Abstract
Objective
This study investigated the use of bone-conduction headsets paired to a wireless, remote microphone on speech discrimination and word identification for children with normal hearing.
Design
Children were tested with and without the headset, using the McCormick speech discrimination test in quiet and in speech-shaped noise to measure word-discrimination thresholds. Additionally, open-set word identification in noise was assessed while children were simultaneously engaged in a visual-monitoring task.
Study sample
Twenty normal-hearing children, aged 4–11 years.
Results
Median word-discrimination threshold in quiet (n = 20) was 20.5 dB(A) without a headset and 11.5 dB(A) with a headset (Z = –3.826, p = 0.0001). In noise, the median word-discrimination threshold (n = 20) was 52 dB(A) without a headset and 40.5 dB(A) with a headset (Z = –3.926, p< 0.0001). For open-set word identification (n = 11), children performed significantly better with a headset than without it, with an average improvement of 23 percentage points (t(10) = −5.227, p = 0.0004, two tailed).
Conclusions
A bone-conduction headset paired to a Bluetooth microphone improved discrimination of distant speech in quiet and in noise and open-set word identification in noise.
Background
It is commonly acknowledged that classrooms can be noisy environments and that children with hearing loss often struggle to understand speech in these settings (e.g., Lewis, Valente, and Spalding Citation2015; Noh and Park Citation2012; Hicks and Tharpe Citation2002). Even for normal-hearing children, noise can be disruptive for learning: children require a higher speech to noise ratio (SNR) to achieve the same performance than adults in speech-identification tasks, especially if English is not their first language or if they have a language or communication disability (Nelson and Soli Citation2000; Vickers et al. Citation2013). A previous study (Holland Brown et al. Citation2019), showed that wearing an off-the-shelf bone-conduction (BC) headset developed for the leisure industry, when paired with a remote, wireless microphone worn by the main talker, significantly improved speech discrimination in noise for children with otitis media with effusion (OME). It is not clear whether children with normal hearing benefit from a BC headset to the same extent as children with OME when paired to a remote, wireless microphone to improve understanding at a distance, in noise, or in situations with reverberation. The advantage of BC headsets rather than traditional headphones that cover the ear, is that BC headsets do not cover the ear canal, ensuring the student is not excluded from discussions on their table or with their classmates, provided their voices are audible via air conduction (AC) and are not masked by teacher’s voice (Tharpe, Ricketts, and Sladen Citation2003). On the other hand, if the sound quality of the BC device were degraded (Letowski, Mermagen, and Vause Citation2004), due to limitations in its frequency response or due to distortion, the BC signals could be a hindrance. There are no reports on whether it is advantageous, in terms of speech discrimination or identification, for children to wear a BC headset paired to a remote, wireless microphone when AC thresholds are normal. This is of interest, not only for normal-hearing children, but also for children who have OME, who are likely to go through periods of having normal hearing thresholds given the fluctuating nature of their hearing loss, or for children who have other hearing conditions, such as Auditory Processing Disorder (APD).
The primary aim of this study was to determine whether speech discrimination improved when using a BC headset paired with a remote, wireless microphone for children with normal hearing thresholds. Word-discrimination thresholds were adaptively measured using the McCormick Toy Test (McCormick Citation1977) in quiet and in the presence of speech-shaped noise.
Additionally, speech identification scores for open-set words with and without the headset were obtained while the children were engaged in a visual-monitoring task. The original motivation for collecting these data was to explore listening effort with and without the headset. A dual-task paradigm experiment (Picou, Charles, and Ricketts Citation2017), composed of a speech-identification task and a visual-monitoring task was carried out. However, only speech identification outcomes are reported, as the number of children recruited was not enough to reach an appropriate sample size to derive statistically valid analyses of the visual-monitoring reaction-time data (Brysbaert and Stevens Citation2018). Analysis of the data for the word-recognition component was adequately powered.
Finally, the subjective impressions of the children about the wearability and comfort of the headphones were collected by using a bespoke questionnaire.
We hypothesised that both word discrimination and identification would improve when a headset paired with a remote, wireless microphone was used, and that most children would find the headset comfortable and acceptable.
Methods
Participants
Children were recruited from local schools and the Peacock Children’s Centre in Cambridge. Inclusion criteria were children aged 4–11 years, with no developmental concerns, and no concerns about hearing loss or language development. English needed to be the child’s main language. At assessment, only the children who passed a hearing screen at 20 dB HL with type A tympanometry traces (Jerger, Citation1970) were included. Exclusion criteria were: any history, risk or concern of hearing loss, developmental or language disabilities and English as a non-dominant language. Twenty children (median age 7 years 5 months, range: 4 years 5 months − 11 years 6 months) participated.
