Abstract
Objective
To objectively measure audibility in patients wearing bone conduction devices (BCDs) with a new approach using a skin microphone at the patient’s forehead.
Design
The skin microphone was attached by a softband and shielded by an earmuff. This set-up was confirmed not to be influenced by neither noise floor nor sound bypassing the BCD. Sound field warble tones were used for measuring aided hearing thresholds and maximum power output (MPO) whereas an international speech test signal (ISTS) was presented at different speech levels.
Study sample
29 patients were tested (two were bilateral), 19 used percutaneous, eight used active transcutaneous and two used passive transcutaneous devices.
Results
The skin microphone responses at ISTS levels, hearing threshold and MPO, could be obtained in all patients. Two patients with poor audibility are highlighted in this article as examples. After adjusting the gain of the BCD, they were retested with the skin microphone (for verification) and with speech-in-noise tests (for validation). Both tests confirmed an improved audibility after the adjustments.
Conclusion
In summary, the proposed measurement of audibility of speech using a skin microphone is a promising method that can be used in a clinical setting for all types of BCDs.
Introduction
Already from the early development of percutaneous bone anchored hearing aids, there has been a need for objective measurements to evaluate the individual benefit of such devices. In order to measure the objective frequency response independent of the user, the skull simulator (SS) TU-1000 was developed (Håkansson and Carlsson Citation1989). The SS is a useful tool for verifying the hearing aid function, gain and maximum power output (MPO), but there is still no clinical method available for objective measurements of the audibility in individual patients using bone conduction devices (BCDs).
When fitting a BCD, an in-situ measurement must be performed to determine the hearing thresholds via the BCD. The appropriate gain is then calculated via a company specific software algorithm, and individual adjustments are then made based on the patient’s experience of the sound. However, from a clinical perspective, objective data regarding the extent of amplified speech reaching the patient's inner ears remains unavailable. Clinicians must instead depend on fitting software algorithms, which offer only estimated optimal fits. Additionally, clinicians rely on the patient's subjective experience of loudness and sound quality, as well as aided sound field measurements. When the fitting is done there is currently no possibility to investigate whether the patient’s hearing is within the dynamic range (DR) or not, where the DR is defined as the range between aided hearing threshold levels and the MPO using a specific BCD. In a consensus statement on BCDs and middle ear implants in patients with conductive and mixed hearing loss, the authors suggest a battery of different audiological tests to evaluate the fitting process (Maier et al. Citation2022). The consensus statement also highlights the importance that the device is fitted and programmed by an experienced audiologist so that the patients get the best possible fitting and benefit from the hearing aid, since it is still lacking an objective clinical method to measure the individual audibility.
Several studies have been published with different suggestions on methods for solutions on how to measure the audibility in patients using BCDs. In a study by Hodgetts et al. (Citation2010), three different methods were investigated to estimate the audibility of aided speech for percutaneous devices: an aided sound field approach measuring thresholds with a reference microphone right next to the bone anchored hearing aid (BAHA) microphone, a real-ear approach where an additional microphone was placed in the ipsilateral ear canal near the tympanic membrane, and an accelerometer approach where an accelerometer was attached to the actual transducer. The accelerometer approach was found to be the most accurate but this method could only be applied to percutaneous devices (Hodgetts et al. Citation2010). Mertens et al. (Citation2014) presented how MPO, and the individual DR could be calculated by measuring the input-to-output function of a Bonebridge device (MED-EL, Innsbruck, Austria), by placing a probe microphone in the contralateral ear canal (Mertens et al. Citation2014). A disadvantage of both described approaches is that they cannot be used on persons who do not have an ear canal or for other reasons cannot have a probe in the ear canal. Reinfeldt et al. (Citation2019) proposed an alternative method to measure nasal sound pressure (NSP) through the nostril, which proved to give higher signal to noise ratio than the ear canal sound pressure. The NSP method was developed primarily to verify the function of the Bone Conduction Implant (BCI) (Reinfeldt et al. Citation2015; Eeg-Olofsson et al. Citation2014) during installation surgery where ear canals are not accessible. This method was also used to follow the implant function over time, but it requires that the nostril must be tightened around the sound probe to stabilise its position and to avoid surrounding noise to pass into the nose space. Patients also have to hold their breath during the measurement (approx. 20 sec) to reduce breathing noise, which some patients experience as uncomfortable. Furthermore, the clinical status of the nasal mucosa may vary which is a limitation with this method (Sabine Reinfeldt et al. Citation2019). In Nie et al. (Citation2022), another set-up for measuring audibility was introduced. By placing a piezoelectric thin-film force transducer between the stimulation point and the bone conduction transducer the audibility of pink noise can be determined (Nie et al. Citation2022). A major limitation with this method is that it can only be used for patients wearing softband/headband.
