Abstract
Chronic obstructive pulmonary disease (COPD) is smoking-related and associated with increased cytotoxic CD8+ T-cells in the airway. There is a wide range of susceptibility to the damaging effects of cigarette smoke with only a small proportion of smokers progressing to COPD. We have previously reported increased intracellular Th1 cytokines in blood, BAL and intraepithelial CD8+T cells in current and ex-smokers with COPD, whereas healthy smokers showed localized Th1 response in the lung only. We thus hypothesised that Th1-associated chemokines or their receptors on CD8+T-cells may be differentially expressed in the blood of healthy smokers, current smoker COPD subjects and those who had ceased smoking. We investigated chemokines, chemokine receptors and Th1 and cytotoxic T-cell markers in blood and BAL using flow cytometry, ELISA and cytometric bead array. In blood, CXCR3, CCR4, intracellular CCR3 and the Th1 marker 62L−CD45RO+ were increased in both COPD groups and healthy smokers. In contrast, cytotoxic T-cells, ITAC, MIG, IFN-γ and CCR5 were increased in both COPD groups but not smokers. In BAL, the Th1 marker 62L−CD45RO+, CCR5, CXCR3, IFN-γ, RANTES, IL-8, MCP-1, MIG and ITAC were increased in both COPD groups and smokers versus controls. Our findings are consistent with systemic inflammation in COPD associated with an increased influx of cytotoxic and Th1 cells into the airway. The differential expression of specific chemokines and their receptors in blood from COPD subjects and healthy smokers suggests that inclusion of these markers in any panel designed for the non-invasive investigation of smokers with a disposition to COPD would be clinically relevant.
INTRODUCTION
Chronic obstructive pulmonary disease (COPD) is currently the fourth leading cause of death in the world and is predicted to become the third most common cause of death and the fifth greatest cause of chronic disability in the world by the year 2020 (Citation[1]). Treatment options for COPD are limited and there are currently no therapies that completely halt disease progression (Citation[2]).
Recent studies by us and others have shown persistent abnormalities in inflammatory mediators even in COPD patients who have ceased smoking (Citation[3], Citation[4]). The reason for this remains unknown although elevated numbers of macrophages and CD8+ T-cells in the airways are thought to be central to the persistent inflammation. COPD is associated with increased numbers of cytotoxic CD8+ T-cells in both the airway and peripheral blood (Citation[4], Citation[5]).
Although the role of these cells in the pathogenesis of COPD is poorly understood, increased numbers of activated T-cells in the alveolar wall have been shown to correlate with the extent of emphysema (Citation[6]) and increased expression of granzyme b by CD8+ T-cells has been directly associated with airway epithelial cell apoptosis in COPD (Citation[7]). We have also recently shown a negative correlation between FEV1 and the percentage of BAL and intra-epithelial CD8+ T-cells (from bronchial brushings) producing TNF-α (Citation[4]). Chemokines and their receptors are considered to play an important role in regulating the migration of immune cells, including CD8+ T-cells, from the peripheral blood into inflamed tissue, such as the lung (Citation[8]). There have been reports of elevated isolated chemokines in bronchoalveolar lavage fluid (BAL) and sputum from patients with COPD (Citation[9], Citation[10], Citation[11], Citation[12]). We and others have suggested that COPD is associated with a Th1 T-cell response with increased intracellular T-cell production of Th1 cytokines (Citation[4]) and lung tissue T-cell expression of Th1 markers CCR5 and CXCR3 (Citation[13], Citation[14]). However, the effects of smoking status on Th1-associated chemokines and their receptors in the BAL and blood from subjects with COPD have not been studied. We hypothesised that increased levels of Th1-associated chemokines and their receptors in peripheral blood potentially lead to the influx of cytotoxic and Th1 T-cells that we have previously reported in the BAL of current and ex-smokers with COPD.
Although smoking is the main risk factor for developing COPD, there is a very wide range of susceptibility to the damaging effects of smoke, with some heavy smokers having minimal physiological dysfunction. It would thus be beneficial to identify smokers with a susceptibility to COPD, so that the disease is diagnosed or treated before extensive permanent damage occurs. We have previously reported increased intracellular Th1 cytokines in blood, BAL and intraepithelial CD8+T cells in COPD, whereas healthy smokers showed localized Th1 response in the lung only (Citation[4]). We thus hypothesised that Th1-associated chemokines or their receptors on CD8+T-cells may be differentially expressed in the blood of healthy smokers, current smoker COPD subjects and those who had ceased smoking.
We investigated chemokines, chemokine receptors and Th1 and cytotoxic T-cell markers in both peripheral blood and BAL from current and ex-smoker COPD subjects, healthy smokers and never-smoker controls.
MATERIALS AND METHODS
Subject population
Blood analysis of chemokine receptor expression, cytotoxic and Th1 markers, soluble chemokines and intracellular IFN-γ was performed on 25 never smoker controls, 20 current smoker COPD subjects, 20 ex smoker COPD subjects and 10 healthy smokers (). For ethical reasons, bronchoscopy was not performed on those subjects with an FEV1 of less than 1.4 L. Analysis of soluble chemokines was thus performed on BAL from 22 never-smoker controls, 19 current smoker and 19 ex-smoker COPD subjects and 11 healthy smokers (). Chemokine receptor expression and intracellular IFN-γ was investigated in BAL from a cohort of these subjects: 5 never-smoker controls, 8 current and 9 ex-smoker COPD subjects and 5 healthy smokers. The clinical phenotypes of these subjects were within the ranges presented for the subjects in the total ‘BAL’ group ().
