ABSTRACT
Reversed-phase high-performance liquid chromatography, coupled with coulometric electrochemical detection, was successfully applied for the quantification of added folic acid (FA) in fortified fruit juices and cereal products. The method allowed good separation of the 5-HCO-H4 folate and folic acid in cereal samples. The retention times of vitamins were repeatedly determined by isocratic elution using 40 mM sodium phosphate dibasic, heptahydrate buffer, and 8% acetonitrile (v/v) (0.9 mL/min, pH 5.5) as mobile phase with the Supelco LC 18 column 5 μm (25 cm × 4.6 mm). Folate concentrations were measured using a trienzyme (hog kidney folate conjugase, α-amylase, and protease) folate extraction method.
Folate is a generic term referring to the mono- to polyglutamate derivatives of pteroic acid that occur naturally in many foods, and have a closely related biological activity. During the last decade, the importance of adequate folate intake has become well recognized in the reduction of the rate of neural tube defects. These vitamins cofactors are essential for the synthesis of purines and pyrimidines and in the production of methionine from homocysteine (Ball Citation2006; Bailey Citation2005; Cravo and Camilo Citation2000; Das Citation2003; Finglas et al. Citation2006; Malinow et al. Citation1999). The most important dietary sources are fortified foods, liver, green vegetables, and cereal products (Ball Citation2006). Naturally occurring folates in reduced form are heat labile and readily destroyed by oxidation. Fortification of food with vitamins is intended to compensate for the loss of these compounds due to the heat treatment to which they are subjected during manufacture (Akhtar et al. Citation1999). The predominant natural forms of folate in animal products are 5-methyltetrahydrofolate (5-CH3–H4 folate) and tetrahydrofolate (H4 folate); in fruits and vegetables 5-CH3–H4 folate; and in cereal products 5-formyltetrahydrofolate (5-HCO-H4 folate) and 10-formyl-folic acid, and they have been detected in reasonable amounts, together with 5-CH3–H4 folate and folic acid (Ball Citation2006).
The determination of B vitamins in various samples is rather difficult due to the chemical instability and complexity of the matrices in which they usually exist. Vitamins are most often determined in the free form, which involves hydrolysis of the phosphorylated forms and/or those bound to proteins (and optionally glycosylated) during the extraction step performed prior to the chromatographic separation. The extraction procedure was considered to have the greatest impact on analytical results. During the last decade, the use of a trienzyme treatment method has been developed for more efficient extraction of folates from food than the conventional methods. This method includes the use of protease and amylase in combination with traditional folate conjugase treatment following heat extraction (De Souza and Eitenmiller Citation1990; Hyun and Tamura Citation2005; Johnston et al. Citation2002; Tamura Citation1998; Yon and Hyun Citation2003). The methods for vitamin determination require the individual approach to analyze each vitamin by different physical, chemical, and microbiological analytical procedures (Eitenmiller and Landen Citation1999). Usually the folate content of food is quantified by microbiological assays, carried out as Lactobacillus casei–based turbidimetric assay or titrimetric methods (AOAC International Citation2003). To the most popular chromatographic techniques belong high-performance liquid chromatography (HPLC). The modifications of the HPLC coupled to ultraviolet-visible absorbance (Heudi et al. Citation2005), fluorometric (Gregory et al. Citation1984: Gujska and Kuncewicz Citation2005; Ruggeri et al. Citation1999), and electrochemical detections have been presented in the literature. The gradient elution is usually applied for the separation of individual folate determination (Eitenmiller and Landen Citation1999). Their determination by ion-pairing chromatography with reversed phase is the most frequent method (Breithaupt Citation2001; CitationEitenmiller and Landen 1990; Pfeiffer et al. 1997). Alternative methods are based on redox reaction with electrochemical detectors such as coulochemic instruments (Bagley and Selhub Citation2000; Flangan et al. Citation2005). The B vitamins are easily oxidized or reduced with online working electrode in electrochemical cell. The high sensitivity and lower limits of detection are obtained using the electrochemical detection allowing the analysis of folate from distribution in red blood cells and lymphocytes (Bagley and Selhub Citation2000).