Another way was proposed by van Berneveld et al. (Citation2018) and Snik et al. (Citation2019) who suggested a method based on “effective gain” to determine the fitting ranges of various BCD models available in the market at that time (van Barneveld et al. Citation2018; Snik et al. Citation2019). The effective gain is based on how well the BCD can close the air-bone gap and is defined as unmasked audiometric bone conduction thresholds minus aided sound field thresholds, both in dB HL. If the air-bone gap is completely closed, these two measures are equal, and the effective gain is zero which means that the conduction hearing loss is fully compensated and restored to normal with the device. This is a method to fit the gain function, assuming zero effective gain is optimum, but it does not tell how much of a speech signal that is audible. To determine the dynamic range, also the MPO must be determined, and they investigated three different methods which were found to give comparable results. Two of them are based on indirect SS measurements and the third method, more relevant to the current study as it is fully in-situ based, is using a sound probe in the ipsilateral occluded ear canal. To determine the MPO, the sound probe is used to detect when the ear canal sound level saturates. At that saturation level the MPO is determined by the input SPL at the BCD microphone. The dynamic range is then determined by the MPO plus the effective gain. No speech signals were used to measure audibility, and again a normal ear canal is required.
Despite the need, no objective method has yet been brought into clinical practice that objectively measures the audibility for patients with BCDs. This is mainly due to various limitations and that several of the proposed methods also require technical equipment such as a SS that is not available in most clinics. Other reasons might be that calibration procedures between different output domains are a bit challenging and that the time for visits is quite limited both for the patient and the clinician. All these aspects are important to consider if a new clinical method will be accepted in clinical routine – hardware should mainly be plug and play and work together with existing equipment.
Our goal when starting to investigate this area of research was to, for any type of BCD and in the same session, measure both the DR and the response at normal speech levels and present them in the same graph. Adjustments of the BCD can then be made so that as much as possible of the speech levels are within the DR. The same principle is used clinically today for objective verification of conventional air-conducted hearing aids and is then called “real ear measurement” (REM). REM is a simple and safe way to measure audibility, where the clinician, in the same graph, can see hearing thresholds, MPO, target curve and how much amplification the patient receives via the hearing aid before and after eventual adjustments. A similar method, that may be called “real bone measurement” (RBM), is highly desirable also for BCDs.