Table 1 Patient demographics of the population studied (data expressed as median ± data range), * p < 0.05 versus never smoker controls
Volunteers were specifically recruited for the study and informed consent obtained. Ethics approval was obtained from the Royal Adelaide Hospital. Patients underwent spirometry and chest x-ray as part of their routine clinical assessment. For COPD subjects there was no exacerbation of the disease for 6 weeks prior to involvement in the study. Clinical infection was excluded based on no significant bacterial growth in BAL (occasional ‘oral flora’). The diagnosis of COPD was established using the GOLD criteria (FEV1/FVC < 70%) with clinical correlation (Citation[14]). Seven of the current and 11 of the ex-smoker COPD subjects were treated with inhaled corticosteroids.
Blood collection
Venous blood was collected into tubes containing 10 U/mL preservative free sodium heparin (DBL, Sydney, Australia). Blood differential cell counts were performed using a CELL DYN 4000 (Abbott Diagnostics, Sydney, Australia). Samples were processed for flow cytometry and analysed within 1 hour of collection. For subsequent chemokine analysis, samples were centrifuged at 3000 × g for 5 minutes then plasma was removed and frozen at −70°C.
Bronchoscopy procedure
BAL was obtained via flexible bronchoscopy, as we have previously described (Citation[16]). Briefly, a 50 mL aliquot of sterile normal saline was instilled into the airway with a syringe then aspirated using low suction. To obtain sufficient T-cells for analysis two further 50 mL aliquots of saline were instilled and aspirated in the same way. For each collection from an individual patient the aspirated BAL specimens 2 and 3 were pooled, kept on ice and processed within 1 h of collection. The first aliquot was not used for T-cell analysis due to bronchial contamination but processed for microbiological testing. BAL cells were washed in RPMI 1640 (Gibco, BRL, Germany), and re-suspended in RPMI 1640 media supplemented with 10% fetal calf serum (Gibco) and 1% weight per volume penicillin/streptomycin (Gibco) (culture medium) at a concentration of 3 × 106/ml.
Total cell counts in BAL were performed by a diagnostic laboratory (Histopathology Department, IMVS, Adelaide, Australia). BAL differential cells counts were performed using flow cytometry as previously described (Citation[16]).
Flow cytometric investigation of T-cell surface receptors
For BAL and peripheral blood, the percentages of CD3 and CD8+ T-cells were calculated using flow cytometry as previously described (Citation[17]). Absolute numbers were calculated from total leucocyte counts. Samples were analysed using a panel of fluorescently conjugated monoclonal antibodies (Mabs) to characterise leukocyte sub-types, and a combination of conjugated and unconjugated monoclonal antibodies to assess T-cell surface expression of chemokine receptors. Conjugated Mabs were purchased from BD Biosciences (BD) (San Jose, CA, USA) and Coulter Immunotech (Florida, USA). Unconjugated Mabs were purchased from R&D (MN, USA). Details of the various Mabs used are presented in .
Table 2 Mabs used for investigation of chemokine receptor expression
One hundred μ L of blood cells were lysed by the addition of 2 mL of Facslyse (BD) and samples incubated for 10 min at room temperature in the dark. Samples were centrifuged at 500 × g for 1 minute and the supernatant discarded. White cells were washed with 2mL of Isoton II (Coulter Immunotech) containing 0.5% BSA (wash buffer). Twenty-five μ L of Intragram P (Commonwealth Serum Laboratories (CSL), Australia) was added and the cells incubated at room temperature for 10 min prior to addition of 2.5 μ L of the appropriately pre-titrated Mab for 10 min and washing with 2 mL of wash buffer.
For unconjugated antibodies, 2.5 μ L of diluted biotinylated anti-mouse antibody titrated for optimal staining (Vector Laboratories, Burlingame, USA) was incubated with the cells for 10 min. Cells were washed with wash buffer and incubated for 10 min in the dark with 2.5 μ L of strepavidin-R-phycoerythrin conjugate (Sigma, Missouri, USA). Cells were further washed then incubated with a fluorochrome conjugated Mab to identify leucocyte sub-types for 10 min at room temperature in the dark. Cells were again washed and data acquisition was performed immediately using a FACSCalibur flow cytometer (BD). Data analysis was performed using CellQuest software (BD). Quadrant markers were set using appropriate Ig controls. Results were expressed as a percentage of positive cells. Surface maker staining of BAL derived T-cells was performed as described for blood T-cells, without the cell lysis procedure. Examples of flow cytometric analysis of chemokine receptors on blood and BAL are presented in .
Figure 1 Representative flow cytometry dot plots of chemokine receptor expression on T-cells from blood (A-E) and BAL (F-G) from a never smoker control and a COPD subject. A. and F. CCR5; B and G. CXCR3; C. CCR4; D. CXCR4 and E. intracellular CCR3. T cells were selected using SSC vs. CD3 (data not shown). Quadrant markers were set using a cell population known to lack expression of the marker of interest. The chemokine [PE stained] of interest is on the y-axis and CD8 [FITC] on the x-axis (with the exception of CCR5 [FITC] on the x-axis with CD8 [PE] on the y-axis). The percentage of cells expressing chemokine receptors are shown in the relevant quadrants.
