Abstract
Platelets play an important role in the pathophysiology of chronic obstructive pulmonary disease (COPD) by mediating thrombotic, inflammatory, and immune processes in the lung. We conducted a systematic review and meta-analysis of studies investigating the platelet count and three platelet indices, mean platelet volume (MPV), platelet distribution width (PDW), and platelet to lymphocyte ratio (PLR) in stable COPD vs. non-COPD patients and in stable COPD vs. acute exacerbation of COPD (AECOPD) patients (PROSPERO registration number: CRD42021228263). PubMed, Web of Science, Scopus and Google Scholar were searched from inception to December 2020. Twenty-seven studies were included in the meta-analysis, 26 comparing 4,455 stable COPD patients with 7,128 non-COPD controls and 14 comparing 1,251 stable COPD with 904 AECOPD patients. Stable COPD patients had significantly higher platelet counts (weighted mean difference, WMD = 13.39 x109/L, 95% CI 4.68 to 22.11 x109/L; p < 0.001) and PLR (WMD = 59.52, 95% CI 29.59 to 89.44; p < 0.001) than non-COPD subjects. AECOPD patients had significantly higher PLR values than stable COPD patients (WMD = 46.03, 95% CI 7.70 to 84.35; p = 0.02). No significant differences were observed in MPV and PDW. Between-study heterogeneity was extreme. In sensitivity analysis, the effect size was not modified when each study was sequentially removed. The was no evidence of publication bias. In our meta-analysis, specific platelet biomarkers were associated with stable COPD (platelet count and PLR) and AECOPD (PLR). However, the observed heterogeneity limits the generalizability of the findings. Further studies are required to determine their prognostic utility and the effects of targeted interventions in COPD.
Introduction
An increasing body of evidence suggests that platelets exert significant modulatory effects on inflammatory- and immune-mediated pathways, in addition to their established role in hemostasis and thrombosis [Citation1, Citation2]. Therefore, alterations in platelet activity might play an important pathophysiological role in several acute and chronic disease states that are associated with excessive local and/or systemic inflammatory and thrombotic burden. One such condition is chronic obstructive pulmonary disease (COPD), a leading cause of disability and mortality worldwide that is characterized by airway inflammation, excessive mucus production, progressive destruction of the lung parenchyma with loss of elasticity, and abnormal remodeling of the pulmonary vasculature [Citation3]. A number of studies support the proposition that platelets are involved in the pathogenesis and the progression of COPD, particularly through an increase in leukocyte elastase activity, the formation of platelet-monocyte aggregates, and the dysregulation of specific hypoxia-related signaling pathways [Citation4–6]. Therefore, biomarkers of platelet function might be useful in the early diagnosis of COPD and, particularly, in predicting those COPD patients that are at a higher risk of acute exacerbation of the disease (AECOPD), a condition that often requires hospitalization and is associated with significantly worse survival outcomes [Citation6]. A number of platelet indices, in addition to platelet count, have been used for clinical and research purposes in respiratory and non-respiratory disease states. They include the mean platelet volume (MPV), a marker of platelet size [Citation7], the platelet distribution width (PDW), a marker of the variability in platelet size distribution [Citation8], and the platelet to lymphocyte ratio (PLR), a marker of inflammation [Citation9]. Given the increasing evidence of the pathophysiological role of platelets in COPD, we conducted a systematic review and meta-analysis of studies that investigated the platelet count, MPV, PDW, and PLR in stable COPD vs. non-COPD patients and in stable COPD vs. AECOPD patients.
Materials and methods
Search strategy, eligibility criteria, and study selection
A systematic search was conducted, from inception to December 2020, in the electronic databases PubMed, Web of Science, Scopus and Google Scholar, using the following terms and their combination: “platelets” or “MPV” or “mean platelet volume” or “PDW” or “platelet distribution width” or “PLR” or “platelet to lymphocyte ratio” and “COPD” or “chronic obstructive pulmonary disease” (PROSPERO registration number: CRD42021228263). Abstracts were independently screened by two investigators. If relevant, the two investigators independently reviewed the full articles. Eligibility criteria were as follows: (i) assessment of platelet count and/or MPV and/or PDW and/or PLR in COPD or AECOPD patients; (ii) comparison between stable COPD patients and non-COPD subjects or between stable COPD and AECOPD patients (case-control design); (iii) studies conducted in adults; (iv) ≥10 recruited patients with COPD or AECOPD; (v) English language; and (vi) full-text publications. The references of retrieved articles and reviews were also searched to identify additional studies. Any disagreement between the reviewers was resolved by a third investigator. The Newcastle-Ottawa Scale (NOS) was used to assess study quality [Citation10]. No specific review protocol was developed.
Statistical analysis
Weighted mean differences (WMD) were calculated to build forest plots of continuous data and to evaluate differences in the primary endpoints, platelet count, MPV, PDW, and PLR values, between non-COPD subjects and stable COPD patients or between stable COPD and AECOPD patients. Mean and standard deviation were extrapolated, if necessary, from median and IQR values or median and range values, as previously reported [Citation11, Citation12]. WMD heterogeneity across studies was assessed using the Q-statistic (the significance level was set at p < 0.10). The I2 statistic, a quantitative measure of inconsistency across studies, was also used (I2<25%, no heterogeneity; I2 between 25-50%, moderate heterogeneity; I2 between 50-75%, large heterogeneity; and I2>75%, extreme heterogeneity) [Citation13, Citation14]. Statistical heterogeneity was defined as an I2 value ≥50%. A random-effects model was used in the presence of high heterogeneity. Sensitivity analyses were conducted to investigate the influence of each study on the overall WMD by removing one study at the time [Citation15]. To evaluate the presence of potential publication bias, associations between study size and magnitude of effect were investigated using the Begg’s adjusted rank correlation test and the Egger’s regression asymmetry test, with a p < 0.05 level of significance [Citation16, Citation17]. Confidence intervals (CIs) at 95% were reported for each effect size and the overall effect, with a p < 0.05 level of significance. Statistical analyses were performed using Stata 14 (STATA Corp., College Station, TX, USA). The study was fully compliant with the PRISMA statement on the reporting of systematic reviews and meta-analyses [Citation18].