In a study by Persson et al. (Citation2022), a new promising method using a skin microphone (SM), is proposed to measure the audibility of speech in all types of BCDs (Persson et al. Citation2022). This method has met most of the desired features requested and is based on a modified skin microphone system, similar to the one developed by Hodgetts et al. (Citation2018) (Hodgetts et al. Citation2018) and comprising a shielding arrangement around the microphone casing. The whole method was dependent on a shielding arrangement to minimise ariel sound transmission from the sound field speaker directly to the microphone unit, otherwise, and in this article referred to as “by-passing” the BCD. The complete system, including microphone unit and preamplifier in a brass casing and with the shielding arrangement covering, is defined as the skin microphone (SM) system in what follows. With the SM placed on the forehead and then measuring the bone conducted vibrations radiated outwards through the skin at MPO and at hearing thresholds, the DR was determined for the individual patient. A speech signal was then presented from the sound field speakers to the BCD, with the SM in the same spot (as when measuring MPO and thresholds), and it can then be determined and visualised in a graph how much of the speech signal that maps into the DR. This method is based on that the vibration transmission through the skull bone is assumed to be linear. The linearity assumption means that, for example a 10 dB increase of the BCD mechanic output will create a 10 dB increase of the response in an arbitrary position of the skull, meaning that it will be a 10 dB increase at the forehead as well as in the cochlea. That vibration transmission through the skull bone can be assumed to be linear for speech level sounds was first shown on living subjects by Håkansson et al. (Citation1986, Citation1996). Furthermore, using this skin microphone approach, the audibility of all types of BCDs, such as percutaneous, passive and active transcutaneous BCD, should be possible to investigate as long as the skull transmitted sound is above the noise floor of the SM. It is also very convenient for the patient as active participation is only needed at the threshold measurements whereas for the MPO and speech responses no specific patient involvement is needed as they are independent of the patients neural hearing ability. The electrical output of the skin microphone can principally be connected directly to any equipment with sound probe measurement capability of air conduction devices which are available in most clinics. When measuring with the SM in the same spot, the hearing thresholds (voltage from the SM) can be used as reference and thus no calibration of the SM is needed regarding coupler to dial differences or BCD mechanic output in Newton from a SS.
In the study by Persson et al (Citation2022) it was shown that with the SM, the audibility of a speech signal could be measured on five essentially normal hearing subjects using a skin drive BAHA on a soft band (Persson et al. Citation2022). In this paper, the authors want to investigate this method further by measuring the audibility on a larger group of patients using different types of BCDs.
Aim of study
The main aim of this study is to investigate the audibility in patients fitted with different types of BCDs, using a skin microphone placed on the forehead.
A secondary aim is to verify if the results can be used to detect and then to improve the audibility in any non-optimally fitted patients.
Materials and methods
Materials
A total of 29 patients (14 males, 15 females) participated, where two of the patients had bilateral BCDs, which resulted in measurement data from a total of 31 devices. In Supplement Table 1 includes the patients’ aetiology, best BC-PTA4 hearing thresholds and BCD devices used. The average age among patients was 63.8 years (range 27-86 years) and they were diagnosed with either mixed (20 patients), or purely conductive (9 patients) hearing loss, whereas two of the patients with mixed hearing loss were rehabilitated for single sided deafness (SSD).
Percutaneous implants, or bone anchored hearing aids, were used by 19 of the patients (two of which used bilateral devices), active transcutaneous implants (BCI) by eight patients, while two patients had passive transcutaneous devices which were attached over intact skin using a softband/headband. Two patients used their BCD as contralateral routeing of signals (CROS) hearing aid, which means that their BCD transmits sound from the deaf side to the hearing ear. BCD models used were different Baha® models from Cochlear BAS (Mölnlycke, Sweden) and Ponto models from Oticon Medical (Askim, Sweden). All BCI patients used the same implanted transducer system as was used in the original clinical study (Eeg-Olofsson et al. Citation2014) at Chalmers and Sahlgrenska University Hospital, Gothenburg, Sweden, but with different audio processors: two used the original audio processor (“BCI Cth”), while others used two versions of audio processors from Oticon Medical used in their clinical studies (latest “BCI Sentio” or previous “BCI Fusion”). Pooling of patients for analysing results were made after generic type of devices in the following groups: BAHA (percutaneous), BCI (active transcutaneous), Skin (passive transcutaneous) and CROS (percutaneous) devices as shown in the left column of Supplement Table 1.
