabic, F[2,14] = 0.89, p = 0.43, MSE = 14.01; whistle tube, F[2,15] = 1.42, p = 0.27, MSE = 25.46).
Figure 3. Mean score for each presentation condition at each training stage for Pilot Experiment 1 and Pilot Experiment 2.

Regression lines were fitted using the mean accuracy score at each LU for the four-presentation condition in Pilot 1 (score = 32.51 + 0.18 × LU) and Pilot 2 (score = 28.89 + 0.26 × LU). Therefore, LU, or stage of training, served as the predictor variable and raw score served as the response variable. The only difference between these two conditions was that Pilot 2 only had sequential training in LU1, compared to Pilot 1 that included sequential training in all LUs. The slope of the lines between the two pilot studies were evaluated using independent-samples t-tests. The slope of the regression line for Pilot 1 was significantly greater than Pilot 2, t(94)=3.74, p < 0.0003, SE = 0.32. Therefore, the results supported that the four-presentation condition yielded more learning. For untrained stimuli, the mean scores for the four-presentation condition were greater by 7–18% than for the Pilot 2 scores. Given the poorer results with Pilot 2, at least one sequential presentation was included in the main experiment.
For the main experiment, a mixed-factor ANOVA analysed the effect of training strategy and the LU (pre-test and LU8) on raw score. Training strategy served as the between-subjects factor and LU served as the within-subjects factor. The main effect for training strategy was not significant (at p < 0.05), F(3,36)=0.34, p = 0.78, MSE = 47.62, =0.03. The interaction for LU and training strategy was also not significant, F(3,36)=2.13, p = 0.11, MSE = 13.9,
=0.15. The main effect for LU was significant, F(1,36)=201.26, p < 0.001, MSE = 13.9,
=0.85. Follow-up testing for LU was not performed given that only two levels of the variable were measured and mean performance at LU8 was clearly better.
In observing the data in , performance appeared to asymptote after LU4. To determine if significant effects existed at LU4 compared to the pre-test, a mixed-factor ANOVA evaluated the effect of training strategy and LU on raw score at pre-test and LU4. Training strategy served as between-subjects factor and LU (pre-test and LU4) served as the within-subjects factor. The interaction for training strategy and LU was not significant, F(3,36)= 2.60, p = 0.07, MSE = 13.65, =0.18. The main effect for training strategy was also not significant, F(3,36)= 0.59, p = 0.628, MSE = 45.5,
=0.05. A main effect for LU was significant, F(1,36)= 192.09, p < 0.001, MSE = 13.65,
=0.84. To evaluate if significant changes in performance occurred among training strategies at LU4 and LU8, a mixed-factors ANOVA was performed. Non-significant results were obtained for the following: interaction of training strategy and LU (F[3,36] = 0.73, p = 0.542,
=0.06), training strategy (F[3,36] = 0.40, p = 0.76,
=0.03) and LU (F[1,36] = 0.59, p = 0.45,
=0.02). Results of mixed-factors ANOVA comparing performance at pre-test, LU4, and LU8 support that no significant improvement in localisation accuracy existed beyond LU4.
In order to evaluate significant changes in performance from pre-test through LU4 by training strategy, regression lines were fitted for each training strategy (adaptive, score = 17.7 + 2.78 × LU; choose, score = 18.7 + 3.26 × LU; adaptive + choose, score = 18.3 + 2.94 × LU; and DRILCOM, score = 22.52 + 1.67 × LU). The LU served as the predictor variable and the raw score served as the response variable. A between-subjects one-way ANOVA found significant differences in slopes in the fitted regression lines among the four training strategies, F(3,36)= 3.04, p = 0.041. A Tukey post hoc test showed a significant difference between the slopes for choose (M = 3.26, SD = 1.52) and DRILCOM training conditions (M = 1.67, SD = 0.76), p = 0.035. Comparisons using the adaptive (M = 2.78, SD = 1.31) and adaptive + choose (M = 2.94, SD = 1.63) conditions were not significant. Results support that a greater increase in localisation accuracy occurs as training progresses with the choose training strategy vs. DRILCOM.
A mixed-factor ANOVA analysed the effect of training strategy and LU on response time at pre-test to LU4. Training strategy served as the between-subjects factor and LU served as the within-subjects factor. An interaction effect for LU and training strategy was not significant, F(3,36)= 0.46, MSE = 0.18, p = 0.71, =0.04. The main effect for training strategy was not significant, F(3,36)= 0.26, MSE = 0.55, p = 0.86,
=0.02. A main effect for LU was significant, F(1,36)= 12.41, MSE = 0.18, p = 0.001,
=0.26. Regression analysis was performed in order to evaluate if an improvement in raw score predicted a reduction in response time from adjacent LUs from pre-test to LU4 (adjacent LU difference score response time= −0.05 − 0.01 × adjacent difference score). Analysis was conducted on changes in absolute score and response time in the progression of training between adjacent sessions (Later LU − Earlier LU). A negative number in response time indicated an improvement (decrease in response time). Results were not significant, F(1,158)=3.05, p = 0.08, R2= 0.03; however, the general trend was that response time decreased with an increase in score. Extending the analyses to LU8 by fitting a regression line (adjacent difference score response time= −0.03 − 0.01 × adjacent difference score) resulted in significant results, F(1,318)= 8.76, p = 0.003, R2=0.03. Results suggest that with progression of training, accuracy improves and response time decreases, but the training takes approximately twice as long to see this change in response time.
