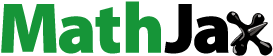
ABSTRACT
Background: The present study aims to further explore the role of STK33 in hypopharyngeal squamous cell carcinoma (HSCC), with special attention given to the possible relationship between STK33 alteration and calcium. Methods: An in vivo experiment and microarray analysis were performed to investigate the impact of STK33 knockdown (STK33-RNAi) on the biological behaviors and the gene profile alterations of a HSCC cell line (Fadu). Cell viability and morphological change of Fadu cells in response to Ionomycin were measured by MTT assay and acridine orange staining. The concentration of intracellular calcium ([Ca2+]i) was detected by laser scanning confocal microscope with fluo-3/AM. The mRNA and protein expressions of relevant genes were examined by real-time PCR and Western blot. Results: STK33-RNAi retarded the Fadu cell proliferation and the metastasis in nude mice and led to up- and down-regulation of the expressions of abundance of genes, especially, the downregulation of the CAPN1 gene. Ionomycin increased the [Ca2+]i and decreased the survival rates of Fadu cells in a time-dependent manner. Moreover, Ionomycin resulted in the elevation of CAPN1 mRNA expression in normal Fadu cells and, conversely, had almost no effect on CAPN1 expression in STK33-RNAi cells. Conclusions: Findings from this work further validate that STK33 is a potential oncogene and plays an important role in tumorigenesis of HSCC via regulation of numerous genes. In addition, there exists the reciprocal influence between STK33 and [Ca2+]i in Fadu cells.
KEYWORDS:
Background
Hypopharyngeal squamous cell carcinoma (HSCC) is a common human malignancy worldwide, which results in substantial morbidity and mortality annually.Citation1 Despite new treatment options that have been employed to treat this tumor, the survival rate has not been dramatically improved during the last 2 decades.Citation2,3 Such a situation is mainly due to the development of distant metastases and the emergence of therapy-resistant recurrences.Citation4 Therefore, it is indispensable to gain a deeper understanding of the biology of this disease, which, in turn, may lead to developing more effective therapies.
It is widely recognized that most cancers result from a multifactorial process that includes the activation of oncogenes as well as the inactivation of tumor suppressor genes.Citation1 Although the initiation and progression of HSCC involve numerous genetic and epigenetic factors, the molecules that participate in tumorigenesis of this kind of carcinoma are still little known. Serine/threonine kinase 33 (STK33) gene, which was discovered by comparative genome sequencing of the human chromosome 11 region 11p15,Citation5 encodes a serine/threonine kinase. This protein is a member of the calcium/calmodulin dependent kinase (CAMK) family, which exhibits a non-ubiquitous and low level of expression in most tissues.Citation6 Available data show that STK33 participates in the dynamic changes in depolymerization of cytoskeleton and affects the cell structure and a series of important functions by specifically phosphorylating the intermediate filament protein vimentin.Citation7 More recently, compelling evidence has indicated the involvement of serine/threonine kinase 33 (STK33) gene in tumorigenesis.Citation8 However, the precise function and mechanism of STK33 in HSCC have been sparingly studied and remain unclear as yet.
Previous studies have documented that the serine/threonine kinases of the CAMK family participate in a wide variety of the biological processes in cells.Citation9 Activity of these protein kinases can be regulated by numerous chemical signals, including Ca2+/calmodulin. Since STK33 belongs to the CAMK family, thus, it is conceivable that the regulation of STK33 by intracellular Ca2+ might contribute, at least in part, to the STK33-mediated tumorgenisis. It is well known that Ca2+, which has been recognized as a primary element of cells, is critically involved in the determination of the cell fate. The change of intracellular free calcium is a common signal mechanism that modulates physiological processes in most cells.Citation10
However, whether STK33 is regulated by Ca2+/calmodulin or regulates Ca2+/calmodulin has not been explored till now. Therefore, the present study, on the basis of our previous study,Citation11 was designed to further investigate the role of STK33 via an in vivo experiment and microarray analysis in STK33-RNAi Fadu cells, with special attention given to the possible relationship between STK33 alteration and intracellular calcium level.