Ethic
The study was approved by Swedish Ethical Review Authority (Dnr 2019-05103 and amendment Dnr 2022-04951-02) and was conducted in accordance with the principles stated in the Declaration of Helsinki. The patients gave their informed consent to participate in the study after being informed and participation in the study was voluntary and no remuneration was given.
Methods
To determine the audibility, the same setup () was used as in the study (Persson et al. Citation2022) comprising a custom made battery-operated microphone unit and a preamplifier circuit incorporated in a stiff sound-insulated metallic casing, see . In , the microphone unit is attached with a soft hairband on the forehead. To ensure a minimum of influence of direct aerial sound transmission from the speaker, an earmuff (3 M™ PELTOR™ Optime™ III, USA) was placed over the microphone unit which both together form the skin microphone system, see .
Figure 1. Measurement set-up with equipment as described in the text (a). What is measured and controlled by the boxes “AC40,” “Callisto,” “Agilent” can, in a clinical version, be incorporated in one and the same system such as Callisto from Interacoustics, Verifit from Audioscan or similar other audiometric test systems. To the right, the brass encapsulated microphone unit (b), which is held in place on the forehead by a soft band (c) and a shielding earmuff (d) to avoid direct sound from the speaker to interfere with the microphone unit, altogether forming the skin microphone system.
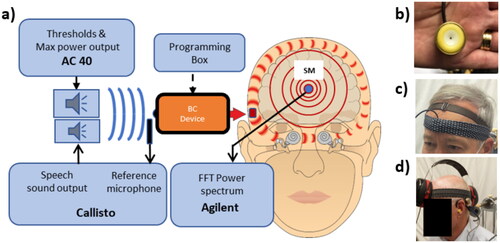
Before the measurements started, all automatic functions in the audio processors, such as feedback manager, noise manager and impulse manager, were turned off and the microphones were set in omnidirectional mode. No other adjustments were made to the BCDs which were the patients’ presently used devices. The following measurements were conducted, and for all of them the SM and BCDs were in place: MPO and hearing thresholds using warble tones, power spectrum from International speech test signal (ISTS) at 55, 65 and 75 dB SPL, as well as noise floor and by-pass transmission of ISTS at 75 dB, both with audio processor switched off. A Fast Fourier Transform power spectrum (Agilent 35670, Keysight Technologies, USA) was used to measure the uncalibrated voltage output from the SM for the frequency range 200–8000 Hz (Δf = 32 Hz) and presented in dB re 0.01 mV.
All sound field measurements were performed in a sound insulated test room (16 m3) with a loudspeaker placed 1 metre in front of, and at the same height as, the patient’s head. The loudspeaker was driven from an AC40 audiometer (Interacoustics AS, Assen, Denmark) via a power amplifier (Rotel RB-976MkII) and calibrated for sound field dB HL at the BCD microphone location. For presentation of the ISTS, a Callisto system (Interacoustics AS, Assen, Denmark) was used with a mini speaker (Edfire) placed 25 cm laterally from the BCD, whereas a reference microphone was placed on the patient’s ear near the BCD microphone to control the output of the speech sound levels. To ensure that no sound was transmitted via the ear canal during the sound field measurements and to reduce the occlusion effect, deeply inserted earplugs (3 M™ PELTOR™ Optime™ III) were placed bilaterally.
Results
Overall results
This new method to measure audibility is aimed to be used clinically in the individual patient, but in this study, the results are presented as averages, first on all patients and then on subgroups of patients.
The overall average results of all 29 patients are shown in , presented as the uncalibrated voltage from the SM in dB re 0.01 mV. The wide band ISTS speech spectrum is covering the whole frequency range (with linear resolution f = 25 Hz), whereas the MPO and the thresholds are only measured at typical audiometric frequencies using standard band limited warble tones. The average patient was to be reasonably well fitted with good audibility for the 75 dB SPL ISTS speech over the whole frequency range, whereas some audibility was lacking for the 55 and 65 dB levels at higher frequencies.
