Abstract
Mesenchymal stem cells (MSC) participate to tumor stroma development and several evidence suggests that they play a role in facilitating cancer progression. Because melanoma often shows extracellular pH low enough to influence host cell as tumor cell behavior, the aim of this study is to elucidate whether acidity affects cross talk between MSC and melanoma cells to disclose new liaisons promoting melanoma progression, and to offer new therapeutic opportunities. We found that MSC grown in a low pH medium (LpH-MSC) stimulate melanoma xenografts more than MSC grown in a standard pH medium. LpH-MSC express a higher level of TGFβ that is instrumental of epithelial-to-mesenchymal transition (EMT)-like phenotype induction in melanoma cells. LpH-MSC profile also shows a switching to an oxidative phosphorylation metabolism that was accompanied by a forced glycolytic pathway of melanoma cells grown in LpH-MSC-conditioned medium. Metformin, an inhibitor of mitochondrial respiratory chain was able to reconvert oxidative metabolism and abrogate TGFβ expression in LpH-MSC. In addition, esomeprazole, a proton pump inhibitor activated in acidosis, blocked TGFβ expression in LpH-MSC through the downregulation of IkB. Both agents, metformin and esomeprazole, inhibited EMT profile in melanoma cells grown in LpH-MSC medium, and reduced glycolytic markers. Thus, acidosis of tumor microenvironment potentiates the pro-tumoral activity of MSC and orchestrates for a new potential symbiosis, which could be target to limit melanoma progression.
Abbreviations
MSC | = | Mesenchymal stem cells |
EMT | = | epithelial to mesenchymal transition |
OxPhos | = | oxidative phosphorylation |
TGF β | = | transforming growth factor-β |
MMP | = | Matrix metalloproteinases |
pHe | = | extracellular pH |
HIF-1α | = | Hypoxia-inducible factors-1α |
Introduction
Human bone marrow-derived mesenchymal stem cells (MSC), when stimulated by specific signals, are released from their niche into circulation and recruited into target tissues where they contribute to tissue homeostasis.Citation1 Several characteristics of MSC, such as potential to differentiate into multiple lineages and ability to expand ex vivo while retaining their original lineage differentiation commitment, make these cells interesting tool for regenerative therapy. Furthermore, MSC upon expansion in culture can be genetically manipulated and used appropriately.Citation2 Among the several conditions able to recruit MSC, tumors are particularly efficient, thus, large number of MSC participate to the developing tumor-associated stroma.Citation3 Indeed, MSC labeled ex vivo and injected i.v. into tumor-bearing animals localize preferentially to tumor microenvironment.Citation4 Role of MSC in modulating cancer progression is still under debate, with some indications suggesting antitumor activity,Citation5,6 and some others showing promoting activity.Citation4,7-9 MSC, whether mixed with low metastatic breast cancer cells, induce an increased metastatic dissemination through a paracrine manner elicited by the CCL5 chemokine-CCR5 receptor interactionsCitation9 or interleukin 17B/IL-17B receptor signaling.Citation4 MSC also trigger in human colorectal cancer cells an increased invasiveness, which requires a cell-to-cell contact mediated by TGFβ.Citation10 A pro-oncogenic role of MSC was demonstrated in human prostate cancer cells; in particular, medium conditioned by MSC stimulates proliferation, migration and MMP-dependent invasion.Citation11 Thus, MSC-tumor cell interaction acquires a particular importance and further understanding of these interactions is required to determine their role in tumor progression. Among the several alterations of tumor stroma influencing these cell interactions, the low pH might be critical.Citation12-14 Extracellular pH (pHe) of tumors is frequently acidic as a result not only of poor perfusion but also of a proper metabolic activity of tumor cells themselves. It is known that tumor cells use glycolysis even when there is enough O2 to support mitochondrial function (aerobic glycolysis), a phenomenon called “Warburg effect.”Citation15,16 When oxygen tension reduces, HIF-1α-dependent glycolytic genes are readily induced in tumor cells and an anaerobic glycolysis develops.Citation17,18 The increased glucose uptake by tumor cells may be visualized in tumor-bearing patients using 18F-deoxyglucose positron emission tomography (18FdG–PET) imaging. Both, aerobic and anaerobic glycolysis produce acidic metabolites, which are promptly extruded and tumor microenvironment becomes acidic. Now, pH-sensitive PET radiotracers may be used for in vivo measurement of tumor pH, a water-soluble membrane peptide that inserts and folds across a cellular membrane lipid bilayer in response to low pH has been synthetized and used to detect tissue acidity and to diagnose primary tumors and metastatic lesions.Citation19
Because melanoma represents one of the most aggressive human cancer able to metastasize to multiple sites, and, as most solid tumors, shows extracellular acidosis, we decided to elucidate whether acidity affects cross-talk between MSC and melanoma cells, also to disclose new evil liaisons promoting melanoma progression and, possibly, to offer new strategies for therapy. We found that melanoma cells injected into immunodeficient mice together with MSC exposed to a low pH (pH 6.7) medium generate tumors with a higher growth rate than melanoma cells injected alone or together with MSC grown in standard pH medium. Phenotype acquired by melanoma cells exposed to a medium conditioned by acidic MSC express a mesenchymal-like profile and a new potential metabolic symbiosis between acidic MSC and melanoma cells is described. Novel therapeutic interventions are investigated.
Results
Low pH-exposed MSC enhance in vivo melanoma growth
To investigate whether an acidic microenvironment potentiates MSC stimulation in vivo growth of melanoma cells, we exposed human bone marrow-derived MSC to a pH 6.7 acidified medium. We know that extracellular pH of melanoma ranges frequently from 6.7 to 6.9 and MSC might be influenced by this alteration.Citation20 MSC growth in standard or in acidic medium were cultivated for 24 hours in a normoxic condition (21% O2) and standard glucose content. At the end of the incubation period, acidic and non-acidic MSC were collected from dishes and viability determined before their usage. Viability of MSC either acidic or non-acidic was >98%. Thus, mixtures of MSC and melanoma cells, low pH exposed MSC and melanoma cells or melanoma cells alone were injected into the subcutaneous tissues of SCID bg/bg immunodeficient mice. The growth kinetics of tumors from MSC exposed to low pH medium and melanoma cells was higher than that of tumors derived from MSC and melanoma cells or melanoma cells alone, along 24 days of observation (). We show in that acidic MSC medium accelerates the growth of melanoma cells in vitro, however in a manner not different from that of influenced by medium conditioned by MSC grown in standard pH media; this means that other mechanisms are involved in in vivo promoting effect of acidic MSC. Melanoma cells when grow in a medium conditioned by acidic MSC show a better resistance to a pro-apoptotic insult ().
