ABSTRACT
The effect of concentration, molar ratios of reagents, pH, and temperature on formation of chloro-organic products in reaction of tert-butyl ethers with chloride ions and hydrogen peroxide has been determined. A significant effect of Cl− ions and H2O2 molar ratios on the rate of chloro-organic product formation has been observed. Studies on oxychlorination of tert-butylethyl ether (ETBE) at pH 7, 3.5, and 2.5 have been carried out. It was found that introduction of hydronium ions into the reaction system considerably hastened the process of chloro-organic product formation. Hydronium ions contribute to the formation of the reactive tert-butyl carbocation, which undergoes secondary reactions in the presence of reactive forms of chlorine and oxygen. Moreover, the effect of temperature on ETBE (tert-butylethyl ether) and MTBE (tert-butylmethyl ether) conversions was verified. The reactions of MTBE and ETBE oxychlorination were carried out at temperatures of 5°C, 20°C, and 35°C.
INTRODUCTION
Tert-butyl ethers are added to fuels as the oxygen components since they increase the octane number of petrol. They contribute to the improvement of air quality by reducing the emission of carbon oxide and precursors of free radicals in the exhaust gases. On the other hand, out-of-control leakages of fuels containing tert-butyl ethers are the source of their presence in the environment. Ethers are identified in ground, surface, storm, and waste waters as well as in air (Squillace et al. Citation1996; EPA Citation1998; Halden et al. Citation2001). Squillace et al. (Citation1996) showed that MTBE is the second, after chloroform, volatile organic compound most often identified in ground waters. MTBE concentration in rain water as well as in ground water is significantly conditioned by the season of the year. It was shown that concentration of ethers in the water environment was higher in winter than in summer time (Delzer et al. Citation1996; Achten et al. Citation2001; Baehr et al. Citation2001). The available data suggest that the volatile organic compounds are characterized by higher stability at low temperatures (below 10°C) than at higher ones. Temperature rise contributes to their quick vaporization and increase of their chemical activity in the gaseous phase. An increase in the amounts of the volatile organic compounds in air enhances the probability of their contact with radical forms and oxidative agents. Moreover, ethers being cumulated in air during the rainy season are washed out and they migrate together with rain waters to ground waters. This fact is of particular importance in urban areas because of the frequent traffic jams and numerous petrol stations that are potential sources of ether emission into the environment. On the other hand, chloride ions, oxidants, and pH-reducing agents present in waters can result in conversion of ethers to compounds that are much more aggressive for the environment.
Table 1 Composition of the solutions studied
Our studies showed that tert-butyl ethers in the presence of oxidative agents and chloride ions can be a source of toxic chloro-organic compounds (Gaca et al. Citation2004;
Gackowska and Gaca Citation2004; Cysewski et al. Citation2006). As a result of reaction of MTBE/or ETBE with HCl/H2O2 and metal chloride/oxidant/H+ system, we obtained 2-chloro-2-methylpropane, 3-chloro-2-methylpropene, 1,2-dichloro-2-methylopropane, 3-chloro-2-chloromethylpropene, and chloroacetone besides products of oxidation such as tert-butyl alcohol, acetone, 2-methylpropene, and tert-butyl hydroperoxide. The mechanism of formation of the identified dichlorocompounds was described by Cysewski et al. (Citation2006).
In order to check which factors have an effect on the rate of chloro-organic compound formation, studies on the effect of concentration, molar ratios of reagents, pH, and temperature were undertaken. On the basis of investigations carried out by O'Reilly et al. (Citation2001), Achten et al. (Citation2002), Arp and Schmidt (Citation2004), and Fischer et al. (Citation2004), it can be assumed that pH and temperature will play a significant role in the process of chloro-organic compound formation since the above-mentioned parameters have an effect on the stability of tert-butyl ethers. We can assume that different behaviors of ethers at various ambient temperatures and at various pH values will affect the formation of ether conversion products.
EXPERIMENTAL
MTBE 98%, 1,2-dichloro-2-methylpropane, 3-chloro-2-chloromethylpropene (Sigma-Aldrich Germany), ETBE (Polish Petrol Concern Orlen” S.A. Płock Poland), tert-butyl alcohol, hydrochloric acid 36%, nitric acid (V) 65%, hydrogen peroxide 30%, and sodium chloride (Polish Chemical Reagents) were used in our studies. Samples for experiments were prepared by liquid-liquid extraction technique. Carbon disulfide (Merck) was applied as a solvent. Samples were analyzed by gas chromatograph HP 5890-Hewlett Packard equipped with flame ionization detector FID. Operating parameters were as follows: column HP-1 (0.53 mm × 60 m × 0.2 μm) temperature of injector 250°C, temperature of detector 250°C, temperature program: 40°C/4 min-10°C/min-200°C.