![Figure 1 Representative flow cytometry dot plots of chemokine receptor expression on T-cells from blood (A-E) and BAL (F-G) from a never smoker control and a COPD subject. A. and F. CCR5; B and G. CXCR3; C. CCR4; D. CXCR4 and E. intracellular CCR3. T cells were selected using SSC vs. CD3 (data not shown). Quadrant markers were set using a cell population known to lack expression of the marker of interest. The chemokine [PE stained] of interest is on the y-axis and CD8 [FITC] on the x-axis (with the exception of CCR5 [FITC] on the x-axis with CD8 [PE] on the y-axis). The percentage of cells expressing chemokine receptors are shown in the relevant quadrants.](/cms/asset/4f40f4c0-bf4d-43b3-b38a-0f4ce8ef85ff/icop_a_372586_uf0001_b.gif)
Flow cytometric determination of intracellular chemokine receptor expression
Staining procedure was similar to surface marker analysis, except for the inclusion of a cell permeabilising step to allow the detection of the intracellular signal. Following lysis of red blood cells, 500 μ L of Facsperm (BD) was incubated with the cells at room temperature for 10 minutes. Cells were washed with 2 mL of wash buffer and cell surface staining performed as described above.
ELISA determination of soluble chemokines
Levels of MCP-1, MCP-3 and ITAC in plasma and BAL were measured using an ELISA kit (R&D) according to the manufacturer's instructions.
Cytometric Bead Array for investigation of soluble chemokines
Plasma chemokines (IL-8, RANTES, MIG, and IP-10) were measured simultaneously using a Cytometric Bead Array Human Chemokine kit (BD) as we have previously described (Citation[18]).
Flow cytometric investigation of intracellular IFN-γ
BAL or peripheral blood was incubated for 24 h, at 37°C in 5% CO2 in air, with 25 ng/mL PMA (Sigma, St. Louis, MO), 1 μ g/mL ionomycin (Calbiochem, La Jolla, CA) and 10 μ g/mL Brefeldin A (as a Golgi block, inhibiting intracellular transport thus retaining the cytokines produced during activation inside the cell). To enable intracellular staining, the cells were permeabilised with 500 μ L of FACSPerm (BD) for 10 min at room temperature, and then washed with wash buffer, centrifuged, supernatant discarded, and the cells incubated at room temperature for 10 min with 25 μ L of Intragram P (CSL) (to block Fc receptors and reduce non-specific binding) then with the fluorescent-conjugated antibodies to intracellular IFN-γ and surface markers to enable cell subset identification. Acquisition and analysis was carried out as described above.
Statistical analysis
The Kruskall–Wallis and Mann–Whitney U-tests were applied to analyse the non-normally distributed data. Correlation between disease severity (FEV1) or CD8+ T-cell numbers in BAL and levels of chemokines or expression of chemokine receptors was performed using Spearman's rank correlation. Analysis was performed using SPSS software. p values < 0.05 were considered significant.
RESULTS
Increased CD8+ T-cells in COPD in BAL and peripheral blood
Differential counting of BAL was performed using flow cytometry as previously described (Citation[16]). Absolute leucocyte numbers were significantly increased in both COPD groups and healthy smokers (). To account for these differences, lymphocyte numbers were thereafter calculated as absolute numbers relative to BAL leucocyte counts. For BAL, absolute numbers of lymphocytes were not significantly different between COPD subjects or healthy smokers compared to controls (). The percentage of CD8+ T-cells was higher in BAL from both COPD groups and healthy smokers compared to controls, while the absolute numbers of these cells were significantly increased only in the ex-smoker COPD group ().
Table 3 Differential cell counts
In peripheral blood, there were no significant differences in lymphocyte numbers or subsets in healthy smokers compared to controls. The absolute number of CD8+ T-cells was significantly higher in peripheral blood from current and ex-smoking COPD subjects compared to controls ().
Leukocyte counts in blood and BAL from never smoker controls, current and ex-smoker COPD subjects and healthy smokers. Data represent median [data range]. Both the absolute CD8+ T-cell numbers and the percentage of CD8+ T-cells are shown. *p < 0.05 versus controls.
Increased expression of chemokine receptors in COPD
A summary of all data is presented in . In peripheral blood, chemokine receptors CXCR3, CXCR4, CCR3, CCR4 and CCR5 (on CD3+ and CD8+ T-cells), the cytotoxic T-cell marker CD11A bright (on CD8+ T-cells), the Th1 marker 62L−CD45RO+ (on CD3+ T-cells) (Citation[20]) were investigated. Chemokine receptors CXCR3, CXCR4, CCR5 (on CD3+ T-cells), the Th1 marker 62L−CD45RO+ (on CD3+ T-cells) and the cytotoxic T-cell marker CD28bright (on CD8+ T-cells) were the only surface molecules investigated on BAL samples due to the limited numbers of T-cells.
Table 4 Summary of results
In BAL and peripheral blood, the percentage of T-cells expressing CXCR3 was increased in both COPD groups and healthy smokers compared to controls (). In BAL, the percentage of T-cells expressing CCR5 was increased in both COPD groups and smokers (). In peripheral blood , the percentage of CD3+and CD8+ T-cells expressing CCR5 was significantly increased in both COPD groups but not smokers ().
Figure 2 A. Cell membrane expression of CCR5 and CXCR3 (as a percentage of CD3+ T-cells) in peripheral blood (mean ± SEM). Similar results were noted for CD8+ T-cells (not presented). B. BAL. Cell membrane expression of CCR5 and CXCR3 (as a percentage of CD3+ T-cells). * p < 0.05 versus never-smoker controls.