Results
From a total of 2,233 studies initially identified, 2,202 were excluded after the first screening because they were either duplicates or irrelevant. After a full-text review of the remaining 31 articles, four were further excluded because they did not meet the inclusion criteria or provided incomplete information. Thus, 27 studies were included in the meta-analysis () [Citation19–45].
Stable COPD patients vs. non-COPD subjects
Platelet count
Twenty-three studies, published between 2003 and 2020, investigated the platelet count in 4,291 stable COPD patients (mean age 68 years, 63% males) and 6,969 non-COPD controls (mean age 65 years, 47% males) () [Citation19–30, Citation32–35, Citation37, Citation39–44]. In 19 studies, COPD was diagnosed according to the Global Obstructive Lung Disease (GOLD) guidelines [Citation19, Citation21, Citation23–25, Citation27–29, Citation32–35, Citation37, Citation39, Citation40, Citation42–44], in three according to the American Thoracic Society/European Respiratory Society (ATS/ERS) guidelines [Citation20, Citation22, Citation26], and in one according to the Polish Respiratory Society guidelines (PSR) [Citation41]. The forest plot for platelet counts in non-COPD and stable COPD patients is shown in . In five studies, stable COPD patients had lower platelet counts than non-COPD controls (mean difference range, −31 to −6 × 109/L) [Citation23, Citation26, Citation34, Citation37, Citation42], although the difference was statistically significant only in one study [Citation37]. In the remaining 18 studies, stable COPD patients had higher platelet counts (mean difference range, 4 to 66 × 109/L) [19–Citation22, Citation24, Citation25, Citation27–30, Citation32, Citation33, Citation35, Citation39–41, Citation43, Citation44], and the difference was statistically significant in seven [Citation21, Citation22, Citation25, Citation28, Citation32, Citation39, Citation40]. The extreme heterogeneity between studies (I2=75.9%, p < 0.001) required the use of random-effect models. Pooled results showed that the platelet count was significantly higher in patients with stable COPD (WMD = 13.39 × 109/L, 95% CI 4.68 to 22.11 × 109/L; p < 0.001; statistical power = 0.99). In sensitivity analysis, the corresponding pooled WMD values were not influenced when each study was in turn removed (effect size ranged between 11.1 and 15.4 × 109/L, ). Furthermore, the WMD remained significant (WMD = 14.95 × 109/L, 95% CI 4.52 to 25.38 × 109/L; p = 0.005), with similar between-study variance (I2=75.4%; p < 0.001), after removing two studies accounting for ∼70% of participants [Citation19, Citation43]. The Begg's (p = 0.245) and Egger's t-tests (p = 0.192) showed no significant evidence of publication bias. To explore possible sources of heterogeneity, we investigated by meta-regression analysis the effects of age, gender, body mass index (BMI), publication year, guidelines used for diagnosis, continent where the study was conducted (Europe, Africa, Asia, and America), forced expiratory volume at 1 sec (FEV1), FEV1/forced vital capacity (FVC), white blood cell count (WBC), and C-reactive protein (CRP) (). As shown in , there were no significant differences (t=-0.10; p = 0.92) in pooled WMD values between European (WMD = 13.61 × 109/L, 95% CI 1.65 to 25.54 × 109/L; p = 0.025) and Asian studies (WMD = 13.28 × 109/L, 95% CI −3.09 to 29.65 × 109/L; p = 0.11) even though in the latter the significant difference in pooled platelet counts between stable COPD and non-COPD subjects was lost. A relatively lower heterogeneity in European (I2=60.0%; p = 0.005) vs. Asian studies (I2=86.0%; p < 0.001) was also observed. Similarly, analysis based on specific guidelines () showed comparable pooled WMD values between studies using GOLD (WMD = 13.14 × 109/L, 95% CI 3.98 to 22.30 × 109/L; p = 0.005) and those using ATS/ERS guidelines (WMD = 21.13, 95% CI −27.25 to 69.51 × 109/L; p = 0.39) although in the latter the differences in platelet count between non-COPD and stable COPD subjects were no longer significant. The between-study variance in GOLD studies (I2=75.9%; p < 0.001) was relatively lower than that observed in ATS/ERS studies (I2=87.4%; p < 0.001). Age (t=-0.34; p = 0.74) gender (t=-0.71; p = 0.49), publication year (t=-0.74; p = 0.47), BMI (t=-0.74; p = 0.48), FEV1 (t = 0.91; p = 0.38), FEV1/FVC (t = 0.64; p = 0.54), WBC (t=-0.13; p = 0.90), and CRP (t=-0.37; p = 0.72) were not significantly associated with WMD.
Figure 2. Forest plot of studies examining the platelet count in stable COPD patients and non-COPD subjects.
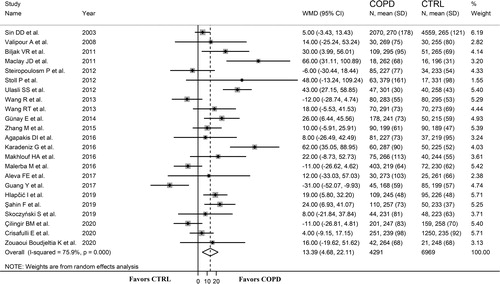
Table 1. Demographic characteristics of the studies included in the meta-analysis.
Table 2. Platelet indices assessed in the meta-analysis.
Table 3. Parameters used in meta-regression analysis.
