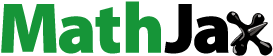
Abstract
Objective: Acoustic dosimetry (AD) data collected on the International Space Station (ISS) were analysed to investigate the impact of impulse noise on crew noise exposure.
Design: The noise exposure during work (LAeq16h) and sleep (LAeq8h) time, and the number of impulses >115 dB peak that occurred during each measurement activity, were calculated from the AD data. Two parametric studies were used to estimate the effect of 1) impulses in the original data set, and 2) hypothetical impulses of different levels, durations and quantities on LAeq16h.
Study sample: Twelve sets of AD data collected on the ISS from November 2017 to October 2018.
Results: The ISS work time noise limit (72 dBA) was exceeded in four of the 12 data sets. In three of those, there were over 100 impulses >115 dB peak and the number of impulses was significantly correlated with LAeq16h. However, the impulses only caused a meaningful increase in LAeq16h when the number of occurrences was large (>50), or when both the level and duration of the impulses were large.
Conclusions: Continued monitoring of impulse noise data is recommended to facilitate the investigation of exceedances or abnormalities in future AD data acquired on the ISS.
This article was based on a presentation at the 2019 Annual Conference of the National Hearing Conservation Association, and is part of the 10th dedicated supplement to hearing loss prevention
Introduction
During long-duration missions on the International Space Station (ISS), ambient noise levels and personal noise exposure data are closely monitored and applied against the ISS acoustic requirements for crew health and performance. The Multilateral Medical Operations Panel (MMOP) Acoustics Sub-working Group (MAS), which operates under the MMOP Environmental Health and Safety Group, is responsible for providing information to the MMOP about the effects of acoustics on crew health and safety. Acoustic dosimetry (AD) measurements are conducted for each crew member at least once every two months. Based on the World Health Organization’s recommendations, the ISS noise exposure limit is 70 dBA in a 24 h period (Berglund, Lindvall, and Schwela Citation1999). Since crewmembers typically live in the ISS for a period of six months with no relief from its noise environment, 24 h noise exposure must be considered rather than conventional National Institute for Occupational Safety and Health (NIOSH) 8 h occupational noise exposure limits (National Institute for Occupational Safety and Health Citation1998). More specifically, The ISS medical operations requirements (NASA Citation2012) state that the limits are 72 dBA during work time of 16 h (LAeq16h) and 62 dBA during sleep time of 8 h (LAeq8h) for adequate hearing rest. The document also states that impulse noises of 1 s duration or less must not exceed 140 dB peak SPL to reduce the risk of noise-induced hearing loss (NIHL), and a limit of 125 dB peak SPL is recommended to reduce the impact of startle (NASA Citation2012).
The primary sources of noise on the ISS are the fans, pumps and airflow associated with the environmental control and life support and thermal control systems (Allen and Denham Citation2018). The data that are obtained from the AD and sound level meter surveys have enabled the detection and remediation of exceedances in noise limits. Recent examples include the replacement of ventilation system backpressure plates and unclogging of the intra-module ventilation fan (Allen and Denham Citation2018). Other noise limit exceedances have been attributed to crew activity (Limardo, Allen, and Danielson Citation2017). Overall, the noise monitoring data have shown that the acoustic conditions of the ISS have improved over time with the addition of quieter modules and crew sleeping quarters (Allen and Denham Citation2018).
Members of the MAS have expressed concern regarding the accuracy of the LAeq8hr and LAeq16h metrics that are calculated from the AD data, particularly regarding the contribution of impulse noise. Although payloads that produce impulse noise in excess of 140 dB SPL peak are not permitted on the ISS (NASA Citation2012), impulse noise of lower levels caused by acoustic [e.g., use of the centrifuge or treadmill (T2)] or non-acoustic sources (e.g. taps on the microphone) are present in the AD data, and their contributions to personal noise exposure are unknown. Royster et al. previously reported minimal effects of thumping, hollering and blowing in a dosimeter microphone on the time-weighted average (TWA) in a pink noise background noise of 85 and 90 dBA (Royster, Berger, and Royster Citation2000). Indeed, such artefacts in the noise dosimetry data are not expected to contribute to the overall measured noise levels when the background noise is high (>80 dBA). However, in moderate noise environments such as the ISS and terrestrial occupational settings such as offices, schools or hospitals, artefacts in noise dosimetry data are a valid concern.