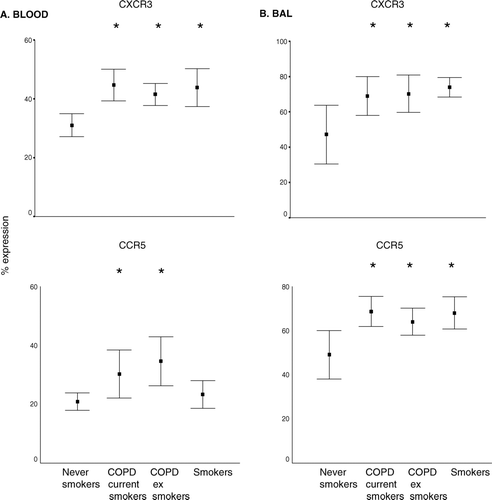
The percentage of CD3+ and CD8+ T-cells expressing CCR4 was significantly increased in blood from both current and ex-smoker COPD subjects and smokers compared to controls (). CXCR4 expression was detected on T-cells in peripheral blood and BAL [data range of expression as a percentage of CD8+ T-cells from all subjects: 60–73%] but no significant differences were noted among the groups.
Figure 3 Intracellular expression of CCR3 and cell membrane expression of CCR4 (as a percentage of CD3+ T-cells) in peripheral blood (mean ± SEM). * p < 0.05 versus never smoker controls. Note increased expression of CCR4 and intracellular CCR3 in all groups compared to never smoker controls. Similar results were noted for CD3+ T-cells (not presented). Data is presented as box plots showing median ± 25th and 75th percentiles (solid box) and the 10th and 90th percentiles shown by whiskers outside the box.
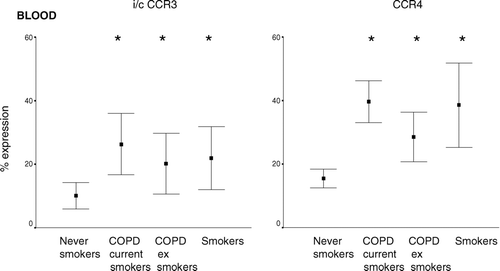
CCR3 expression was low on the surface of T-cells in any patient group; however this was the only chemokine receptor which was detected intracellularly in peripheral blood T-cells. Intracellular expression of CCR3 was increased in peripheral blood CD3+ and CD8+ T-cells from both COPD groups. There was also a non-significant trend for increased intracellular CCR3 expression in CD3+ and CD8+ T-cells from healthy smokers ().
Increased Th1 and cytotoxic T-cell markers in COPD
Absent expression of CD62L on CD45RO+T-cells was applied as an indicator of Th1-type T-cells (Citation[20]). This subset was increased in peripheral blood and BAL T-cells from both COPD groups and smokers compared to controls (), although the increase did not reach statistical significance in BAL from current smokers with/without COPD.
Figure 4 Cytotoxic and Th1 markers in A. peripheral blood and B. BAL (mean ± SEM). Th1 type T-cells were considered to be CD3+CD45RO+CD62L− T-cells. Cytotoxic T-cells were considered to be CD8+CD11abright or CD8+CD28bright. * p < 0.05 versus never smokers. Note the significantly increased percentage of Th1 type T-cells in both COPD groups and healthy smokers but an increase in cytotoxic T-cells only in the COPD groups.
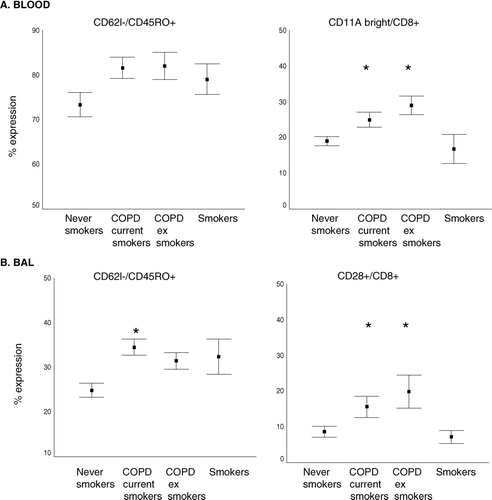
Expression of CD28bright or CD11abright on CD8+ T-cells was applied as an indicator of cytotoxic T-cells(Citation[21]). In BAL from both COPD groups but not smokers, there was a significantly greater percentage of CD8+ T-cells that expressed the cytotoxic marker CD28bright compared to controls (). Similarly, in peripheral blood from both COPD groups but not smokers there was a significantly greater percentage of CD8+ T-cells expressing the CD11abright cytotoxic marker ().
Intracellular IFN-γ
In blood, the percentage of CD8+ T-cells expressing intracellular IFN-γ was significantly increased in both COPD groups but not smokers compared to controls (). In BAL, the percentage of CD8+ T-cells expressing intracellular IFN-γ was significantly increased in both COPD groups and smokers compared to controls ().
Figure 5 Intracellular expression of IFN-γ (as a percentage of CD8+ T-cells) in A. blood and B. BAL from COPD subjects, asymptomatic smokers and healthy never-smokers measured current and ex-smoker by flow cytometry (mean ± SEM). * p < 0.05 versus never-smokers. There were significantly increased levels of IFN-γ in blood CD8+ T-cells from both current and ex-smoker COPD subjects but no change in healthy smokers compared to never-smoker controls.