MPV
Eighteen studies, published between 2011 and 2020, investigated the MPV in 1,063 non-COPD (mean age 61 years, 62% males) and 1,834 stable COPD subjects (mean age 68 years, 69% males) () [Citation21, Citation23, Citation25–36, Citation39, Citation40, Citation42, Citation44]. The GOLD guidelines were used in 16 studies [Citation21, Citation23, Citation25, Citation27–30, Citation32–36, Citation40, Citation42, Citation44], the ATS/ERS guidelines in one [Citation26], whereas no details regarding guidelines were provided in the remaining study [Citation31]. The forest plot of MPV values in non-COPD and stable COPD subjects is shown in . In seven studies, stable COPD patients had lower MPV values compared to non-COPD subjects (mean difference range, −0.84 to −0.05 fL) [Citation26–28, Citation32, Citation36, Citation39, Citation42], and the difference was statistically significant in five [Citation26–28, Citation32, Citation39]. By contrast, in eight studies stable COPD patients had significantly higher MPV values (mean difference range, 0.17 to 0.71 fL) [Citation21, Citation23, Citation29–31, Citation33, Citation34, Citation40], barring one study [Citation30]. Pooled results showed that MPV values were not significantly different between the two groups (WMD = 0.01 fL, 95% CI −0.26 to 0.28 fL; p = 0.94; statistical power = 0.03), with an extreme heterogeneity between studies (I2=89.3%; p < 0.001). Sensitivity analysis showed that the corresponding pooled WMD values were not influenced when each study was in turn removed (effect size ranged between −0.03 and 0.09 fL, ). No significant publication bias was detected using the Begg's (p = 0.705) or Egger's t-test (p = 0.85). In meta-regression analysis, age (t = 0.85; p = 0.41), gender (t = 0.52; p = 0.61), publication year (t=-0.03; p = 0.98), BMI (t=-1.79; p = 0.12), continent (t=-0.45; p = 0.66), diagnostic guideline (t = 0.41; p = 0.69), FEV1/FVC (t = 0.16; p = 0.87), WBC (t = 1.08; p = 0.30), and CRP (t =-0.02; p = 0.99) were not significantly associated with WMD. However, there was a trend toward significance between FEV1 and WMD (t=-1.96; p = 0.08). Sub-group analysis revealed that between-study variance remained extreme after grouping studies according to diagnostic guideline or continent.
PDW
Nine studies, published between 2012 and 2020, investigated the PDW in 593 non-COPD subjects (mean age 57 years, 62% males) and 938 stable COPD patients (mean age 65 years, 71% males) () [Citation23, Citation28, Citation29, Citation32, Citation33, Citation35, Citation39, Citation40, Citation42]. The GOLD guidelines were used in all studies. The forest plot of PDW values in non-COPD and COPD subjects is shown in . In four studies, stable COPD patients had lower PDW values compared to non-COPD subjects (mean difference range, −1.40 to −0.30%) [Citation29, Citation35, Citation39, Citation42], although the difference was statistically significant only in two studies [Citation39, Citation42]. In five studies, stable COPD patients had higher PDW values compared to non-COPD subjects (mean difference range, 0.01 to 2.22%) [Citation23, Citation28, Citation32, Citation33, Citation40], although the difference was statistically significant only in two studies [Citation33, Citation40]. Pooled results showed that PDW values were similar in the two groups (WMD = 0.19%, 95% CI −0.47 to 0.85%; p = 0.58; statistical power = 0.40), with an extreme heterogeneity between studies (I2=89.4%; p < 0.001). Sensitivity analysis showed that the corresponding pooled WMD values were not affected when each study was in turn removed (effect size ranged between −0.08 and 0.38%, ). There was no significant publication bias using the Begg's (p = 0.917) or Egger's t-test (p = 0.848). As shown in , there were no significant differences (t=-1.98; p = 0.08) in pooled WMD values between European (WMD=-0.46%, 95% CI −0.91 to −0.01%; p = 0.043) and Asian studies (WMD = 0.03%, 95% CI −0.72 to 0.77%; p = 0.95), although the former reported significant differences in pooled PDW values between stable COPD and non-COPD subjects. Furthermore, virtually no heterogeneity was observed in European studies (I2=0.0%; p = 0.64). In meta-regression analysis, age (t=-1.62; p = 0.15), gender (t=-0.75; p = 0.48), publication year (t=-0.61; p = 0.56), and WBC (t = 1.68; p = 0.14) were not significantly associated with WMD. Other parameters (BMI, FEV1, FEV1/FVC and CRP) could not be assessed because of lack of data.
PLR
Four studies, published between 2016 and 2019, assessed the PLR in 379 stable COPD patients (mean age 64 years, 75% males) and 255 non-COPD subjects (mean age 63 years, 69% males) () [Citation32, Citation38–40]. The GOLD guidelines were used in all studies. The forest plot of PLR values in the two groups is shown in . In all studies, stable COPD patients had significantly higher PLR values compared to non-COPD subjects (mean difference range, 15 to 185). Pooled results showed that PLR values were significantly higher in stable COPD patients (WMD = 59.52, 95% CI 29.59 to 89.44; p < 0.001; statistical power = 1), with an extreme heterogeneity between studies (I2=95.6%; p < 0.001). Sensitivity analysis showed that the direction of WMD values was not influenced when each study was in turn removed (effect size ranged between 35 and 74, ) even though the study by Karadeniz et al seemed to have a tangible impact on effect size [Citation32]. Albeit attenuated, the WMD remained significant after removing this study (WMD = 35.38, 95% CI 10.58 to 60.16; p < 0.001; I2=94.6%; p < 0.001). The assessment of publication bias and meta-regression analysis could not be performed because of the relatively small number of studies.