National Health Service Ethical approval was obtained from South Central – Oxford C Research Ethics Committee (Integrated Research Application System [IRAS] Project ID:251222, REC Reference 19/SC/0106). The study was registered in clinicaltrials.gov (identifier NCT03695575). Informed consent and assent were obtained from parents and children respectively. Reimbursement of travel expenses was offered. Children were given a toy, refreshments, and a certificate to acknowledge their participation.
Basic hearing evaluation
An otoscopic examination was carried out using a Welch Allyn 3.5v Otoscope. Tympanometry was performed with an Interacoustics Titan tympanometer using a 226 Hz probe. Air-conduction (AC) hearing sensitivity was screened at 20 dB across four frequencies (500, 1000, 2000, and 4000 Hz) using a “Kamplex KLD 23 mha” audiometer and TDH-39P headphones, employing an age-appropriate technique (either conditioned-play or conventional button-press response audiograms).
BC headset and remote wireless microphone
The BC headset and lapel microphone set-up used here was similar to that used in a previous study (Holland Brown et al. Citation2019). The BC headset was an Aftershokz Aeropex (), which consisted of two BC transducers that rest on the zygomatic arches in front of the ears and are supported by a band that runs around the back of the head/neck. The frequency response of the headset presents variations in output within a 10-dB range around 1,000 and 10,000 Hz; Between 100 and 1000 Hz, variations of output level occur within the range of 20 dB (Manning, Mermagen, and Scharine Citation2017). This headset has been shown to improve speech discrimination in quiet (Holland Brown et al. Citation2019) and in noise (Manning, Mermagen, and Scharine Citation2017; Holland Brown et al. Citation2019). The headset was paired with a Sena lapel microphone via Bluetooth. This remote, wireless microphone has a sampling rate of 48,000 Hz and uses a proprietary noise-reduction algorithm. Before each test session, listening checks were performed to verify that the headset and the microphone were appropriately paired. The volume of the headset was set to three steps below the maximum level and each child was asked whether the sound level was comfortable in volume before carrying out the tests. All children accepted the default setting.
Closed-set discrimination task: automated McCormick Toy Test
As in our previous study (Holland Brown et al. Citation2019), speech discrimination was measured using an automated version of the McCormick Toy Test (McCormick Citation1977) in quiet and in background noise. The speech level that led to 71% correct speech discrimination, termed “word-discrimination threshold” (WDT) (Summerfield et al. Citation1994), was determined. The test was carried out inside a sound-attenuating booth. The McCormick test includes 14 words that are paired in terms of vowel, but contrast in the consonants. For example: cup and duck, or horse and fork. These test items are represented by toys, which are laid out in front of the child before testing. Each child was seated at a small table which had the McCormick toys on its surface. Before starting the test, it was verified that the child was familiar with the vocabulary of the test. Words were played using a Phoenix Automated Speaker system (Soundbyte Solutions Ltd.) operated by an experienced tester. On each trial, a pre-recorded female speaker asked the child to point to a randomly selected item using a carrier phrase. For example: “Where’s the tree?” The presentation level of the speech was automatically adjusted depending on whether the child pointed to the correct item, using an adaptive algorithm in two stages. First, the appropriate level range for testing was determined by achieving two reversals following a one-up one-down procedure with a step size of 12 dB. Then, the final outcome was determined using a two-down one-up procedure with 6-dB steps and six reversals. The outcome of the test was updated with each reversal. In order to optimise data collection and reduce fatigue effects, the number of reversals was decreased from the default 6 to 5. Decreasing the number of reversals has been shown to have no significant effect on the WDT (Hall, Munro, and Heron Citation2007). The loudspeaker of the Phoenix was placed 2 m away from the child, at an azimuth of 0° and roughly at the height of the child’s head. The distance between the loudspeaker and the child aimed to replicate the distance between teacher and a pupil sitting in the first row of a standard classroom (Department for Education Citation2014). A Kamplex KM4 sound level meter was used to calibrate the speech level to be 60 dB(A) at 1 m from the loudspeaker, following the calibration method recommended by the manufacturer. However, since children sat at 2 m from the loudspeaker, levels were measured at the position of the child’s head to estimate actual presentation level. Therefore, WDTs are expressed as sound level measured at the position of the children’s head.
The conditions tested were given by the use of the headset (either with or without headset) and the background (quiet or speech-shaped noise). Therefore, there were four test conditions. For the tests in noise, speech-shaped noise was generated using an Interacoustics Equinox Audiometer and delivered via a Guymark loudspeaker located to the right-hand side of the child at 1.66 m distance and 90° azimuth. For the tests with the headset on, the remote, wireless microphone was placed in front and slightly below the loudspeaker, simulating a location on the lapel of a teacher. The level of the noise was calibrated to be 62 dB(A) at the position of the child’s head and was kept constant during the test. This level was assumed to be representative of the noise level in a standard classroom in use (Jamieson et al. Citation2004).