Figure 2. Overall average results (a) as measured directly at the SM output in dB re 0.01 mV, and (b) when normalised by hearing thresholds, where the dynamic range is the area between the MPO and the thresholds. These are averages of individual dB values, which have a large spread among patients.
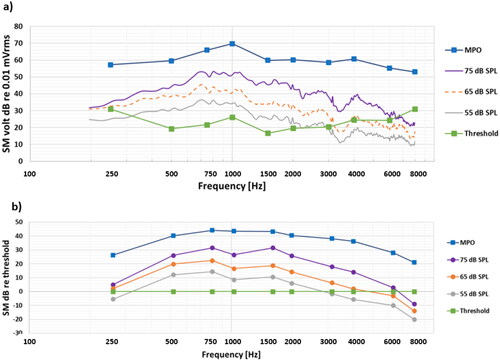
For further analyses it is necessary to transfer the wide band speech signal response to third octave band values around audiometric frequencies to show how the speech levels measured map the dynamic range limited by the MPO and thresholds. For simplicity these values can be normalised by the thresholds (subtracting all responses in dB by the threshold dB values, meaning that thresholds are set to 0 dB) as shown in to better illustrate the audibility.
The lack of audibility at 55 and 65 dB SPL, speech levels at higher frequencies calls for further analysis of the results especially as audibility at these levels are important for the understanding of normal speech. The patients with lack of audibility at these levels belong to a rather heterogenic group regarding type of device used and regarding their degree of sensorineural hearing loss. Therefore, the results for 65 dB SPL speech level were analysed in subgroups regarding type of device. At first, an observation was that the BCI users seems to have similar or better audibility over the frequency range from 500 to 4 kHz than the average of all BAHA users. This may reflect that there is a wider range of sensorineural hearing loss and higher PTA4 in the BAHA users (Best PTA4 average 26.8 dB HL and range 3.8 – 50 dB HL) relative to the BCI users (Best PTA4 average 16.3 dB HL and range 2.5 – 30 dB HL). For a more relevant comparison to the BCI users the BAHA users were further divided into three subgroups; BC-PTA4 ≤30dB; BC-PTA4 >30dB; and SSD patients (wearing the BAHA on the deaf side as a CROS device) and all results are presented in . The average BC-PTA4 (± 1SD) in the BAHA subgroups were 17.8 (8.0), 34.3 (5.2) and 21.9 (3.1) dB HL, respectively, whereas for the BCI users it was 16.3 (9.2) dB HL and for the Skin drive users it was 36.9 (13.1) dB HL. The various BAHA models may play a role depending on their different power capacity, but model-based subgroups are too small for meaningful comparisons.
Using audibility to further optimise a BCD fitting
In a clinical setting, average results and group analysis is not the main objective and patients are instead analysed individually. An initial fitting of a BCD is usually performed using company specific software, where in-situ thresholds are first measured and then used together with a certain algorithm, such as NAL or DSL, to suggest an initial fitting proposal. For verification of the initial fitting proposal, the skin microphone approach can be used to verify that the fitting is acceptable. What “acceptable” means is vague, but from the audibility perspective it means that as much as possible of the speech sound should be audible. Using this method, it means that as much as possible of the speech responses of the ISTS measured at 55-65-75 dB SPL should be within the dynamic range, which is in between the threshold and the MPO measured by the SM in the same spot of the forehead. In this study, most patients were found to be rather well fitted, but one patient was considered slightly misfitted, see red circled areas in . This patient was called back for an adjustment of the overall gain and in the 750-1 kHz and 3.5-4.5 kHz regions. The gain adjustment was objectively verified in a SS measurement and a subsequent remeasurement of the audibility was made where the results are shown in . As expected, the audibility improved in the adjusted frequency regions which was also validated by aided sound field measurements of speech recognition score in noise (63 dB SPL, S/N= +4 dB) which improved by 18% units and SNR threshold at speech level (Hagerman 5-word sentences at 63 dB SPL) improved by 0.95 dB.