Precision was evaluated by injection of samples (repeated six times) obtained after extraction of ETBE, 1,2-dichloro-2-methylpropen, and 3-chloro-2-chloromethylpropene solutions at concentrations of 1 mL/L, 10 μL/L, and 10 μL/L, respectively. The values of relative standard deviations were determined as follows: ETBE, 3.37%; 1,2-dichloro-2-methylpropen, 2.84%; and 3-chloro-2-chloromethylpropene, 4.41%.
In the next stage, a linearity of calibration curves for ETBE 1,2-dichloro-2-methylpropan and 3-chloro-2-chloromethylpropene was determined. For that purpose, water solutions of ETBE (10, 5, 1, 0.5, 0.2, and 0.05 mL/L) and 1,2-dichloro-2-methylpropen and 3-chloro-2-chloromethylpropene (20, 10, 5, 1, and 0.5 μL/L) were prepared. Then, liquid-liquid extraction was performed by the use of carbon dioxide and calibration curves were determined. The linear correlation coefficients (R2) were as follows: ETBE, 0.987; 1,2-dichloro-2-methylpropan, 0.994; and 3-chloro-2-chloromethylpropene, 0.996.
ETBE solution at a concentration of 0.07 mol/L with the addition of 0.7 mol of NaCl and 0.35 mol of H2O2 was applied in our investigations. In order to determine the effect of pH on the process of chloro-organic product formation, the samples with pH values of 7, 3.5, and 2.5 were prepared. Solutions at pH values of 2.5 and 3.5 were obtained by addition of suitable amounts of nitric acid (V). pH of solution was measured by pH-meter Elmetron CX-741.
Studies on the effect of temperature were carried out in the presence of ETBE and MTBE as well as tert-butyl alcohol (TBA), which was the product of oxidation of ethers. Composition of the solution studied is presented in .
RESULTS AND DISCUSSION
The degradation rate of ether and formation of 1,2-dichloro-2-methylpropane, 3-chloro-2-chloromethylpropene, and TBA depend on molar rations of reagents, concentration of hydronium ions, and temperature. Molar ratios of reagents are of the fundamental importance. By applying the excess of hydrogen peroxide and hydrochloric acid in relation to ETBE, the process of chlorocompound formation was hastened (Gaca et al. Citation2004). It can be explained by the specific character of the H2O2/Cl−/H+ system. According to the literature data, the H2O2/Cl−/H+ system is of the complex character and it is described by a series of secondary reactions. They are both ionic (Livingstone and Bray Citation1925; de la Mare et al. Citation1954) and radical (Davies and Kustin Citation1973) reactions. In both cases, the reactive forms of chlorine (RFCh) are formed. The ions Cl−, ClO− ClO2−, and Cl+ are formed by ionic path, whereas Cl· radical is formed by radical reactions. The formed RFCh can further react with chloro-organic compounds present in solution with formation of chloro-organic products. It results from the above statement that neither the ionic nor the radical path in the process of chloro-organic product formation by reaction of tert-butyl ethers with H2O2/Cl−/H+ system can be excluded.
It was observed that H+ ions play an important role in the process of toxic chloro-organic compound formation. In neutral medium, ether was relatively stable. No chloro-organic derivatives were identified in the products. Insignificant ETBE loss in reactions proceeding with participation of hydrogen peroxide and sodium chloride at pH 7 proves to slow the process of ether oxidation ().
FIGURE 1 Change of ETBE concentration in time depending on pH, in reaction of ETBE with NaCl/H2O2/HNO3.
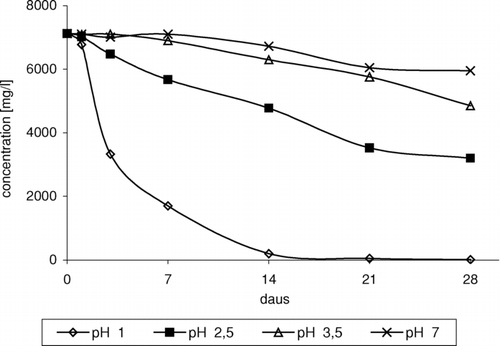
Acidification of the medium resulted in series of reactions leading to formation of chloro-organic compounds as well as the products of ether oxidation and degradation (Gaca et al. Citation2004). In the case of 1,2-dichloro-2-methylpropane, it was observed that in solution at pH 3.5, small amounts of product were formed after a long time. Reduction of the pH value resulted in shortening of time of product formation. In solution at pH 1, product was formed relatively quickly and in higher amounts ().