In the current study, we analysed the unweighted peak levels (LZpeak) to answer the following questions: 1) What impact, if any, do high-amplitude impulses have on LAeq8h and LAeq16h?, 2) What are the possible sources of high-amplitude impulse noise on the ISS?, and 3) Should the impulse noise limit be lowered to 125 dB to minimise startle and further protect the crewmembers from NIHL? To keep the discussion of impulse noise within context for the ISS, it is emphasised that we are considering impulse noise up to 140 dB that can be logged with conventional acoustic dosimeters. Dosimetry for impulse noise greater than 140 dB, which presents a number of technical challenges that have been investigated by others (Kardous and Willson Citation2004; Smalt et al. Citation2017; Davis et al. Citation2019), is outside of scope for the ISS and the current study.
Method
Calculation of noise levels
An SV 102 A + Class 1 Dual Channel Dosimeter (Svantek; Warsaw, Poland) was used to collect the noise data. The noise levels from three ADs across 12 measurement sessions were analysed, covering the period from November 2017 to October 2018. During each session, three crew members wore a dosimeter for 24 h for crew-worn data (CW) and three dosimeters were mounted at various locations on the ISS for static dosimetry (SD) data. The SD data were collected the day after the CW measurements. The AD logged noise levels at 1 min intervals and included LZpeak (unweighted) and equivalent A-weighted (LAeq) sound pressure levels, as well as the 1/3rd octave band levels. It is noted that LZpeak represents the highest level measured in each 1 min interval and does not account for additional lower-level impulses that may have occurred during the same interval. The 1 min interval is not an instrumentation limit, but a practical one due to the long duration of data collection by crew members and data storage capabilities. This interval was an accepted limitation of the AD data analysis.
The cumulative noise exposures, LAeq8h for sleep and LAeq16h for work time, were calculated for each crewmember, as well as the number of impulses, LZpeak, that exceeded 115 dB peak. For comparison with the well-known NIOSH recommended exposure limit for occupational noise (National Institute for Occupational Safety and Health Citation1998), the 8 h time-weighted average (TWA) of 85 dBA, the LAeq16h were also converted to TWA-equivalent values. The conversion was calculated using
(1)
(1)
where D is the daily dose, or percentage of time the noise exposure was at a hazardous level (National Institute for Occupational Safety and Health Citation1998). The duration in minutes T, at which a given level L, becomes hazardous is
(2)
(2)
with 85 dBA being the critical limit using a 3 dB exchange rate. The dose is calculated as
(3)
(3)
where, for the current dataset, L = LAeq16h and C = 16 h or 960 min.
Parametric studies
To estimate the impact of impulse noise on LAeq16h, two types of parametric studies were done. In the first, the data was adjusted to remove the contribution of impulse noise over 115 dB peak. To achieve this, the adjusted level, LAeq16hadj, was calculated as
(4)
(4)
where
and N is the number of samples logged during work time. For time intervals in which LZpeak >115 dB, the corresponding LAeq was replaced with a floor value of 60 dBA to minimise the contribution of the impulse noise. The 60 dBA floor was chosen based on previous data, because it is well below the 68–71 dBA work time range for LAeq16h that was reported from previous measurements (Limardo, Allen, and Danielson Citation2017). Then, the difference in the work time noise level with the impulses removed was calculated as,
(5)
(5)
LAeq16hdiff was then compared with the number of LZpeak >115 dB that had occurred within each data set to estimate the impact of the number of peaks had on elevating the LAeq16h.
The second parametric study estimated the impact of short duration impulses on the LAeq16h. The purpose was to calculate the hypothetical change in LAeq16h from its original value if impulses of different amplitudes and durations had occurred. To accomplish this, a few representative CW datasets were selected for study. The 1-min LAeq data were upsampled in Matlab from 0.016 Hz to 20 and 100 Hz, corresponding to time intervals, and thus impulse durations, of 50 and 10 ms, respectively. Up to 10 samples were then replaced at random with 115, 120, or 125 dB amplitudes. The hypothetical LAeq16h, with the inclusion of the impulses, was then calculated:
(6)
(6)
where up to 10 values of LAeqk were randomly replaced with 115, 120 or 125 dBA amplitudes. Real impulse sounds such as gunfire have durations of less than 1 ms (Buck, Hamery, and Zimpfer Citation2010). However, within enclosures, the impedance characteristics of reflecting surfaces alter the peak and spectrum of the signal (Hamernik and Hsueh Citation1991). Impulse durations used in NIHL studies in animals have ranged widely from rifle shots of <1 ms to white noise bursts of 200 ms (Danielson et al. Citation1991; Henderson et al. Citation1991; Hight et al. Citation2003). In the current study, impulse durations of 10 and 50 ms were chosen to illustrate the differences between two different impulse durations rather than to emulate specific noise sources.