Bonebridge system – reimplanted in one patient
To further strengthen the versatility of this skin microphone approach, one of the patients, who has used two different active transcutaneous implant systems (BCI and Bonebridge) at the same anatomical location, were tested with both systems. This patient received the original BCI implant in fall 2013, but was recently (spring 2023) subjected to an internal implant failure after almost 10 years of successful use. Because of a lack of additional physical BCI systems from the initial clinical study, it was decided to reimplant with a Bonebridge, which is commercially available and of similar design.
In a first fitting of the Bonebridge, using the recommended approach in the company programming software, the patient came back after two weeks and had the audibility measured with the SM and for the 65 dB ISTS speech level, as shown by the dash line in . This was deemed quite poor and an increase of the gain below 1 kHz and around 2.5 kHz were made. As can be seen in , the audibility of the Bonebridge improved and became more similar to the original BCI implant system. The improvement in audibility after adjustment of the Bonebridge gain was also validated by an increased speech recognition in noise score of +44% units. Regarding the comparison between the BCI and the Bonebridge system on this patient, the fitting could have been adjusted to be more equal, by changing the gain settings, but that was not the purpose as both devices have been fitted independently and the patient was satisfied in both cases.
Discussion
General
This new method, using a skin microphone approach, has in this study been shown to be useful to determine the audibility of BCDs in general, independent of generic type. This finding is of particular importance for active transcutaneous BCDs, such as BCI-Sentio, Bonebridge and Osia since the transducer is permanently implanted in these systems and its function cannot be tested explicitly together with the audio processor. For BAHA devices or skin drive devices, on the other hand, it is possible to hear that the device is functioning using a bite rod, or in more detail by performing a frequency response measurement using a mechanical coupler such as the SS or an artificial mastoid, but that is not possible with active transcutaneous devices.
For patients with an implanted transducer, the skin microphone approach can also be used as a kind of stethoscope. This can clinically be a very important application if a patient comes back and complains about the sound in their device, it is hard to know where the problem is located, even if you can measure with the SM that the audibility is poor. It could be a malfunction of the audio processor or the implanted parts (not only the transducer is implanted but also an inductive receiver coil, connected to demodulation and electric filter circuits) or a change of the patient’s residual hearing. By using the SM and connecting the output to sound protected headphones, the audiologist can listen to what the patient potentially hears through the skull bone (preferably listening to a radio or a third person talking) and thus get a good overview of what has happened. This was tested on some of the patients, and also on some of the investigators, and found to be a potentially viable application.
Potential error investigation
Possible errors with this method include, but are not limited to, interference of measurement noise and by direct sound by-passing the BCD reaching the SM directly from the speaker. It was noticed in preclinical measurements that direct sound could reach the internal microphone unit in the SM directly and even stronger than the sound via the BCD. Therefore, extra sound insulation with the Peltor earmuff was introduced. Also, the inherent noise floor of the measurement equipment can cause a problem, especially for the speech signal in patients with good cochlear function. This noise could either originate from the measurement system or from the BCD noise, typically from the audio processor microphone noise and/or surrounding noise picked up by the microphone. In the threshold measurement with warble tones, the noise floor limitation is solved by raising the signal at the threshold sufficiently above the noise floor and then reading off the level. The correct threshold is then calculated backward by reducing the read-off level by the same amount as the signal was raised above the threshold when read-off.
To investigate the degree of erroneously competing by-passed sound and the noise floor, three additional measurements were performed in all patients. First, one measurement was made where the BCD was removed (Supplement Figure 1a) when a 75 dB SPL level of ISTS was presented to investigate the by-pass of sound. Then, two noise floor measurements were performed (Supplement Figure 1b), one with the BCD in place and turned on (total noise floor) and one with the BCD removed (only noise floor from the SM measurement system).