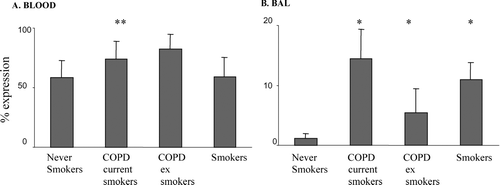
INCREASED LEVELS OF CHEMOKINES IN PLASMA AND BAL FROM COPD SUBJECTS
IP-10
Plasma and BAL levels of IP-10 were not significantly increased in COPD subjects or smokers compared to controls [data range from all subjects in plasma 147–2541 pg/mL; BAL 44–2500 pg/mL].
MCP-1
MCP-1 levels were significantly increased in BAL from all groups compared to controls (). There was a non-significant trend for increased expression in blood from both COPD groups and smokers.
Figure 6 Soluble MCP-1, MIG and ITAC levels in plasma and BAL from current and ex-smoker COPD subjects, healthy smokers and never-smoker controls (mean ± SEM). MCP-1 and ITAC were measured by ELISA and MIG by cytometric bead array A. plasma B. BAL. * p < 0.05 versus never-smokers. All chemokines were increased in BAL from all groups compared to controls. Note increased levels of MIG and ITAC in plasma from both current and ex-smoker COPD subjects but no change in healthy smokers.
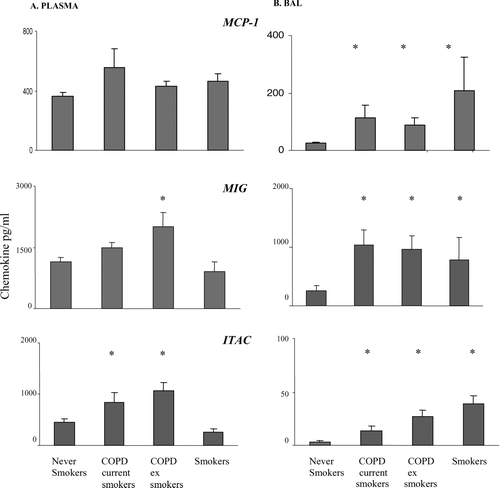
MIG and ITAC
Levels of MIG and ITAC were significantly increased in BAL from both COPD groups and smokers compared to controls (). In contrast, levels of these chemokines were significantly increased in plasma from both COPD groups but not smokers ().
RANTES
Levels of RANTES were significantly increased in BAL from both COPD groups and smokers compared to controls (). There were no significant changes in levels of RANTES in plasma from any group [data range from all subjects 7–1973 pg/mL].
Figure 7 Soluble cytokine levels in BAL from current and ex-smoker COPD subjects, healthy smokers and never-smoker controls measured by cytometric bead array (mean ± SEM). A. RANTES B. IL-8. * p < 0.05 versus never-smokers. ** p < 0.05 versus current smokers. ns: no significant differences between groups. There were no significant differences in levels of these soluble chemokines in plasma (not shown).
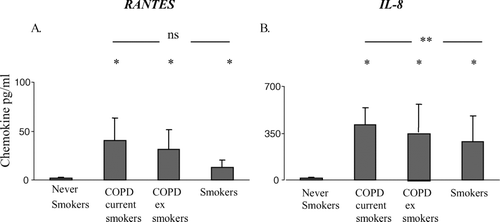
IL-8
Levels of IL-8 in BAL from both COPD groups and smokers were significantly increased compared to controls () although there were no significant differences in plasma from any group [data range from all subjects in plasma 12–2500 pg/mL]. In BAL, when compared to current-smoker COPD subjects, smokers exhibited significantly lower levels of IL-8.
Correlation between chemokines, chemokine receptors and COPD severity
There was a weak but significant correlation between expression of CXCR3 and CCR5 on blood T-cells and disease severity (lower FEV1) (). In BAL, there was also a weak (but not significant) correlation between T-cell expression of CXCR3 and FEV1 (correlation 0.331,ns). There were no significant correlations between levels of other blood or BAL chemokine receptors and disease severity (FEV1) (data not shown).
Figure 8 Correlation between A. % CCR5 expression and B. % CXCR3 expression by peripheral blood CD8+ T-cells and COPD disease severity (FEV1).
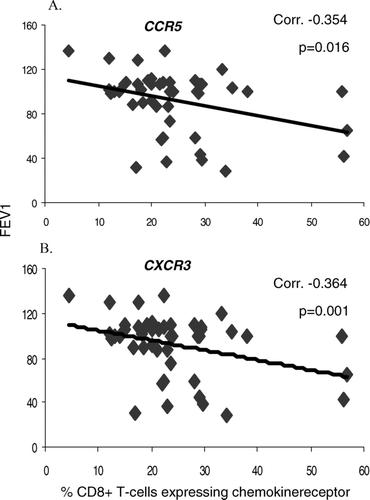
Intracellular or secreted cytokines/chemokines, chemokine receptors and Th1 and cytotoxic T-cell markers in peripheral blood and BAL from current and ex smoker COPD subjects and healthy smokers compared to never-smoker controls. Note differential expression of CD28bright on CD8+ T-cells from BAL, CCR5 and CD11Abright in blood and IL-8, MIG, ITAC and IFN-g in blood from healthy smokers compared to COPD subjects. *p < 0.05 versus never-smokers, i/c intracellular, ns not significantly different from never-smoker controls, nt not tested, CBA cytometric bead array, BAL bronchoalveolar lavage.