The order of conditions (quiet–no headset, quiet–headset, noise–no headset, noise–headset) was randomised across children to control for learning and fatigue effects. After the four runs were completed, children were offered a break. If children were happy to continue testing after the break, they were invited to perform the open-set identification task.
Open-set identification task
Open-set identification was tested within a listening effort dual-task paradigm task (Picou, Charles, and Ricketts Citation2017). A computer interface, written on Presentation software (Neurobehavioral Systems, Inc., Berkeley, CA, USA) and kindly provided by Erin Picou, was used to administer the test and collect the children’s responses. The test was carried out in the same sound-attenuating booth used for the McCormick test. A touch-screen was placed on a table in front of the child. The test interface presented a list of words to the child. The speech material for this task were lists of monosyllable words selected by Picou, Charles, and Ricketts (Citation2017) from previously published word lists. Picou, Charles, and Ricketts (Citation2017) created eight lists of twenty-five words each, all including only concrete nouns, and verified that intelligibility was approximately even across lists. They produced recorded lists uttered by a female American English speaker at 44,000 Hz sampling rate and 16-bits resolution. Because the same speaker was used for all conditions tested and lists were randomised across conditions, it was assumed that any decrease in the performance of the children, who were British-English speakers, due to the accent of the talker or the vocabulary used would not differ across conditions and thus not affect the conclusion of the study. With each word presentation, one of two shapes were shown on the screen. The shapes were either a yellow circle, a blue circle, a yellow triangle, or a blue triangle. Children were given the following instructions, as worded by Picou et al. (Picou, Charles, and Ricketts Citation2017) “listen to the words and repeat every word you hear. Also, as soon as you see a blue circle or yellow triangle on the screen, touch the screen to make the shape go away as quickly as possible. If you see a blue circle or yellow triangle, touch the screen before repeating the word. Do not touch the screen if you see a blue triangle or a yellow circle, instead only say the word”. For each 25-trial list, 18 contained a shape and 7 contained a small fixation cross. Visual stimuli were shown 500 ms after word onset. The proportion of probe visual trials (blue circle or yellow triangle) had a median of 9 trials per list for the headset condition (range 6–12) and 8 trials for the headset condition (range 7–15). The remainder shape trials contained the wrong colour-shape combination.
First, it was verified whether each child could do the secondary task by running the secondary task only. If a child could perform the secondary task alone, they were given one practice run without the headset before completing the task twice: once without and once with the headset. The order of conditions (with or without the headset) was randomised across children. Speech was presented at 57 dB SPL and the background noise was presented at 62 dB SPL, as measured with the sound level meter mentioned above. Thus, the SNR was −5 dB SNR. For each child and condition, word-identification scores and reaction time for the secondary task were obtained. However, due to the reduced number of children who could carry out the task, the sample size was not appropriate to carry out analyses on the reaction-time data. Thus, only the speech-identification outcomes are reported, which reflect the ability of the children to understand open-set speech in noise while focussing on a visual task which increased the cognitive load faced by the listener.
Questionnaire
At the end of the test session, a questionnaire was used to collect the children’s impressions on comfort and appearance of the headset. It was completed by the child alone or with help from the adult who accompanied them during the session. Children were told that the questionnaires were anonymous, that they could be completely honest and that there was no right or wrong answer. Free-text questions were included in order to enrich the data (Boynton and Greenhalgh Citation2004). The questionnaire is shown in .
Table 1. Responses to questionnaire on comfort and acceptability of the BC headsets by subjects.
Results
Basic hearing evaluation
Otoscopic examination revealed healthy tympanic membranes in all cases. Children were assessed to have normal air-conduction (AC) hearing thresholds better than or equal to 20 dB HL at the frequencies 500, 1000, 2000, and 4000 Hz as well as type-A tympanograms. One child who showed an abnormal tympanometry trace did not continue any further with testing and was instead referred into the NHS Audiology Service for further evaluation.
Word-discrimination thresholds
shows the WDTs in quiet and in noise for the group. The effect of the headset was assessed separately in quiet and in noise. Statistical analysis was performed using SPSS (IBM SPSS Statistics for Macintosh, Version 27.0 (SPSS Inc., Chicago, IL, USA). Because data were not normally distributed for some of the conditions, non-parametric analyses were carried out using the related-samples Wilcoxon Signed Rank Test. The median for the conditions in quiet and in noise were compared separately. In quiet, the median WDT was 20.5 dB(A) (range: 13–31) without a headset and 11.5 dB(A) (range: 7–16) with a headset. The difference between these two thresholds was statistically significant (Z = −3.826, p = 0.0001, effect size dz = 2.49Footnote1). In noise, the median WDT was 52 dB(A) (range: 48–63) without a headset and 40.5 dB(A) (range: 33–49) with a headset. The difference was also statistically significant (Z = −3.926, p = 0.00009, effect size dz = 3.27). The median improvement of the WDTs with a headset was 10 dB in quiet and 13 dB in noise.
