Abstract
Chronic Obstructive Pulmonary Disease (COPD) is a prevalent condition that poses a significant burden on individuals and society due to its high morbidity and mortality rates. The diaphragm is the main respiratory muscle, its function has a direct impact on the quality of life and prognosis of COPD patients. This article aims to review the structural measurement and functional evaluation methods through the use of diaphragmatic ultrasound and relevant research on its application in clinical practice for COPD patients. Thus, it serves to provide valuable insights for clinical monitoring of diaphragm function in COPD patients, facilitating early clinical intervention and aiding in the recovery of diaphragm function.
1. Background
Chronic obstructive pulmonary disease (COPD) is a prevalent condition characterized by persistent airflow limitation. It has a global prevalence of 10.3% (95% CI 8.2–12.8) [Citation1]. Currently, COPD ranks among the top three causes of death worldwide [Citation2]. With the aging population, the prevalence of COPD is projected to rise. Due to the high prevalence, morbidity, and mortality rates associated with COPD, a significant burden is placed on both families and society. Unfortunately, prevention and treatment options for COPD are currently facing a challenge [Citation3].
The primary clinical symptoms of COPD include dyspnea and reduced activity tolerance, with decreased respiratory muscle function being a leading cause of dyspnea in COPD patients [Citation4]. The diaphragm is the primary inspiratory muscle, responsible for 60–80% of all respiratory muscle function [Citation5]. As a result, it significantly impacts the quality of life and prognosis of patients. The diaphragm is a muscular structure that separates the thoracic cavity from the abdominal cavity. It is composed of all 3 muscle fiber types, and has a high percentage of fatigue-resistant muscle fibers [Citation6]. It is impacted by various factors such as weight, nerves, metabolism, and muscles [Citation7–9]. Patients with COPD experience pathophysiological changes due to factors, such as airflow obstruction, increased airway resistance, and lung hyperinflation. These changes can lead to diaphragm shortening, fiber contractile protein consumption, and oxidative stress, which can affect the structure, metabolism, and function of the diaphragm [Citation10–13].
At present, the detection of the diaphragm includes lung function tests, chest X-ray, CT, MRI, ultrasound, pressure detection, electromyographic examination, and so forth [Citation14].
Compared to other examinations, such as CT, diaphragmatic ultrasonography (US) has become a popular research tool because of its ability to noninvasively infer the diaphragmatic muscle mass [Citation15], represent residual work capacity, and predict the response to noninvasive ventilation (NIV) [Citation16] and mechanical ventilation. In this article, the ultrasound evaluation method of the diaphragm and the application of diaphragm ultrasound in COPD are reviewed.
2. Overview of diaphragmatic ultrasound
Ultrasonography (US) has been extensively researched and proven to be superior in assessing diaphragmatic function. Its reliability, validity, and reproducibility have also been recognized by some scholars [Citation17,Citation18]. Currently, different methods of ultrasonic evaluation of the diaphragm have been proposed including, measuring diaphragm thickness (DT) and mobility using 2D B-mode or M-mode. The following section describes the methods used to assess diaphragmatic function.
2.1. Diaphragm thickness and diaphragm thickening fraction
To measure diaphragm thickness (DT) and thickening fraction (TF), a high-frequency linear-array probe is used while the patient is in a supine or semi-recumbent position. The ultrasound probe is placed longitudinally parallel to the long axis of the body and perpendicular to the chest wall, typically between the eighth and tenth intercostal space, at the anterior axillary line or midway between the anterior and mid-axillary lines. The image displays three parallel hyperechoic layers. The upper and lower hyperechoic layers correspond to the pleura and peritoneal membranes while the middle hypoechoic layers represent the diaphragm (shown in ).
Figure 1. Diaphragm. A high-frequency linear array probe is utilized to identify the diaphragm located between the eighth to tenth intercostal space. The resulting image typically displays three parallel high and low echo bands, with the upper and lower layers representing the pleural and peritoneal membranes, respectively, and the diaphragm located in the middle.
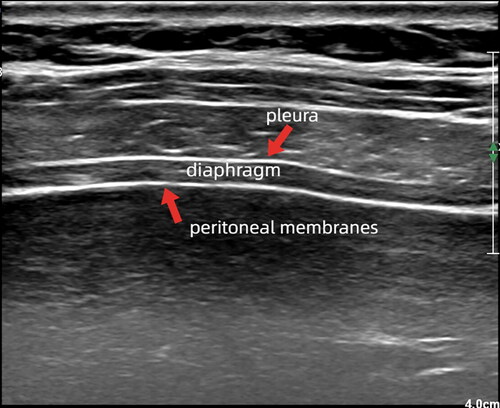
Ultrasound provides a noninvasive assessment of DT [Citation19]. The DT can be obtained by measuring the distance between the two hyperechoic layers directly from the frozen B-mode images or M-mode images. The accuracy of US measurement of DT has been demonstrated [Citation20,Citation21]. In most studies, the right and left DTs have been measured as the distance from the middle of the pleural membrane to the middle of the peritoneal membrane. Alternatively, some studies have proposed measuring from the inner edges or the outer edges of the pleural and peritoneal membranes [Citation22]. This article recommends measuring the inner-inner distance for DT, since values are fractions of a millimeter.
Researchers suggest using M-mode for measuring DT because it allows accurate detection of respiratory cycle variations and acquisition of end-expiration and end-inspiration measurements [Citation23]. Diaphragmatic function has been related to inspiratory thickeness. So, for better detection of diaphragmatic dysfunction (DD), it is necessary to measure TF. TF can be calculated as follows: TF = (thickness at end inspiration – thickness at end expiration)/thickness at end expiration.
DT increased as lung volume increased, with a more rapid rate of thickening at the higher lung volumes [Citation21]. It is noteworthy that the position of the subject affects the thickness of the diaphragm. Specifically, DT is more than 20% higher in sitting and standing positions compared to the supine position at the end of maximal inspiratory and calm expiration (p < 0.05) [Citation24]. Studies have determined the reference value of right DT in healthy individuals while in a seated position. DT varies depending on the sex and the stage of respiration, not body mass index or thorax circumference [Citation25]. At the end of calm expiration, DT is 0.21 ± 0.04 cm for males and 0.19 ± 0.04 cm for females. At the end of calm inspiration, the thickness is 0.28 ± 0.06 cm for males and 0.25 ± 0.06 cm for females. At the end of maximum inspiration, the thickness is 0.43 ± 0.08 cm for males and 0.39 ± 0.08 cm for females [Citation26]. While a study found that the reference values for supine DT at the end of calm expiration were 0.19 ± 0.04 cm (95% CI 0.17–0.20 cm) for males and 0.14 ± 0.03 cm (95% CI 0.13–0.15 cm) for females (p = 0.01) [Citation25]. It is important to note that although many researchers have come up with reference values for DT, there is no consensus due to the varied characteristics among populations and high individual differences.