Figure 1. Low pH-exposed MSC enhance in vivo and in vitro melanoma growth. (A) Tumor growth of SCID bg/bg mice inoculated with melanoma cells alone or together with MSC grown in standard or acidic medium. (B) Cell growth of A375M6 human melanoma cells treated with medium conditioned by MSC previously grown in standard pH medium (SpH-MSC A375M6) or by MSC grown in low pH medium (LpH-MSC A375M6). (C) Western blot analysis of PARP expression in A375M6 cells grown in media conditioned by acidic or non acidic MSC and treated with H2O2. Bar graph derived from the ratio between Cleaved Parp and Parp normalized to the intensity of β-Tubulin bands. Values presented are means ±SEM of 3 independent experiments. Asterisk indicates p < 0.05.
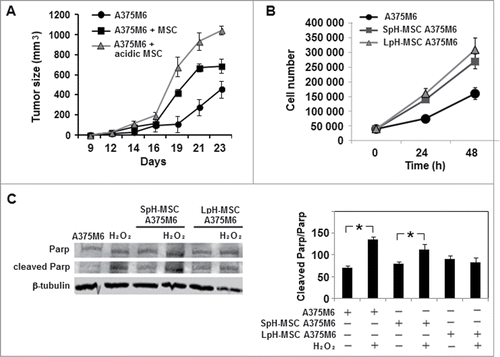
EMT-like profile of human melanoma cells grown in a medium conditioned by acidic MSC
Considering the high tumorigenic activity of acidic MSC, we incubated melanoma cells in media conditioned by MSC previously grown in low pH medium (LpH-MSC medium) to verify the profile acquired by LpH-MSC medium-stimulated melanoma cells. We have found that melanoma cells grown for 24 hours in LpH-MSC medium express a spindle and dendritic shape similar of melanoma cells grown in media conditioned by MSC grown in standard pH medium (SpH-MSC medium), but different from control cells (). A clear change of classical EMT markers, such as N-cadherin and SNAIL up-regulation and E-cadherin down-regulation are more pronounced in cells exposed to LpH-MSC medium (). Moreover, melanoma cells grown in LpH-MSC medium express a reduced amount of IkB, suggesting a stimulation of NF-kB (). We found that melanoma cells exposed to LpH-MSC medium do not express a higher invasion capacity through Matrigel-coated filters (), although these cells express a higher motility determined in the absence of Matrigel (). Only when we suspended melanoma cells in LpH-MSC conditioned medium, they expressed a higher MMP-dependent ability to invade Matrigel-coated filters than cells suspended in SpH-MSC medium or standard medium, as revealed by Ilomastast, a broad inhibitor of metalloproteases, added to MSC-conditioned media (). LpH-MSC medium, in our experimental protocol, works as chemotactic agent as well as SpH-MSC medium, probably due to the expression of the stromal cell-derived factor 1 (SDF-1) that reaches the same elevated expression levels in acidic or non acidic MSC. Cells grown in LpH-MSC medium are the most attracted () to the conditioned media and it might be related to the high expression of CXCR4, the SDF1 receptor, in these cells ().
Melanoma cells grown in LpH-MSC medium express as a further mesenchymal aspect of their profile an increased level of TGFβ, TGFR1 and TGFR2 than cells exposed to SpH-MSC medium (). It is possible that an upregulation of TGFβ family members will contribute in maintaining in melanoma cells exposed to LpH-MSC medium traits of a mesenchymal phenotype. Melanoma cells used in our experiments, when they were stimulated by exogenous TGFβ showed an increase of MMP-dependent invasiveness abrogated by Ilomastast, higher levels of N-cadherin and Snail transcription factor, a reduced level of E-cadherin and an up-regulation of TGFβ mRNA ().
Figure 3. Expression levels of TGFβ and TGFβ receptors in melanoma cells grown in medium conditioned by acidic MSC. qPCR analysis of (A) TGFβ, TGFR1, TGFR2, TGFR3 in melanoma cells treated with conditioned media of MSC grown at low pH. and (B) TGFβ and EMT markers in melanoma cells treated for 24 hours with TGFβ 10 ng/ml. (C) Invasiveness of A375M6 cells treated with TGFβ 10 ng/ml, in the presence or absence of Ilomastat and performed using Matrigel-coated filters. Migration is measured as a percentage of the control. Values presented are means ± SEM of 3 independent experiments. Asterisk indicates p < 0.05
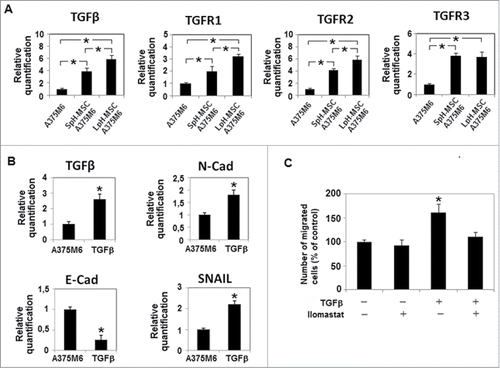
Metabolic symbiosis between acidic MSC and melanoma cells exposed to media conditioned by acidic MSC themselves.
We investigated the metabolism of acidic MSC and conditioned melanoma cells, in order to disclose a possible stromal-neuroepithelial metabolic coupling in acidic tumor microenvironment. We found that MSC, when exposed to an acidic medium, they change their metabolic profile down-regulating glucose transporter 1 and 3 (GLUT1 and 3) expression and up-regulating ratio between monocarboxylate transporter 1 and 4 (MCT1 and 4) (). High level of MCT1 promotes lactate upload whereas reduction of GLUT 1 and 3 reduces glucose uptake, meaning that acidic MSC potentially switch from glycolysis to OxPhos, a change also suggested by reduction of lactate dehydrogenase A (LDH-A) and PKM2 expression (). Reduction of c-Myc and p-Akt transcription factors are additional indicators of a reduction in glycolysis pathway (). In parallel, melanoma cells grown in LpH-MSC medium show an upregulation of GLUT1 and specially GLUT3, and a reduction of MCT1 associated with an increased level of MCT4 (). GLUT3 is designed as the neuronal GLUT and has both a higher affinity for glucose and at least a fivefold greater transport capacity than GLUT 1,2,4. LDH-A also increases in melanoma cells exposed to LpH-MSC medium, as well as p-Akt transcription factor (). Thus, melanoma cells conditioned by LpH-MSC medium may generate lactate and H+ functional for LpH-MSC underwent oxidative phosphorylation.