DISCUSSION
This is the first comprehensive study of a range of chemokines and T-cell chemokine receptors in peripheral blood and BAL from current and ex-smokers with COPD and healthy smokers. Several of the changs in chemokines and their receptors noted in the airways in COPD were reflected in the peripheral blood, supporting current opinion that COPD is a systemic disease. Both current and ex-smoker COPD subjects showed a significant increase in several chemokines and their receptors as well as percentage and absolute numbers of CD8+ T-cells compared to never-smoker controls in peripheral blood and BAL. These findings are consistent with reports by others, however other studies have not divided the COPD subjects and controls based on their smoking status (Citation[19], Citation[22]).
In the present study, the increase in chemokines and their receptors was accompanied by an increased percentage of cytotoxic CD8+ T-cells in BAL, consistent with the persistence of ongoing peripheral and airway inflammation in COPD subjects who had ceased smoking (Citation[23]). There were no changes in cytotoxic T-cell markers in peripheral blood or BAL from healthy smokers, compared to never-smoker controls although there was an increase in CD8+ T-cells in BAL from smokers.
Increased expression of CXCR3 and CCR5, IL-8, MCP-1, ITAC and MIG in sputum, lung tissue or BAL from subjects with COPD has been reported by others (Citation[10], Citation[13], Citation[14], Citation[24], Citation[25], Citation[26]). Interestingly, these studies have reported an association between levels of CXCR3 in the lungs of COPD subjects with increased CD8+ T-cell numbers in the lung (Citation[13]) and an association between loss of lung function in COPD subjects with a high percentage of T-cells that expressed CCR5 and CXCR3 (Citation[14]). However these studies did not investigate the role of smoking status among those with COPD nor did they compare levels of chemokines and their receptors in BAL and blood.
The present study found that peripheral blood levels of MCP-1 and the percentage of blood CD8+ T-cells expressing CXCR3, CCR4, and intracellular CCR3 were increased in both current and ex-smoker COPD groups and healthy smokers, suggesting a chronic smoking-related phenomenon with systemic involvement that is not improved by smoking cessation once COPD disease has established. It is possible that the persistence of these chemokines and their receptors after smoking cessation in COPD results from changes to the airway after years of noxious insult or the continued immune stimuli by chronic colonisation of the respiratory tract with bacterial or viral pathogens (Citation[27]).
Levels of ITAC, MIG and the percentage of CD8+ T-cells expressing IFN-γ and CCR5 were increased in the blood of both COPD groups but not healthy smokers, suggesting a link to COPD disease progression rather than smoking per se. In contrast, these differences were not noted in BAL (levels of IFN-γ, RANTES, IL-8, MCP-1, MIG and ITAC were all increased in BAL from both COPD subjects and healthy smokers). Thus the relatively non-invasive investigation of peripheral blood may be useful to monitor inflammatory markers that could potentially distinguish smokers with sub-clinical COPD. Of interest in the present study were the occasional healthy smokers who showed increased levels of these mediators. These subjects may be suitable for inclusion in a longitudinal study where they are closely monitored (both clinically and by computed tomographic (CT) scan and measurement of lung function) for onset of clinically evident COPD.
In this regard, IL-8 in BAL has been proposed as a marker of smokers susceptible to pulmonary emphysema. A study by Tanino et al. (Citation[11]) investigated IL-8 levels in smokers with CT evidence of subclinical emphysema reported that IL-8 levels could distinguish between current-smokers with sub-clinical emphysema and current smokers without emphysema. In our present study, levels of both IL-8 and RANTES in BAL were much lower (IL-8 reaching statistical significance) in healthy smokers compared to current-smoker COPD subjects, supporting the inclusion of these cytokines in any study that attempts to characterise smokers susceptible to COPD.
We and others have shown that COPD is associated with a Th1 T-cell response (Citation[4], Citation[13]). Consistent with these reports, the present study noted increased expression of chemokine receptors CXCR3 and CCR5 (both markers of Th1 cells) in both plasma and BAL from patients with COPD. In addition, there was increased expression of 62L−CD45RO+ (a further Th1 marker) on blood and BAL T-cells from COPD subjects and healthy smokers. Levels of IP-10 and MIG, both ligands for CXCR3 and CCR5, were also upregulated in BAL and plasma from COPD subjects, consistent with the increased receptor expression.
Levels of the Th1 cytokine, IFN-γ and IFN-γ inducible chemokines (MIG and ITAC) were also increased in plasma and BAL from COPD subjects compared to never-smoker controls. The induction of CXCR3, and possibly CCR5 by IFN-γ, provides a possible mechanism by which chemokines and their receptors are involved in the inflammatory process of patients with COPD. Production of MIG and ITAC by airway cells such as epithelial cells may result in the recruitment of CXCR3 and CCR5 expressing type 1 T cells into the airways (Citation[13]), which in turn release more IFN-γ and further up-regulate chemokine production through a positive feedback. It is notable that levels of a further IFN-γ inducible chemokine, IP-10, were not significantly different between groups either in plasma or BAL. This is inconsistent with the observed increase in MIG and I-TAC, as they are ligands for the same receptor and activated under the same conditions. Other studies that have investigated IP-10 in COPD have found increased expression compared to non-COPD patients, however these studies investigated lung sections and bronchial biopsies (Citation[13], Citation[14]).