Although the impact of COPD on diaphragm function has been established, studies examining DT in COPD patients have yielded mixed results, likely due to the complexity of diaphragmatic function, inconsistent measurement methods, and the lack of reference values. Baria et al. utilized ultrasound to measure DT in 50 COPD patients and 150 healthy controls, and found no significant difference in DT or TF between the two groups [Citation27]. A study conducted by Ogan came to similar conclusions. Additionally, there was no significant difference in DT observed between COPD severity, changes in respiratory function, frequency of exacerbations, and symptom scores (mMRC) [Citation28]. Similarly, a team did not find significant differences in diaphragm thickening rates among patients with different disease severities, symptom severities, and frequencies of COPD attacks. However, the study lacked a control group [Citation29].
Differently, a study including 38 patients with COPD and 30 healthy volunteers with varying degrees of health, indicated that the DT, thickness changes, and TF of COPD patients were lower than those of the healthy volunteers [Citation30]. Similarly, Okura’s research indicates that individuals with COPD have lower DT and TF than healthy individuals during maximum spirometry tests [Citation31]. Although there are controversy surrounding studies on DT in patients with COPD, it continues to be an important parameter for assessing the diaphragm. Further research on DT has the potential to enhance the depth and scope of research content, ultimately enabling a more significant role in clinical applications.
2.2. Diaphragm excursion
To gain diaphragm mobility, a low-frequency (1–5 MHz) convex probe is used while the patient is in a supine or semi-recumbent position. The probe should be placed below the costal margin, between the midclavicular and anterior axillary lines. Using the liver as the acoustic window, the probe points to the medial, cranial, and dorsal side, so that the ultrasound beam reaches the right dome of the diaphragm perpendicularly. The ultrasound image shows the inferior vena cava on the right side of the screen and the gallbladder in the middle. Additionally, the right diaphragm appears as a thick and curved hyperechoic line. Once a good quality B-mode image is obtained, operators should adjust the M-mode interrogation line to be perpendicular to the movement of the hemidiaphragm. Recording the movement of the diaphragm, diaphragm excursion (DE) is the vertical distance from the baseline to the highest point at the end of inspiration and expiration (shown in ). The influence of lung gas and breathing can often make it difficult to use the standard M-mode. In such cases, anatomical motion-mode (AMM) US can be used to improve the situation. Several authors have concluded that AMM provides a more comprehensive record of the mobility of the two hemidiaphragms compared to the standard M-mode [Citation32]. To detect left DE, position the probe under the costal margin or between the anterior axillary line and midaxillary line in the low intercostal space. Use the splenic window to obtain a clear 2D image of the left diaphragm and record in M-mode as described on the right.
Figure 2. Diaphragm excursion. The M-mode is utilized to detect diaphragm movement. And diaphragm excursion refers to the vertical distance from the baseline to the highest point at the end of both inspiration and expiration.
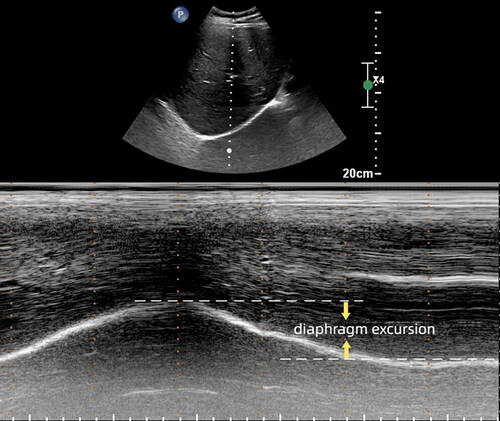
Measuring DE in patients using M-mode has been shown to be a reliable method [Citation33–36]. To objectively quantify DE and DT, at least three images should be evaluated and averaged. It is worth noting that the right diaphragm has the liver as an acoustic window and is less disturbed, so it is more widely used in clinical practice. Additionally, studies have shown that DE varies based on body position, with higher mobility observed in the supine position compared to sitting or standing positions at the same inspiratory volume [Citation37].
While there have been numerous studies conducted on DE, some scholars have established a reference value for DE in the standing position. However, it is worth noting that clinical patients often find it difficult to tolerate this position. Therefore, this article focuses solely on the sitting position. It is important to mention that reference values for the average DE in healthy individuals have been established [Citation38]. During quiet respiration, the right DE is1.9 ± 0.5 cm for males and 1.7 ± 0.4 cm for females. During deep breathing, the right DE is 6.6 ± 1.3 cm for males and 5.4 ± 1.1 cm for females.
Patients with COPD have lower DE than normal due to prolonged air retention [Citation39], a view shared by many scholars [Citation40,Citation41]. Recent studies suggest that DE may be linked to dyspnea scores and 6-m walking experiments in patients with COPD. Consequently, it is believed that DE can play a significant role in exercise tolerance and dyspnea in COPD patients [Citation40]. Ongoing research in this area may lead to the development of a more widely used, accurate, and convenient monitoring method for the diagnosis and prognosis of COPD patients.
2.3. Other methods
2.3.1. Tissue Doppler imaging
Tissue Doppler imaging (TDI) is a widely utilized ultrasound technique used to measure the velocity of myocardial movement. It utilizes the Doppler principle to measure high-amplitude, low-velocity signals of cardiac tissue movement [Citation42]. TDI measurements are both reliable and relatively easy to obtain. As a result, some researchers have utilized TDI to evaluate the right diaphragmatic peak motion velocities in newborns and have found it to be a feasible and reliable method for assessing diaphragm function [Citation43]. Previous studies have utilized TDI to assess both healthy individuals and ICU patients experiencing respiratory failure. These studies have discovered that individuals who experience weaning failure exhibit higher peak contraction and relaxation velocity, as well as higher relaxation rates, when compared to healthy subjects and successfully weaning patients. As a result, TDI has the ability to differentiate between patients who have failed a weaning trial and those who have succeeded, while also displaying a strong correlation with Pdi-derived parameters [Citation44].