Figure 4. Metabolic profile of acidic MSC. (A) Quantitative real-time PCR of GLUT1, GLUT3, PKM2, LDHA and c-Myc and (B) Western blot analysis of AKT, p-AKT, MCT1 and MCT4 expression in MSC grown in acidic medium for 24 hours. Each band of western blot was quantified by densitometric analysis and the corresponding histogram was constructed as relative to β-tubulin. Values presented are means ±SEM of 3 independent experiments. Asterisk indicates p < 0.05.
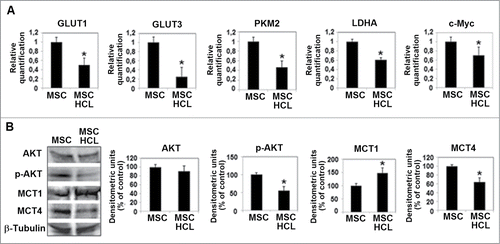
Figure 5. Media conditioned by acidic MSC change the metabolic profile of A375M6 melanoma cells. (A) Quantitative real-time PCR of GLUT1, GLUT3, LDHA and c-Myc and (B) Western blot analysis of AKT, p-AKT, MCT1 and MCT4 expression in melanoma cells grown for 24 hours in media conditioned by non acidic MSC (SpH-MSC A375M6) or acidic MSC (LpH-MSC A375M6). Each band of western blot was quantified by densitometric analysis and the corresponding histogram was constructed as relative to β-tubulin. Values presented are means ±SEM of 3 independent experiments. Asterisk indicates p < 0.05.
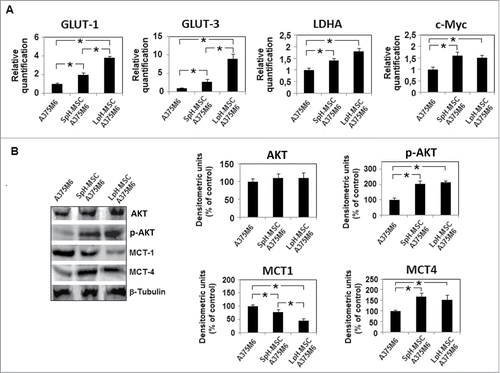
A nowadays emerging inhibitor of mithocondrial respiratory chain is metformin, the most widely used antidiabetic drug that belongs to the Biguanide class. When we added metformin (1mM, a dose used in diabetic patients) to the acidic medium used to grow MSC, MSC recovered high levels of GLUT1, 3 and rather unexpectedly level of TGFβ decreased dramatically even lower than that expressed by MSC grown in standard pH medium (). These findings lead to investigate GLUT expression in melanoma cells exposed to LpH- metformin-treated MSC medium; this investigation reveals a significant reduction of both type of GLUT associated with a recovered E-cadherin expression balanced by a significant diminution of N-cadherin (), almost indicating a mesenchymal-to-epithelial transition (the so-called MET program). Melanoma cells exposed to LpH-metformin-treated MSC medium also showed a significant reduction of invasiveness ().
Figure 6. Metformin acts on MSC metabolism and abolishes the effects of acidic MSC conditioned medium on melanoma cells. MSC were cultured in acidic medium in the presence of metformin 1mM for 24 hours. (A) Evaluation by quantitative real-time PCR of metformin effects on GLUT1, GLUT3 and (B) TGFβ mRNA expression in acidic and non acidic MSC. After 24 hours, melanoma cells were grown in media conditioned by acidic MSC treated with metformin (LpH-metf-MSC A375M6). (C) Quantitative mRNA analysis of EMT-related genes N-Cadherin, Snail, E-Cadherin and (D) GLUT-1 and GLUT-3 in melanoma cells grown in conditioned media conditioned. (E) Cell invasiveness across Matrigel-coated porous menmbranes of Boyden chambers determined in A375M6 cells grown in conditioned media. Migration is measured as a percentage of the control. Data are expressed as means ±SEM of 3 independent experiments. Asterisk indicates p < 0.05.
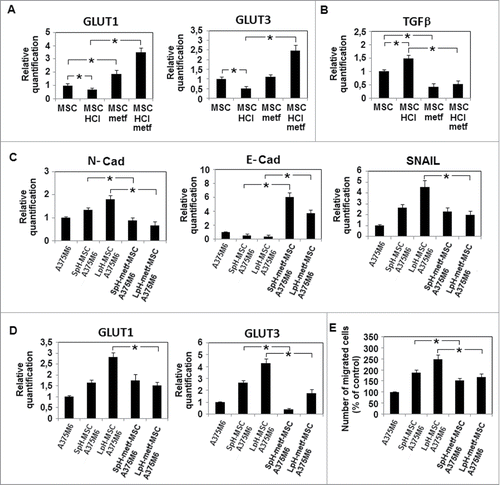
TGFβ released by acidic MSC drives EMT-like profile of melanoma cells exposed to LpH-MSC medium and esomeprazole abolishes it.
Low pH-exposed MSC express a very high amount of TGFβ, more than MSC (see: TGFβ determination by Western blotting in , top panel) and when we silenced TGFβ in these cells (, bottom panel), LpH-MSC medium-exposed melanoma cells re-gained an E-cadherin/N-cadherin ratio comparable to unconditioned cells, and TGFβ and SNAIL1 transcription factor reduced significantly (). In parallel, LpH-TGFβ-silenced MSC medium does not promote motility and MMP-dependent invasiveness (). Silencing experiments reveal that TGFβ from acidic MSC is also a promoter of both GLUT1,3 expression (). p17 peptide, a molecular agent able to recognize and inhibit TGFβR3,Citation21 is used to confirm TGFβ−induced EMT-like profile of melanoma cells grown in LpH-MSC medium. shows that p17 suppresses TGFβ and GLUT 1,3 expression and reverts E-Cadherin/N-Cadherin ratio in LpH-MSC conditioned melanoma cells.
Figure 7. TGFβ−released by acidic MSC drives EMT-like profile of melanoma cells exposed to LpH-MSC medium. MSC were transfected with specific TGFβ siRNAs and treated with acidic medium for 24 hours. (A) Western blot analysis of TGFβ expression in acidic MSC (top panel) and quantitative real-time PCR of TGFβ mRNA expression in MSC after gene silencing (bottom panel). After 24 hours, melanoma cells were grown in media conditioned by acidic MSC silenced for TGFβ. (LpH-si-MSC A375M6) and quantitative real time PCR was used to evaluate the expression of TGFβ (B), genes related to EMT (C) and metabolism (D).18s rRNA was used as reference gene. (E) Invasiveness of A375M6 cells grown in conditioned media, performed using Matrigel-coated filters. Migration is measured as a percentage of the control. (F) Wound healing assay of melanoma cells treated with media conditioned by silenced MSC grown at low pH. The wound closure was evaluated 18 hours since its creation. (G) Quantitative real time PCR was used to evaluate the expression of TGFβ, genes related to EMT and GLUT1 and GLUT3, in melanoma cells grown in media conditioned by acidic MSC and treated with p17, a peptide inhibitor of TGF-β1. Data are expressed as means ±SEM of 3 independent experiments. Asterisk indicates p < 0.05.