This work presents the application of several procedures including a trienzyme extraction and reversed-phase HPLC with coulometric electrochemical detection for the quantification of folic acid and 5-HCO-H4 folate in fortified food products.
MATERIALS AND METHODS
All solutions were prepared with analytical reagent grade compounds. Sodium phosphate dibasic heptahydrate was obtained from Sigma-Aldrich (St. Louis, MO, USA). Other reagents were also of HPLC grade—that is, orthophosphoric acid (Riedel de Häen, Seelze, DA), and solvents: acetonitrile and water (Baker, HPLC Analyzed). Water was purified using the Milli-Q system from Millipore (Eschborn, Germany). Enzymes, α-amylase, protease, and kidney acetone powder, porcine, type II were provided by Sigma Chemical (Sigma Aldrich). The hog kidney conjugase was prepared according to Gregory at al. (1984) and Pfeiffer et al. (Citation1997). The folate standards (folic acid and 5-formyltetrahydrofolate [calcium salt]) were obtained from Sigma Aldrich. The certified reference material wholemeal flour (CRM 121) was obtained from the Community Bureau of Reference, BCR (Belgium). The reference material was subsampled (5 g) and stored frozen.
Fruit juices, fruit drinks (vitamin fortified), and cereal products were purchased from local supermarkets. All samples were commercially available. The various solid products were finely ground, subsampled (50–100 g), and stored at –18°C until analysis.
The various samples of fruit juices (20 mL) were finely mixed with additional 20 mL phosphoric buffer (pH 6.8); cereal products (2 g) were homogenized by stirring in 20 mL of phosphoric buffer (pH 6.8) and left for 10 min in a water bath at 100°C. After cooling the pH was adjusted to 4.9. The enzymatic digestion prior to separation and quantification step made it possible to release the vitamins bound to proteins or sugars. The extraction procedure was based on a study of Gujska and Kuncewicz (Citation2005). The homogenate was subjected to trienzyme treatment: 3 mL hog kidney folate conjugase and 1 mL α−amylase (4 h at 37°C) followed by 2 mL protease (1 h at 37°C). After enzyme incubation, samples were heated to 100°C for 5 min to inactivate the enzyme, then cooled in ice and centrifuged. The residue was resuspended in extraction buffer and centrifuged again, and the combined supernatants were filled to an exact volume (50 mL), filtered through a Whatman 1 Chr filter paper, then flushed with nitrogen and stored at –30°C. Samples were prepared under dim light and then kept in the dark. Prior to HPLC analysis all samples were filtered through a 0.22-μm pore-size filter. Samples should always be protected from light and working under subdued lighting conditions. The working calibration solution was prepared daily and further diluted with mobile phase.
The HPLC system was coupled with pump P 580 (Dionex) and Coulochem II electrochemical detector (ESA, Inc.) operated by the Chromeleon Chromatography Management System.
HPLC ANALYSIS
The samples were separated isocratically on the reversed-phase LC 18 column 5 μm (25 cm × 4.6 mm), produced by Supelco, Inc. The mobile phase, consisting of 40 mM sodium phosphate dibasic, heptahydrate, and 8% acetonitrile (v/v), was adjusted to pH 5.5 with 85% phosphoric acid. The mobile phase was filtered through a 0.22-μm pore membrane filter and degassed before use. The column was equilibrated at 25°C at a flow rate of 0.9 mL/min. The volume of injection was 20 μL. The column eluate was monitored with electrochemical detector Coulochem II (model 5020A, ESA) equipped with dual analytical cell (model 5010) and guard cell (model 5020). The guard cell was connected in-line before injection port and was used to eliminate the interference with baseline stability. The detector response was set to give a full-scale detection for 1 μA and 50 μA current output received from the analytical cell. The HPLC was operated in a constant flow mode and the flow rate was kept at 0.9 mL/min. Peak area of the electrochemical signal at the porous graphite electrode was used for the analysis of folate form distribution. In the EC detection both sensitivity and selectivity were adjusted by varying the potential maintained between the working and reference electrodes; higher potentials induce a response from more compounds and therefore compromise selectivity. Potential is related to the free energy of the overall reaction. The current of the measurement cell is correlated with the working electrode potential. The relation between potential and resulting current is plotted in a current voltage diagram (Flangan et al. Citation2005). Optimum working potential can be ascertained by repeated injections of a solution of the analyte at differing detector voltages. For obtaining optimum detection, the electrode potentials for the guard cell, electrode E1, and electrode E2 were set at 0.85 V, 0.20 V, and 0.80 V, respectively. A high enough potential at the electrodes has to be applied in order to achieve high sensitivity and baseline stability.