Despite the potential benefits of TDI, there are still several unanswered questions surrounding its accuracy in capturing diaphragmatic muscle contraction velocity [Citation45], the need for standardization of the method, and a better understanding of its capabilities and limitations [Citation46]. However, as this technology continues to develop, TDI has the potential to become a reliable, noninvasive, and real-time assessment tool for diaphragm function.
2.3.2. Elastography
Elastography is a novel technique used to measure tissue stiffness and is commonly applied in areas such as the thyroid or breast. When the diaphragm becomes abnormal, its stiffness changes, making elastography a promising, noninvasive method for assessing diaphragm function.
Previous studies have evaluated diaphragmatic shear moduli in healthy volunteers performing submaximal inspiratory tasks at different inspiratory mouth pressure levels. They have shown that the diaphragmatic shear modulus increases with inspiratory mouth pressure [Citation47]. In addition, a study has been conducted on the relationship between the gold standard trans-diaphragmatic pressure (Pdi) and the shear modulus of the diaphragm during the inspiration process of healthy volunteers. The findings suggest that changes in diaphragm stiffness evaluated by shear wave elastography are reflective of changes in PDI [Citation48]. Quentin Fossé conducted a study on 30 ICU patients who were mechanically ventilated. The patients were monitored using ultrasound evaluation and PDI to explore the possibility of its use. The study concluded that there was a correlation between changes in diaphragm shear modulus (ΔSMdi) and changes in PDI (R = 0.45, 95% CI [0.35–0.54], p < 0.001)[Citation49]. There is not enough evidence of potential clinical applications, despite statistically significant correlations. Elastography has been utilized by scholars to assess the diaphragm in both COPD patients and normal individuals. A comparison of the two groups revealed that the diaphragm of COPD patients has a significantly higher shear wave velocity (SWV) at functional residual capacity [Citation50]. CHEN conducted a study using elastography to evaluate the diaphragm and discovered that diaphragm stiffness increases with disease severity in COPD patients and is closely linked to lung function [Citation51].
Elastography is a relatively new technique for assessing the diaphragm, but it faces certain challenges, such as limited sample size and a lack of standardized sampling methods. Despite these obstacles, its potential clinical utility for evaluating diaphragm function and predicting disease prognosis warrants further investigation.
2.3.3. Strain imaging
Strain imaging is a technique that tracks ultrasound speckles over time, allowing for the precise measurement of anatomical movement and deformation. This enables the calculation of tissue displacement, velocity, and deformation in two directions, regardless of the angle between the ultrasonic beam and the direction of tissue excursion [Citation52]. Due to these advantages, strain imaging has proven to be an effective tool for assessing myocardial function. To expand the scope of its application and reap the potential benefits of strain imaging, researchers have utilized this technique on healthy volunteers to evaluate diaphragm function.
Orde et al. found that strain on the right diaphragm aligns with traditional B and M modes in assessing diaphragm function, such as TF and caudal displacement [Citation53]. Moreover, strain imaging may prove to be a simpler method for assessing left diaphragm function when compared to M-mode [Citation54].
Assessing the contraction pattern of the diaphragm is also possible due to the benefits of strain. The right hemidiaphragm can be divided into three segments, including the crura, the dome, and the zone of apposition. By using strain to assess the diaphragm of normal volunteers, Ye’s team observed that the shortening of the right hemidiaphragm (negative strain value) first appeared in the zone of apposition and then in the crura, while the dome of the diaphragm stretched passively (positive strain value). Furthermore, when breathing deeply, the longitudinal strain of the right hemidiaphragm was higher than that of the crura [Citation55].
The utilization of Speckle tracking technology in healthy individuals receiving noninvasive pressure support ventilation has revealed a significant increase in both TF and transverse strain when utilizing continuous positive airway pressure (CPAP) and pressure-supported ventilation (PSV). Additionally, a strong correlation was observed between transverse strain and TF (r = 0.753; p < 0.001). Compared to M-mode, strain imaging is capable of capturing a wider range of diaphragmatic regions and has a higher accuracy in tracking motion, as shown by research [Citation56]. Studies have also demonstrated that strain and strain rates have a strong correlation with PDI (strain R2 = 0.72, strain rate R2 = 0.80) and diaphragmatic electrical activity (strain R2 = 0.60; strain rate R2 = 0.66) in healthy volunteers with increased inspiratory load (0–50%) and are superior to TF [Citation57].
Researchers have found that strain imaging can indeed be used to assess diaphragmatic function in surgical patients. Some scholars have even applied strain to patients after aortocoronary artery bypass grafting and concluded that Speckle tracking US is superior to conventional ultrasound measurement in assessing changes in diaphragm function after surgery [Citation58].
Strain imaging has the potential to be a valuable tool in the study of diaphragmatic function. However, currently available ultrasonographic machine software is designed for evaluating heart function and there is no machine specifically developed for studying diaphragmatic strain.
3. Application of diaphragmatic ultrasound in COPD
3.1. Diaphragmatic ultrasound for the diagnosis of COPD and determination of the severity
According to the GOLD COPD report, the diagnosis of COPD primarily relies on the presence of symptoms, such as cough, sputum production, dyspnea, and pulmonary function tests. It is crucial to note that the forced expiratory volume at the first second/forced vital capacity (FEV1/FVC) should be less than 0.70 after inhaling bronchodilators. The severity of airway obstruction and disease is judged based on lung function and questionnaire scores. The risk of exacerbation is estimated by the number of exacerbations and symptoms.
In order to better supplement the diagnosis of COPD, researchers have examined the M-mode index of obstruction (MIO), which measures the forced expiratory diaphragmatic excursion in the first second (FEDE1)/the maximum expiratory diaphragmatic excursion (EDEMax). The study found a statistical difference between the group of 124 patients with airway obstruction and the normal group. Airway obstruction was diagnosed using an MIO < 77, which had a sensitivity of 83.33%, specificity of 96.61%, positive predictive value of 95.5%, and negative predictive value of 73.7% [Citation59].
There are some challenges in using ultrasound to diagnose COPD. Further research is necessary to improve the accuracy and clinical utility of diaphragmatic ultrasound for COPD diagnosis. The research population should be expanded, the operation process standardized, and a population database established to determine diagnostic reference values.
In judging the severity of the disease in 32 patients with COPD, scholars found that the thickness and thickness changes of the diaphragm are related to lung volume, and the diaphragm thickening value is associated with lung function FEV1 [Citation60]. Similar studies have been conducted by other researchers who have concluded that DE and diaphragm thickening rate are correlated with lung function FEV1, while DT is not associated with FEV1 [Citation61]. Taehwa scholars only agree with some of the above views, he believes that DT and DE are related to FEV1, but the correlation between DE and FEV1 is stronger than that of thickness. Additionally, the correlation is higher on the right side compared to the left. It has also been suggested that a cutoff value of 6.7 cm for right DE should be used during heavy breathing, and there is a statistically significant difference in FEV1% between the two groups [Citation62]. Similarly, Evrin’s study not only supports a correlation between DE and FEV1 but also suggests that DE is linked to the frequency of exacerbations per patient per year [Citation63].