Noise source identification
In some cases, it is possible to guess the sound source based on its spectral characteristics. For example, a tonal spectrum would suggest a rotating source. Longer duration impacts and impulses such as jackhammering and blasts will have less energy at low frequencies than short impulses of equivalent peak levels (Royster, Berger, and Royster Citation2000; Berglund, Hassmen, and Job Citation1996). Using the LZpeak data, the spectral characteristics of selected high-amplitude values were examined in an effort to link them to possible impulse noise sources.
It is noted that crewmembers will have in-ear noise exposures related to headphone use that are not captured by the AD measurements. This limitation of the current analysis is included in the discussion.
Data analysis
Pearson correlations were calculated between the LAeq16h levels and the number of impulses >115 dB that occurred, and between the LZpeak and LAeq levels that were logged at 1 min intervals. A binary logistic regression was applied to the number of high-amplitude LZpeak occurrences and LAeq16h to determine if the number of impulses predicts an exceedance in the work time noise limit, 72 dBA. For the parametric studies, the effects of impulse level, duration, and quantities on the LAeq16h were observed.
Results
Noise levels
The CW results (average of data from three crewmembers) for LAeq8h and LAeq16h, as well as the LAeq16h converted into TWA values, are shown in . The noise levels exceeded the 72 dBA limit for work time for four of the measurement activities (late January, April, May and early June) and exceeded the sleep time limit of 62 dBA once (early June). The TWA values ranged from 71.0 to 78.6 dBA and were far below the 85 dBA NIOSH noise limit.
Table 1. Average work time LAeq16h and TWA equivalent, and sleep time LAeq8h data from three crew-worn dosimeters from November 2017 to October 2018.
shows the combined total number of high-amplitude Lpeak values (>115 dB) from the three CW dosimeters during work hours, as well as the average work-time noise exposure, LAeq16h. The sleep time results are not shown due to limited data (there were only 11 CW and 32 SD instances of Lpeak >115 dB, in total across all data sets). Across all data sets, there were two instances of peaks exceeding the impulse noise limit of 140 dB peak in the CW data (144.8 and 141.2 dB). Three of the four data sets in which the work time noise limit was exceeded also had the most number of Lpeak >115 dB (April, May and early June).
Figure 1. Total number of high-level Lpeak readings by three crew-worn acoustic dosimeters (bars, left axis) and average work time noise exposure, LAeq16h (diamonds, right axis), shown per measurement activity.

Pearson correlations were calculated between LAeq16h and the number of Lpeak readings above 115, 120 and 125 dB. The results are shown in . Significant moderate correlations were found for the number of peaks at all levels.
Table 2. Correlations between daytime noise exposure (LAeq16h) and the number of high-amplitude peaks for three CW dosimeters across 12 data collections (n = 36).
A binary logistic regression was applied to determine if the number of high-level impulses for each set of CW AD data (n = 36) predicted an exceedance in the daytime noise limit, 72 dBA. The result was marginally significant for the number of peaks >115 dB (β = 0.052, p = 0.05), but not significant for the number of peaks >125 dB (β = 0.246, p = 115).
Pearson correlations were calculated for the 1 min LAeq levels and LZpeak levels above 115, 120 and 125 dB. The results are shown in . A significant correlation was found between LAeq and LZpeak when all data were included, while significant, but weak, correlations were found for LZpeak >115 and LZpeak >120 dB. When only Lpeak data >125 dB were included, there was no correlation with LAeq.
Table 3. Correlations between 1 min LAeq and LZpeak levels from crew-worn dosimetry.
Parametric studies
For each set of crewmember AD data (n = 36), LAeq16hdiff was calculated to estimate the LAeq16h without the contribution of impulses >115 dB peak. The resulting values are plotted against the number of impulses in . LAeq16hdiff ranged from negligible (less than 0.5 dB) for a small number of peaks to over 4 dB when there were over 100 peaks.