Electroactive compounds, such as folate, begin to oxidize at a specific electrode, depending on their individual oxidation potential. Because coulometric electrodes operate at close to 100% efficiency, compounds that oxidize at lower potential electrodes are completely oxidized; therefore, the oxidized compounds are not detected at higher potentials (Flangan et al. Citation2005). The electroactivity of compounds monitored with a coulometric detector is dependent on the presence of functional group of molecules. The current peak at the positive potential is directly related to the concentration of the analyzed vitamins showing that the response should be caused by the electroactivity of vitamins.
RESULTS AND DISCUSSION
The calibration concentration range was established through consideration of the interval of values attributable to natural concentrations specific for each of the food samples studied. Such approach was necessary to give accurate, precise, and linear results. The calibration graphs were constructed by plotting the peak area based on results corresponding to triplicate injections of vitamins standards. The linearity of the calibration graphs was highly acceptable as proved based on a high correlation coefficient (r ≥ 0.998). The concentration ranges for food samples were as follows: 50 to 2500 and 50–5000 μg/mL for folic acid and 5-HCO-H4 folate, respectively. The limit of detection (LOD) of the method was used, calculated as three standard deviations of the background noise of the standard finally diluted in the same buffer as the food sample. The LOD for standard solution of folic acid amounted to 1.3 ng/mL. Subsequently, the LOD established for standards of 5-HCO-H4 folate was 4.5 ng/mL.
and illustrate chromatograms of the vitamins studied the analyzed food samples. The calibration concentration range was established through consideration of the interval of values attributable to natural concentrations specific for each of the food samples studied. The retention time (10 replicates) for folic acid was tR 15.0 ± 0.3 min, while its value for 5-HCO-H4 folate was tR 7.0 ± 0.2 min. A certified reference material as wholemeal flour (CRM 121) was analyzed to check the accuracy of the analysis. The analytical results obtained for the analyzed certified reference material (as folic acid) were satisfying—that is, the recoveries (as a measure of accuracy) for the analytes studied ranged between 98.0% and 103.6%.
FIGURE 1 Chromatogram of the main folate forms present in wheat flour type 550 1—5-HCO-H4 folate (tR 7.0 min, 0.85 μg/mL), 2—folic acid (tR 15.0 min, 1.13 μg/mL). EC detector sensitivity 1 μA. LC 18 column 5 μm (4.6 mm × 25 cm) and a mobile phase, consisting of 40 mM sodium phosphate dibasic, heptahydrate buffer, and 8% acetonitrile (v/v), pH 5.5. The sample size and the final dilution used for the analyzed vitamin determination varied according to the vitamin content in products.