Figure 2. WDT for each condition (“Headset” and “No Headset”) and background (quiet or noise). Individual data points are shown by white circles. The horizontal lines denote the medians. Boxes enclose the interquartile range, and whiskers show the upper and lower quartiles. Data points above or below the upper or lower quartiles, respectively, are outliers. Figure made using the ggplot2 package (Wickham Citation2016) in R (R Core Team Citation2021).
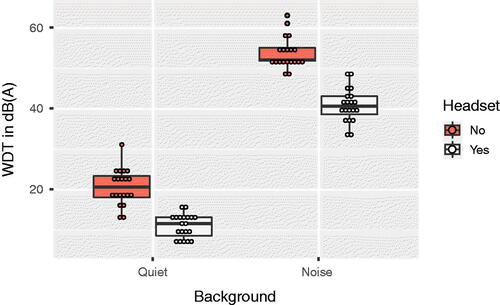
Open-set identification
Results are available for eleven children. Percent-correct word-identification scores are shown in . Children’s word identification was significantly higher when the children were wearing a headset. On average, children identified 14 words correctly (57%, SD = 9.31) without a headset and 20 words (80%, SD = 10.12) with a headset. Percent-correct scores were transformed into rationalised arcsine units (RAU) for statistical analysis. Using the headset led to a 23-percentage-point average increase in word identification. A paired-samples t-test showed that this difference was statistically significant (t(10) = −5.227, p = 0.0004, two tailed, effect size dz = 2.21).
Figure 3. Percent correct scores for the open-set word-identification task. The horizontal lines denote the medians. Boxes enclose the interquartile range, and whiskers show the upper and lower quartiles. Data points above or below the upper or lower quartiles, respectively, are outliers. Figure made using the ggplot2 package (Wickham Citation2016) in R (R Core Team Citation2021).
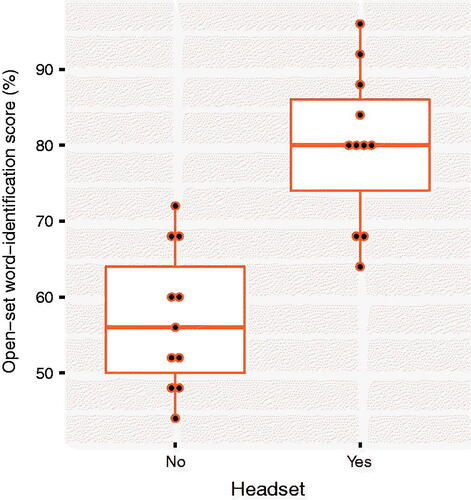
Questionnaire
Fifteen anonymous questionnaires were returned (15/20) giving a 75% return rate for the sample. summarises the responses to the questions. Overall, the general opinion of the children about the headset was positive (“good”, “amazing”, “cool”) or neutral (“OK”) although three out of the fifteen children gave negative feedback. In one case, the negative feedback was based on the appearance (“weirdly shaped”). However, all children described the look of the headset using positive or neutral words. The other cases of negative feedback were related to the sound quality, which was described as “echoey” by two children. Responses reflected that most children thought that the headset would be accepted by their peers, but three children thought that the device would not be accepted (“weird”, “bit strange”, “rubbish”). One child gave a neutral response (“not sure”). The headset was rated as “comfortable” and “easy to put on” by nearly all children (n = 13 in both cases), and no reports of the headset being uncomfortable were made. All children reported that the headset was easy to use.
To the one question about the effect of the headset on the children’s impressions of their own hearing experience, eleven children gave positive feedback such as “brilliant”, “fun”, “better”, “it is easy to hear”, “it is clear”, “very good”. However, three children gave neutral or negative answers, describing the sound as “echoey and louder”, and stating that “it was hard to hear the quieter sounds” (which may have reflected the difficulty of the test), “it was buzzing” (which may have referred to vibrotactile sensation or to sound quality). One child did not provide an answer.
We included a question intended to gather information about the children’s impressions on listening effort. The question was: “Was it easier to concentrate while wearing it?” While eight children responded “yes”, one replied “no” and four replied “I don’t know”. Two children left this question blank.
Discussion
We have assessed the effect of wearing a BC headset paired with a Bluetooth microphone on the discrimination of distant speech in quiet and in noise for children with normal hearing sitting in a classroom-like configuration. The experimental setup aimed to be representative of a standard classroom with usual background noise, spatially separated (as if produced from other children or nearby classrooms) from a pupil sitting 2 m away from the teacher.
It is of interest to report the SNRs at which normal-hearing children performed the McCormick test in noise, without the headset. The median SRT was achieved at −10 dB SNR (range −14–+1). Children significantly improved their performance in quiet and in noise when they were wearing the headset, with a median improvement of 10 dB in quiet and 13 dB in noise.