Figure 2. Difference in LAeq16h with Lpeak readings >115 dB removed, versus the number of Lpeak readings >115 dB.

For verification of the upsampled data that were used to calculate LAeq16hhyp, the LAeq16h of upsampled data were calculated before the hypothetical impulses were added, and compared to the original data. The results were within 0.1 dB of each other. The results for the parametric study of impulse duration and level, showing LAeq16hhyp for up to 10 added impulses, are shown in for impulses of duration 10 ms and 50 ms. The top figure shows LAeq16hhyp for a baseline level of 73 dBA. The 115 dB peak impulses had a negligible effect (<1 dB increase) on the LAeq16h for both impulse durations and up to 10 occurrences. The LAeq16h increased by >1 dB only when there was more than one 125 dB peak, 50 ms impulse. For the 68.5 dBA baseline level shown at the bottom of , the increase in LAeq16h was more pronounced. The 115 dB peak, 50 ms impulses increased the LAeq16h by >1 dB when three or more impulses were added, while a single 125 dB peak of 50 ms caused an overall increase of 2 dB. Notably, for 10 added impulses of 125 dB peak, 50 ms duration, the increase from baseline level of 68.5 dBA was about 10 dB compared to about 6 dB for the 73 dBA baseline level.
Figure 3. (a) Parametric study results for increase in LAeq16h when up to 10 impulses of 10 or 50 ms duration, and amplitudes of 115, 125 and 125 dB were introduced in the CW AD data, for LAeq16h = 73 dBA. (b) Parametric study results for increase in LAeq16h when up to 10 impulses of 10 or 50 ms duration, and amplitudes of 115, 125 and 125 dB were introduced in the CW AD data, for LAeq16h = 68.5 dBA.

The 1/3 octave band spectra were available for 47 of the 107 LZpeak readings that were greater than 125 dB. The 1/3rd octave band spectra of selected high-amplitude impulses are shown in . In some cases, it was possible to associate the AD data with a specific event. For example, an LZpeak of 144.8 dB occurred just before a battery swap, suggesting that the microphone could have been touched. During one AD activity, T2 noise was measured. Some of the impulses had the highest levels at 20,000 Hz (labelled as “low-frequency” in ), suggesting that the source was non-acoustic (e.g. a caused by a “thump” on microphone). Conversely, some of the impulses showed an increase of amplitude with frequency, with the highest levels at 20,000 Hz (labelled as “high-frequency” in ), which could occur if the microphone was dropped onto a hard surface. Other impulses had tonal characteristics, suggesting a rotating mechanical source. Of the 47 impulses, 10 were identified as tonal, 10 as high-frequency, 10 as low-frequency and one as flat (the 144.8 dB mentioned above). The remaining 16 impulses did not have any spectral features that could convincingly be flagged as non-acoustic.
Discussion
What impact, if any, do high-amplitude impulses have on the LAeq16h and LAeq8h?
The lack of high-amplitude impulses in the CW sleep time data (11 instances across all measurements) indicated that the LAeq8h is not affected by impulse noise. Similarly, the relatively small number of peaks in the SD data (32 across all measurements) indicated that the static noise measurements were not affected by impulse noise. This supports previous observations that the high-level impulses were caused by crew activity rather than ambient noise sources. Due to limited AD units, the SD data are currently collected on different days than the CW data. In the future, concurrent SD and CW data collections could be considered to allow for better identification of acoustic versus non-acoustic impulses.
For the crew-worn data, the number of peaks >115 dB ranged from 33 to 181 in a 24 h period. The number of peaks >115 dB for a given measurement had a moderately significant correlation with its corresponding LAeq16h, although the correlation weakened slightly when only peaks above 120 or 125 dB were included (). A binary logistic regression indicated that while the number of impulses >115 dB was a significant predictor for noise exposure exceedance (LAeq16hr >72 dBA), the relationship did not hold when using the number of impulses >125 dB. It is intuitive that the 1 min readings for LZpeak and LAeq should be related, and this was generally true when all data were included (). However, when the correlation was calculated using only the highest amplitude peaks (>125 dB), the result was not significant. These results suggest that the highest amplitude peaks were caused by very short-duration impulses that did not register in the corresponding LAeq reading. Unfortunately, in the absence of time signal recordings, we were unable to confirm the duration of the logged impulses.