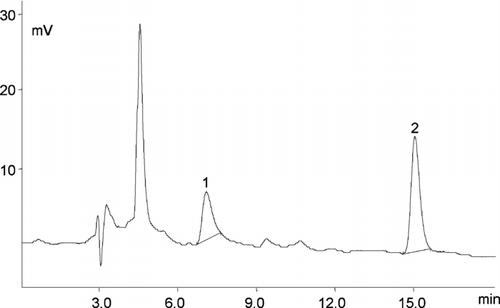
FIGURE 2 Chromatogram of the folic acid in fortified fruit juices—2 folic acid (tR 15.0 min, 3.2 μg/mL). EC detector sensitivity 1 μA. LC 18 column 5 μm (4.6 mm × 25 cm) and a mobile phase, consisting of 40 mM sodium phosphate dibasic, heptahydrate buffer, and 8% acetonitrile (v/v), pH 5.5. The sample size and the final dilution used for the analyzed vitamin determination varied according to the vitamin content in products.
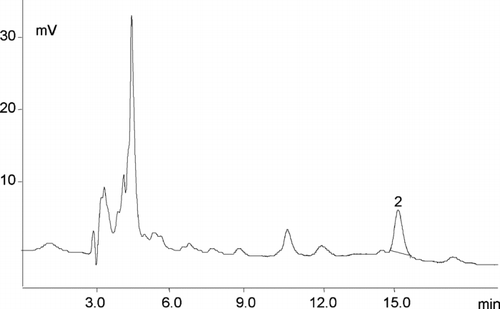
summarizes the results obtained for the determination of folic acid in the analyzed fruit drinks and fruit juices. The levels of folic acid detected by the present method ranged from 58% to 116% compared with the amounts labeled. Only in one case the folic acid concentration was higher than labeled. Consequently, the corresponding serving size of juice samples does not provide the amounts of vitamin expected by the consumers. These mean values were noticeably lower than FA values measured by Breithaupt (Citation2001). The folate contents in some commercial cereal-grain products are presented in . For five cereal-grain products, mean analyzed values ranged from 154.3 to 414.9 μg/100 g−1 of the products and were significantly higher than label statements. Our data indicate that the folate content in some enriched products is slightly higher than expected from the label declaration. Data reported by Rader et al. (Citation1998, Citation2000) for total folate content of enriched cereal-grain products showed that some products were fortified at “higher-than-minimum” levels. The potential for excesses of folic acid is of concern because this vitamin is the type for which a tolerable upper intake level has been recommended (Institute of Medicine Citation1998).
Table 1 Determination of folic acid in commercial vitamin-fortified fruit juices and fruit drinks (μg/100 mL)
Table 2 Determination of folic acid in commercial vitamin-fortified cereal-grain products (μg/100 g)
CONCLUSIONS
The method used in this study (trienzyme deconjugation, extraction procedure, reversed-phase HPLC separation) provides sufficient selectivity to resolve folic acid from other compounds in fortified fruit juices and cereal products and allowed a good separation of the 5-HCO-H4 folate in cereal samples. Coupling this separation with coulometric detection provides a suitable instrumental method for determining folic acid in food samples. The simple mobile phase and the isocratic elution used to separate folic acid and folate yielded low detection limits, good sensitivity, and resolution within a minimum analysis time of 16 min. The simplicity of the procedure, satisfying detection and sensitivity (coulochemic electrochemical), should make our method possible to adopt for routine quality control of folic acid-enriched foods.