Stable COPD vs. AECOPD patients
Platelet count
Twelve studies, published between 2007 and 2020, evaluated the platelet count in 1,138 stable COPD (mean age 69 years, 62% males) and 732 AECOPD patients (mean age 68 years, 61% males) () [Citation20, Citation22, Citation25, Citation27–30, Citation32, Citation34, Citation40, Citation44, Citation45]. The GOLD guidelines were used in nine studies [Citation25, Citation27–30, Citation32, Citation34, Citation40, Citation44], the ATS/ERS guidelines in two [Citation20, Citation22], whilst no information was provided in the remaining study [Citation45]. The forest plot of platelet counts in stable COPD and AECOPD patients is shown in . In three studies, AECOPD patients had lower platelet counts compared to stable COPD patients (mean difference range, −32 to −8 × 109/L) [Citation25, Citation34, Citation40], although the difference was statistically significant only in one study [Citation25]. In nine studies, AECOPD patients had higher platelet counts (mean difference range, 5 to 60 × 109/L) [Citation20, Citation22, Citation27–30, Citation32, Citation44, Citation45], however the difference was statistically significant only in two studies [Citation28, Citation45]. Random-effect models were used because of the substantial heterogeneity between studies (I2=76.8%; p < 0.001). Overall, pooled results showed that platelet counts were non-significantly higher in patients with AECOPD (WMD = 12.02 × 109/L, 95% CI −4.55 to 28.58 × 109/L; p = 0.16; statistical power = 0.38). In sensitivity analysis, the effect size was not influenced when each study was in turn removed (effect size ranged between 5.4 and 16.2 × 109/L, ). The Begg's (p = 0.373) and Egger's (p = 0.754) t-tests showed no significant evidence of publication bias. In sub-group analysis, there were no significant differences (t=-0.49; p = 0.64) in pooled WMD values between European (WMD = 14.69 × 109/L, 95% CI −4.08 to 33.45 × 109/L; p = 0.067) and Asian studies (WMD = 8.35 × 109/L, 95% CI −18.11 to 34.81 × 109/L; p = 0.54, ) although the former showed a trend toward a significant difference between COPD and AECOPD patients. A relatively lower heterogeneity was observed in European studies (I2=43.4%; p = 0.116) when compared to Asian studies (I2=87.0%; p < 0.001). In meta-regression analysis, age (t = 0.78; p = 0.45), gender (t = 0.21; p = 0.84), publication year (t=-1.25; p = 0.24), BMI (t=-0.19; p = 0.85), diagnostic guideline (t = 1.63; p = 0.13), FEV1/FVC (t = 0.43; p = 0.69), WBC (t = 0.05; p = 0.97), and CRP (t=-0.63; p = 0.55) were not significantly associated with the WMD. By contrast, FEV1 was significantly associated with the effect size (t = 2.58; p = 0.042).
MPV
Ten studies, published between 2012 and 2020, reported MPV values in 1,094 stable COPD (mean age 69 years, 62% males) and 747 AECOPD patients (mean age 67 years, 61% males) () [Citation25, Citation27–30, Citation32, Citation34, Citation36, Citation40, Citation44]. The GOLD guidelines were used in all studies. The forest plot of MPV values in stable COPD and AECOPD patients is shown in . In seven studies, AECOPD patients had significantly lower MPV values than stable COPD subjects (mean difference range, −1.20 to −0.30 fL) [Citation25, Citation27, Citation28, Citation30, Citation32, Citation40, Citation44]. In three studies, AECOPD patients had higher MPV values (mean difference range, 0.20 to 1.10 fL) [Citation29, Citation34, Citation36], with non-significant differences in one study [Citation29]. Overall, pooled results showed a trend toward significantly lower MPV values in AECOPD patients (WMD=-0.38 fL, 95% CI −0.81 to 0.05 fL; p = 0.084; statistical power = 0.96), with extreme heterogeneity between studies (I2=93.1%; p < 0.001). Sensitivity analysis showed that the corresponding pooled WMD values were not altered when each study was in turn removed (effect size ranged between −0.55 and −0.29 fL, ) even though the funnel plot in Supplementary Figure 10 indicates a possible distortive effect of one study [Citation29]. After removing this study, pooled results showed that the MPV values were significantly lower in patients with AECOPD (WMD =-0.55 fL, 95% CI −0.90 to −0.20 fL; p = 0.002) but still with extreme heterogeneity (I2=88.7%; p < 0.001). No significant publication bias was detected with the Begg's (p = 0.917) and Egger's (p = 0.561) t-tests. No differences in effect size, or changes in heterogeneity, were observed between European and Asian studies. In meta-regression analysis, age (t = 0.11; p = 0.92), gender (t=-0.38; p = 0.71), publication year (t=-0.12; p = 0.91), and WBC (t=-0.39; p = 0.71) were not significantly associated with WMD. Other parameters (BMI, FEV1, FEV1/FVC and CRP) could not be assessed because of the lack of data.
PDW
Four studies, published between 2014 and 2019, evaluated the PDW in 371 AECOPD (mean age 65 years, 55% males) and 438 stable COPD patients (mean age 64 years, 59% males) () [Citation28, Citation29, Citation32, Citation40]. The GOLD guidelines were used in all studies. The forest plot for PDW values in stable COPD and AECOPD patients is shown in . In all studies, AECOPD patients had higher PDW values compared to controls (mean difference range, 0.10 to 2.40%) although the difference was statistically significant only in one study [Citation32]. Pooled results showed that PDW values were similar in the two groups (WMD = 0.83%, 95% CI −0.27 to 1.94%; p = 0.14; statistical power = 0.55), with extreme heterogeneity between studies (I2=87.3%; p < 0.001). Sensitivity analysis showed that the direction of pooled WMD values was not altered when each study was in turn omitted (effect size ranged between 0.14 and 1.13%, Supplementary Figure 11) even though the study of Karadeniz et al seemed to exert an important effect on the effect size [Citation32]. After removing this study, the PDW values remained similar in the two groups (WMD = 0.14%, 95% CI −0.14 to 0.41; p = 0.33), with virtual disappearance of between-study heterogeneity (I2=0.00%, p = 0.76). Assessment of publication bias and meta-regression analysis was not possible because of the small number of studies.
PLR
Three studies, published between 2016 and 2019, investigated the PLR in 290 AECOPD (mean age 64 years, 54% males) and 270 stable COPD patients (mean age 63 years, 53% males) () [Citation32, Citation38, Citation40]. The GOLD guidelines were used in all studies. The forest plot for PLR values in AECOPD and stable COPD patients is shown in . In all studies, AECOPD patients had higher PLR values compared to stable COPD subjects (mean difference range, 23 to 69), and in two the difference was statistically significant [Citation38, Citation40]. Pooled results showed that the PLR values were significantly higher in AECOPD patients (WMD = 46.03, 95% CI 7.70 to 84.35; p = 0.02; statistical power = 0.95), with extreme heterogeneity between studies (I2=83.5%; p = 0.002). Sensitivity analysis showed that the direction of WMD was not altered when each study was in turn removed (effect size ranged between 23 and 68). Assessment of publication bias and meta-regression analysis was not possible because of the small number of studies.