A study evaluated patients with AECOPD within 72 h of exacerbation and after symptom improvement using diaphragmatic ultrasound. It showed that the right TF significantly increased from the initial values to the follow-up values. The correlation between changes in mobility during stable and exacerbation phases was found to be positive with respect to the time until the next exacerbation and negative with respect to the time required for recovery from an exacerbation [Citation64]. As a noninvasive tool for assessing diaphragmatic function, ultrasound can be practically useful in managing patients with AECOPD. Further research is needed for thorough validation.
In a study of 55 COPD patients, researchers divided them into stable and acute exacerbation phases based on their symptoms. The study found that the TFmax and DEmax models were as effective as FVC and FEV1 in diagnosing disease deterioration. TFmax was found to be highly specific (78.8%) while DEmax was extremely sensitive (95.2%). In a multivariate regression analysis of age, sex, BMI, and lung function, the researchers identified a significant association between AECOPD and low TFmax (OR 8.40; 95% CI 1.55–45.56) as well as low DEmax (OR 11.51; 95% CI 1.15–115.56) based on the cutoff grouping [Citation65].
With advances in technology and standardization of procedures, diaphragmatic ultrasound may have great potential in diagnosing COPD and assessing its prognosis.
3.2. Diaphragmatic ultrasound to assess diaphragmatic dysfunction in patients with COPD
Due to pathophysiological changes in the diaphragm, such as oxidative stress and inflammation, DD occurs in COPD patients. According to a study, the prevalence of DD was found to be 24.3% during AECOPD. DD is a common occurrence in critically ill patients and has been linked to increased morbidity and mortality [Citation66]. Additionally, it can result in prolonged mechanical ventilation and intensive care stays [Citation67]. While the gold standard for testing diaphragmatic function is through measurement of PDI, it may not be effective in diagnosing unilateral diaphragmatic paralysis due to its poor sensitivity. This is because even with one effective hemidiaphragm, sufficient PDI can be generated during restful breathing. A study claimed that 6% of asymptomatic participants have a dyskinetic diaphragm [Citation68].
Ultrasound is a safe, noninvasive, accurate, and convenient technique widely used in intensive care. Scholars have demonstrated its feasibility and high reproducibility in detecting diaphragm dysfunction in critically ill patients [Citation69]. However, there are deficiencies in the quantitative assessment of DD. A study has shown that ultrasound has a diagnostic sensitivity of 93% and a specificity of 100% for the detection of neuromuscular diaphragm dysfunction [Citation70]. Commonly used methods for diagnosing diaphragmatic paralysis include measuring the DT, which should be less than 0.2 cm, and the TF, which should be less than 20% [Citation71]. Diaphragmatic weakness and paralysis can be differentiated based on the direction of diaphragm movement. Patients with diaphragmatic weakness exhibit decreased diaphragmatic movements during inspiration, while patients with diaphragmatic paralysis show paradoxical movements [Citation72]. The optimal cutoff values for DE in the diagnosis of DD in mechanically ventilated adult patients range from 1.0 to 1.4 cm during normal spontaneous breathing and 2.5 cm for maximum inspiratory force.
It is important to note that DE should only be used as an indicator of diaphragmatic function in spontaneously breathing patients [Citation69]. It also should be noted that the supine position may obscure the paradoxical movement of the diaphragm when assessing for hemidiaphragm dysfunction. So the DT should be measured to assess the function of the diaphragm. On the side of hemidiaphragm dysfunction, the DT will not be significantly thickened or may even became thinner [Citation38].
3.3. Diaphragmatic ultrasound predicts weaning outcomes in patients with COPD
In patients with COPD who experience an exacerbation of their illness due to infection, the use of either invasive or NIV is crucial. NIV is the preferred initial treatment for those with respiratory failure caused by AECOPD, although failure rates can range from 5 to 40% [Citation73]. It is important to keep in mind that patients who require invasive mechanical ventilation after unsuccessful NIV treatment have a significantly higher risk of mortality [Citation74]. Therefore, numerous scholars have conducted research on the effectiveness of diaphragmatic ultrasound in predicting the outcome of NIV for AECOPD (). The DE was found to be greater in the successful NIV group before and after NIV than in the failed group [Citation75]. NIV failure (p < 0.001, R2 = 0.27), prolonged ICU stay (p = 0.02, R2 = 0.13), prolonged mechanical ventilation (p = 0.023, R2 = 0.15), and tracheostomy requirement (p = 0.006, R2 = 0.20) have been noted in patients with DD. According to Kaplan–Meyer survival estimates, NIV failure in respiratory intensive care units (RICUs) was significantly associated with DD (log-rank test p = 0.001, HR = 8.09 (95% CI: 2.7–24.2)). Additionally, mortality was also significantly associated with DD (log-rank test p = 0.039, HR = 4.08 (95% CI: 1.0–16.4)) [Citation76]. While this view is supported by other scholars, it is important to note that patients with DD have a higher risk of NIV failure compared to patients without DD (hazard ratio, 4.4; p < 0.001). The correlation between DT change (ΔTdi) and Pdi when sniffing respiration is also noteworthy (r = 0.81; p = 0.004). Moreover, ΔTdi < 20% is a more accurate predictor of NIV failure than initial pH, early changes in arterial pH and carbon dioxide partial pressure after NIV initiation [Citation73]. In predicting NIV failure, DD had a sensitivity of 84.6% (95% CI: 54.6–98.1), specificity of 91.5% (95% CI: 79.6–97.6), positive predictive value of 73.3% (95% CI: 51.2–87.8), and negative predictive value of 95.6% (95% CI: 85.7–98.7) [Citation77]. A study has indicated that the ratio of odds (OR) for NIV failure using the DD standard of TF ≤ 20 and the calculated new standard of TF ≤ 19.04 before NIV was 6, respectively. After 2 h of NIV, the DD criterion of TF ≤ 35.3 had a better diagnostic profile and higher log odds and hazard ratio than other thresholds (<20; ≤19.04) in estimating NIV failure [Citation78].
Table 1. Characteristics and findings of articles using diaphragmatic ultrasound in NIV.