To estimate the effect of high-level impulses on the LAeq16h values for the CW data, we calculated the LAeq16hadj by limiting the 1 min LAeq values to 60 dBA if the corresponding LZpeak values were >115 dB. The result was then subtracted from the original LAeq16h to obtain LAeq16hdiff (Equationequation 5(5)
(5) ) to provide an estimate of the change in the work time noise exposure without impulse noise. The results shown in show that the maximum change in the daytime noise exposure was about 4.5 dB with over 100 peaks removed, e.g. the LAeq16h for that crewmember would have been 4.5 dB lower without impulse noise. There were only a small number of cases in which the estimated change in LAeq16h was more than 2 dB with the impulse noise removed (6 of 36 cases). Generally, we can conclude that impulse noise only had a meaningful effect on the LAeq16h noise exposure of the crew when the number of impulses is unusually high. This relationship may be evaluated later, on a case-by-case basis.
The current study is the first analysis of impulse noise on the ISS, and there is no existing data on the duration of impulse noises on orbit. Due to high demand on crew time, it is unlikely that time signal recordings will be captured on the ISS specifically for the study of impulse noise. In the absence of this information, short (10 ms) and long (50 ms) impulse durations were chosen for the purpose of the second parametric study. The study demonstrated the hypothetical change in overall sound level that would occur if impulses of different durations, widths and quantities were added to the LAeq16h data. The differences ranged from negligible to 10 dB for the most extreme case of ten 125 dB peak, 50 ms impulses added to a baseline LAeq16h of 68.5 dBA. The data shown in suggest that 125 dB impulses are relatively infrequent, with fewer than 10 occurrences across three CW AD measurements, with the exception of one measurement activity (April 2018). Similar to our conclusions from the first parametric study, we do not expect that impulse noise, whether generated by acoustic or non-acoustic sources, would have a meaningful impact on the LAeq16h data. However, the number of impulses >125 dB in the CW data should be monitored to determine if further investigation is warranted. Such investigations could be conducted by the members of the MAS on the ground rather than the ISS crewmembers.
The ISS LAeq16h limit of 72 dBA is based on the WHO limit of 70 dBA for 24 h, over a lifetime of exposure. By contrast, the NIOSH occupational noise limit, TWA = 85 dBA, is equivalent to 82 dBA for 16 h. In a confined environment, habitability is also an important factor. Given the extended amount of time that the crewmembers spend on the ISS, it is suitable to take a conservative approach for use as a medical standard. The data show that there are occasional exceedances in of the 72 dBA limit for LAeq16h, although the TWA equivalent values are well below 85 dBA. While occasional exceedances in the conservative ISS noise limit, whether caused by impulse or continuous noise, might be of little or no consequence to crewmember hearing health, it is important for members of the MAS to identify noisy activities and advise crewmembers to wearing hearing protection accordingly. For example, since previous data have shown that the noise from the T2 reaches 80 dBA when operating at 10 mph (Allen and Denham Citation2018), wearing of hearing protection is mandatory for crewmembers when using the treadmill at high speeds. Increases in T2 noise beyond nominal levels are used to flag a maintenance requirement.
What are the possible sources of high-amplitude impulse noise on the ISS?
Noisy events such as T2 operation and maintenance work, such drilling and removing panels, are easily identified from the crew work schedules. However, it is not always possible to identify crewmember activities during the AD measurements. For the highest amplitude impulses, it is of interest to look at the 1/3rd octave band spectra, as shown in . Considering the spectra of real impact and impulse noise sources, a jackhammer has less energy at low frequencies than at high frequencies while blasts and artillery noise tend to have most of the energy between 50 and 250 Hz, decreasing at higher frequencies (Royster, Berger, and Royster Citation2000; Berglund, Hassmen, and Job Citation1996). Looking at , it is difficult to imagine an acoustic noise source that would have its highest energy below 50 Hz (low frequency), or have a steady increase in amplitude with frequency up to 20,000 Hz (high frequency). Rather, these peaks could be caused by brushing, hitting or dropping the microphone.