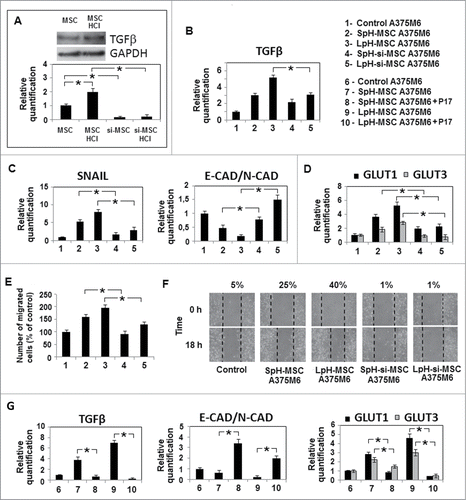
According to our experience on using esomeprazole, a proton pump inhibitor able to inhibit NF-kB in acidic melanoma cells,Citation22 we treated acidic MSC with esomeprazole and consequently abrogated TGFβ mRNA expression (). Exposure of melanoma cells to LpH-esomeprazole-treated MSC medium decreases TGFβ and SNAIL1 mRNA expression, motility and invasiveness and restores E-Cadherin/N-Cadherin ratio ().
Figure 8. Esomeprazole inhibits the aggressiveness of melanoma cells conditioned by acidic MSC. MSC were cultured in acidic medium in the presence of esomeprazole 100μM for 24 hours. (A) Quantitative real-time PCR of TGFβ mRNA expression in MSC. After 24 hours, melanoma cells were grown in media conditioned by acidic MSC treated with esomeprazole (LpH-eso-MSC A375M6) and quantitative real time PCR was used to evaluate the expression of TGFβ (B), genes related to EMT (C) and metabolism (D).18s rRNA was used as reference gene. (E) Inhibition by esomeprazole of invasiveness, performed resuspending melanoma cells in MSC conditioned media, and motility of A375M6 grown in LpH-eso-MSC medium. Migration is measured as a percentage of the control. Data are expressed as means ± SEM of 3 independent experiments. Asterisk indicates p < 0.05.
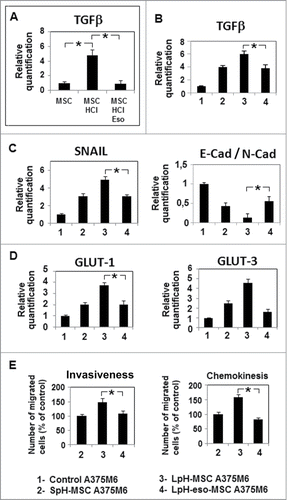
Discussion
The result of both aerobic and anaerobic metabolism of tumor cells is a developing extracellular acidosis, which represents a re-appreciated alteration of tumor microenvironment. This study investigates role of extracellular acidosis in the complex scenario of cross talk among MSC and melanoma cells. The external pH of human melanoma has been reported to be within the range of 6.4 to 7.3,Citation20,23 low enough to influence behavior of several inflammatory host cells of tumor “reactive” stroma, including MSC. We found that MSC exposed to an acidic pH medium and co-injected with melanoma cells into immunodeficient animals are able to promote growth rate of melanoma xenografts higher than MSC grown in standard pH media. The promoted tumorigenesis expressed by melanoma cells co-injected with acidic MSC correlates with a mesenchymal-like phenotype compatible with an EMT program acquired by melanoma cells exposed to LpH-MSC medium. A clear elongated morphology, resistance to apoptosis and reduction in E-cadherin/N-cadherin ratio characterize melanoma cells exposed to LpH-MSC medium. The higher resistance to apoptosis of melanoma cells exposed to LpH-MSC medium might be ascribed to a stimulated Akt activity.Citation24 It is known that melanoma cells transfected with N-cadherin acquire a fibroblast-like shape Citation25 and N-cadherin acting as an oncogene promotes malignancy.Citation26 In addition, Alonso et al. showed that N-cadherin expression promotes in human melanoma cells metastasis.Citation27 Evaluation of IkB revealed a strong increase of NF-kB expression in melanoma cells grown in LpH-MSC medium, a finding that we also described in a previous paper demonstrating that acidity triggers in melanoma cells NF-kB activation, and silencing NF-kB, we may inhibit invasiveness and N-cadherin up-regulation.Citation28 NF-kB and melanoma progression is reviewed by Amiri and Richmond.Citation29 Elevation of NF-kB expression in melanoma cells grown in LpH-MSC medium is potentially involved in several aspects of the acquired phenotype, such as stabilization of Snail transcription factor,Citation30 loss of E-Cadherin expression,Citation31 attenuation of TGFβ-induced inhibition of proliferationCitation32 and, finally, metabolism.Citation33 Thereafter, these cells express an increased motility and invasiveness after suspension in MMP-enriched LpH-MSC medium. It is known that exposure to low pH medium stimulates MMP-9 expression trough NF-kB signaling in melanoma cells.Citation28,34 Members of TGFβ family are also considered markers of EMT. We found that melanoma cells exposed to LpH-MSC medium express increased levels of TGFβ and TGFβRI and RII, which means that the mesenchymal phenotype acquired by melanoma cells can be maintained by an autocrine loop. TGFβR2 is considered an absolute requirement for TGFβ-mediated EMT program.Citation35
Crosstalk among MSC and melanoma cells in low pH condition is also characterized and, possibly, sustained by an elicited metabolic symbiosis. This symbiosis is based on OxPhos metabolism expressed by acidic MSC and a forced glycolytic metabolism of melanoma cells grown in LpH-MSC medium. We may speculate whether pH becomes low enough to influence metabolism of tumor cells, MSC switch to OxPhos in order to remove lactic acid and H+ from the microenvironment, attenuating acidosis and maintaining a glycolysis-dependent proliferative activity in tumor cells. Evidence indicates that tumor cells prevent cytosolic acidification by activating a number of transporters that extrude the protons and lactate produced during glycolysis, because an acidic intracellular pH may drive a metabolic switch from aerobic glycolysis to OxPhos.Citation36 An enhanced Warburg effect in lung adenocarcinoma cells conditioned by MSC making tumor cells resistant to radical oxygen species was reported.Citation37 Our observation extends the metabolic symbiosis scenario between hypoxic and oxygenated tumor cells and between tumor cells and stromal cells. Sonveaux et al.'s study demonstrates that lactate secreted by hypoxic tumor cells is taken up through MCT1 by normoxic tumor cells that utilize it for OxPhos.Citation38 Then, it was demonstrated that stromal cells may perform aerobic glycolysis under the influence of tumor cells and donate lactate to feed OxPhos metabolism of tumor cells, the so-called “Reverse Warburg Effect.”Citation39-41
Metformin, a drug that is found to be effective in tumors,Citation42-45 when it was added in acidic medium, which will be used for MSC conditioning, blocks TGFβ expression and reverts inhibition of GLUT 1 and 3 elicited by acidic medium in MSC. Moreover, melanoma cells conditioned by LpH-metformin-treated MSC medium exhibited a reconstituted epithelial-like profile and a reduced invasion, that were associated with a reduction of GLUT 1, 3.