As seen in Supplement Figure 1c, the 75 dB SPL speech response from the SM decreased by 33 dB at 1 kHz (average 27 dB at PTA4 frequencies) when the device was removed, which indicates that on the average there is a good margin to erroneous by-pass sound influence. If the speech signal would be 10 dB lower (65 dB SPL) this attenuation would be kept unchanged as both the responses, with and without a BCD in place, will drop 10 dB.
Regarding the noise floor, the SM contribution is generally less than the contribution from the BCD itself including the ambient room noise floor, see black curve which is equal or below the yellow curve in Supplement Figure 1c. The signal to noise ratio is 42 dB at 1 kHz (average 27 dB at PTA4 frequencies) which gives a safe signal to noise ratio also for the 65 dB SPL speech level (and up to 6 kHz for the 55 dB SPL speech level).
Both the by-pass attenuation and the noise floor results shown in Supplement Figure 1c are based on the average of all patients, but for the individual patient, these values can vary. The signal to noise ratio between SM noise floor and SM speech level responses in the individual patients will be dependent on e.g. the patients sensorineural hearing loss – with a better cochlear function the speech levels from the BCD will be lower and closer to the noise floor. In such patients, the by-pass sound response is closer to becoming an issue as the speech responses via the BCD will be closer to the erroneous by-pass sound level which is fixed for the given speech level.
A clinical version under development
The present measurement system comprising different equipment for sound generation and signal processing of the skin microphone output, as well as the skin microphone system itself, can be adapted to a more user-friendly system.
Together with Audioscan (Canada), a skin microphone system is under development where the microphone unit has an integrated sound protection casing. The design aim is to develop a unit which is easy and comfortable to attachment and fits to any head size and also to have a unit which can be used as a plug-and-play accessory to the already existing sound test systems, as e.g. the Audioscan Verifit system.
Conclusions
In this study, it has been shown that a skin microphone system can be a valuable tool for verifying that the BCD fitting gives the patient adequate use of their device in terms of audibility.
It was also shown that audibility can be measured independently of type of BCD used, explicitly being percutaneous as well as active or passive transcutaneous.
Further it was shown that the proposed skin microphone system response could be used in individual patients to improve their initial fitting by BCD gain adjustments, to increase the audibility.
This method requires a minimum of additional equipment to a standard audio test stations frequently used in clinics today and do not need specific calibration of BCD output relating to normal force thresholds or Real Ear to Coupler Differences.
Practically, the skin microphone approach could also be used as a bone conduction stethoscope where the investigator could listen to the patient’s BCD in-situ.
More studies are needed to fully evaluate the potential use of the skin microphone approach especially after a final commercial version is available.
Supplemental Material
Download MS Word (202.3 KB)Supplemental Material
Download MS Word (17.2 KB)Disclosure statement
No potential conflict of interest was reported by the author(s).
Additional information
Funding
References
- Eeg-Olofsson, M., B. Håkansson, S. Reinfeldt, H. Taghavi, H. Lund, K.-J F. Jansson, E. Håkansson, and J. Stalfors. 2014. “The bone conduction implant–first implantation, surgical and audiologic aspects.” Otology & Neurotology: official Publication of the American Otological Society, American Neurotology Society [and] European Academy of Otology and Neurotology 35 (4):679–685. https://doi.org/10.1097/MAO.0000000000000203.
- Hodgetts, W. E., B. E. Håkansson, P. Hagler, and S. Soli. 2010. “A comparison of three approaches to verifying aided Baha output.” International Journal of Audiology 49 (4):286–295. https://doi.org/10.3109/14992020903142676.
- Hodgetts, W., D. Scott, P. Maas, and L. Westover. 2018. “Development of a novel bone conduction verification tool using a surface microphone: Validation with percutaneous bone conduction users.” Ear and Hearing 39 (6):1157–1164. https://doi.org/10.1097/AUD.0000000000000572.