FIGURE 2 Change of 1,2-dichloro-2-methylopropane concentration in time depending on pH, in reaction of ETBE with NaCl/H2O2/HNO3.
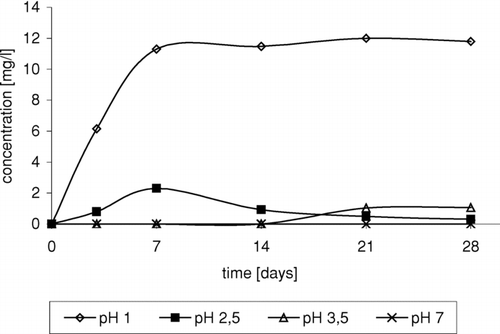
Strongly acid medium is required in the process of 3-chloro-2-chloromethylpropene formation (). The presence of 3-chloro-2-chloromethylpropene was observed only in solution at pH 1.
FIGURE 3 Change of 3-chloro-2-chloromethylopropene concentration in time depending on pH, in reaction of ETBE with NaCl/H2O2/HNO3.
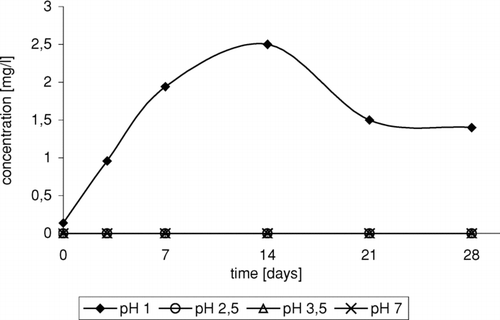
Formation of tert-butyl ether conversion products can be explained by the fact that in the first stage of reaction, dealkoxylation and carbocation formation occur under the influence of H+ ions present in the reaction mixture ().
The formed carbocation can further react and in the first stage, tert-butyl alcohol, 2-chloro-2-methylpropane, and 2-methylpropene are formed. Then, as a result of the primary product conversions, 1,2-dichloro-2-methylpropane and 3-chloro-2-chloromethylpropene are formed (Cysewski et al. Citation2006).
The successive parameter that has an effect on oxychlorination of tert-butyl ethers is temperature. Studies showed that at a temperature of 5°C, the increase in 1,2-dichloro-2-methylpropane was insignificant and it proceeded very slowly both in reaction of ETBE with NaCl/H2O2/HNO3 and of ETBE with HCl/H2O2 (, series 1 and 4). Temperature rise by 15° contributed to the increase in chlorocompound yield and the rate of its formation (, series 2 and 5).
In reaction with participation of MTBE, the effect of temperature on 1,2-dichloro-2-methylpropane formation is similar. However, it is formed more slowly and with the lower yield (, series 7 and 9).
The higher concentration of chloro-organic product was obtained in the case when the temperature of the reaction system was 35°C. After 3 days, in reactions of ETBE with NaCl/H2O2/HNO3 and with HCl/H2O2, concentration of 1,2-dichloro-2-methylpropane was 27 mg/L and 13 mg/L (, series 3 and 6); however, in reactions with MTBE, it was 17 mg/L and 15 mg/L, respectively (, series 8 and 10).
FIGURE 4 Change of 1,2-dichloro-2-methylpropane concentration in time, depending on temperature, in following reactions: ETBE/NaCl/H2O2/HNO3: 1, (5°C); 2, (20°C); 3, (35°C), ETBE/HCl/H2O2: 4, (5°C); 5, (20°C); 6, (35°C), MTBE/NaCl/H2O2/HNO3: 7, (20°C); 8, (35°C), MTBE/HCl/H2O2: 9, (20°C); 10, (35°C).