All changes that we found in melanoma cells exposed to LpH-MSC medium, including mesenchymal phenotype and metabolic adaptation, could be ascribed to TGFβ secreted by acidic MSC into their growth medium and use of p17 peptide, a TGFβR3 inhibitor, and silencing experiments proved that. Both treatments revert EMT-like profile and decrease GLUT expression in melanoma cells. We cannot exclude that other inflammatory cytokines secreted by MSC, such as TNFα, might potentiate TGFβ effets.Citation46 Numerous reports underlined that through TGFβ secretion MSC promote a malignant phenotype in tumor cells of different histotype Citation10,21 The higher level of TGFβ secreted by acidic MSC does not prevent proliferation of LpH-MSC-adapted melanoma cells, a condition that might be related to SMAD/NF-kB signaling interplays, as suggested by Freudlsperger et al.'s findings in head and neck cancers.Citation32 We also found that esomeprazole, a proton pump inhibitor activated by acidic medium, inhibits TGFβ of acidic MSC, which corresponds to a MET program in melanoma cells grown in LpH-esomeprazole-treated MSC medium. These cells were characterized by a reduction of N-cadherin, TGFβ, invasiveness and motility, and elevation of E-cadherin. Graham RM et al. show that the inhibition of the vacuolar ATPase induces a reduction in tumor burden and metastasis in breast cancer.Citation47
Overall, our results stress that acidosis of tumor microenvironment potentiates the pro-tumoral activity of MSC, representing a reasonable target for melanoma.
Material and Methods
Cell lines and culture conditions
Mesenchymal Stem Cells (MSC) obtained from bone marrow aspirates of donors, which signed informed consent, were expanded in Dulbecco's modified Eagle's medium with low glucose, (DMEM 1000; Gibco, Life Technologies, MB, Italy), supplemented with foetal bovine serum (FBS, Boerhinger Mannheim, Germany) 10% and incubated at 37°C in humidified atmosphere containing 95% air and 5% CO2.
The melanoma cell line A375M6 was isolated in our laboratory from lung metastasis of SCID bg/bg mice i.v. injected human melanoma A375P line, obtained from American Type Culture Collection (ATCC, Rockville, MD). Melanoma cells were cultivated in Dulbecco's Modified Eagle Medium high glucose (DMEM 4500, Gibco) supplemented with 10% FBS, at 37°C in a 10% CO2 humidified atmosphere.
Cells were harvested from subconfluent cultures by incubation with a trypsin-EDTA solution (Life Technologies), and propagated every 3 days. Viability of the cells was determined by trypan blue exclusion test. Cultures were periodically monitored for mycoplasma contamination using Chen's fluorochrome test.Citation48
Acidic treatment
Chemical acidified medium was obtained by the addition of HCl 1N in DMEM 1000 10%FCS, pH value was monitored by a pHmeter (Orion PH Meter 520A-1). As pH value was stable (6,6-6,7), acidified medium was added to cell cultures and the seal cap was tightly closed. pH was evaluated also at the end of each experiment. Protein content of MSC at various pH was determined and found not different. For inhibition assays, metformin (Sigma-Aldrich, St. Louis, Missouri) 1mM or esomeprazole (AstraZeneca, Sweden) 100 μM were added to cell cultures 1h before acidic treatment.
Preparation of conditioned medium by MSC
MSC were seeded at density of 1×105 cell/ml in complete media in T-25 tissue culture flasks and grown to 70% of confluence. Cells were treated with acidic medium for 24 hours and then washed twice with PBS and incubated in DMEM 4500, 2% FCS medium for 24 hours. Conditioned media were collected and filtered with a 0.22 μm filter and tumor cells were incubated in MSC conditioned media for 24 hours. In some experiments, conditioned medium was prepared using MSC pretreated with siRNA targeting human TGFβ and negative contructs obteined from Riboxx Life Science/Euroclone, Milano, Italy (IBONI siRNA-pool). siRNA constructs were incorporated into RiboxxFECT transfection reagent according to the manifacturer's reccomendations and transfections were performed 24 hours before acidic treatment. Moreover, to target TGFβ in media conditioned by MSC, TGFβR3 antagonist peptide p17 (Digna Biotech, Pamplona, Spain) was used at the final concentration of 100 μg/ml.
Western blotting analysis
Cells were washed with ice cold PBS containing 1 mM Na4VO3, and lysed in 100 μl of cell lysis buffer containing: 75 mM Tris–HCl (pH 7.4), 9 M Urea, 0.15 M β-mercaptoethanol. Aliquots of supernatants containing equal amounts of protein (65 μg) in Laemmli buffer were separated on 8% (v/v) SDS–PAGE gel. Fractionated proteins were transferred from the gel to a PVDF nitrocellulose membrane using an electroblotting apparatus (Bio-Rad, Segrate, MI, Italy). Blots were stained with Ponceau red to ensure equal loading and complete transfer of proteins, then they were blocked for 2 hours, at room temperature, with Odyssey blocking buffer (Dasit Science, Cornaredo, MI, Italy). Subsequently, the membrane was probed at 4°C overnight with primary antibodies diluited in a solution of 1:1 Odyssey blocking buffer/T-PBS buffer. The primary antibodies were: rabbit anti-PARP, rabbit anti-Akt, rabbit anti-P-Akt (Cell Signaling Technology, Danvers, MA, USA), rabbit anti-N-Cadherin (Biorbyt, Cambridge, UK), rabbit anti- E-Cadherin, rabbit anti-IkB, rabbit anti-MCT-1, rabbit anti-MCT-4 (Santa Cruz Biotechnology, Santa Cruz, California). The membrane was washed in T-PBS buffer, incubated for 1 hour at room temperature with goat anti-rabbit IgG Alexa Flour 680 antibodies (Invitrogen, Monza, Italy), and then visualized by an Odyssey Infrared Imaging System (LI-COR® Bioscience). Mouse anti-α-tubulin monoclonal antibody (Sigma, Saint Louis, MO, USA) was used to assess equal amount of protein loaded in each lane.