In quiet, the median threshold without a headset was 20.5 dB(A) (range: 13-31) and this reduced to 11.5 dB(A) (range: 7-16) with a headset. With speech-shaped background noise, without a headset, children reached the WDT at a median of 52 dB(A) (range: 48–63) and this improved to 40.5 dB(A) (range: 33–49) when they were wearing a headset. The difference across conditions with and without a headset was statistically significant in noise and in quiet. These improvements are similar to that reported by Tharpe, Ricketts, and Sladen (Citation2003) for children with minimal hearing loss using a frequency modulated remote microphone system (FM system) binaurally with open ear canals where speech was delivered from the frontal position. The differences in the median thresholds with and without the headsets were slightly bigger for the children tested in our previous study (Holland Brown et al. Citation2019), which were 16 dB in quiet and 14 dB in noise, compared to 9 and 11.5 for the present study. However, it is worth mentioning that, apart from the differences expected from the fact that children in that study had OME, the Phoenix loudspeaker was placed at 3 m from the children, and not at 2 m as in the present study.
The WDTs in quiet without the headset are generally consistent with expected outcomes for normal-hearing children. In the Avon Longitudinal Study of Parents and Children (ALSPAC) study, (Hall, Munro, and Heron Citation2007) reported a level of 28 dB(A) for children with normal hearing using the automated McCormick toy test at the age of 31 months. The WDT measured here, 20.5 dB(A), is likely to be due to the inclusion of older children in this sample. An average WDT of 18.6 dB(A) has been reported for adults (Ousey et al., Citation1989).
As in our previous study (Holland Brown et al. Citation2019), only one repetition of the outcome measures was obtained for each child for each condition. Collecting a single repetition per child and condition makes the outcomes susceptible to random variability. However, the differences observed at the individual level for both the WDT and the open-set word-identification task were large in most cases. For the WDT, differences were mostly greater than the critical difference of 7 dB for the WDT in quiet (Summerfield et al. Citation1994) and the 6.8 dB critical difference for the WDT in noise (Lovett, Summerfield, and Vickers Citation2013). For all participants, differences across conditions with and without a headset ranged between 0 and 18 dB for the test in quiet and 4 and 25 dB for the test in noise, with all but four children showing differences of 7 dB or smaller in quiet, and only one child showing a difference smaller than 7 dB in noise.
We obtained open-set word-identification scores in the context of a dual-task paradigm measure of listening effort. The dual task consisted of an open-set word-identification task (primary task) and a visual-monitoring task (secondary task). Unfortunately, due to the small number of children that were able to carry out the task, it was not possible to analyse the outcomes for the visual-monitoring task to assess differences in listening effort. Outcomes for the open-set word-identification task could be statistically analysed and are reported. Word identification was largely improved by wearing the headset, with a highly significant difference of 23 percentage points across identification scores.
Finally, we requested feedback from the participants about the wearability and ease of use of the headset. The response rate was 75%. Because the questionnaire was offered at the end of the session, many children may have been tired after testing, resulting in five children (25%) not taking part in answering the questionnaire. Most children accepted the headset and found it easy to use. Some children gave positive feedback about their listening experience with the headset and a small number gave reports of adverse sound quality, which are discussed above.
This study could be of interest for normal-hearing children, but also for children with OME, who are likely to go through periods of having normal-hearing thresholds given the fluctuating nature of their hearing loss, or for children with other hearing disorders, such as “Auditory Processing Disorder” (APD). Children with APD typically have normal-hearing thresholds for pure tones but struggle to understand speech, especially in noisy environments. APD has detrimental effects on language, speech, literacy, attention, and academic performance (Moore et al. Citation2018). Although there is no universally accepted diagnostic criteria or test battery for APD and there is much yet to be understood about the mechanisms underlying it, there is evidence supporting the use of remote-microphone devices to improve the signal-to-noise ratio (SNR) (Keith and Purdy Citation2014; Johnston et al. Citation2009; Schafer et al. Citation2014).
Wireless remote-microphone technology may improve listening experience in noisy and reverberant environments such as the classroom. One approach is through the use of remote-microphone hearing assistance technologies, RMHAT (American Academy of Audiology Citation2008), which are composed of a microphone worn by the speaker (usually the teacher in the classroom) and a receiver worn by the student. The purpose of the microphone being close to the mouth of the speaker is to reduce background noise, distance and reverberation effects and therefore the SNR of the spoken message is improved (Thibodeau Citation2014). There are RMHAT systems designed for normal-hearing users which have been shown to be effective for children with APD (Johnston et al. Citation2009; Friederichs and Friederichs Citation2005; Sharma, Purdy, and Kelly Citation2012) and for children with an autism spectrum diagnosis, providing benefits in listening, communication, social interaction, and salivary cortisol (reflecting stress levels) in group or one-to-one listening situations (Rance et al. Citation2017). However, although these devices may offer more flexible fitting options, their provision is limited by their relatively high cost. Another difference across the setup used here and RMHATs is that the latter stimulate using air conduction, and are typically provided by qualified audiologists with considerable expertise. Nevertheless, even though the setup described here could be described as an “over the counter” device, it would ideally be used with involvement of qualified health professionals. Over the counter devices offer a potential solution to remote care, which is in demand due to long waiting lists, especially after the onset of the COVID-19 pandemic (Holland Brown et al. Citation2021).