Discussion
In our systematic review and meta-analysis, significant differences in specific platelet indices were observed between patients with stable COPD, those with AECOPD, and non-COPD subjects. In particular, patients with stable COPD had significantly higher platelet counts and PLR values when compared with non-COPD controls whereas AECOPD patients had significantly higher PLR values when compared with patients with stable COPD. By contrast, no significant between-group differences were observed with either MPV or PDW. Despite the extreme between-study heterogeneity observed for each of the studied platelet markers sensitivity analysis showed that the overall effect size was not influenced when individual studies were removed. Furthermore, no evidence of publication bias was observed. A number of clinical and demographic parameters, e.g. age, gender, BMI, publication year, guidelines used for diagnosis, continent where the study was conducted, FEV1, FEV1/FVC, WBC and C- CRP failed to show significant associations with the WMD for each platelet index, barring a significant association between FEV1 and platelet count WMD observed in studies comparing stable COPD and AECOPD patients.
There is increasing evidence that platelets, in addition to their well-established role in the regulation of hemostasis and coagulation, exert significant effects on inflammation and immunity control mechanisms through the release of specific chemicals and microparticles [Citation46–48]. Furthermore, megakaryocytes, the platelet precursors, can travel from the bone marrow to the lungs, where they are primarily trapped in the pulmonary capillaries and the interstitium [Citation49]. As a result, the lung can account for up to 50% of the total platelet production from locally residing megakaryocytes [Citation49]. The potential detrimental effects of alterations in platelet function on the respiratory system include the loss of tissue elasticity and structural integrity of the alveoli, the presence of a sustained prothrombotic state with consequent pulmonary vascular remodeling, and the dysregulation of key hypoxia signaling pathways [Citation50]. For example, there is evidence from animal studies that the human platelet factor 4 can significantly increase the activity of elastase in leukocytes, with a consequent reduction in lung elastin content of approximately 20%, loss of lung elasticity, and morphological changes indicating emphysema [Citation51]. Studies have also shown increased platelet activation in patients with stable COPD when compared to non-COPD controls, with consequent release of inflammatory mediators and formation of platelet-monocyte aggregates [Citation22]. Notably, AECOPD patients exhibit an even more pronounced platelet activation when compared to patients with stable COPD [Citation5]. The release of specific mediators, e.g. through the platelet-derived growth factor signaling pathway, might contribute to the development of pulmonary vascular remodeling and pulmonary hypertension in COPD and other respiratory diseases [Citation52]. Finally, the increased expression of the hypoxia inducible factor-2α and the synthesis of plasminogen activator inhibitor-1 by platelets, particularly in the presence of hypoxia, is also able to trigger a pro-thrombotic and pro-inflammatory state, with negative consequences on vascular remodeling and lung function [Citation6, Citation53, Citation54]. While the direct evaluation of these mediators and signaling pathways is not practical in the routine clinical setting a number of platelet indices have been developed to investigate platelet homeostasis and function in health and disease. The MPV, originally used for the investigation of thrombocytopenia, has been shown to be associated with clinical outcomes in several conditions. In particular, an increase in MPV has been linked with platelet activation, altered thrombotic homeostasis, and arterial and venous occlusive events [Citation7]. Similarly, an increased PDW has been proposed as a marker of platelet activation, coagulation, and vaso-occlusive events [Citation8], whereas the PLR has been shown to accurately reflect the shifts in platelet and lymphocyte counts that occur during inflammatory and pro-thrombotic states in the setting of various autoimmune conditions and other systemic inflammatory states, e.g. cancer [Citation9, Citation55–57]. In these studies, the PLR has been shown to significantly correlate with the degree of systemic inflammation, the severity of the underlying condition, the clinical outcomes, and the possible response to pharmacological treatment [Citation9]. The results of our systematic review and meta-analysis showed that the PLR is a particularly promising platelet index in COPD in terms of diagnostic accuracy and disease worsening. Albeit in a relatively small number of studies, stable COPD patients had higher PLR values than non-COPD subjects and AECOPD patients had higher PLR values than stable COPD patients. This suggests an increasing systemic inflammatory burden, captured by the PLR, with the development of COPD and its exacerbations. However, the cross-sectional nature of the studies identified warrants further investigations regarding the additional diagnostic value and the clinical significance of the temporal variations of the PLR in this patient group. It is also important to highlight that the forest plots of the PDW between AECOPD and stable COPD patients and of the PLR between stable COPD patients and non-COPD subjects and between AECOPD and stable COPD patients were derived from a relatively limited number of studies, mostly by the same research group, which might introduce another source of bias. In our meta-analysis, both the MPV and the PDW failed to significantly discriminate between stable COPD patients and non-COPD controls and between stable COPD and AECOPD patients. In particular, the results obtained for the MPV are in agreement with those of a recent systematic review and meta-analysis that specifically investigated this platelet index [Citation58]. Furthermore, the platelet count was able to discriminate between stable COPD patients and non-COPD controls but not between stable COPD and AECOPD patients. This is at odds with the results of a study that reported that thrombocytosis, rather than platelet count, was significantly associated with AECOPD [Citation59].
The extreme between-study heterogeneity observed limits the generalizability of the findings. However, it should also be emphasized that the overall effect size, in terms of WMD for each of the indices assessed and the comparisons performed (stable COPD vs. non-COPD subjects and AECOPD vs. stable COPD patients), was not significantly influenced in sensitivity analysis. Furthermore, we did not observe any evidence of publication bias. Meta-regression analysis did not lead to the identification of additional sources of heterogeneity, except for the FEV1 in studies investigating the platelet count in stable COPD and AECOPD patients. It is possible that other, not adequately reported, factors that are strongly associated with the presence and the severity of COPD might have been responsible, at least in part, for the reported heterogeneity. Two such factors are smoking status and atherosclerotic cardiovascular disease, which are well-known to increase platelet activity and aggregation and alter per se the MPV, PDW and PLR [Citation60–63]. Another potential source of heterogeneity is related to the lack of standardization of the methods and techniques used for assessing the platelet count and the related indices. This includes differences in venipuncture techniques, type of anticoagulant used, storage temperature, period between sampling and analysis, and type of automated analyzer used [Citation7, Citation60].