Methods
Cell culture
The HSCC cell line, Fadu cells (Biosis, Shanghai, China), was cultured in the DMEM supplemented with 10% fetal bovine serum and streptomycin (100 µg/ml) and penicillin (100 U/ml) in a humid incubator (37°C, 5% CO2).
Isolated cells were seeded at a density of 5×105 cells/well in 6-well plate for one day. Then after treatment with 1.5 μM Ionomycin for 1 h, 2 h, 4 h, 6 h, and 24 h respectively, protein and RNA were extracted from cells to do the Western blot and PCR.
Infection of lentiviral vectors with specific RNAi for STK33
STK33-RNA interference (RNAi) lentiviral vector (GV115-GFP-STK33 shRNA) and GFP-lentiviral vector (GV115-GFP), which was applied as a negative control (Mock RNAi), were constructed by GeneChem Co, Ltd (Shanghai, China). Isolated cells were seeded in a 50 ml culture flask at a concentration of 5×105 cells/flask. Cells were infected with lentivirus at a multiplicity of infection (MOI) of 10 particles/cell in complete medium plus 5 µg/ml Polybrene(Sigma, MO, USA) at 37°C, 5% CO2. Twelve hours later, the medium with lentivirus was changed to the complete medium. Cells were observed by fluorescent microscopy everyday. On the fifth day, infected cells were used in the experiment.
Animal studies
Use of animals for these experiments was approved by the Ethics Committee of the Provincial Hospital Affiliated with Shandong University. The animal care and experimental protocol were approved by The Animal Care Committee of Shandong University, P.R. China (NO. ECAESDUSM 20123011).
Thirty nu/nu male nude mice (5 weeks old, Vital River, Beijing, China) were randomly divided into 3 groups: control group(n = 9), mock group(n = 9) and STK33-RNAi group(n = 12). The mice were housed in laminar flow cabinets under specific pathogen-free conditions and fed ad libitum 1×106 cells/mouse were injected subcutaneous in the right axilla of the mice. Tumor volume (in mm3) was determined by caliper measurements performed every other day and calculated by using the following formula: volume = length×width2×0.5. The tumors that arose in those mice were harvested when they all reached 1.5 cm in diameter in control group. The tumors and lungs were taken from the mice. Then the tissues were fixed with 4% paraformaldehyde and the paraffin-embedded tissue blocks were made.
Hematoxylin-eosin staining and immunohistochemistry (IHC)
3–5 μm sections were cut from formalin-fixed paraffin-embedded tissue blocks, and then IHC and H&E staining were carried out. Briefly, the sections were stained with hematoxylin for 5–8 min and eosin for 2 min respectively. Changes of basic morphology were observed under light-microscopy.
As for IHC staining, the sections were performed with 3% hydrogen peroxide for 15 min at 37°C to quench the endogenous peroxidase, and citrate buffer(pH = 6.0) for the antigen retrieva. The non- specific binding was blocked by treatment with the blocking reagent, and then the slides were incubated with anti-STK33 antibody (1:100, Santa Cruz, CA, USA) at 4°C overnight. Subsequently, the slides were incubated at room temperature for 1 h with secondary antibody (ZSGB, Beijing, China), and then DAB was used for visualization of the immunoreaction. PBS instead of anti-STK33 antibody was added for the negative control. The level of STK33 protein expression was analyzed by using an Image-Pro Plus 6.0.
Detection of [Ca2+]i
Isolated cells were seeded at a density of 1.6×104 cells/ well in a confocal special dish for one day. Then cells were loaded with 10 μM Fluo-3/AM (Beyotime, China) for 30 min at 37°C. The fluorescence intensity was quantified using laser scanning confocal microscope at an excitation of 488 nm and an emission of 535 nm.
Acridine orange staining
After being treated with 1.5 μM Ionomycin for 0 h and 6 h respectively, the slides were incubated with 0.01% acridine orange at room temperature for 5 min in dark, and imaged immediately after washing.