RANTES has been shown to be an agonist for CCR5, however its role in COPD has not been extensively evaluated although there have been reports of strong gene expression for RANTES on bronchial biopsies from subjects with exacerbations of chronic bronchitis (Citation[28]). The present study noted increased levels of RANTES in BAL but not plasma of both current and ex-smoker COPD groups and healthy smokers compared to never-smoker controls, suggesting a role for RANTES in the CCR5 mediated recruitment of Th1 type T-cells into the lung.
In contrast to the increases in Th1 biased mediators, a significant increase in CCR4 expression in peripheral blood CD8+ T-cells from both COPD groups and healthy smokers compared to never-smokers was also noted. CCR4 has classically been associated with increased infiltration of Th2 type cells, which lead to its implications in asthma. However, a recent study has showed elevated expression of TARC and MDC in models of cigarette smoke-induced pulmonary inflammation (Citation[29]). MDC and TARC are both chemokines which bind to the CCR4 receptor. This study suggests that some chemokine receptors may not be specifically associated with Th1 or Th2 type responses, but may interplay between the two.
In this first investigation of intracellular T-cell chemokine receptors, CCR3 was the only intracellular receptor that could be detected. Several reports have suggested that CCR3 is internalised upon binding to its ligand, eotaxin, desensitising the receptor to the ligand (Citation[30]). This process is thought to promote the antagonistic effects of eotaxin on MCP-1/CCR2 mediated chemotaxis of leucocytes by promoting the movement of leucocytes away from a gradient of eotaxin. Our findings of increased MCP-1 and an increased percentage of T-cells expressing intracellular CCR3 in blood, taken together with a previous report of increased eotaxin in COPD, suggest that this antagonistic process may be relevant to T-cell trafficking in the disease.
Interestingly, the present study showed that expression of CXCR3 and CCR5 by CD8+ T-cells in peripheral blood negatively correlated with disease severity, suggesting that therapeutic agents that target these chemokine receptors may effectively reduce the Th1 bias as well as controlling the influx of cytotoxic CD8+ T-cells into the airway in COPD. The lack of correlation between CXCR3 and CCR5 expression by CD8+ T-cells in BAL and FEV1 may be due to chemokine receptor shedding through the indirect action of TNF-α (Citation[31]). In this regard we have previously shown a negative correlation between FEV1 and TNF-α production by CD8+ T-cells in the BAL of COPD patients (Citation[4]).
Taken together, our findings are consistent with systemic inflammation in smokers with and without COPD that may be associated with increased chemotaxis of cytotoxic and Th1 T-cells into the airway. The differential expression of certain chemokines and their receptors in blood and BAL from smokers and COPD subjects indicate a potential application to identify smokers with possible sub-clinical COPD.
GLOSSARY
BAL: | = | bronchoalveolar lavage |
CD: | = | cluster designation |
COPD: | = | chronic obstructive pulmonary disease |
FEV1: | = | forced expiratory volume in one second |
FVC: | = | forced vital capacity |
IP-10: | = | interferon-gamma inducible protein |
IL-8: | = | interleukin-8 |
ITAC: | = | interferon inducible T cell chemoattractant |
Mab: | = | monoclonal antibody |
MIG: | = | monokine induced by interferon gamma |
RANTES: | = | Regulated on activation normal T cell expressed and secreted |
Th1: | = | T helper type 1 |
ACKNOWLEDGMENTS
The authors thank the staff of Thoracic Medicine, Royal Adelaide Hospital, for their help in obtaining patient samples. The work was supported by an NHMRC Project Grant, NHMRC Clinical Career Development Award, Allen & Hanburys Respiratory Research Fellowship and Royal Adelaide Hospital Clinical Project Grant.
REFERENCES
- Viegi G, Scognamiglio A, Baldacci S, Pistelli F, Carrozzi L. Epidemiology of chronic obstructive pulmonary disease (COPD). Respiration 2001; 68(1)4–19
- Barnes P J. Mediators of chronic obstructive pulmonary disease. Pharmacol Rev 2004; 56(4)515–548
- Hodge S, Hodge G, Holmes M, Reynolds P N. Increased airway epithelial and T-cell apoptosis in COPD remains despite smoking cessation. Eur Respir J 2005; 25(3)447–454
- Hodge G, Nairn J, Holmes M, Reynolds P N, Hodge S. Increased intracellular T helper 1 proinflammatory cytokine production in peripheral blood, bronchoalveolar lavage and intraepithelial T cells of COPD subjects. Clin Exp Immunol 2007; 150(1)22–29
- Majo J, Ghezzo H, Cosio M G. Lymphocyte population and apoptosis in the lungs of smokers and their relation to emphysema. Eur Respir J 2001; 17(5)946–953
- Finkelstein R, Fraser R S, Ghezzo H, Cosio M G. Alveolar inflammation and its relation to emphysema in smokers. Am J Respir Crit Care Med 1995; 152: 1666–1672, (5 Pt 1)