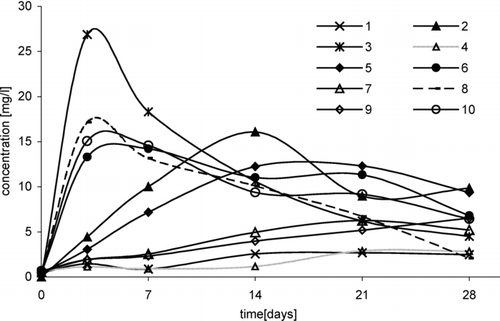
FIGURE 5 Change of 3-chloro-2-chloromethylpropene concentration in time, depending on temperature, in the following reactions: ETBE/NaCl/H2O2/HNO3: 1, (5°C); 2, (20°C); 3, (35°C), ETBE/HCl/H2O2: 4, (5°C); 5, (20°C); 6, (35°C), MTBE/NaCl/H2O2/HNO3: 7, (20°C); 8, (35°C), MTBE/HCl/H2O2: 9, (20°C); 10, (35°C).
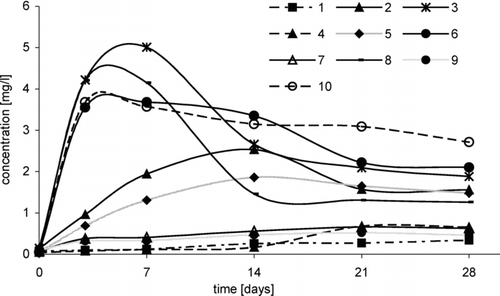
FIGURE 6 Change of dichlorocompound concentrations in time, depending on temperature, in reaction of TBA with HCl/H2O2: 1,2-dichloro-2-methylpropane: 1, (20°C); 2, (35°C), 3-chloro-2-chloromethylpropene: 3, (20°C); 4, (35°C)
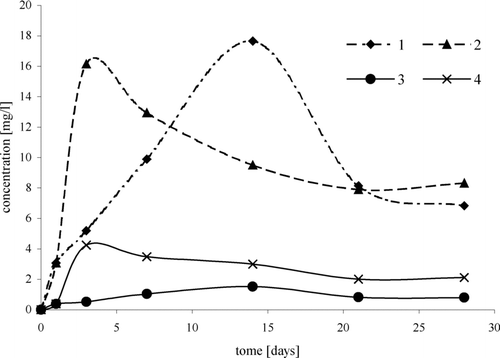
In reactions with participation of sodium chloride (, series 3 and 8), concentration of 1,2-dichloro-2-methylpropane was higher than that in reactions with HCl (, series 6 and 10).
In reactions of both ethers (ETBE and MTBE) with hydrochloric acid and hydrogen peroxide at a temperature of 35°C, processes of chloroproduct formation and decomposition proceeded similarly.
Similar effect of temperature was observed during formation the second chloroproduct (i.e., 3-chloro-2-chloromethylpropene). At a temperature of 5°C, 3-chloro-2-chloromethylpropene was formed very slowly and in small quantities (, series 1 and 4). At a temperature of 35°C, 3-chloro-2-chloromethylpropene was formed in the highest concentration (about 5 mg/L) after 7 days of reaction between ETBE and NaCl/H2O2/HNO3 (, series 3). However, in reaction of ETBE with HCl/H2O2, the yield of dichlorocompound was lower and at a temperature of 35°C, its concentration was about 4 mg/L (, series 6). Similar results were obtained in reaction with participation of MTBE (, series 8 and 10). However, at a temperature of 20°C, 3-chloro-2-chloromethylpropene was formed in higher quantities in reaction with ETBE than in reaction with MTBE (, series 2 and 7, 5 and 9).
Moreover, reactions of TBA with the H2O2/HCl system at temperatures of 20 and 35°C were carried out. At higher temperature, the reaction runs at higher rate ().
The results described above are consistent with those presented by Huang et al. (Citation2002), who showed that in the process of MTBE oxidation in the presence of persulfates (without chloride ions) at temperatures within the range of 20°C to 50°C, the highest amounts of ether were converted at a temperature of 50°C. Studies on the effect of temperature showed also that ETBE in the presence of Cl−, H+ ions, and hydrogen peroxide was more reactive than MTBE. The similar effect of reactivity of ethers on their conversions was observed by Norris and Rigby (Citation1932), who showed that tert-butyl chloride was formed more rapidly in reaction of 27% HCl with ETBE than with MTBE.