Assessment for cell viability by MTT
Isolated cells were seeded at a density of 0.6×104 cells/ well in a 96-well plate for one day. Then the cells were treated with or without 1.5 μM Ionomycin for 0 h, 6 h, 12 h, and 24 h respectively. MTT (5 mg/ml, 20 μl) was added to each well 4 hours before the indicated time points. Then the medium was changed to 100 μl DMSO to dissolve the formazan. The plates were shaken for 20 min to dissolve and homogenize the well contents completely, and the absorbance(OD) was measured at 570 nm on an ELISA Reader (Thermo Multiskan MK3, Finland). The mean values were obtained from triplicate experiments. The cell relative viability rate was calculated according to the following formula:
(ODblank is adjusted to zero)
Microarray analysis
Affymetrix HTA2 dataset analysis was conducted by Shanghai Biotechnology Corporation (SBC). We conducted a Diffgene which is an operational method combining t-text and SAM to compare gene difference between Fadu cells and STK33-RNAi Fadu cells. Genes were considered significantly differentially expressed when fold change was ≥2 and p ≤ 0.05.
RNA extraction and qRT-PCR
Total RNA from the samples was extracted with TRIzol (Invitrogen, USA). Then, RNA was reverse transcribed into cDNA (Takara, Dalian, China).
The expression of STK33 was evaluated by qRT-PCR, which was performed on the Eppendorf AG 22331 Hamburg system using SYBR Green real-time PCR (Takara, Dalian, China). PCR primers (Sangon Biotech, Shanghai, China) for the genes were listed in. qRT-PCR conditions were as follows: 95°C for 2 min; then 40 cycles of 95°C for 15 s, renaturation at different temperatures, and 72°C for 40 s. Data were analyzed using the ΔΔCt method and GAPDH served as the internal control. PCR conditions were as follows: 94°C for 5 min, 94°C for 40 s, then 35 cycles of Tm °C for 40 s, and 72°C for 90 s, 72°C for 10 min.
Table 1. Primer, amplicon size and annealing temperature of each gene for qRT-PCR and PCR.
Western blot
Total proteins were extracted with lysis buffer according to the protocol. The protein concentration of the samples was measured using the BCA protein assay kit (Beyotime, Shanghai, China). Then, 20 μg protein sample was denatured and separated by 10% SDS-PAGE gels electrophoresis and transferred onto PVDF (polyvinylidene fluoride) membranes. The membranes were blocked overnight at 4°C and were incubated with antibody to STK33 (1:500, Santa Cruz, CA, USA) for 2 h at room temperature, or were blocked for 1 h at room temperature and were incubated with antibody to β-actin (1:2000, Biosynthesis, Beijing, China) overnight at 4°C. Subsequently, the membranes were incubated with the secondary antibody (1:5000) for 1 h at room temperature. Finally, protein signals were detected by Chemiluminescent reagents (Millipore, USA) and visualized after exposure to the X-ray film. The relative optical density ratio was calculated with the Image J software by comparison to β-actin.
Statistical analysis
Data were expressed as mean ± standard error of mean (SEM) and statistically analyzed by one-way analysis of variance (ANOVA), and the LSD method was used to compare the difference between different groups. Statistical analysis was performed using the SPSS17.0 software package. Values of p ≤ 0 .05 were considered to be statistically significant.
Results
STK33 inactivation inhibits HSCC tumorigenesis and metastasis in vivo
To assess the effect of STK33 on tumorigenesis in vivo, the STK33-RNAi-expressing Fadu cell was stably established. STK33 knockdown in stable clones was confirmed by PCR (). Cells were subcutaneously injected into the right flank of male nude mice, and the growth of the resultant primary tumors was monitored. As shown in, after injection, the Fadu cells (control group) developed into a tumor mass on day 14 and on 39th day the average tumor volume of control group was 694.3 ± 83 .7 mm3. The tumors formed by the Mock Fadu cells (mock group) exhibited a growth rate similar to that of the control group, and reached a volume of 566.2 ± 196 .2 mm3 on day 39. However, the stable STK33-RNAi-expressing Fadu cells (STK33-RNAi group) grew slowly, and on day 39, only formed small tumors, which were 91.7 ± 36 .6 mm3, hence were about 16.2% of the control ones. There was a significant difference in tumor size between STK33-RNAi group and control/mock groups. These data demonstrate that STK33 knockdown effectively suppressed Fadu tumor growth in vivo ().