RNA isolation, semiquantitative PCR and quantitative PCR (qPCR)
Total RNA was extracted from cells by using TRI Reagent (Sigma). The amount and purity of RNA were determined spectrophotometrically. cDNA synthesis was obtained by incubating 2 μg of total RNA with 4 U/μl of M-MLV reverse transcriptase (Promega, San Luis Obispo, California) according to the manufacturer's instructions.
mRNA levels of CXCR4 and SDF-1 were determined by an internal-based semiquantitative RT–PCR. Aliquots of 2 μl of the cDNA mixture were used for PCR amplification. The end-point PCR reactions were carried out in 50 μl of a solution containing specific primers and 0.1 U/μl of GoTaq Polymerase (Promega), using a Perkin-Elmer Thermal cycler. Aliquots of 10 μl of each PCR mixture were applied to a 2% agarose gel, electrophoresed and visualized. cDNA products were evaluated on the basis of standard PCR markers; each band was quantified by densitometric analysis and the corresponding histogram was constructed as relative to GAPDH.
Quantitative real time PCR (qPCR) was performed using the GoTaq® Probe Systems (Promega). The qPCR analysis was carried out in triplicate using an Applied Biosystems 7500 Sequence Detector with the default PCR setting: 40 cycles of 95° for 15 seconds and 60°C for 60 seconds. mRNA was quantified with the ΔΔCt method as described.Citation49 mRNA levels were normalized to 18S as an endogenous control. Primer sequences are reported in .
Table 1. Primer sequences for PCR
Invasion assay
Invasiveness of A375M6 melanoma cells grown for 24 hours in media conditioned by acidic or non-acidic MSC, was determined in vitro on Matrigel-coated polycarbonate filters, with 8 µm pore size, 6.5 mm diameter, mounted in Boyden's chambers. 1,5×105 cells (200 µL), were seeded in the upper compartment and incubated for 6 hours at 37°C in 10% CO2 in air. In some experiments, invasion was performed in the presence of a MMPs inhibitor Ilomastat (Millipore, Billerica, Massachussets). After incubation, cells on the upper side of the filters were wiped off mechanically using cotton swabs and the membranes were fixed overnight in ice-cold methanol. Cells on the lower side of the membranes were stained with Diff Quick solutions and counted.
Motility assay
Motility of A375M6 melanoma cells was determined in vitro on polycarbonate filters, with 8 µm pore size, 6.5 mm diameter, mounted in Boyden's chambers. 1,5×105 cells, re-suspended in 200 µL of DMEM 2% FBS, were seeded in the upper compartment and incubated for 6 hours at 37°C in 10% CO2 in air. 400 μl of DMEM supplemented with 10% FBS or of media conditioned by mesenchymal stem cells were added in the lower compartment as attractant. After incubation, cells on the upper side of the filters were wiped off mechanically using cotton swabs and cells on the lower side of the insert membrane were fixed overnight in ice-cold methanol. Cells were stained with Diff Quick solutions, washed and counted.
Wound healing assay
Cell migration was also evaluated by an in vitro wound healing assay. Cells were grown at 80-90% confluence in 35 mm dishes; the cell layer was wounded with a sterile 200 ml pipette tip and incubated in 1% FBS colture medium for 24 hours. The wound was observed and photographed using phase contrast microscopy.
In vivo experiments
Investigation has been conducted in accordance with national guidelines and has been approved by the ethics committee of the Animal Welfare Office of the ItalianWork Ministry and conformed to the legal mandates and Italian guidelines for the care and maintenance of laboratory animals. Eight-week-old female SCID-bg/bg mice (Charles River Laboratories, Lecco, Italy) were injected subcutaneously with 200 μl of PBS containing 1×106 melanoma cells alone or 1×106 melanoma cells mixed with 0,5×106 acidic or non acidic MSC (4 animals/group). Tumor development was monitored at regular intervals by measuring tumor volume determined by the formula (L×W2)/2, where L and W are the length and width of tumor mass.
Statistical analysis
Densitometric data are expressed as means ± standard errors of the mean (SEM) depicted by vertical bars from representative experiment of at least 3 independent experiments. Statistical analysis of the data was performed by Student's t-test, and p ≤ 0.05 was considered statistically significant.
Disclosure of Potential Conflicts of Interest
No potential conflicts of interest were disclosed.
Funding
This study was financially supported by grants from Istituto Toscano Tumori and Ente Cassa di Risparmio di Firenze.
References
- Chamberlain G, Fox J, Ashton B, Middleton J. Concise review: mesenchymal stem cells: their phenotype, differentiation capacity, immunological features, and potential for homing. Stem Cells 2007; 25:2739-49; PMID:17656645; http://dx.doi.org/10.1634/stemcells.2007-0197
- Kumar S, Chanda D, Ponnazhagan S. Therapeutic potential of genetically modified mesenchymal stem cells. Gene Ther 2008; 15:711-5; PMID:18356815; http://dx.doi.org/10.1038/gt.2008.35
- Cuiffo BG, Karnoub AE. Mesenchymal stem cells in tumor development: emerging roles and concepts. Cell Adh Migr 2012; 6:220-30; PMID:22863739; http://dx.doi.org/10.4161/cam.20875
- Goldstein RH, Reagan MR, Anderson K, Kaplan DL, Rosenblatt M. Human bone marrow-derived MSCs can home to orthotopic breast cancer tumors and promote bone metastasis. Cancer Res 2010; 70:10044-50; PMID:21159629; http://dx.doi.org/10.1158/0008-5472.CAN-10-1254