REFERENCES
- Akhtar M. J., Khan M. A., Ahnad I. Photodegradation of folic acid in aqueous solution. J. Pharm. Biomed. Anal. 1999; 25: 269–275
- AOAC International. Official Methods of Analysis, , et al. AOAC International, Arlington, VA 2003
- Bagley P. M., Selhub J. Analysis of folate form distribution by affinity followed by reversed-phase chromatography with electrochemical detection. Clin. Chem. 2000; 46: 404–411
- Bailey L. B. Do low doses of folic acid result in maximum lowering of homocysteine?. Am. J. Clin. Nutr. 2005; 82: 717–718
- Ball G. F. M. Vitamins in Foods. Analysis, Bioavailability, and Stability, , et al. Taylor & Francis, Boca Raton, FL 2006
- Breithaupt D. E. Determination of folic acid by ion-pair RP-HPLC in vitamin fortified fruit juices after solid-phase extraction. Food Chem. 2001; 74: 521–525
- Cravo M. L., Camilo M. E. Hyperhomocysteinemia in chronic alcoholism: relations to folic acid and vitamins B6 and B12 status. Nutrition 2000; 16: 296–302
- Das U. N. Folic acid says NO to vascular diseases. Nutrition 2003; 19: 686–692
- De Souza S, Eitenmiller R. Effects of different enzyme treatments on extraction of total folate fron various foods prior to microbiological assay and radioassay. J. Micronutrient Anal. 1990; 7: 37–57
- Eitenmiller R., Landen W. O. Vitamin Analysis for the Health and Food Sciences., , et al. CRC Press, Boca Raton, FL 1999
- Finglas P. M., Meer K., Molloy A., Verhoef P., Pietrzik K., Powers H. J., Straeten D., Jägerstad M., Varela-Moreiras G., Vliet T., Havenaar R., Buttriss J., Wright A. J. A. Research goals for folate and related B vitamin in Europe. Eur. J. Clin. Nutr. 2006; 60: 287–294
- Flangan R. J., Perrett D., Whelpton R. Electrochemical Detection in HPLC. Analysis of drugs and poisons (RSC Chromatography Monographs). , et al. Royal Society of Chemistry, Cambridge 2005
- Gregory J. F., III, Sartain D. B., Day B. P. F. Fluorimetric determination of folacin in biological materials using high performance liquid chromatography. J. Nutr. 1984; 114: 341–353
- Gujska E., Kuncewicz A. Determination of folate in some cereals and commercial cereal-grain products consumed in Poland using trienzyme extraction and high-performance liquid chromatography methods. Eur. Food Res. Technol. 2005; 221: 208–213
- Heudi O., Kiline T., Fontannaz P. Separation of water-soluble vitamins by reversed-phase high performance liquid chromatography with ultra-violet detection: application to polyvitamined premixes. J. Chromatogr. A. 2005; 49: 1070–56
- Hyun T. H., Tamura T. Trienzyme extraction in combination with microbiologic assay in food folate analysis: an updated review. Exp. Biol. Med. 2005; 230: 444–454
- Institute of Medicine. Dietary Reference Intakes for Thiamin, Riboflavin, Niacin, Vitamin B6, Folate, Vitamin B12, Pantothenic Acid, Biotin, and Choline. Institute of Medicine, Food and Nutrition Board. , et al. National Academy Press, Washington, D.C. 1998; 8: 197–305
- Johnston K. E., Lofgren A., Tamura T. Folate concentrations of fast foods measured by trienzyme extraction method. Food Res. Internal. 2002; 35: 565–569
- Malinow M. R., Bostom A. G., Krauss R. M. Homocyst(e)ine, diet, and cardiovascular diseases, a statement for healthcare professionals from the Nutrition Committee, American Hearth Association. Circulation 1999; 99: 178–182
- Pfeiffer C. M., Rogers L. M., Gregory J. F., III. Determination of folate in cereal-grain food products using trienzyme extraction and combined affinity and reversed-phase liquid chromatography. J. Agric. Food Chem. 1997; 45(2)407–413
- Rader J. I., Weaver C. M., Angyal G. Use of a microbiological assay with tri-enzyme extraction for measurement of pre-fortification levels of folates in enriched cereal-grain products. Food Chem. 1998; 62: 451–465
- Rader J. I., Weaver C. M., Angyal G. Total folate in enriched cereal-grain products in the United States following fortification. Food Chem. 2000; 70: 275–289
- Ruggeri S., Vahteristo L. T., Aguzzi A., Finglas P., Carnovale E. Determination of folate vitaminers in food and in Italian reference diet by high-performance liquid chromatography. J. Chromatogr. A. 1999; 855: 237–245
- Tamura T. Determination of food folate. Nutr. Biochem. 1998; 9: 285–293
- Yon M., Hyun T. T. Folate content of foods commonly consumed in Korea measured after trienzyme extraction. Nutr. Res. 2003; 23: 735–746