Figure 1. Effects of STK33 on tumor growth in vivo. (A) Infection of STK33-RNAi into Fadu cells led to a significant decrease in STK33 mRNA expression compared with that in the normal Fadu cells. Data represent the mean ± SEM , *p < 0 .05. (B) Gross appearance of tumor-bearing nude mice and the excised tumor specimens at the end of the treatment. Photographs of animals and tumors were representative from each group. a) control group, b) mock group, c) STK33-RNAi group. (C) Tumor volume and formation time of STK33-RNAi cells derived xenografts differed from those of normal and mock cells. STK33-RNAi markedly decreased the volume of the tumor and delayed the formation of the tumor. Data represent the mean ± SEM, *p < 0 .05. (D) Representative images of STK33 IHC staining and correspondingly quantitative analysis in the 3 groups. STK33-RNAi significantly decreased the STK33 expression in the tumor tissue. Data represent the mean ± SEM, *p < 0 .05. (E) Microscopic view of tumor metastasis by HE staining. a) control group, b) STK33-RNAi group.
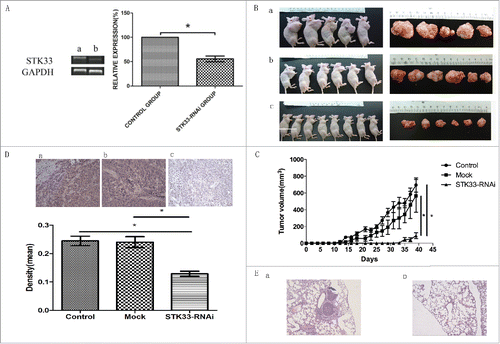
The IHC staining was performed to validate that the cells originated from injected cells. The results showed that STK33 expression in STK33-RNAi cells was much weaker than that in the controls (). Bar graph showed the quantitative data for STK33 obtained by IHC staining using Image Pro Plus 6.0. The mean density values further confirmed the decreased levels of STK33 in tumor tissues.
To further validate the effect of STK33 on tumor invasion, the invasion clones formed on the lungs were checked. As shown in , the histological study with H&E stained paraffin block sections confirmed that control group showed dissemination (); in contrast, STK33-RNAi group showed no dissemination ().
Collectively, knockdown of STK33 resulted in cells that were significantly less tumorigenic compared to the control cells in vivo, indicating that STK33 is a functional gene expressed in the cells to regulate the tumorigenesis, metastasis ability and invasiveness of Fadu cells in vivo.
Alteration of gene expression profiles in response to STK33 knockdown
Given the evidence for a critical pro-proliferative role of STK33 gene in Fadu cells, we employed microarray technology, which can define the gene expression signatures, to determine whether silencing of STK33 in Fadu cells can result in the alteration in gene expression programs in comparison to those in the normal Fadu cells. The STK33-RNAi-expressing Fadu cell was stably established. Differentially expressed genes were determined by microarray analyses using 3 biological replicates from independent knockdown experiments of Fadu cells, as well as control cells.
To visualize the different expression of STK33-RNAi and control group, the Volcano plot was made (). Based on comparison analysis algorithm, STK33-RNAi remarkably altered the expressions of different combinations of probe sets. In STK33-RNAi cells, noticeable expression changes were identified in 140 symbolized genes through microarray analyses. These genes were significantly (p < 0 .05) up- (n = 86) or down-regulated (n = 54).
Figure 2. Differential gene expression values in Fadu cells upon STK33-RNAi. (A) Composite hierarchical and conditional clustering of statistically significant alterations in gene expression from Fadu and STK33-RNAi Fadu cells. Gene expression levels are shown by red for high and green for low expressing genes. (B) Volcano plot showing an overview of gene expression in Fadu and STK33-RNAi Fadu cells. Each dot represented the mean regulation value (log2-fold change of STK33-RNAi versus control cells) of 3 replicas and the p-value(-log10 of p-values). x-axis: Log2(Fold change), y-axis: -log10(p-value). Negative values indicated a decrease and positive values showed an increase in gene expression upon fusion gene knockdown. Red Region: p-value<0 .05, Fold Change≥2 . (C) Changes of selected gene in microarray. (D) STK33-RNAi infection led to a significant decrease in CAPN1 mRNA expression compared with that in the normal Fadu cells. a) control group, b) STK33-RNAi group. (E) Data represent the mean± SEM, *p < 0 .05.