Discussion
As expected, training with a signal that contains both ITD and ILD cues transferred to the untrained stimuli that contained broadband spectral cues. However, the whistle tube simulator that contained primarily high-frequency tonal information (and little or no ITD low-frequency cues) did not transfer. The findings are consistent with the work of Mills (Citation1958) that showed that the minimum audible angle for signals at 3000 Hz can be as much as 7° poorer than for stimuli below 1500 Hz depending on the signal azimuth. In other words, high-frequency stimuli are more difficult to localise than low-frequency signals. Participants also described the whistle tube simulator as annoying. This perception may have increased workload and degraded motivation to respond accurately. While the results illustrate that training with a broadband signal does not transfer to a high-frequency tonal one, the results do not necessarily imply that training to localise an incoming rocket-propelled grenade is infeasible. Employing an actual recording of an incoming RPG may show different results given the spectral content.
While statistical significance was neither the goal nor obtained in the two, three and four-presentation conditions, data trends supported that four presentations within the training sub-units yielded the highest accuracy in the fewest learning units. Additionally, a clear advantage in untrained stimuli performance existed for the four-presentation condition. One limitation of the first pilot study was that participants were not tested with the untrained stimuli after LU4, and thus, the actual transfer after four LUs cannot be calculated from these data. Moreover, the second pilot reinforced the findings of the first pilot by demonstrating a clear advantage of using more presentations in each LU, resulting in greater localisation accuracy across LUs and with untrained stimuli.
While statistical significance did not support the hypothesis that differences existed among training strategies, the “choose” condition demonstrated an advantage by resulting in the greatest slope from LU1 to LU4. Additionally, the choose and adaptive + choose conditions tied for the greatest number correct at LU4, but the choose condition achieved this score in fewer stimulus presentations. Promisingly, the results demonstrated that regardless of training strategy, localisation training improved accuracy over time while decreasing response time. The ad hoc analysis of performance between pre-test and LU4 and between LU4 and LU8 yielded useful data for the follow-on study. Specifically, this finding results in decreasing the training time by about half. However, given that the follow-on study will incorporate advanced hearing protection devices and TCAPS, four learning units may be insufficient to fully capture the range of training effects.
A limitation to this study is that localisation learning decay was not assessed. While the long-term effects of the training has yet to be evaluated, the results from this study can be employed to converge on a solution to prevent attrition of auditory localisation skills. Another limitation, which will be addressed in a follow-on study, is the external validity of training in a laboratory environment. Service members often have more annual training requirements than is technically feasible in a year. To better ensure that the benefit of training exceeds the time and money invested in training, the impact on actual job performance should be quantified. Implications for a localisation training protocol include: a more comprehensive auditory situation awareness fitness-for-duty evaluation, a means to evaluate hearing protection effects on localisation, and remediation of localisation inaccuracies with and without hearing protection. Exactly how much localisation accuracy is needed for service members to maintain auditory situation awareness remains unknown, and it also will vary among different military occupational specialties (MOS’s) and for different tactical missions. However, the DRILCOM testing protocol could measure localisation loss imposed by either hearing loss or hearing protection. Performance on duty-related tasks could then be evaluated according to differing degrees of localisation loss quantified by DRILCOM. Provided that DRILCOM validly predicts duty-related performance, the apparatus and test could clinically assess the functional impact of localisation loss. Subsequent experimental investigations could focus on assisting those with localisation cue loss to remediate localisation errors and improve functioning to a level of acuity to fulfil duty-related tasks.
Disclosure statement
No potential conflict of interest was reported by the authors.
Correction Statement
This article has been republished with minor changes. These changes do not impact the academic content of the article.
Additional information
Funding
References
- Alali, K. A., and J. G. Casali. 2011. “The Challenge of Localizing Vehicle Backup Alarms: Effects of Passive and Electronic Hearing Protectors, Ambient Noise Level, and Backup Alarm Spectral Content.” Noise and Health Journal 13 (51): 99–112. doi:10.4103/1463-1741.77202.
- Alali, K. A., and J. G. Casali. 2012. “Auditory Backup Alarms: Distance-at-First Detection via In-Situ Experimentation on Alarm Design and Hearing Protection Effects.” Work: A Journal of Prevention, Assessment, and Rehabilitation 41: 3599–3607. doi:10.3233/WOR-2012-0671-3599.