- Khakoo AY, Pati S, Anderson SA, Reid W, Elshal MF, Rovira II, Nguyen AT, Malide D, Combs CA, Hall G et al Human mesenchymal stem cells exert potent antitumorigenic effects in a model of Kaposi's sarcoma. J Exp Med 2006; 203:1235-47; PMID:16636132; http://dx.doi.org/10.1084/jem.20051921
- Zhu Y, Sun Z, Han Q, Liao L, Wang J, Bian C, Li J, Yan X, Liu Y, Shao C, Zhao RC. Human mesenchymal stem cells inhibit cancer cell proliferation by secreting DKK-1. Leukemia 2009; 23:925-33; PMID: 19148141; http://dx.doi.org/10.1038/leu.2008.384
- Zhu W, Xu W, Jiang R, Qian H, Chen M, Hu J, Cao W, Han C, Chen Y Mesenchymal stem cells derived from bone marrow favor tumor cell growth in vivo. Exp Mol Pathol 2006; 80:267-74; PMID:16214129; http://dx.doi.org/10.1016/j.yexmp.2005.07.004
- Barcellos-de-Souza P, Gori V, Bambi F, Chiarugi P. Tumor microenvironment: bone marrow-mesenchymal stem cells as key players. Biochim Biophys Acta 2013; 1836:321-35; PMID:24183942
- Karnoub AE, Dash AB, Vo AP, Sullivan A, Brooks MW, Bell GW, Richardson AL, Polyak K, Tubo R, Weinberg RA. Mesenchymal stem cells within tumour stroma promote breast cancer metastasis. Nature 2007; 449:557-63; PMID:17914389; http://dx.doi.org/10.1038/nature06188
- Mele V, Muraro MG, Calabrese D, Pfaff D, Amatruda N, Amicarella F, Kvinlaug B, Bocelli-Tyndall C, Martin I, Resink TJ, et al. Mesenchymal stromal cells induce epithelial-to-mesenchymal transition in human colorectal cancer cells through the expression of surface-bound TGF-β. Int J Cancer 2014; 134:2583-94; PMID:24214914; http://dx.doi.org/10.1002/ijc.28598
- Ye H, Cheng J, Tang Y, Liu Z, Xu C, Liu Y, Sun Y. Human bone marrow-derived mesenchymal stem cells produced TGFbeta contributes to progression and metastasis of prostate cancer. Cancer Invest 2012; 30:513-8; PMID:22646310; http://dx.doi.org/10.3109/07357907.2012.692171
- Peppicelli S, Bianchini F, Calorini L. Extracellular acidity, a “reappreciated” trait of tumor environment driving malignancy: perspectives in diagnosis and therapy. Cancer Metastasis Rev 2014; 33:823-32; PMID:24984804; http://dx.doi.org/10.1007/s10555-014-9506-4
- Fais S, Venturi G, Gatenby B. Microenvironmental acidosis in carcinogenesis and metastases: new strategies in prevention and therapy. Cancer Metastasis Rev 2014; 33:1095-108; PMID:25376898; http://dx.doi.org/10.1007/s10555-014-9531-3
- Gupta SC, Singh R, Pochampally R, Watabe K, Mo YY. Acidosis promotes invasiveness of breast cancer cells through ROS-AKT-NF-κB pathway. Oncotarget 2014; 5:12070-82; PMID:25504433
- Vander Heiden MG, Cantley LC, Thompson CB. Understanding the Warburg effect: the metabolic requirements of cell proliferation. Science 2009; 324:1029-33; PMID:19460998; http://dx.doi.org/10.1126/science.1160809
- Gatenby RA, Gillies RJ. Why do cancers have high aerobic glycolysis? Nat Rev Cancer 2004; 4:891-9; PMID:15516961; http://dx.doi.org/10.1038/nrc1478
- Pouysségur J, Dayan F, Mazure NM. Hypoxia signalling in cancer and approaches to enforce tumour regression. Nature 2006; 441:437-43; PMID:Can't; http://dx.doi.org/10.1038/nature04871
- Denko NC. Hypoxia, HIF1 and glucose metabolism in the solid tumour. Nat Rev Cancer 2008; 8:705-13; PMID:19143055; http://dx.doi.org/10.1038/nrc2468
- Reshetnyak YK, Yao L, Zheng S, Kuznetsov S, Engelman DM, Andreev OA. Measuring Tumor Aggressiveness and Targeting Metastatic Lesions with Fluorescent pHLIP. Molecular Imaging and Biology: MIB: the Official Publication of the Academy of Molecular Imaging 2011; 13:1146-56; http://dx.doi.org/10.1007/s11307-010-0457-z
- Wike-Hooley JL, Haveman J, Reinhold HS. The relevance of tumour pH to the treatment of malignant disease. Radiother Oncol 1984; 2:343-66; PMID:6097949; http://dx.doi.org/10.1016/S0167-8140(84)80077-8
- Laurenzana A, Biagioni A, Bianchini F, Peppicelli S, Chillà A, Margheri F, Luciani C, Pimpinelli N, Del Rosso M, Calorini L, et al. Inhibition of uPAR-TGFβ crosstalk blocks MSC-dependent EMT in melanoma cells. J Mol Med (Berl) 2015; 93(7):783-94; PMID:25694039; http://dx.doi.org/10.1007/s00109-015-1266-2
- Peppicelli S, Bianchini F, Contena C, Tombaccini D, Calorini L. Acidic pH via NF-κB favours VEGF-C expression in human melanoma cells. Clin Exp Metastasis 2013; 30:957-67; PMID:23784694; http://dx.doi.org/10.1007/s10585-013-9595-4
- Vaupel P, Kallinowski F, Okunieff P. Blood flow, oxygen and nutrient supply, and metabolic microenvironment of human tumors: a review. Cancer Res 1989; 49:6449-65; PMID:2684393
- Song G, Ouyang G, Bao S. The activation of Akt/PKB signaling pathway and cell survival. J Cell Mol Med. 2005 Jan-Mar; 9(1):59-71
- Krengel S, Grotelüschen F, Bartsch S, Tronnier M. Cadherin expression pattern in melanocytic tumors more likely depends on the melanocyte environment than on tumor cell progression. J Cutan Pathol 2004; 31:1-7; PMID:14675278; http://dx.doi.org/10.1046/j.0303-6987.2004.0106.x
- Hazan RB, Phillips GR, Qiao RF, Norton L, Aaronson SA. Exogenous expression of N-cadherin in breast cancer cells induces cell migration, invasion, and metastasis. J Cell Biol 2000; 148:779-90; PMID:10684258; http://dx.doi.org/10.1083/jcb.148.4.779
- Alonso SR, Tracey L, Ortiz P, Pérez-Gómez B, Palacios J, Pollán M, Linares J, Serrano S, Sáez-Castillo AI, Sánchez L, et al. A high-throughput study in melanoma identifies epithelial-mesenchymal transition as a major determinant of metastasis. Cancer Res 2007; 67:3450-60; PMID:17409456; http://dx.doi.org/10.1158/0008-5472.CAN-06-3481
- Peppicelli S, Bianchini F, Torre E, Calorini L. Contribution of acidic melanoma cells undergoing epithelial-to-mesenchymal transition to aggressiveness of non-acidic melanoma cells. Clin Exp Metastasis 2014; 31:423-33; PMID:24469963