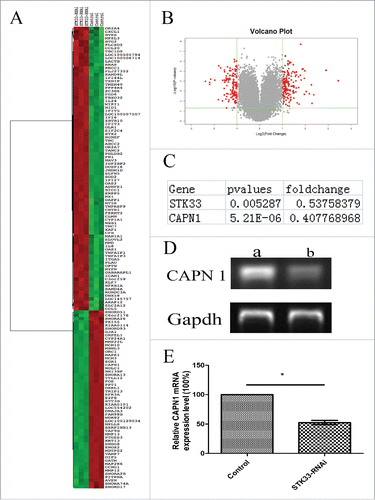
To determine whether specific signal pathways were involved in the STK33 knockdown-induced anticancer effects, we conducted pathway analyses. By defining the enrichment p value of the pathway ≤0 .05, we used well-known pathway interaction databases (Biocarta) to determine the genes that potentially interact with STK33. The investigation indicates that STK33 influences expression of many genes, and regulates many pathways, hence STK33 plays an important role in tumor cells.
To obtain an overview of the biological processes affected by STK33, we analyzed lists of regulated targets of the pathways in our data set using the SBC Analysis System (SBC). Calpain 1(CAPN1) which is a Ca2+-activated non-lysosomal cysteine proteaseCitation12 was found(). Then, PCR analysis of the mRNA level of CAPN1 was performed to verify the difference. The expression of CAPN1 was decreased significantly (p < 0 .05) in STK33-RNAi Fadu cells (). It shows that STK33 knockdown decreases the expression of CAPN1.
Effect of Ionomycin on [Ca2+]i
To determine whether Ionomycin affects [Ca2+]i in Fadu cells, fluorescence intensity of intracellular calcium in Fadu cells was assayed by confocal microscopy using calcium-sensitive dye, Fluo-3/AM. As shown in, [Ca2+]i almost instantly stepped up after addition of the Ionomycin, and attained its peak within 20 s, about 3.5-fold, with a minor [Ca2+]i reduction subsequently. These results demonstrate that the Ionomycin induced rapid increase in [Ca2+]i..
Effect of STK33-RNAi or Ionomycin on cell viability, apoptosis in Fadu cells
The effects of Ionomycin on morphological changes of Fadu cells were examined by acridine orange staining at 6 h after treatment with Ionomycin. As illustrated in , untreated cells showed polygonal shape, while the typical characteristics of apoptosis were presented in the cells treated with Ionomycin, including irregular cell shape, cell shrinkage, chromatin condensation, apoptosis bodies. These differences indicate that Ionomycin could predominately induce apoptosis in Fadu cells.
Figure 4. Effects of STK33-RNAi and Ionomycin on Fadu cells and in vitro cell function test. (A) Induction of apoptosis in Fadu cells by Ionomycin as assessed by AO staining. a) control, b) treated with Ionomycin for 6h. (B) Decrease in cell viability by Ionomycin appeared in time-manner. STK33-RNAi group had higher cell viability than the control group. Data represent the mean ± SEM, *p < 0 .05.
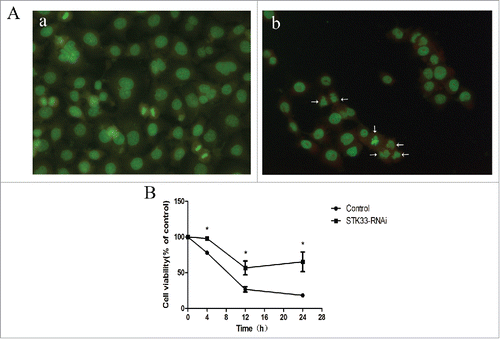
In order to assess the cytotoxic effect of STK33-RNAi and/or Ionomycin on the HSCC, the MTT assay was carried out. As depicted in , a trend of decreasing viability with increasing treatment-time was significantly observed in Fadu cells, indicating that Ionomycin inhibited cell viability in a time-dependent manner. Furthermore, the exposure of STK33-RNAi Fadu cells to Ionomycin didn't result in as big a decrease of cell viability as with exposure of Ionomycin in Fadu cells (p < 0 .05).