Diaphragm ultrasound has a significant reference value in predicting the outcome of mechanical ventilation. Various studies have been conducted to predict the time of weaning by measuring DE and TF. A study included 54 weaned patients, of which 70.4% were COPD patients. After analysis, researchers have established that the critical values for DE, thickness at the end of inspiration and expiration and TF are 1.05 cm, 2.1 cm, 1.05 cm, and 34.2%, respectively. The sensitivity values were reported as 87.5%, 77.5%, 80%, and 90%, while the specificity values were 71.5%, 86.6%, 50%, and 64.3%. When using a DE of ≥1.05 cm and a DT of ≥2.1 cm at end-inspiration to assess the timing of weaning, the sensitivity decreased to 64.9%, but the specificity increased to 100% [Citation79]. According to a study, in COPD patients undergoing a 30-min spontaneous breathing test, successful extubation can be predicted by evaluating both the 30-min DE and the difference between 30-min and 5-min DE. The area under the receiver operator characteristic (AUROC) was 0.867, sensitivity was 92% and specificity was 83.3% [Citation80]. A study has shown that DE is a more reliable predictor of extubation success than TF [Citation81]. However, this study is a small-sample, single-center study and cannot be broadly applied. To enhance the accuracy of weaning predictions, some researchers have proposed modification to the rapid shallow breathing index (RSBI), which is the ratio of respiratory rate (RR) to tidal volume (VT). Instead, they have replaced VT with DE and diaphragm thickening fraction (DTF) to calculate two new indices: the diaphragmatic excursion rapid shallow breathing index (DE-RSBI, respiratory rate RR/DE) and the diaphragm TF rapid shallow breathing index (DTF-RSBI, RR/DTF). In the evaluation of weaning, RSBI is used and the optimal cutoff values for predicting weaning failure are RSBI > 51.2 breaths/min/L, DE-RSBI > 1.38 breaths/min/mm, and DTF-RSBI > 78.1 breaths/min/%. The study found that the AUROC curves for DE-RSBI and DTF-RSBI were significantly higher than those for RSBI (p = 0.004 and p < 0.001, respectively) [Citation82].
In patients with acute exacerbations of COPD (AECOPD), some scholars have utilized the index of respiratory rate to diaphragmatic movement ratio. Their findings concluded that the diaphragm-related respiratory index had an AUROC curve of 0.97 (p < 0.001) and the RSBI had an AUROC curve of 0.67 (p < 0.06). Thus, the diaphragm-related index was found to be superior to the conventional RSBI in predicting weaning outcomes for patients with AECOPD [Citation83]. A study has assessed the effectiveness of the excursion-time (E-T) index, which is the result of multiplying DE and inspiratory time. According to the study, a reduction of the diaphragmatic E-T index of less than 3.8% has a sensitivity of 79.2% and a specificity of 75% in predicting the success of extubation [Citation84].
However, further research is needed in this area. With continued research, diaphragmatic ultrasound has the potential to become a dependable assessment method for determining the appropriate timing for weaning patients off of ventilatory support.
3.4. Other applications of diaphragmatic ultrasound in ventilatory support
Diaphragmatic ultrasound is capable of evaluating respiratory effort. Various studies have indicated a significant correlation between TF and invasive techniques like diaphragm and esophageal time-pressure product (PTPdi and PTPes). This correlation can establish a new noninvasive method for monitoring respiratory load during assisted mechanical ventilation [Citation69]. There are advantages to detecting atrophy in mechanically ventilated patients, including diaphragm thinning that can occur within 48 h of initiation of mechanical ventilation, as well as a reduction in DT over time in each patient [Citation85]. Therefore, it is recommended that measures be taken to prevent diaphragmatic atrophy when providing ventilatory support.
3.5. The role of diaphragmatic ultrasound in assessing pulmonary rehabilitation
Pulmonary rehabilitation is a comprehensive intervention that involves a thorough assessment of the patient. It is a therapy that is tailored to the individual and includes exercise training, education, and behavior change. The goal of pulmonary rehabilitation is to improve the physical and psychological condition of patients with chronic respiratory diseases and promote long-term adherence to healthy behaviors. Integrated management of patients with COPD is crucial due to the complexity of the disease, its multisystem manifestations, and common comorbidities. Thus, pulmonary rehabilitation plays a central role in this management [Citation86]. The effects of pulmonary rehabilitation are best evidenced by the improvement in symptoms, exercise performance, and overall quality of life in individuals diagnosed with COPD [Citation87].
To objectively assess the effects of pulmonary rehabilitation, scholars have utilized diaphragmatic ultrasound. In particular, some authors have examined DE in subjects with severe to very severe COPD both before and after rehabilitation. Results indicate that deep inspiration after rehabilitation resulted in improved DE, with mean values increasing from 4.58 ± 1.83 cm to 5.45 ± 1.56 cm (p = 0.05) [Citation88]. A study concluded that DE was a reliable predictor of improved exercise tolerance in patients with stable COPD who underwent pulmonary rehabilitation [Citation89]. According to previous studies, there is a correlation between the percentage change in diaphragm length after pulmonary rehabilitation in COPD patients with functional residual volume and the improvement in their 6-min walking distance. The sensitivity and specificity for predicting exercise capacity improvement in pulmonary rehabilitation are 83% and 74%, respectively, with a length change cutoff of at least 10% [Citation90].
Diaphragmatic ultrasound has the potential to serve as both a predictor of pulmonary rehabilitation efficacy and a real-time monitoring technique for respiratory muscle training during such rehabilitation.
3.6. Predicting nocturnal oxygen saturation in patients with COPD
Patients with COPD frequently experience a reduction in their oxygen saturation levels during the night. Studies have shown that there is a notable connection between the mean arterial oxygen saturation at night and other factors such as maximal inspiratory port pressure (r = 0.65), maximal inspiratory trans-diaphragmatic pressure (r = 0.53), FEV1 (r = 0.61), arterial oxygen saturation (SaO2) (r = 0.75), and PaCO2 (r = −0.44) [Citation91]. To investigate a more noninvasive and straightforward method, researchers examined the correlation between diaphragmatic ultrasound and blood oxygen saturation. A study involved 32 male patients with varying degrees of COPD severity. The researchers measured DT, TF, and blood oxygen saturation levels at the end of maximum inspiration and maximal expiration. After conducting statistical analysis, the study found a positive correlation between nocturnal oxygen saturation and TF. There was a positive correlation between DTF (r = 0.70) and daytime PaO2 (r = 0.69). Additionally, the combination of these factors proved to be more effective in predicting nocturnal SaO2 in patients [Citation92].