- Amiri KI, Richmond A. Role of nuclear factor-kappa B in melanoma. Cancer Metastasis Rev 2005; 24:301-13; PMID:15986139; http://dx.doi.org/10.1007/s10555-005-1579-7
- Wu Y, Deng J, Rychahou PG, Qiu S, Evers BM, Zhou BP. Stabilization of snail by NF-kappaB is required for inflammation-induced cell migration and invasion. Cancer Cell 2009; 15:416-28; PMID:19411070; http://dx.doi.org/10.1016/j.ccr.2009.03.016
- Poser I, Domínguez D, de Herreros AG, Varnai A, Buettner R, Bosserhoff AK. Loss of E-cadherin expression in melanoma cells involves up-regulation of the transcriptional repressor Snail. J Biol Chem 2001; 276:24661-6; PMID:11323412; http://dx.doi.org/10.1074/jbc.M011224200
- Freudlsperger C, Bian Y, Contag Wise S, Burnett J, Coupar J, Yang X, Chen Z, Van Waes C. TGF-β and NF-κB signal pathway cross-talk is mediated through TAK1 and SMAD7 in a subset of head and neck cancers. Oncogene 2013; 32:1549-59; PMID:22641218; http://dx.doi.org/10.1038/onc.2012.171
- Mauro C, Leow SC, Anso E, Rocha S, Thotakura AK, Tornatore L, Moretti M, De Smaele E, Beg AA, Tergaonkar V, et al. NF-κB controls Energy homeostasis and metabolic adaptation by upregulating mitochondrial respiration. Nat Cell Biol 2011; 13:1272-9; PMID:21968997; http://dx.doi.org/10.1038/ncb2324
- Kato Y, Lambert CA, Colige AC, Mineur P, Noël A, Frankenne F, Foidart JM, Baba M, Hata R, Miyazaki K, et al. Acidic extracellular pH induces matrix metalloproteinase-9 expression in mouse metastatic melanoma cells through the phospholipase D-mitogen-activated protein kinase signaling. J Biol Chem 2005; 280:10938-44; PMID:15657063; http://dx.doi.org/10.1074/jbc.M411313200
- Han G, Lu SL, Li AG, He W, Corless CL, Kulesz-Martin M, Wang XJ. Distinct mechanisms of TGF-beta1-mediated epithelial-to-mesenchymal transition and metastasis during skin carcinogenesis. J Clin Invest 2005; 115:1714-23; PMID:15937546; http://dx.doi.org/10.1172/JCI24399
- Neri D, Supuran CT. Interfering with pH regulation in tumours as a therapeutic strategy. Nat Rev Drug Discov 2011; 10:767-77; PMID:21921921; http://dx.doi.org/10.1038/nrd3554
- Ohkouchi S, Block GJ, Katsha AM, Kanehira M, Ebina M, Kikuchi T, Saijo Y, Nukiwa T, Prockop DJ. Mesenchymal stromal cells protect cancer cells from ROS-induced apoptosis and enhance the Warburg effect by secreting STC1. Mol Ther 2012; 20:417-23; PMID:22146344; http://dx.doi.org/10.1038/mt.2011.259
- Sonveaux P, Végran F, Schroeder T, Wergin MC, Verrax J, Rabbani ZN, De Saedeleer CJ, Kennedy KM, Diepart C, Jordan BF, et al. Targeting lactate-fueled respiration selectively kills hypoxic tumor cells in mice. J Clin Invest 2008; 118:3930-42; PMID:19033663
- Pavlides S, Whitaker-Menezes D, Castello-Cros R, Flomenberg N, Witkiewicz AK, Frank PG, Casimiro MC, Wang C, Fortina P, Addya S, et al. The reverse Warburg effect: aerobic glycolysis in cancer associated fibroblasts and the tumor stroma. Cell Cycle 2009; 8:3984-4001; PMID:19923890; http://dx.doi.org/10.4161/cc.8.23.10238
- Fiaschi T, Marini A, Giannoni E, Taddei ML, Gandellini P, De Donatis A, Lanciotti M, Serni S, Cirri P, Chiarugi P. Reciprocal metabolic reprogramming through lactate shuttle coordinately influences tumor-stroma interplay. Cancer Res 2012; 72: 5130-40; PMID:22850421; http://dx.doi.org/10.1158/0008-5472.CAN-12-1949
- Bonuccelli G, Avnet S, Grisendi G, Salerno M, Granchi D, Dominici M, Kusuzaki K, Baldini N. Role of mesenchymal stem cells in osteosarcoma and metabolic reprogramming of tumor cells. Oncotarget 2014; 5:7575-88; PMID:25277190
- Cerezo M, Tichet M, Abbe P, Ohanna M, Lehraiki A, Rouaud F, Allegra M, Giacchero D, Bahadoran P, Bertolotto C, et al. Metformin blocks melanoma invasion and metastasis development in AMPK/p53-dependent manner. Mol Cancer Ther 2013; 12:1605-15; PMID:23741061; http://dx.doi.org/10.1158/1535-7163.MCT-12-1226-T
- Rattan R, Ali Fehmi R, Munkarah A. Metformin: an emerging new therapeutic option for targeting cancer stem cells and metastasis. J Oncol 2012; 2012:928127; PMID:22701483; http://dx.doi.org/10.1155/2012/928127
- Blandino G, Valerio M, Cioce M, Mori F, Casadei L, Pulito C, Sacconi A, Biagioni F, Cortese G, Galanti S, et al. Metformin elicits anticancer effects through the sequential modulation of DICER and c-MYC. Nat Commun 2012; 3:865; PMID:22643892; http://dx.doi.org/10.1038/ncomms1859
- Del Barco S, Vazquez-Martin A, Cufí S, Oliveras-Ferraros C, Bosch-Barrera J, Joven J, Martin-Castillo B, Menendez JA. Metformin: multi-faceted protection against cancer. Oncotarget 2011; 2:896-917; PMID:22203527
- Borthwick LA, Gardner A, De Soyza A, Mann DA, Fisher AJ. Transforming Growth Factor-β1 (TGF-β1) Driven Epithelial to Mesenchymal Transition (EMT) is Accentuated by Tumour Necrosis Factor α (TNFα) via Crosstalk Between the SMAD and NF-κB Pathways. Cancer Microenviron 2012; 5:45-57; PMID:21792635; http://dx.doi.org/10.1007/s12307-011-0080-9
- Graham RM, Thompson JW, Webster KA. Inhibition of the vacuolar ATPase induces Bnip3-dependent death of cancer cells and a reduction in tumor burden and metastasis. Oncotarget 2014; 5:1162-73; PMID:24811485
- Chen TR. In situ detection of mycoplasma contamination in cell cultures by fluorescent Hoechst 33258 stain. Exp Cell Res 1977; 104:255-62; PMID:65285; http://dx.doi.org/10.1016/0014-4827(77)90089-1
- Livak KJ, Schmittgen TD. Analysis of relative gene expression datausing real-time quantitativePCR andthe2; -Delta Delta C(T) Method. Methods 2001; 25:402-8; PMID:11846609; http://dx.doi.org/10.1006/meth.2001.1262