Effect of Ionomycin on protein expression of STK33 in Fadu cells
To determine the effect of Ionomycin on the protein expression of STK33, western blot was performed. Compared with untreated Fadu cells, the protein expression of STK33 increased after exposure to Ionomycin for 1, 2, 4 and 6 hours (). Statistical analysis showed that the protein expression of STK33 was significantly higher in 1, 2, 4, and 6 hours groups in comparison with those in the control group (p < 0 .05) (). It is concluded that Ionomycin can increase the expression of the STK33 protein.
Figure 5. Effect of Ionomycin in Fadu cells on STK33 protein expression. (A) Representative protein gel blots showed the alterations in protein expressions of STK33 in the cells after exposure to 1.5 μM Ionomycin for 0 h, 1 h, 2 h, 4 h, 6 h and 24 h. (B) Graphs show the mean ± SEM after normalization to β-Actin protein. Results shown as means ± SEM, *p < 0 .05.
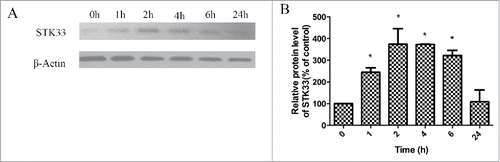
STK33 may protect the cell from high [Ca2+]i by calpain
qRT-PCR analyses of the mRNA levels of CAPN1 were performed to estimate the relationship between STK33 and CAPN1. The expression of CAPN1 was increased significantly(p < 0 .05) in Fadu cells treated with Ionomycin for 1, 2, 4, 6 and 24 hours (). However, for the STK33-RNAi group, there was no significantly difference before and after the treatment ().
Figure 6. Effect of Ionomycin in Fadu cells on CAPN1 mRNA expression. (A) Quantification of mRNA expressions of CAPN1 in the Fadu cells treated with 1.5 μM Ionomycin for 0 h, 1 h, 2 h, 4 h, 6 h and 24 h qRT-PCR. Results shown as means ± SEM, *p < 0 .05. (B) Quantification of mRNA expressions of CAPN1 in the STK33-RNAi Fadu cells treated with Ionomycin by qRT-PCR. Results shown as means ± SEM, *p < 0 .05.
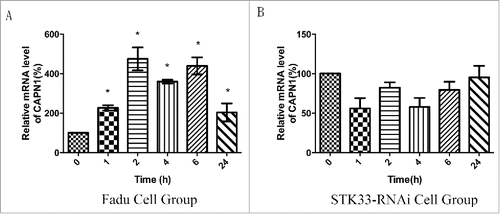
Discussion
Recent studies on human somatic tumors have documented that STK33 acts as a novel tumor gene.Citation13,14 To date, however, little is known about the precise mechanism(s) by which STK33 exerts its effects in tumorigenesis. The present study represents the natural extension of our preliminary studies on Fadu cellsCitation11 so as to gain further insight into mechanisms underpinning the action of STK33.