Limitations
The experimental set-up for the determination of the WDT had some limitations in terms of ecological validity. First, the use of an adaptive task in order to measure the WDT might have led to SNRs that are not representative of real environments (Naylor Citation2016). Additionally, a single-source of lateral steady-state noise was used, which is unrealistic compared to school environments (Ricketts, Picou, and Galster Citation2017). However, the observed improvement in WDT is likely to be associated with benefits in other environments as it results from the improvement in SNR at the ear of the listener as a consequence of using a wireless, remote microphone. The remote microphone should improve the SNR for background modulated noise such as multi-talker babble, and for multiple sources of background noise.
As highlighted in our previous work, the difficulties in measuring the output of bone-conduction devices in general and the Aftershokz device in particular, remain a limitation. We used subjective reports of loudness comfort as a means to ensure appropriate listening levels.
It is also worth mentioning that, as the remote, wireless microphone used here has a built-in noise reduction algorithm, it is not possible to separate the contributions of the headset itself and the remote, wireless microphone. It is possible that, if a different microphone had been used, different results would have been obtained.
It is not possible to pair several headsets to the same microphone. This would be problematic if there were more than one child in the class who needed the headset.
The word-identification data was collected within a dual-task paradigm experiment. Children under the age of 7 were generally unable to perform the visual-monitoring task. The outcome of this task, which consisted of RTs for estimation of listening effort, could not be analysed. The RT data are not reported here but can be obtained from the authors to serve the purpose of planning future research. Some details can be found in the Supplementary Materials. The outcomes for the word-identification task were reported here as they represent open-set identification in conditions when the listener is faced with a simultaneous task (for instance, as in Bizley et al. 2015 and Salorio-Corbetto et al. 2022). This increases the cognitive load faced by the listener, as is often the case in real life. Outcomes measured in dual-task paradigms may reflect a trade-off made by the listener in order to maximise their overall performance. It is possible that a word-identification task performed in isolation might have led to a smaller effect size. The assumption underlying this is that, if speech discrimination were challenging, children might have chosen to focus on the visual task instead. Although this may decrease the word-identification scores without the headset, it would ultimately reflect the compromises that listeners establish when listening in noisy environments while carrying out other activities.
Summary and conclusions
In summary, this study showed that wearing a BC headset paired with a Bluetooth microphone improved the discrimination of speech in quiet and in noise in a classroom-like configuration, as well as the identification of open-set words in noise for children with normal hearing. A bespoke questionnaire revealed that the majority of children gave positive comments about the headset and reported that it was comfortable to wear and easy to use. Therefore, the headset could be considered as a potential solution for children who have normal hearing but need an improved SNR to process instructions more easily, such as children with auditory processing difficulties, or for children with OME even at the times that their hearing thresholds are normal.
Supplemental Material
Download MS Word (20.1 KB)Acknowledgments
The authors are very grateful to Erin Picou for kindly providing her Listening Effort Test procedure and code. We thank Dr Alison Sansome for her support on behalf of the sponsor of the study and Dr Debi Vickers for helpful discussion around the topic of the manuscript.
Disclosure statement
Dr Tamsin Holland Brown conceptualised the idea of using bone-conduction headphones paired to a Bluetooth microphone to support children with glue ear and auditory-processing difficulties but has not benefitted financially. The other authors did not declare any conflicts of interest.
Data availability statement
Anonymised data are available on request to the authors.
Additional information
Funding
Notes
1 Effect sizes were calculated using G*Power 3 (Faul et al. 2007) assuming a correlation of 0.5 across groups
References
- American Academy of Audiology. 2008. “American Academy of Audiology Clinical Practice Guidelines: Remote Microphone Hearing Assistance Technologies for Children and Youth from Birth to 21 Years.” https://audiology-web.s3.amazonaws.com/migrated/HAT_Guidelines_Supplement_A.pdf_53996ef7758497.54419000.pdf.
- Bizley, J. K., N. Elliott, K. C. Wood, and D. A. Vickers. 2015. “Simultaneous Assessment of Speech Identification and Spatial Discrimination: A Potential Testing Approach for Bilateral Cochlear Implant Users?” Trends in Hearing 19: 233121651561957. 2331216515619573. doi:10.1177/2331216515619573.