CONCLUSION
The stables in neutral medium ethers can migrate to considerable distances and thus, they contribute to contamination of drinking waters. In the case when they come into contact with acid sewage, they can contribute to formation of new, more reactive products, which, in turn, in the presence of reactive forms of chlorine, are a potential source of toxic chloro-organic products. On the basis of results obtained, it can be assumed that intensity of chloro-organic compound formation is higher in summer than in the winter season. Therefore, introduction of tert-butyl ethers into the environment has the repercussions in formation (as a result of the secondary reactions) of the successive portion of chloro-organic compounds that are relatively stable and can cumulate in various organisms.
REFERENCES
- Achten C., Kolb A., Püttmann W. Methyl tert-butyl eter (MTBE) in urban and rural precipitation in Germany. Atmos. Environ. 2001; 35: 6337–6345
- Achten C., Kolb A., Püttman W. Methyl tert-butyl ether (MTBE) in river and wastewater in Germany. Environ. Sci. Technol. 2002; 36: 3652–3661
- Arp H. P. H., Schmidt T. C. Air-water transfer of MTBE, its degradation products and alternative fuel oxygenates: the role of temperature. Environ. Sci. Technol. 2004; 38: 5405–5412
- Baehr A. L., Charles E. G., Baker R. J. Methyl tert-butyl ether degradation in the unsaturated zone and the relation between MTBE in the atmosphere and shallow groundwater. Wat. Resours. Res. 2001; 37(2)223
- Cysewski P., Gackowska A., Gaca J. Experimental and theoretical studies on formation and degradation of chloro organic compounds. Chemosphere 2006; 63: 165–170
- Davies G., Kustin K. Hydrogen peroxide-chlorine reaction and its catalysis by manganese(III)-manganese(II). Inorg. Chem. 1973; 12: 961–962
- de la Mare P. B. P., Ketley A. D., Vernon C. A. The kinetics and mechanism of aromatic halogen substitution. Part. Acid-catalysis chlorination by aqueous solution of hypochlorous acid”. J. Chem. Soc 1954; 1290–1297
- Delzer G. C., Zamorski J. S., Lopes T. J., Bosshat R. L. Occurrences of the gasoline oxygenate MTBE, BTEX compounds in urban stormwater in the United State. , et al, 1996, Water-Resources Investigations Report 96-4145, US Geological Survey
- EPA 510-F-97-015. U.S. Environmental Protection Agency 1998 MTBE Fact Sheet #2 Remediation of MTBE Contaminated Soil and Groundwater. , et al, 1998, www.epa.gov/OUST.mtbe/
- Fischer A., Müller M., Klasmeier J. Determination of Henry's law constant for methyl tert-butyl (MTBE) at groundwater temperatures. Chemosphere 2004; 54: 689–694
- Gaca J., Gackowska A., Noetzelmann S. Effect of UV radiation on ETBE ether chlorination in the presence of oxidizing agent. Proceedings of PTChem Wrocław Poland (in Polish). 2004; 363
- Gackowska A., Gaca J. Chloroorganic products of tert-butyl ether degradation in the presence of chloride ions. Polish J. Environ. Studies Suppl. 2004; 13: 68–72
- Halden R. U., Happel A. M., Schoen S. R. Evaluation of standard methods for the analysis of methyl tert-butyl ether and related oxygenates in gasoline-contaminated groundwater. Environ. Sci. Technol. 2001; 35: 1469–1474
- Huang K.-C., Couttenye R. A., Hoag G. E. Kinetics of heat-assisted persulfate oxidation of methyl tert-butyl ether (MTBE). Chemosphere 2002; 49: 413–420
- Livingstone R. S., Bray W. C. The catalytic decomposition of hydrogen peroxide in an acid chlorine-chloride solution. J. Am. Chem. Soc. 1925; 47: 2069–2083
- Norris J. F., Rigby G. W. The reactivity of atoms and groups in organic compounds. XII. The preparation and properties of mixed aliphatic ethers with special reference to those containing the tert.-butyl radical. J. Am. Chem. Soc. 1932; 54: 2088–2100
- O'Reilly K. T., Moir M. E., Taylor Ch. D., Smith Ch. A., Hyman M. R. Hydrolysis of tert-butyl methyl ether (MTBE) in dilute aqueoua acid. Environ. Sci. Technol. 2001; 35: 3954–3961
- Squillace P. J., Zagorski J. S., Wilber W. G., Price C. V. Preliminary assessment of the occurrence and possible sources of MTBE in groundwater in the United States, 1993–1994. Environ. Sci. Technol. 1996; 30: 1721–1730