Pending confirmation in large prospective studies, the use of platelet indices might be useful to further improve diagnostic accuracy and risk stratification in COPD. Furthermore, it might lead to the identification of novel therapeutic targets in this patient group. This proposition is supported by the results of a systematic review and meta-analysis of five studies in 11,117 patients with COPD, including 3,069 with AECOPD. In this study, the use of antiplatelet agents was associated with a significant reduction in all-cause mortality (odds ratio 0.81, 95% CI 0.75 to 0.88). Notably, the beneficial effects of this class of drugs were independent of AECOPD, ischemic heart disease, use of cardiovascular drugs, and cardiovascular risk factors [Citation64]. In conclusion, our systematic review and meta-analysis has shown that specific platelet indices, particularly the PLR, might assist with COPD diagnosis and risk stratification. Larger prospective studies, ideally following rigorous and consistent procedures for sample collection and analysis, are now required to support the clinical use of platelet indices for the management of patients with COPD.
Authorship contribution
Initial idea: AZ, PP, AGF, CC, AAM; Data collection: AZ, ES, SM; Data analysis: AZ, AAM; Data interpretation: AZ, PP, AGF, CC, AAM; Writing - first draft: AAM; Writing - Review & Editing, AZ, PP, ES, SM, AGF, CC, AAM.
Declaration of interest
No potential conflict of interest was reported by the authors.
Data availability
The data that support the findings of this systematic review and meta-analysis are available from the corresponding author, AZ, upon reasonable request.
Additional information
Funding
References
- Manne BK, Xiang SC, Rondina MT. Platelet secretion in inflammatory and infectious diseases. Platelets. 2017;28(2):155–164. DOI:https://doi.org/10.1080/09537104.2016.1240766
- Rayes J, Bourne JH, Brill A, et al. The dual role of platelet-innate immune cell interactions in thrombo-inflammation. Res Pract Thromb Haemost. 2020;4(1):23–35. DOI:https://doi.org/10.1002/rth2.12266
- Rabe KF, Watz H. Chronic obstructive pulmonary disease. Lancet. 2017;389(10082):1931–1940. DOI:https://doi.org/10.1016/S0140-6736(17)31222-9
- Robert L, Labat-Robert J. Platelets, micro-particles and elastase. a review with extrapolation to the mechanism of generation and bio-pathology of platelet fragments. Pathol Oncol Res. 2017;23(2):455–458. DOI:https://doi.org/10.1007/s12253-017-0199-1
- Munoz-Esquerre M, Ferreiro JL, Huertas D, et al. Impact of acute exacerbations on platelet reactivity in chronic obstructive pulmonary disease patients. Int J Chron Obstruct Pulmon Dis. 2018;13:141–148. DOI:https://doi.org/10.2147/COPD.S152660
- Xu X, Wang H, Wang Z, et al. Plasminogen activator inhibitor-1 promotes inflammatory process induced by cigarette smoke extraction or lipopolysaccharides in alveolar epithelial cells. Exp Lung Res. 2009;35(9):795–805. DOI:https://doi.org/10.3109/01902140902912519
- Noris P, Melazzini F, Balduini CL. New roles for mean platelet volume measurement in the clinical practice? Platelets. 2016;27(7):607–612. DOI:https://doi.org/10.1080/09537104.2016.1224828
- Vagdatli E, Gounari E, Lazaridou E, et al. Platelet distribution width: a simple, practical and specific marker of activation of coagulation. Hippokratia. 2010;14(1):28–32.
- Gasparyan AY, Ayvazyan L, Mukanova U, et al. The platelet-to-lymphocyte ratio as an inflammatory marker in rheumatic diseases. Ann Lab Med. 2019;39(4):345–357. DOI:https://doi.org/10.3343/alm.2019.39.4.345
- Wells GA, Shea B, O’Connell D, et al. The Newcastle-Ottawa Scale (NOS) for assessing the quality of nonrandomised studies in meta-analyses. The Ottawa Hospital Research Institute 2013. Available at http://www.ohri.ca/programs/clinical_epidemiology/oxford.asp.
- Wan X, Wang W, Liu J, et al. Estimating the sample mean and standard deviation from the sample size, median, range and/or interquartile range. BMC Med Res Methodol. 2014;14:135. DOI:https://doi.org/10.1186/1471-2288-14-135
- Hozo SP, Djulbegovic B, Hozo I. Estimating the mean and variance from the median, range, and the size of a sample. BMC Med Res Methodol. 2005;5(1):13. DOI:https://doi.org/10.1186/1471-2288-5-13
- Bowden J, Tierney JF, Copas AJ, et al. Quantifying, displaying and accounting for heterogeneity in the meta-analysis of RCTs using standard and generalised Q statistics. BMC Med Res Methodol. 2011;11:41. DOI:https://doi.org/10.1186/1471-2288-11-41
- Higgins JP, Thompson SG. Quantifying heterogeneity in a meta-analysis. Stat Med. 2002;21(11):1539–1558. DOI:https://doi.org/10.1002/sim.1186
- Tobias A. Assessing the influence of a single study in the meta-analysis estimate. Stata Tech Bull. 1999;47:15–17.