In the field of medicine, diaphragmatic ultrasound has the potential to complement other diagnostic tests, enabling more accurate prediction and assessment of oxygen saturation levels in patients with COPD. This noninvasive and simple method holds promise for improving patient care in the future.
4. Limitations
Ultrasound is an evolving technique that has gained widespread acceptance as a tool for assessing diaphragms. However, there are some limitations. First, the acquisition and analysis of ultrasound images rely on operators who need to possess a certain level of experience and knowledge to ensure accurate and comprehensive results. Standardized training is necessary for operators to achieve this level of proficiency.
In addition, ultrasound, like other monitoring methods, can be affected by various factors within the body, such as lung and gastrointestinal gas, as well as disturbances caused by lesions in the observation area. These factors can also result in poor imaging of the left diaphragm. Due to the technical challenges involved in examining the left diaphragm, some researchers have limited their assessments to the right diaphragm in certain reports.
Besides, numerous studies have indicated the significant potential of diaphragmatic ultrasound. However, the majority of these research projects have utilized single-center and small-sample studies, thus requiring further verification of the results.
Recent international guidelines have focused on the functional assessment of the diaphragm to address or refine the differential diagnosis of respiratory failure in intensive care units but there is no universal approach to US characterization of diaphragmatic function [Citation18]. As each study has a different purpose, there may be variations in research methods and measurement standards. If diaphragmatic ultrasound is to be widely used in clinical practice, an accurate and reliable ultrasound guide is needed to help operators use the correct technology and evaluation methods. This is also a major focus of future research.
5. Conclusions
Ultrasound can be used to assess diaphragm function through various techniques, such as conventional DT, TF, DE, elastography, and strain imaging. This is crucial for the diagnosis, prognosis, and treatment monitoring of patients with COPD. Diaphragm ultrasound has the potential to become a precise, user-friendly, and non-intrusive technique for comprehensive evaluation of diaphragm function, as technology advances, operation improves, and research population grows.
Author contributions
H.M. wrote the manuscript. All authors reviewed the literature and edited and commented on the manuscript. The final version was reviewed and approved by all authors for publication.
Acknowledgments
The authors express their gratitude to all the patients and families whose participation has contributed to the advancement of knowledge regarding COPD.
Disclosure statement
The authors have no conflicts of interest to declare.
Funding
This research did not receive any specific grant from funding agencies in the public, commercial, or not-for-profit sectors.
References
- Adeloye D, Song P, Zhu Y, et al. Global, regional, and national prevalence of, and risk factors for, chronic obstructive pulmonary disease (COPD) in 2019: a systematic review and modelling analysis. Lancet Respir Med. 2022;10(5):1–10. doi: 10.1016/S2213-2600(21)00511-7.
- Global Initiative for Chronic Obstructive Lung Disease. Global strategy for diagnosis, management, and prevention of COPD. 2023. [accessed January 9, 2023]. Available from: http://www.goldcopd.org
- López-Campos JL, Tan W, Soriano JB. Global burden of COPD. Respirology. 2016;21(1):14–23. doi: 10.1111/resp.12660.
- Beaumont M, Forget P, Couturaud F, et al. Effects of inspiratory muscle training in COPD patients: a systematic review and meta-analysis. Clin Respir J. 2018;12(7):2178–2188. doi: 10.1111/crj.12905.
- Jia Y, Zhang Q. Research progress on diaphragm ultrasound in chronic obstructive pulmonary disease: a narrative review. Ultrasound Med Biol. 2022;48(4):587–597. doi: 10.1016/j.ultrasmedbio.2021.10.019.
- Faulkner JA, Maxwell LC, Ruff GL, et al. The diaphragm as a muscle. Contractile properties. Am Rev Respir Dis. 1979;119(2 Pt 2):89–92. doi: 10.1164/arrd.1979.119.2P2.89.
- Thurlbeck WM. Diaphragm and body weight in emphysema. Thorax. 1978;33(4):483–487. doi: 10.1136/thx.33.4.483.
- Arora NS, Rochester DF. Effect of body weight and muscularity on human diaphragm muscle mass, thickness, and area. J Appl Physiol Respir Environ Exerc Physiol. 1982;52(1):64–70. doi: 10.1152/jappl.1982.52.1.64.
- Rochester DF. The diaphragm: contractile properties and fatigue. J Clin Invest. 1985;75(5):1397–1402. doi: 10.1172/JCI111841.
- Derenne JP, Macklem PT, Roussos C. The respiratory muscles: mechanics, control, and pathophysiology. Part III. Am Rev Respir Dis. 1978;118(3):581–601. doi: 10.1164/arrd.1978.118.3.581.
- Similowski T, Yan S, Gauthier AP, et al. Contractile properties of the human diaphragm during chronic hyperinflation. N Engl J Med. 1991;325(13):917–923. doi: 10.1056/NEJM199109263251304.
- Ottenheijm CAC, Heunks LMA, Sieck GC, et al. Diaphragm dysfunction in chronic obstructive pulmonary disease. Am J Respir Crit Care Med. 2005;172(2):200–205. doi: 10.1164/rccm.200502-262OC.
- Ottenheijm CAC, Heunks LMA, Dekhuijzen PNR. Diaphragm muscle fiber dysfunction in chronic obstructive pulmonary disease: toward a pathophysiological concept. Am J Respir Crit Care Med. 2007;175(12):1233–1240. doi: 10.1164/rccm.200701-020PP.
- Laghi FA, Jr., Saad M, Shaikh H. Ultrasound and non-ultrasound imaging techniques in the assessment of diaphragmatic dysfunction. BMC Pulm Med. 2021;21(1):85. doi: 10.1186/s12890-021-01441-6.
- Corradi F, Isirdi A, Malacarne P, et al. Low diaphragm muscle mass predicts adverse outcome in patients hospitalized for COVID-19 pneumonia: an exploratory pilot study. Minerva Anestesiol. 2021;87(4):432–438. doi: 10.23736/S0375-9393.21.15129-6.
- Corradi F, Vetrugno L, Orso D, et al. Diaphragmatic thickening fraction as a potential predictor of response to continuous positive airway pressure ventilation in covid-19 pneumonia: a single-center pilot study. Respir Physiol Neurobiol. 2021;284:103585. doi: 10.1016/j.resp.2020.103585.