- Boynton, P. M., and T. Greenhalgh. 2004. “Selecting, Designing, and Developing Your Questionnaire.” BMJ (Clinical Research ed.) 328 (7451): 1312–1315. Available at: http://www.bmj.com/content/328/7451/1312.abstract. doi:10.1136/bmj.328.7451.1312.
- Brysbaert, M., and M. Stevens. 2018. “Power Analysis and Effect Size in Mixed Effects Models: A Tutorial.” Journal of Cognition 1 (1): 9. doi:10.5334/joc.10.
- Department for Education. 2014. “Area Guidelines for Mainstream Schools.” Building Bulletin 103. Accessed October 21, 2020. Available at: https://assets.publishing.service.gov.uk/government/uploads/system/uploads/attachment_data/file/905692/BB103_Area_Guidelines_for_Mainstream_Schools.pdf.
- Faul, F., E. Erldfelder, A.-G. Lang, and A. Buchner. 2007. “G*Power 3: A Flexible Statistical Power Analysis Program for the Social, Behavioral, and Biomedical Sciences.” Behavior Research Methods 39 (2): 175–191. doi:10.3758/bf03193146.
- Friederichs, E., and P. Friederichs. 2005. “Electrophysiologic and Psycho-Acoustic Findings following One-Year Application of a Personal Ear-Level FM Device in Children with Attention Deficit and Suspected Central Auditory Processing Disorder.” Journal of Learning Disabilities 12: 31–36.
- Hall, A. J., K. J. Munro, and J. Heron. 2007. “Developmental Changes in Word Recognition Threshold from Two to Five Years of Age in Children with Different Middle Ear Status.” International Journal of Audiology 46 (7): 355–361. doi:10.1080/14992020701331570.
- Hicks, C. B., and A. M. Tharpe. 2002. “Listening Effort and Fatigue in School-Age Children with and without Hearing Loss.” Journal of Speech, Language, and Hearing Research 45 (3): 573–584. doi:10.1044/1092-4388(2002/046).
- Holland Brown, T. M., M. Salorio-Corbetto, R. Gray, A. James Best, and J. E. Marriage. 2019. “Using a Bone-Conduction Headset to Improve Speech Discrimination in Children with Otitis Media with Effusion.” Trends in Hearing 23: 2331216519858303–2331216519858309. doi:10.1177/2331216519858303.
- Holland Brown, T. M., I. Fitzgerald O’Connor, J. Bewick, and C. Morley. 2021. “Bone Conduction Hearing Kit for Children with Glue Ear.” BMJ Innovations 7 (4): 600–603. doi:10.1136/bmjinnov-2021-000676.
- Jamieson, D.G., G. Kranj, K. Yu, and W. E. Hodgetts. 2004. “Speech Intelligibility of Young School-Aged Children in the Presence of Real-Life Classroom Noise.” Journal of the American Academy of Audiology 15 (7): 508–517. doi:10.3766/jaaa.15.7.5.
- Jerger, J. 1970. “Clinical Experience with Impedance Audiometry.” Archives of Otolaryngology- Head and Neck Surgery 92 (4): 311–324. doi:10.1001/archotol.1970.04310040005002.
- Johnston, K. N., A. B. John, N. V. Kreisman, J. W. Hall III, and C. C. Crandell. 2009. “Multiple Benefits of Personal FM System Use by Children with Auditory Processing Disorder (APD).” International Journal of Audiology 48 (6): 371–383. doi:10.1080/14992020802687516.
- Keith, W. J., and S. C. Purdy. 2014. “Assistive and Therapeutic Effects of Amplification for Auditory Processing Disorder.” Seminars in Hearing 35 (01): 027–038. doi:10.1055/s-0033-1363522.
- Letowski, T., T. Mermagen, and N. Vause. 2004. “Bone Conduction Communication in Noise: A Preliminary Study.” Proceedings of the XXI International Congress on Sound and Vibrations, 3037–3044.
- Lewis, D. E., D. L. Valente, and J. L. Spalding. 2015. “Effect of Minimal/Mild Hearing Loss on Children’s Speech Understanding in a Simulated Classroom.” Ear & Hearing 36 (1): 136–144. doi:10.1097/AUD.0000000000000092.
- Lovett, R., Q. Summerfield, and D. Vickers. 2013. “Test-Retest Reliability of the Toy Discrimination Test with a Masker of Noise or Babble in Children with Hearing Impairment.” International Journal of Audiology 52 (6): 377–384. doi:10.3109/14992027.2013.769064.
- Manning, C., T. Mermagen, and A. Scharine. 2017. “The Effect of Sensorineural Hearing Loss and Tinnitus on Speech Recognition over Air and Bone Conduction Military Communications Headsets.” Hearing Research 349: 67–75. doi:10.1016/j.heares.2016.10.019.