- Begg CB, Mazumdar M. Operating characteristics of a rank correlation test for publication bias. Biometrics. 1994;50(4):1088–1101. DOI:https://doi.org/10.2307/2533446
- Sterne JA, Egger M. Funnel plots for detecting bias in meta-analysis: guidelines on choice of axis. J Clin Epidemiol. 2001;54(10):1046–1055. DOI:https://doi.org/10.1016/S0895-4356(01)00377-8
- Liberati A, Altman DG, Tetzlaff J, et al. The PRISMA statement for reporting systematic reviews and meta-analyses of studies that evaluate healthcare interventions: explanation and elaboration. BMJ. 2009;339:b2700. DOI:https://doi.org/10.1136/bmj.b2700
- Sin DD, Man SF. Why are patients with chronic obstructive pulmonary disease at increased risk of cardiovascular diseases? The potential role of systemic inflammation in chronic obstructive pulmonary disease. Circulation. 2003;107(11):1514–1519. DOI:https://doi.org/10.1161/01.cir.0000056767.69054.b3
- Valipour A, Schreder M, Wolzt M, et al. Circulating vascular endothelial growth factor and systemic inflammatory markers in patients with stable and exacerbated chronic obstructive pulmonary disease. Clin Sci. 2008;115(7):225–232. (Lond). DOI:https://doi.org/10.1042/CS20070382
- Biljak VR, Pancirov D, Cepelak I, et al. Platelet count, mean platelet volume and smoking status in stable chronic obstructive pulmonary disease. Platelets. 2011;22(6):466–470. DOI:https://doi.org/10.3109/09537104.2011.573887
- Maclay JD, McAllister DA, Johnston S, et al. Increased platelet activation in patients with stable and acute exacerbation of COPD. Thorax. 2011;66(9):769–774. DOI:https://doi.org/10.1136/thx.2010.157529
- Steiropoulos P, Papanas N, Nena E, et al. Mean platelet volume and platelet distribution width in patients with chronic obstructive pulmonary disease: the role of comorbidities. Angiology. 2013;64(7):535–539. DOI:https://doi.org/10.1177/0003319712461436
- Stoll P, Wuertemberger U, Bratke K, et al. Stage-dependent association of BDNF and TGF-β1 with lung function in stable COPD. Respir Res. 2012;13:116. DOI:https://doi.org/10.1186/1465-9921-13-116
- Ulasli SS, Ozyurek BA, Yilmaz EB, et al. Mean platelet volume as an inflammatory marker in acute exacerbation of chronic obstructive pulmonary disease. Pol Arch Med Wewn. 2012;122(6):284–290. DOI:https://doi.org/10.20452/pamw.1284
- Wang R, Cao Z, Li Y, et al. Utility of N-terminal pro B-type natriuretic peptide and mean platelet volume in differentiating congestive heart failure from chronic obstructive pulmonary disease. Int J Cardiol. 2013;170(2):e28–e29. DOI:https://doi.org/10.1016/j.ijcard.2013.10.048
- Wang RT, Li JY, Cao ZG, et al. Mean platelet volume is decreased during an acute exacerbation of chronic obstructive pulmonary disease. Respirology. 2013;18(8):1244–1248. DOI:https://doi.org/10.1111/resp.12143
- Günay E, Sarınç Ulaşlı S, Akar O, et al. Neutrophil-to-lymphocyte ratio in chronic obstructive pulmonary disease: a retrospective study. Inflammation. 2014;37(2):374–380. DOI:https://doi.org/10.1007/s10753-013-9749-1
- Zhang M, Li Y, Zhang J, et al. Mean platelet volume is elevated in exacerbated and convalescent COPD patients. Clin Chim Acta. 2015;451(Pt B):227–231. DOI:https://doi.org/10.1016/j.cca.2015.10.001
- Agapakis DI, Massa EV, Hantzis I, et al. The role of mean platelet volume in chronic obstructive pulmonary disease exacerbation. Respir Care. 2016;61(1):44–49. DOI:https://doi.org/10.4187/respcare.04132
- Demir M, Acet H, Kaya H, et al. Relationship between metabolic syndrome and epicardial fat tissue thickness in patients with chronic obstructive pulmonary disease. Anatol J Cardiol. 2016;16(6):405–411. DOI:https://doi.org/10.14744/AnatolJCardiol.2015.6566
- Karadeniz G, Aktoğu S, Erer OF, et al. Predictive value of platelet-to-lymphocyte ratio in exacerbation of chronic obstructive pulmonary disease. Biomark Med. 2016;10(7):701–710. DOI:https://doi.org/10.2217/bmm-2016-0046
- Makhlouf HA, Sadek SH, Nafady AAH. Platelet function in diabetic and nondiabetic patients with chronic obstructive pulmonary disease: a case control study. Clin Respir J. 2018;12(1):48–56. DOI:https://doi.org/10.1111/crj.12477
- Malerba M, Nardin M, Radaeli A, et al. The potential role of endothelial dysfunction and platelet activation in the development of thrombotic risk in COPD patients. Expert Rev Hematol. 2017;10(9):821–832. DOI:https://doi.org/10.1080/17474086.2017.1353416
- Aleva FE, Temba G, de Mast Q, et al. Increased platelet-monocyte interaction in stable COPD in the absence of platelet hyper-reactivity. Respiration. 2018;95(1):35–43. DOI:https://doi.org/10.1159/000480457
- Farah R, Ibrahim R, Nassar M, et al. The neutrophil/lymphocyte ratio is a better addition to C-reactive protein than CD64 index as a marker for infection in COPD. Panminerva Med. 2017;59(3):203–209.
- Guang Y, Jie Z, Feng D, et al. Surrogate scale for evaluating respiratory function based on complete blood count parameters. J Clin Lab Anal. 2018;32(5):e22385. DOI:https://doi.org/10.1002/jcla.22385
- El-Gazzar AG, Kamel MH, Elbahnasy OKM, et al. Prognostic value of platelet and neutrophil to lymphocyte ratio in COPD patients. Expert Rev Respir Med. 2020;14(1):111–116. DOI:https://doi.org/10.1080/17476348.2019.1675517
- Hlapcic I, Somborac-Bacura A, Popovic-Grle S, et al. Platelet indices in stable chronic obstructive pulmonary disease - association with inflammatory markers, comorbidities and therapy. Biochem Med (Zagreb). 2020;30(1):010701.
- Şahin F, Koşar AF, Aslan AF, et al. Serum biomarkers in patients with stable and acute exacerbation of chronic obstructive pulmonary disease: A comparative study. J Med Biochem. 2019;38(4):503–511. DOI:https://doi.org/10.2478/jomb-2018-0050
- Skoczynski S, Krzyzak D, Studnicka A, et al. Chronic obstructive pulmonary disease and platelet count. Adv Exp Med Biol. 2019;1160:19–23.