- Hodge S, Hodge G, Nairn J, Holmes M, Reynolds P N. Increased airway granzyme b and perforin in current and ex-smoking COPD subjects. COPD J Chron Obstruct Pulmon Dis 2006; 3: 1–9
- Hodge S, Hodge G, Flower R, Han P. Surface activation markers of T lymphocytes: role in the detection of infection in neonates. Clin Exp Immunol 1998; 113(1)33–38
- Keatings V M, Collins P D, Scott D M, Barnes P J. Differences in interleukin-8 and tumor necrosis factor-alpha in induced sputum from patients with chronic obstructive pulmonary disease or asthma. Am J Respir Crit Care Med 1996; 153: 530–534
- Traves S L, Culpitt S V, Russell R E, Barnes P J, Donnelly L E. Increased levels of the chemokines GROalpha and MCP-1 in sputum samples from patients with COPD. Thorax 2002; 57(7)590–595
- Tanino M, Betsuyaku T, Takeyabu K, Tanino Y, Yamaguchi E, Miyamoto K, Nishimura M. Increased levels of interleukin-8 in BAL fluid from smokers susceptible to pulmonary emphysema. Thorax 2002; 57(5)405–411
- Hall Z, Barnes P J, Donnely L E. Alveolar macrophage production of CXCR3 chemokines. Am J Respir Crit Care Med 2004; 169: A169
- Saetta M, Mariani M, Panina-Bordignon P, Turato G, Buonsanti C, Baraldo S, Bellettato C M, Papi A, Corbetta L, Zuin R, et al. Increased expression of the chemokine receptor CXCR3 and its ligand CXCL10 in peripheral airways of smokers with chronic obstructive pulmonary disease. Am J Respir Crit Care Med 2002; 165(10)1404–1409
- Grumelli S, Corry D B, Song L Z, Song L, Green L, Huh J, Hacken J, Espada R, Bag R, Lewis D E, et al. An immune basis for lung parenchymal destruction in chronic obstructive pulmonary disease and emphysema. PLoS Med 2004; 1(1)e8
- Fabbri L M, Hurd S S. Global Strategy for the Diagnosis, Management and Prevention of COPD: 2003 update. Eur Respir J 2003; 22(1)1–2
- Hodge S J, Hodge G L, Holmes M, Reynolds P N. Flow cytometric characterization of cell populations in bronchoalveolar lavage and bronchial brushings from patients with chronic obstructive pulmonary disease. Cytometry B Clin Cytom 2004; 61(1)27–34
- Han P, Hodge G, Story C, Xu X. Phenotypic analysis of functional T-lymphocyte subtypes and natural killer cells in human cord blood: relevance to umbilical cord blood transplantation. Br J Haematol 1995; 89(4)733–740
- Hodge G, Hodge S, Haslam R, McPhee A, Sepulveda H, Morgan E, Nicholson I, Zola H. Rapid simultaneous measurement of multiple cytokines using 100 microl sample volumes—association with neonatal sepsis. Clin Exp Immunol 2004; 137(2)402–407
- Traves S L, Donnelly L E. Chemokines and their receptors as targets for the treatment of COPD. Curr Respir Med Rev 2005; 1: 15–32
- Kanegane H, Tosato G. Activation of naive and memory T cells by interleukin-15. Blood 1996; 88(1)230–235
- Oughton J A, Kerkvliet N I. Novel phenotype associated with in vivo activated CTL precursors. Clin Immunol 1999; 90(3)323–333
- de Jong J W, van der Belt-Gritter B, Koeter G H, Postma D S. Peripheral blood lymphocyte cell subsets in subjects with chronic obstructive pulmonary disease: association with smoking, IgE and lung function. Respir Med 1997; 91(2)67–76
- Rutgers S R, Timens W, Kaufmann H F, van der Mark T W, Koeter G H, Postma D S. Comparison of induced sputum with bronchial wash, bronchoalveolar lavage and bronchial biopsies in COPD. Eur Respir J 2000; 15(1)109–115
- Tzanakis N, Chrysofakis G, Tsoumakidou M, Kyriakou D, Tsiligianni J, Bouros D, Siafakas N M. Induced sputum CD8+ T-lymphocyte subpopulations in chronic obstructive pulmonary disease. Respir Med 2004; 98(1)57–65
- Keatings V M, Collins P D, Scott D M, Barnes P J. Differences in interleukin-8 and tumor necrosis factor-alpha in induced sputum from patients with chronic obstructive pulmonary disease or asthma. Am J Respir Crit Care Med 1996; 153(2)530–534
- Traves S L, Smith S J, Barnes P J, Donnelly L E. Specific CXC but not CC chemokines cause elevated monocyte migration in COPD: a role for CXCR2. J Leukoc Biol Aug, 2004; 76(2)441–450
- Sethi S, Murphy T F. Bacterial infection in chronic obstructive pulmonary disease in 2000: a state-of-the-art review. Clin Microbiol Rev 2001; 14(2)336–363
- Jeffery P K. Lymphocytes, chronic bronchitis and chronic obstructive pulmonary disease. Novartis Found Symp 2001; 234: 149–161
- Ritter M, Goggel R, Chaudhary N, Wiedenmann A, Jung B, Weith A, Seither P. Elevated expression of TARC (CCL17) and MDC (CCL22) in models of cigarette smoke-induced pulmonary inflammation. Biochem Biophys Res Commun 2005; 334(1)254–262
- Ogilvie P, Bardi G, Clark-Lewis I, Baggiolini M, Uguccioni M. Eotaxin is a natural antagonist for CCR2 and an agonist for CCR5. Blood 2001; 97(7)1920–1924
- Hornung F, Scala G, Lenardo M J. TNF-α induced secretion of C-C chemokines modulates CC chemokine receptor 5 expression on peripheral blood monocytes. J Immunol 2000; 164: 6180–6187