- Håkansson, B., and P. Carlsson. 1989. “Skull simulator for direct bone conduction hearing devices.” Scandinavian Audiology 18 (2):91–98. https://doi.org/10.3109/01050398909070728.
- Håkansson, B., P. Carlsson, A. Brandt, and S. Stenfelt. 1996. “Linearity of sound transmission through the human skull in vivo.” The Journal of the Acoustical Society of America 99 (4 Pt 1):2239–2243. https://doi.org/10.1121/1.415411.
- Håkansson, B., P. Carlsson, and A. Tjellström. 1986. “The mechanical point impedance of the human head, with and without skin penetration.” The Journal of the Acoustical Society of America 80 (4):1065–1075. https://doi.org/10.1121/1.393848.
- Maier, H., T. Lenarz, P. Agha-Mir-Salim, M. J. H. Agterberg, A. Anagiotos, S. Arndt, G. Ball, M. Bance, M. Barbara, U. Baumann, et al. 2022. “Consensus statement on bone conduction devices and active middle ear implants in conductive and mixed hearing loss.” Otology & Neurotology 43 (5):513–529. Stefan, Christof Stieger https://doi.org/10.1097/MAO.0000000000003491.
- Mertens, G., J. Desmet, A. F. Snik, and P. Van de Heyning. 2014. “An experimental objective method to determine maximum output and dynamic range of an active bone conduction implant: the Bonebridge.” Otology & Neurotology: official Publication of the American Otological Society, American Neurotology Society [and] European Academy of Otology and Neurotology 35 (7):1126–1130. https://doi.org/10.1097/mao.0000000000000347.
- Nie, Y., J. Sang, C. Zheng, J. Xu, F. Zhang, and X. Li. 2022. “An objective bone conduction verification tool using a piezoelectric thin-film force transducer.” Frontiers in Neuroscience 16:1068682. https://doi.org/10.3389/fnins.2022.1068682.
- Persson, A.-C., B. Håkansson, M. Caveramma Mechanda, W. Bill Hodgetts, K.-J. Fredén Jansson, M. Eeg-Olofsson, and S. Reinfeldt. 2022. “A novel method for objective in-situ measurement of audibility in bone conduction hearing devices - a pilot study using a skin drive BCD.” International Journal of Audiology 62 (4):357–361. https://www.ncbi.nlm.nih.gov/pubmed/35238713.
- Reinfeldt, S., B. Håkansson, H. Taghavi, K.-J. Fredén Jansson, and M. Eeg-Olofsson. 2015. “The bone conduction implant: Clinical results of the first six patients.” International Journal of Audiology 54 (6):408–416. https://doi.org/10.3109/14992027.2014.996826.
- Reinfeldt, S., C. Rigato, B. Håkansson, K.-J. Fredén Jansson, and M. Eeg-Olofsson. 2019. “Karl Johan Fredén Jansson, and Måns Eeg-Olofsson. 2019. "Nasal sound pressure as objective verification of implant in active transcutaneous bone conduction devices.” Medical Devices (Auckland, N.Z.)12:193–202. 12: 193-202. https://doi.org/10.2147/MDER.S197919.
- Snik, A., H. Maier, B. Hodgetts, M. Kompis, G. Mertens, P. van de Heyning, T. Lenarz, and A. Bosman. 2019. “Efficacy of Auditory Implants for Patients With Conductive and Mixed Hearing Loss Depends on Implant Center.” Otology & Neurotology: official Publication of the American Otological Society, American Neurotology Society [and] European Academy of Otology and Neurotology 40 (4):430–435. https://doi.org/10.1097/MAO.0000000000002183.
- van Barneveld, D. C. P. B. M., H. J. W. Kok, J. F. P. Noten, A. J. Bosman, and A. F. M. Snik. 2018. “Determining fitting ranges of various bone conduction hearing aids.” Clinical Otolaryngology 43 (1):68–75. https://doi.org/10.1111/coa.12901.