- Cilingir BM, Sunnetcioglu A. Platelet distribution with is a usable parameter in chronic obstructive pulmonary disease severity. Eastern J Med. 2020;25(1):97–102. DOI:https://doi.org/10.5505/ejm.2020.24572
- Crisafulli E, Cilloniz C, Liapikou A, et al. Systemic inflammatory response and outcomes in community-acquired pneumonia patients categorized according to the smoking habit or presence of chronic obstructive pulmonary disease. JCM. 2020;9(9):2884. DOI:https://doi.org/10.3390/jcm9092884
- Zouaoui Boudjeltia K, Kotsalos C, de Sousa DR, et al. Spherization of red blood cells and platelet margination in COPD patients. Ann N Y Acad Sci. 2021;1485(1):71–82. DOI:https://doi.org/10.1111/nyas.14489
- De Castro J, Hernandez-Hernandez A, Rodriguez MC, et al. Comparison of changes in erythrocyte and platelet phospholipid and fatty acid composition and protein oxidation in chronic obstructive pulmonary disease and asthma. Platelets. 2007;18(1):43–51. DOI:https://doi.org/10.1080/09537100600800776
- Eriksson O, Mohlin C, Nilsson B, et al. The human platelet as an innate immune cell: interactions between activated platelets and the complement system. Front Immunol. 2019;10:1590.
- Melki I, Tessandier N, Zufferey A, et al. Platelet microvesicles in health and disease. Platelets. 2017;28(3):214–221. DOI:https://doi.org/10.1080/09537104.2016.1265924
- Sprague DL, Sowa JM, Elzey BD, et al. The role of platelet CD154 in the modulation in adaptive immunity. Immunol Res. 2007;39(1–3):185–193. DOI:https://doi.org/10.1007/s12026-007-0074-3
- Lefrancais E, Looney MR. Platelet Biogenesis in the Lung Circulation. Physiology (Bethesda). 2019;34(6):392–401. DOI:https://doi.org/10.1152/physiol.00017.2019
- Mallah H, Ball S, Sekhon J, et al. Platelets in chronic obstructive pulmonary disease: An update on pathophysiology and implications for antiplatelet therapy. Respir Med. 2020;171:106098. DOI:https://doi.org/10.1016/j.rmed.2020.106098
- Lonky SA, Wohl H. Stimulation of human leukocyte elastase by platelet factor 4. Physiologic, morphologic, and biochemical effects on hamster lungs in vitro. J Clin Invest. 1981;67(3):817–826. DOI:https://doi.org/10.1172/JCI110099
- Wu K, Tang H, Lin R, et al. Endothelial platelet-derived growth factor-mediated activation of smooth muscle platelet-derived growth factor receptors in pulmonary arterial hypertension. Pulm Circ. 2020;10(3):2045894020948470. DOI:https://doi.org/10.1177/2045894020948470
- Chaurasia SN, Kushwaha G, Kulkarni PP, et al. Platelet HIF-2α promotes thrombogenicity through PAI-1 synthesis and extracellular vesicle release. Haematologica. 2019;104(12):2482–2492. DOI:https://doi.org/10.3324/haematol.2019.217463
- Wang H, Yang T, Li D, et al. Elevated circulating PAI-1 levels are related to lung function decline, systemic inflammation, and small airway obstruction in chronic obstructive pulmonary disease. COPD. 2016;11:2369–2376. DOI:https://doi.org/10.2147/COPD.S107409
- Tian C, Song W, Tian X, et al. Prognostic significance of platelet-to-lymphocyte ratio in patients with ovarian cancer: a meta-analysis. Eur J Clin Invest. 2018;48(5):e12917. DOI:https://doi.org/10.1111/eci.12917
- Erre GL, Buscetta G, Mangoni AA, et al. Diagnostic accuracy of different blood cells-derived indexes in rheumatoid arthritis: a cross-sectional study. Med (Baltimore). 2020;99(44):e22557. DOI:https://doi.org/10.1097/MD.0000000000022557
- Paliogiannis P, Satta R, Deligia G, et al. Associations between the neutrophil-to-lymphocyte and the platelet-to-lymphocyte ratios and the presence and severity of psoriasis: a systematic review and meta-analysis. Clin Exp Med. 2019;19(1):37–45. DOI:https://doi.org/10.1007/s10238-018-0538-x
- Ma Y, Zong D, Zhan Z, et al. Feasibility of mean platelet volume as a biomarker for chronic obstructive pulmonary disease: a systematic review and meta-analysis. J Int Med Res. 2019;47(12):5937–5949. DOI:https://doi.org/10.1177/0300060519887886
- Fawzy A, Putcha N, Paulin LM, SPIROMICS and COPDGene Investigators, et al. Association of thrombocytosis with COPD morbidity: the SPIROMICS and COPDGene cohorts. Respir Res. 2018;19(1):20. DOI:https://doi.org/10.1186/s12931-018-0717-z
- Korniluk A, Koper-Lenkiewicz OM, Kaminska J, et al. Mean Platelet Volume (MPV): new perspectives for an old marker in the course and prognosis of inflammatory conditions. Mediators Inflamm. 2019;2019:9213074. DOI:https://doi.org/10.1155/2019/9213074
- Pujani M, Chauhan V, Singh K, et al. The effect and correlation of smoking with platelet indices, neutrophil lymphocyte ratio and platelet lymphocyte ratio. Hematol Transfus Cell Ther. 2020. DOI:https://doi.org/10.1016/j.htct.2020.07.006.
- Corriere T, Di Marca S, Cataudella E, et al. Neutrophil-to-Lymphocyte Ratio is a strong predictor of atherosclerotic carotid plaques in older adults. Nutr Metab Cardiovasc Dis. 2018;28(1):23–27. DOI:https://doi.org/10.1016/j.numecd.2017.10.022
- Reddy SK, Shetty R, Marupuru S, et al. Significance of platelet volume indices in STEMI Patients: a case-control study. J Clin Diagn Res. 2017;11(4):LC05–LC07.
- Pavasini R, Biscaglia S, d'Ascenzo F, et al. Antiplatelet treatment reduces all-cause mortality in COPD Patients: a systematic review and meta-analysis. COPD. 2016;13(4):509–514. DOI:https://doi.org/10.3109/15412555.2015.1099620