Studies have shown that STK33 is a potential oncogene and it is an essential component of the signaling pathways to maintain the transformed phenotype of cancer cells, such as AML, lung cancer, glioblastoma, et al. In our previous study, we found that STK33 is overexpressed in HSCC specimens and it is significantly associated with certain clinicopathological parameters. In this work, we initially found that STK33-RNAi retarded the tumor proliferation as evidenced by the gross appearance of xenograft tumors and the decrease in tumor volume. In addition, we did not observe the metastasis to lung in the STK33-RNAi group. Those findings indicate that RNAi-mediated silencing of STK33 gene impairs the repopulation capacity of the treated Fadu cells in vivo, thereby proving that STK33 itself contributes to the promotion of tumor growth, suggesting that STK33 plays an important role in tumorigenesis. Taken together, data from this in vivo study further validate our previous conclusion that STK33 is a potential oncogene from the study in vitro and on resected specimens.Citation11
It is known that apoptosis caused by STK33 suppression is mediated via the mitochondrial pathway, PI3K/AKT cascade or the mitogen-activated protein kinase (MAPK) signaling pathway. We found that the knockout of STK33 was able to consistently lead to the up- and downregulated expressions of masses of genes, certain of which paly a critical role in some well-known pathways to control the pivotal properties of cancer cells, inclusive of differentiation, invasion, metastasis, or survival,Citation11 such as MAPK pathway or NF-kB Signaling Pathway. Those results imply that the STK33 gene exert its effects in Fadu cells via multiple signaling networks, in which numerous genes were involved in key specific functions critical for tumor progression. The precise mechanism (s) underlying the interactions among STK33 and those up- or down-regulated genes under the challenge of STK33-RNAi merits further study.
Of those aforementioned genes, CAPN1, which belongs to the calpain superfamily, was markedly downregulated in its mRNA expression in response to STK33-RNAi intervention. It has been documented that calpains are intracellular cysteine-proteases described as being calcium-dependent in vitro, which have a broad spectrum of substrates from proteins of the cytoskeleton to transcription factors, and enzymes, which are thought to be critical for the cell migration, survival, cell cycle, apoptosis and adhesion.Citation15 The findings from this study indicate that CAPN1 activity is altered by STK33-RNAi, implying that CAPN1 is implicated in the STK33-initiated tumorigenesis in Fadu cells. These findings might provide a clue to the process by which STK33 links to calcium in Fadu cells because STK33 is classified as a member of the CAMK family. This leads us to focus on the investigation of the correlation of STK33 activity with the intracellular calcium alteration in Fadu cells.
Ionomycin is an ionophoric polyether antibiotic with affinity for Ca2+, which is produced by Streptomyces conglobatus.Citation16 It is used to raise the intracellular level of calcium in research and as a tool to understand calcium transport across biological membranes. In the present study, we found that exposure of the cells to Ionomycin (1.5 µM) led to an increase in [Ca2+]i. It is well known that high intracellular level of calcium induces cell apoptosis. Correspondingly, administration of Ionomycin decreased the survival rates of Fadu cells in a time-dependent manner as shown by MTT assay, suggesting that Ionomycin was able to inhibit the growth of Fadu cells in vitro. This indicates that Ionomycin exerts its cytocoxity on Fadu cells due to the elevation of [Ca2+]i level. Of particular interest is that STK33-RNAi could counteract the Ionomycin-induced injury on the cells to a certain degree, implying that STK33 knock-out may protect cell damage from high [Ca2+]i triggered by Ionomycin.
Lastly, we explored the possible mechanism of the reciprocal influence between STK33 and [Ca2+]i in Fadu cells. We found that Ionomycin led to the increase in STK33 protein expression, suggesting that high [Ca2+]i promotes the translation activity of STK33 gene. Moreover, Ionomycin resulted in the elevation of CAPN1 mRNA expression in normal Fadu cells and, conversely, had almost no effect on CAPN1 mRNA expression in STK33-RNAi cells, indicating calpain transcription is, indeed, altered in Fadu cells under the condition of STK33 knockdown. This offers evidence that intracellular free calcium might impact the CAPN1 expression via STK33 activity, thereby implicating several fundamental signal transduction pathways, which are closely related to the important role of STK33 in tumorigenesis.
Taken together, this is the first report identifying CAPN1 as a target of STK33, thereby providing the new interconnection between STK33 and CAPN1. We demonstrate that STK33 knockdown reduces cell proliferation and metastasis of Fadu cells in vivo. In addition, there exists a reciprocal influence between STK33 and [Ca2+]i in Fadu cells.
Disclosure of potential conflicts of interest
No potential conflicts of interest were disclosed.
Funding
This work was supported by a grant from the Natural Science Foundation of China (No. 81072200).