It is acknowledged that body-worn noise dosimeter measurements do not account for noise exposure from personal listening devices. Crewmembers are provided with custom-molded in-ear earphones, which also serve as hearing protection devices, for use with personal listening devices. Pre-flight verification of the earphones by the audiologist have shown an average of 26 ± 3 dB of personal noise attenuation. Crewmembers are instructed to not increase the output of their listening devices beyond 50% and are well-informed of the need to protect their residual hearing sensitivity through health education provided by the crew surgeons, audiologist and environments health instructors. The attenuation of ambient noise provided by the custom earphones should reduce the tendency to turn up the listening volume, as has been shown by previous research (Giguere et al. Citation2012). Nevertheless, the noise exposure caused by personal listening devices during leisure time is unknown. This is a topic for future investigation.
Should the impulse noise limit be lowered to 125 dB?
Although the ISS standard recommends a limit of 125 dB SPL to reduce startle (NASA Citation2012), the origins of this limit are not, to the authors’ knowledge, supported by literature. A previous study of the effects of impulse noise on task performance showed that participants habituated similarly to high and low level impulses (Blumenthal et al. Citation2005). However, one study of acoustic startle response (ASR) in simulated microgravity (head-down bed rest) showed that the bed rest participants did not show ASR habituation when compared to controls, and that their ASR response was initially lower than the controls (Benvenuti, Bianchi, and Angrilli Citation2011). Since the evidence for an impulse noise threshold for startle is lacking, and furthermore since ASR could be suppressed in microgravity, there is no rationale to support an impulse noise limit for startle.
As for prevention of NIHL, the only evidence we have found regarding 125 dB peak as an impulse noise limit is the suggestion of it being a critical level of hearing temporary and permanent threshold shift in chinchillas (Hight et al. Citation2003; Roberto et al. Citation1985). Based on analysis of human temporary threshold shift data, Price (Price Citation1981) suggested a critical level of 132 dB for a 5th percentile ear or 140 dB for a 25th percentile ear. There are several national and international standards that use 140 dB as the limit for impulse noise (Berglund, Lindvall, and Schwela Citation1999; National Institute for Occupational Safety and Health Citation1998; Department of Defense Citation2015). Based on literature and existing standards, there does not appear to be adequate evidence to support lowering the impulse noise limit to 125 dB peak. Since the current study showed that impulses of >125 dB peak are relatively infrequent, and that some could be associated with non-acoustic sources, a change to the impulse noise limit is not needed at this time. Continued monitoring of peak levels on the ISS is recommended so that any increases in the levels and number of occurrences can be detected and remediated.
Conclusion
We have shown that while the number of impulses in the AD data was moderately correlated with the LAeq16h, they generally did not produce a meaningful increase in the overall exposure (<2 dB in most cases). However, in unusual cases where there were over 100 impulses >115 dB in the data, the corresponding increase in LAeq16h should be investigated. We have also shown that in the case of very high impulses (>125 dB), the 1/3rd octave band data may be attributed to non-acoustic causes. The current analysis demonstrates that the impulse noise data can provide additional insight in the investigation of exceedances or abnormalities in future AD data acquired on the ISS.
Acknowledgements
The authors are members of the ISS Multilateral Medical Operations Panel, Environmental Health and Safety Group, Acoustics Sub-working Group, and represent the Canadian Space Agency (AN) and National Aeronautics and Space Administration (AB, JL and RD).
Disclosure statement
No potential conflict of interest was reported by the authors.
References
- Allen, C., and S. A. Denham. 2018. “International Space Station Acoustics - A Status Report.” Paper presented at the INTER-NOISE and NOISE-CON congress, 258 (6): 1319–1334.
- Benvenuti, S. M., M. Bianchi, and A. Angrilli. 2011. “Effects of Simulated Microgravity on Brain Plasticity: A Startle Reflex Habituation Study.” Physiology & Behavior 104 (3): 503–506. doi:10.1016/j.physbeh.2011.05.019.
- Berglund, B., T. Lindvall, and D. Schwela. 1999. Guidelines for Community Noise. Geneva, Switzerland: World Health Organization.
- Berglund, B.,. P. Hassmen, and S. F. Job. 1996. “Sources and Effects of Low Frequency Noise.” The Journal of the Acoustical Society of America 99 (5): 2985–3002. doi:10.1121/1.414863.
- Blumenthal, Terry D., Bruce N. Cuthbert, Diane L. Filion, Steven Hackley, Ottmar V. Lipp, and Anton Van Boxtel. 2005. “Committee Report: Guidelines for Human Startle Eyeblink Electromyographic Studies.” Psychophysiology 42 (1): 1–15. doi:10.1111/j.1469-8986.2005.00271.x.