- McCormick, B. 1977. “The Toy Discrimination Test: An Aid for Screening the Hearing of Children above a Mental Age of Two Years.” Public Health 91 (2): 67–74. doi:10.1016/s0033-3506(77)80003-6.
- Moore, D. R., N. G. Campbell, S. Rosen, et al. 2018. Position Statement and Practice Guidance: Auditory Processing Disorder (APD). British Society of Audiology.
- Naylor, G. 2016. “Theoretical Issues of Validity in the Measurement of Aided Speech Reception Threshold in Noise for Comparing Nonlinear Hearing Aid Systems.” Journal of the American Academy of Audiology 27 (7): 504–514. doi:10.3766/jaaa.15093.
- Nelson, P. B., and S. Soli. 2000. “Acoustical Barriers to Learning: Children at Risk in Every Classroom.” Language, Speech, and Hearing Services in Schools 31 (4): 356–361. doi:10.1044/0161-1461.3104.356.
- Noh, H., and Y.-G. Park. 2012. “How Close Should a Student with Unilateral Hearing Loss Stay to a Teacher in a Noisy Classroom?” International Journal of Audiology 51 (6): 426–432. doi:10.3109/14992027.2012.654855.
- Ousey, J., S. Sheppard, T. Twomey, and A. R. Palmer. 1989. “The IHR-Mccormick Automated Toy Discrimination Test—description and Initial Evaluation.” British Journal of Audiology 23 (3): 245–249. doi:10.3109/03005368909076506.
- Picou, E. M., L. M. Charles, and T. A. Ricketts. 2017. “Child-Adult Differences in Using Dual-Task Paradigms to Measure Listening Effort.” American Journal of Audiology 26 (2): 143–154. doi:10.1044/2016_AJA-16-0059.
- R Core Team. 2021. R: A Language and Environment for Statistical Computing. Vienna, Austria: R Foundation for Statistical Computing. https://www.R-project.org/.
- Rance, G., D. Chisari, K. Saunders, and J.-L. Rault. 2017. “Reducing Listening-Related Stress in School-Aged Children with Autism Spectrum Disorder.” Journal of Autism and Developmental Disorders 47 (7): 2010–2022. doi:10.1007/s10803-017-3114-4.
- Ricketts, T. A., E. M. Picou, and J. Galster. 2017. “Directional Microphone Hearing Aids in School Environments: Working toward Optimization.” Journal of Speech, Language, and Hearing Research 60 (1): 263–275. doi:10.1044/2016_JSLHR-H-16-0097.
- Salorio-Corbetto, M., B. Williges, W. Lamping, et al. 2022. “Evaluating Spatial Hearing Using a Dual-Task Approach in a Virtual-Acoustics Environment.” Frontiers in Neuroscience 16: 1–17. Article number 787153. doi:10.3389/fnins.2022.787153
- Schafer, E. C., S. Florence, C. Anderson, J. Dyson, S. Wright, K. Sanders, and D. Bryant. 2014. “A Critical Review of Remote-Microphone Technology for Children with Normal Hearing and Auditory Differences.” Journal of Educational Audiology 20: 1–11.
- Sharma, M., S. C. Purdy, and A. S. Kelly. 2012. “A Randomized Control Trial of Interventions in School-Aged Children with Auditory Processing Disorders.” International Journal of Audiology 51 (7): 506–518. doi:10.3109/14992027.2012.670272.
- Summerfield, Q., A. R. Palmer, J. R. Foster, D. H. Marshall, and T. Twomey. 1994. “Clinical Evaluation and Test-Retest Reliability of the IHR-McCormick Automated Toy Discrimination Test.” British Journal of Audiology 28 (3): 165–179. doi:10.3109/03005369409086564.
- Tharpe, A. M., T. Ricketts, and D. P. Sladen. 2003. “FM Systems for Children with Minimal to Mild Hearing Loss.” Chapter 20. ACCESS: Achieving Clear Communication Employing Sound Solutions: 191–197.
- Thibodeau, L. 2014. “Comparison of Speech Recognition with Adaptive Digital and FM Remote Microphone Hearing Assistance Technology by Listeners Who Use Hearing Aids.” American Journal of Audiology 23 (2): 201–210. doi:10.1044/2014_AJA-13-0065.
- Vickers, D. A., B. C. Bradford, N. C. Macdonald, N. K. Rostamzadeh, N. K. Mason, R. Pandya, and J. E. Marriage. et al. 2013. “Using Personal Response Systems to Assess Speech Perception within the Classroom: An Approach to Determine the Efficacy of Sound Field Amplification in Primary School Classrooms.” Ear and Hearing 34 (4): 491–502. doi:10.1097/AUD.0b013e31827ad76f.
- Wickham, H. 2016. ggplot2: Elegant Graphics for Data Analysis. New York: Springer-Verlag. Available at: https://ggplot2.tidyverse.org.