References
- Argiris A, Karamouzis MV, Raben D, Ferris RL. Head and neck cancer. Lancet 2008; 371:1695-709; PMID:18486742; http://dx.doi.org/10.1016/S0140-6736(08)60728-X
- Singh B. Molecular pathogenesis of head and neck cancers. Jsurg oncol 2008; 97:634-9; PMID:18493941; http://dx.doi.org/10.1002/jso.21024
- Society AC. Cancer Facts & Figures 2015. Atlanta: American Cancer Society 2015
- Longley DB, Johnston PG. Molecular mechanisms of drug resistance. J pathol 2005; 205:275-92; PMID:15641020; http://dx.doi.org/10.1002/path.1706
- Mujica AO, Hankeln T, Schmidt ER. A novel serine/threonine kinase gene, STK33, on human chromosome 11p15.3. Gene 2001; 280; PMID:11738831; http://dx.doi.org/10.1016/s0378-1119(01)00780-6
- Mujica AO, Brauksiepe B, Saaler-Reinhardt S, Reuss S, Schmidt ER. Differential expression pattern of the novel serine/threonine kinase, STK33, in mice and men. FEBS J 2005; 272:4884-98; PMID:16176263; http://dx.doi.org/10.1111/j.1742-4658.2005.04900.x
- Brauksiepe B, Mujica AO, Herrmann H, Schmidt ER. The Serine/threonine kinase Stk33 exhibits autophosphorylation and phosphorylates the intermediate filament protein Vimentin. BMC Biochem 2008; 9:25; PMID:18811945; http://dx.doi.org/10.1186/1471-2091-9-25
- Yang T, Song B, Zhang J, Yang GS, Zhang H, Yu WF, Wu MC, Lu JH, Shen F. STK33 promotes hepatocellular carcinoma through binding to c-Myc. Gut 2014; 65:124-33; PMID:25398772; http://dx.doi.org/10.1136/gutjnl-2014-307545
- Ishida A, Sueyoshi N, Shigeri Y, Kameshita I. Negative regulation of multifunctional Ca(2+)/calmodulin-dependent protein kinases: physiological and pharmacological significance of protein phosphatases. Br J Pharmacol 2008; 154:729-40; PMID:18454172; http://dx.doi.org/10.1038/bjp.2008.127
- Wayman GA, Tokumitsu H, Davare MA, Soderling TR. Analysis of CaM-kinase signaling in cells. Cell Calcium 2011; 50:1-8; PMID:21529938; http://dx.doi.org/10.1016/j.ceca.2011.02.007
- Huang L, Chen C, Zhang G, Ju Y, Zhang J, Wang H, Li J. STK33 overexpression in hypopharyngeal squamous cell carcinoma: possible role in tumorigenesis. BMC cancer 2015; 15:13; PMID:25603720; http://dx.doi.org/10.1186/s12885-015-1009-3
- Smith MA, Schnellmann RG. Calpains, mitochondria, and apoptosis. Cardiovasc Res 2012; 96:32-7; PMID:22581845; http://dx.doi.org/10.1093/cvr/cvs163
- Carter H, Samayoa J, Hruban RH, Karchin R. Prioritization of driver mutations in pancreatic cancer using cancer-specific high-throughput annotation of somatic mutations (CHASM). Cancer Biol Ther 2010; 10:582-7; PMID:20581473; http://dx.doi.org/10.4161/cbt.10.6.12537
- Scholl C, Frohling S, Dunn IF, Schinzel AC, Barbie DA, Kim SY, Silver SJ, Tamayo P, Wadlow RC, Ramaswamy S, et al. Synthetic lethal interaction between oncogenic KRAS dependency and STK33 suppression in human cancer cells. Cell 2009; 137:821-34; PMID:19490892; http://dx.doi.org/10.1016/j.cell.2009.03.017
- Leloup L, Wells A. Calpains as potential anti-cancer targets. Expert Opin Ther Targets 2011; 15:309-23; PMID:21244345; http://dx.doi.org/10.1517/14728222.2011.553611
- Liu WC, Slusarchyk DS, Astle G, Trejo WH, Brown WE, Meyers E. Ionomycin, a new polyether antibiotic. J Antibiot 1978; 31:815-9; PMID:711623; http://dx.doi.org/10.7164/antibiotics.31.815