- Baldwin CE, Paratz JD, Bersten AD. Diaphragm and peripheral muscle thickness on ultrasound: intra-rater reliability and variability of a methodology using non-standard recumbent positions. Respirology. 2011;16(7):1136–1143. doi: 10.1111/j.1440-1843.2011.02005.x.
- Demi L, Wolfram F, Klersy C, et al. New international guidelines and consensus on the use of lung ultrasound. J Ultrasound Med. 2023;42(2):309–344. doi: 10.1002/jum.16088.
- Ueki J, De Bruin PF, Pride NB. In vivo assessment of diaphragm contraction by ultrasound in normal subjects. Thorax. 1995;50(11):1157–1161. doi: 10.1136/thx.50.11.1157.
- Wait JL, Nahormek PA, Yost WT, et al. Diaphragmatic thickness-lung volume relationship in vivo. J Appl Physiol (1985). 1989;67(4):1560–1568. doi: 10.1152/jappl.1989.67.4.1560.
- Cohn D, Benditt JO, Eveloff S, et al. Diaphragm thickening during inspiration. J Appl Physiol (1985). 1997;83(1):291–296. doi: 10.1152/jappl.1997.83.1.291.
- Boussuges A, Rives S, Finance J, et al. Assessment of diaphragmatic function by ultrasonography: current approach and perspectives. World J Clin Cases. 2020;8(12):2408–2424. doi: 10.12998/wjcc.v8.i12.2408.
- Scarlata S, Mancini D, Laudisio A, et al. Reproducibility of diaphragmatic thickness measured by M-mode ultrasonography in healthy volunteers. Respir Physiol Neurobiol. 2019;260:58–62. doi: 10.1016/j.resp.2018.12.004.
- Hellyer NJ, Andreas NM, Bernstetter AS, et al. Comparison of diaphragm thickness measurements among postures via ultrasound imaging. PM R. 2017;9(1):21–25. doi: 10.1016/j.pmrj.2016.06.001.
- Carrillo-Esper R, Pérez-Calatayud ÁA, Arch-Tirado E, et al. Standardization of sonographic diaphragm thickness evaluations in healthy volunteers. Respir Care. 2016;61(7):920–924. doi: 10.4187/respcare.03999.
- Boussuges A, Rives S, Finance J, et al. Ultrasound assessment of diaphragm thickness and thickening: reference values and limits of normality when in a seated position. Front Med (Lausanne). 2021;8:742703. doi: 10.3389/fmed.2021.742703.
- Baria MR, Shahgholi L, Sorenson EJ, et al. B-mode ultrasound assessment of diaphragm structure and function in patients with COPD. Chest. 2014;146(3):680–685. doi: 10.1378/chest.13-2306.
- Ogan N, Aydemir Y, Evrin T, et al. Diaphragmatic thickness in chronic obstructive lung disease and relationship with clinical severity parameters. Turk J Med Sci. 2019;49(4):1073–1078. doi: 10.3906/sag-1901-164.
- Eryüksel E, Cimşit C, Bekir M, et al. Diaphragmatic thickness fraction in subjects at high-risk for COPD exacerbations. Respir Care. 2017;62(12):1565–1570. doi: 10.4187/respcare.05646.
- Topcuoğlu C, Yümin ET, Hizal M, et al. Examination of diaphragm thickness, mobility and thickening fraction in individuals with COPD of different severity. Turk J Med Sci. 2022;52(4):1288–1298. doi: 10.55730/1300-0144.5435.
- Okura K, Iwakura M, Shibata K, et al. Diaphragm thickening assessed by ultrasonography is lower than healthy adults in patients with chronic obstructive pulmonary disease. Clin Respir J. 2020;14(6):521–526. doi: 10.1111/crj.13161.
- Orde SR, Boon AJ, Firth DG, et al. Use of Angle-Independent M-mode sonography for assessment of diaphragm displacement. J Ultrasound Med. 2016;35(12):2615–2621. doi: 10.7863/ultra.15.11100.
- Haber K, Asher M, Freimanis AK. Echographic evaluation of diaphragmatic motion in intra-abdominal diseases. Radiology. 1975;114(1):141–144. doi: 10.1148/114.1.141.
- Ayoub J, Cohendy R, Dauzat M, et al. Non-invasive quantification of diaphragm kinetics using m-mode sonography. Can J Anaesth. 1997;44(7):739–744. doi: 10.1007/BF03013389.
- Testa A, Soldati G, Giannuzzi R, et al. Ultrasound M-mode assessment of diaphragmatic kinetics by anterior transverse scanning in healthy subjects. Ultrasound Med Biol. 2011;37(1):44–52. doi: 10.1016/j.ultrasmedbio.2010.10.004.
- Scarlata S, Mancini D, Laudisio A, et al. Reproducibility and clinical correlates of supine diaphragmatic motion measured by M-mode ultrasonography in healthy volunteers. Respiration. 2018;96(3):259–266. doi: 10.1159/000489229.
- Houston JG, Angus RM, Cowan MD, et al. Ultrasound assessment of normal hemidiaphragmatic movement: relation to inspiratory volume. Thorax. 1994;49(5):500–503. doi: 10.1136/thx.49.5.500.
- Boussuges A, Finance J, Chaumet G, et al. Diaphragmatic motion recorded by M-mode ultrasonography: limits of normality. ERJ Open Res. 2021;7(1):00714-2020. doi: 10.1183/23120541.00714-2020.
- Dos Santos Yamaguti WP, Paulin E, Shibao S, et al. Air trapping: the major factor limiting diaphragm mobility in chronic obstructive pulmonary disease patients. Respirology. 2008;13(1):138–144. doi: 10.1111/j.1440-1843.2007.01194.x.
- Paulin E, Yamaguti WPS, Chammas MC, et al. Influence of diaphragmatic mobility on exercise tolerance and dyspnea in patients with COPD. Respir Med. 2007;101(10):2113–2118. doi: 10.1016/j.rmed.2007.05.024.
- Shiraishi M, Higashimoto Y, Sugiya R, et al. Diaphragmatic excursion correlates with exercise capacity and dynamic hyperinflation in COPD patients. ERJ Open Res. 2020;6(4):00589-2020. doi: 10.1183/23120541.00589-2020.
- Grubb NR, Fleming A, Sutherland GR, et al. Skeletal muscle contraction in healthy volunteers: assessment with Doppler tissue imaging. Radiology. 1995;194(3):837–842. doi: 10.1148/radiology.194.3.7862989.
- Maurizio R, Rinaldi VE, Camerini PG, et al. Right diaphragmatic peak motion velocities on pulsed wave tissue Doppler imaging in neonates: method, reproducibility, and reference values. J Ultrasound Med. 2019;38(10):2695–2701. doi: 10.1002/jum.14974.