- Casali, J. G. 2010a. “Passive Augmentations in Hearing Protection Technology Circa 2010 Including Flat-Attenuation, Passive Level-Dependent, Passive Wave Resonance, Passive Adjustable Attenuation, and Adjustable-Fit Devices: Review of Design, Testing, and Research.” International Journal of Acoustics and Vibrations 15 (4): 187–195. doi:10.20855/ijav.2010.15.4270.
- Casali, J. G. 2010b. “Powered Electronic Augmentations in Hearing Protection Technology Circa 2010 Including Active Noise Reduction, Electronically-Modulated Sound Transmission, and Tactical Communications Devices: Review of Design, Testing, and Research.” International Journal of Acoustics and Vibrations 15 (4): 168–186. doi:10.20855/ijav.2010.15.4269.
- Casali, J. G., and K. Lee. 2015. Objective Metric-Based Assessments for Efficient Evaluation of Auditory Situation Awareness Characteristics of Tactical Communications and Protective Systems (TCAPS) and Augmented Hearing Protective Devices (Technical Report). Blacksburg, VA: Virginia Polytechnic Institute and State University.
- Casali, J. G., and K. Lee. 2019. “Learning to Localize a Broadband Tonal Complex Signal with Advanced Hearing Protectors and TCAPS: The Effectiveness of Training on Open-Ear vs. device-Occluded Performance.” International Journal of Audiology 58 (Suppl 1): S65–S73. doi:10.1080/14992027.2018.1532118.
- Casali, J. G., and M. B. Robinette. 2015. “Effects of User Training Within Electronically-Modulated Sound Transmission Hearing Protectors and the Open Ear on Horizontal Localization Ability.” International Journal of Audiology 54 (Suppl 1): S37–S39. doi:10.3109/14992027.2014.973538.
- Cave, K.C. 2019. “Evaluation of an auditory localization training system for use in portable configurations: variables, metrics, and protocol.” Doctoral dissertation.
- Clasing, J. E., and J. G. Casali. 2014. “Warfighter Auditory Situation Awareness: Effects of Augmented Hearing Protection/Enhancement Devices and TCAPS for Military Ground Combat Applications.” International Journal of Audiology 53 (Suppl 2): S43–S52. doi:10.3109/14992027.2013.860489.
- Defense Manpower Data Center (DMDC). 2019 March 10. “DoD personnel, workforce reports & publications.” DMDC: DoD Data Reports. Accessed May 2019. https://www.dmdc.osd.mil/appj/dwp/dwp_reports.jsp
- DOEHRS-DR. 2016. Defense Occupational Health Readiness System Data Repository (DOEHRS-DR). Aberdeen Proving Ground: U.S. Army Public Health Command. https://doehrswww.apgea.army.mil/doehrsdr
- Emanuel, D. C., S. Maroonroge, and T. R. Letowski. 2009. “Auditory Function.”. In Helmet-Mounted Displays: Sensation, Perception and Cognition Issues, edited by C. E. Rash, M. B. Russo, T. R. Letowski, and E. T. Schmeisser, 279–306. Fort Rucker, AL: U.S. Army Aeromedical Research Laboratory.
- Groenwold, M. R., S. Tak, and E. Matterson. 2011. “Severe Hearing Impairment among Military Veterans-United States, 2010.” Journal of the American Medical Association 306 (11): 1192–1194.
- Matterson, E. A., P. T. Bushnell, C. L. Themann, and T. C. Morata. 2016 April 22. “Hearing Impairment among Noise-Exposed Workers- United States, 2003-2012.” MMWR. Morbidity and Mortality Weekly Report 65 (15): 389–394. doi:10.15585/mmwr.mm6515a2.
- Mills, A. W. 1958. “On Minimum Audible Angle.” The Journal of the Acoustical Society of America 30 (4): 237–246. doi:10.1121/1.1909553.
- Moore, B. C. 1997. “Space Perception.” In An Introduction to the Psychology of Hearing, edited by B. Moore. San Diego, CA: Academic Press.
- Pickles, J. O. 1988. An Introduction to the Physiology of Hearing. San Diego, CA: Academic Press.
- Talcott, K. A., J. G. Casali, J. P. Keady, and M. C. Killion. 2012. “Azimuthal Auditory Localization of Gunshots in a Realistic Field Environment: Effects of Open-Ear Versus Hearing Protection-Enhancement Devices (HPEDS), Military Vehicle Noise, and Hearing Impairment.” International Journal of Audiology 51 (Suppl 1): S20–S30. doi:10.3109/14992027.2011.631591.
- Tufts, J. B., K. Vasil, and S. Briggs. 2009. “Auditory Fitness for Duty: A Review.” Journal of the American Academy of Audiology 20 (9): 539–557. doi:10.3766/jaaa.20.9.3.
- Wolfle, D. 1946. “Military Training and Useful Parts of Learning Theory.” Journal of Consulting Psychology 10 (2): 73–75. doi:10.1037/h0059209.