- Buck, K., P. Hamery, and V. Zimpfer. 2010. “The European Regulation 2003/10/EC and the Impact of its Application to the Military Noise Exposure.” Paper presented at the Proceeding of the 20th International Congress on Acoustics, ICA 2010.
- Danielson, R., D. Henderson, M.A. Gratton, L. Bianchi, and R. Salvi. 1991. “The Importance of “Temporal Pattern” in Traumatic Impulse Noise Exposures.” The Journal of the Acoustical Society of America 90 (1): 209–218. doi:10.1121/1.402361.
- Davis, Shakti K., Paul T. Calamia, William J. Murphy, and Christopher J. Smalt. 2019. “In-Ear and on-Body Measurements of Impulse Noise Exposure.” International Journal of Audiology 58 (sup1): S49–S557. doi:10.1080/14992027.2018.1534012.
- Department of Defense. 2015. “Design Criteria Standard: Noise Limits.” MIL-STD-1474E. https://www.arl.army.mil/www/pages/343/MIL-STD-1474E-Final-15Apr2015.pdf.
- Giguere, C., A. Behar, H. R. Dajani, T. Kelsall, and S. E. Keith. 2012. “Direct and Indirect Methods for the Measurement of Occupational Sound Exposure from Communication Headsets.” Noise Control Engineering Journal 60 (6): 630–644. doi:10.3397/1.3701037.
- Hamernik, R. P., and K. D. Hsueh. 1991. “Impulse Noise: Some Definitions, Physical Acoustics and Other Considerations.” The Journal of the Acoustical Society of America 90 (1): 189–196. doi:10.1121/1.401287.
- Henderson, D., M. Subramaniam, M. Gratton, and S. Saunders. 1991. “Impact Noise: The Importance of Level, Duration, and Repetition Rate.” The Journal of the Acoustical Society of America 89 (3): 1350–1357. doi:10.1121/1.400658
- Hight, Nancy G., Sandra L. McFadden, Donald Henderson, Robert F. Burkard, and Thomas Nicotera. 2003. “Noise-Induced Hearing Loss in Chinchillas Pre-Treated with Glutathione Monoethyester and R-PIA.” Hearing Research 179 (1–2): 21–32. doi:10.1016/S0378-5955(03)00067-4.
- Kardous, Chucri A., and Robert D. Willson. 2004. “Limitations of Using Dosimeters in Impulse Noise Environments.” Journal of Occupational and Environmental Hygiene 1 (7): 456–462. doi:10.1080/15459620490465839.
- Limardo, J., C. Allen, and R. Danielson. 2017. “International Space Station Crewmember’s Noise Exposures from 2015 to Present”. Paper presented at the Proceedings of the 47th International Conference on Environmental Systems, 16–20 July 2017, Charleston, South Carolina. ICES-2017-191.
- NASA. 2012. SSP 50260 (rev C) International Space Station Medical Operations Requirements Document. Houston, TX: Johnson Space Center.
- National Institute for Occupational Safety and Health.1998. Occupational Noise Exposure: Revised Criteria DHHS (NIOSH) Publication No. 98–126. https://www.cdc.gov/niosh/docs/98-126/pdfs/98-126.pdf
- Price, G.R. 1981. “Implications of a Critical Level in the Ear for Assessment of Noise Hazard at High Intensities.” The Journal of the Acoustical Society of America 69 (1): 171–177. doi:10.1121/1.385361.
- Roberto, M., R.P. Hamernik, S. Salvi, D. Henderson, and R. Milone. 1985. “Impact Noise and the Equal Energy Hypothesis.” The Journal of the Acoustical Society of America 77 (4): 1514–1520. doi:10.1121/1.391993.
- Royster, L. H., E. H. Berger, and J. D. Royster. 2000. “Noise Surveys and Data Analysis.”, In The Noise Manual, edited by E. H. Berger. 5th ed. Fairfax, VA: American Industrial Hygiene Association.
- Smalt, Christopher J., Joe Lacirignola, Shakti K. Davis, Paul T. Calamia, and Paula P. Collins. 2017. “Noise Dosimetry for Tactical Environments.” Hearing Research 349: 42–54. doi:10.1016/j.heares.2016.11.008.