- Soilemezi E, Savvidou S, Sotiriou P, et al. Tissue Doppler imaging of the diaphragm in healthy subjects and critically ill patients. Am J Respir Crit Care Med. 2020;202(7):1005–1012. doi: 10.1164/rccm.201912-2341OC.
- McCool FD, Tzelepis GE. Tissue Doppler imaging of the diaphragm: a new kid on the block? Am J Respir Crit Care Med. 2020;202(7):921–922. doi: 10.1164/rccm.202007-2771ED.
- Jonkman AH, Wennen M, Sklar MC, et al. Tissue Doppler imaging of the diaphragm: a novel approach but too early for clinical implementation? Am J Respir Crit Care Med. 2020;202(12):1741–1742. doi: 10.1164/rccm.202007-2958LE.
- Chino K, Ohya T, Katayama K, et al. Diaphragmatic shear modulus at various submaximal inspiratory mouth pressure levels. Respir Physiol Neurobiol. 2018;252–253:52–57. doi: 10.1016/j.resp.2018.03.009.
- Bachasson D, Dres M, Niérat M-C, et al. Diaphragm shear modulus reflects transdiaphragmatic pressure during isovolumetric inspiratory efforts and ventilation against inspiratory loading. J Appl Physiol (1985). 2019;126(3):699–707. doi: 10.1152/japplphysiol.01060.2018.
- Fossé Q, Poulard T, Niérat M-C, et al. Ultrasound shear wave elastography for assessing diaphragm function in mechanically ventilated patients: a breath-by-breath analysis. Crit Care. 2020;24(1):669. doi: 10.1186/s13054-020-03338-y.
- Xu JH, Wu ZZ, Tao FY, et al. Ultrasound shear wave elastography for evaluation of diaphragm stiffness in patients with stable COPD: a pilot trial. J Ultrasound Med. 2021;40(12):2655–2663. doi: 10.1002/jum.15655.
- Chen Y, Li J, Dong B, et al. Two-dimensional shear wave elastography: a new tool for evaluating respiratory muscle stiffness in chronic obstructive pulmonary disease patients. BMC Pulm Med. 2022;22(1):441. doi: 10.1186/s12890-022-02231-4.
- Tuinman PR, Jonkman AH, Dres M, et al. Respiratory muscle ultrasonography: methodology, basic and advanced principles and clinical applications in ICU and ED patients-a narrative review. Intensive Care Med. 2020;46(4):594–605. doi: 10.1007/s00134-019-05892-8.
- Orde SR, Boon AJ, Firth DG, et al. Diaphragm assessment by two dimensional speckle tracking imaging in normal subjects. BMC Anesthesiol. 2016;16(1):43. doi: 10.1186/s12871-016-0201-6.
- Goutman SA, Hamilton JD, Swihart B, et al. Speckle tracking as a method to measure hemidiaphragm excursion. Muscle Nerve. 2017;55(1):125–127. doi: 10.1002/mus.25380.
- Ye X, Xiao HUI, Bai W, et al. Two-dimensional strain ultrasound speckle tracking as a novel approach for the evaluation of right hemidiaphragmatic longitudinal deformation. Exp Ther Med. 2013;6(2):368–372. doi: 10.3892/etm.2013.1133.
- Hatam N, Goetzenich A, Rossaint R, et al. A novel application for assessing diaphragmatic function by ultrasonic deformation analysis in noninvasively ventilated healthy young adults. Ultraschall Med. 2014;35(6):540–546. doi: 10.1055/s-0034-1366090.
- Oppersma E, Hatam N, Doorduin J, et al. Functional assessment of the diaphragm by speckle tracking ultrasound during inspiratory loading. J Appl Physiol (1985). 2017;123(5):1063–1070. doi: 10.1152/japplphysiol.00095.2017.
- Fritsch SJ, Hatam N, Goetzenich A, et al. Speckle tracking ultrasonography as a new tool to assess diaphragmatic function: a feasibility study. Ultrasonography. 2022;41(2):403–415. doi: 10.14366/usg.21044.
- Zanforlin A, Smargiassi A, Inchingolo R, et al. Ultrasound analysis of diaphragm kinetics and the diagnosis of airway obstruction: the role of the M-mode index of obstruction. Ultrasound Med Biol. 2014;40(6):1065–1071. doi: 10.1016/j.ultrasmedbio.2013.12.009.
- Smargiassi A, Inchingolo R, Tagliaboschi L, et al. Ultrasonographic assessment of the diaphragm in chronic obstructive pulmonary disease patients: relationships with pulmonary function and the influence of body composition - a pilot study. Respiration. 2014;87(5):364–371. doi: 10.1159/000358564.
- Schulz A, Erbuth A, Boyko M, et al. Comparison of ultrasound measurements for diaphragmatic mobility, diaphragmatic thickness, and diaphragm thickening fraction with each other and with lung function in patients with chronic obstructive pulmonary disease. Int J Chron Obstruct Pulmon Dis. 2022;17:2217–2227. doi: 10.2147/COPD.S375956.
- Kim T, Huh S, Chung JH, et al. Clinical values of diaphragmatic movement in patients with chronic obstructive pulmonary disease. BMC Pulm Med. 2023;23(1):33. doi: 10.1186/s12890-022-02220-7.
- Evrin T, Korkut S, Ozturk Sonmez L, et al. Evaluating stable chronic obstructive pulmonary disease by ultrasound. Emerg Med Int. 2019;2019:5361620–5361628. doi: 10.1155/2019/5361620.
- Lim SY, Lim G, Lee YJ, et al. Ultrasound assessment of diaphragmatic function during acute exacerbation of chronic obstructive pulmonary disease: a pilot study. Int J Chron Obstruct Pulmon Dis. 2019;14:2479–2484. doi: 10.2147/COPD.S214716.
- An TJ, Yoo YJ, Lim JU, et al. Diaphragm ultrasound is an imaging biomarker that distinguishes exacerbation status from stable chronic obstructive pulmonary disease. Int J Chron Obstruct Pulmon Dis. 2022;17:3–12.
- Supinski GS, Morris PE, Dhar S, et al. Diaphragm dysfunction in critical illness. Chest. 2018;153(4):1040–1051. doi: 10.1016/j.chest.2017.08.1157.
- Dres M, Dubé BP, Mayaux J, et al. Coexistence and impact of limb muscle and diaphragm weakness at time of liberation from mechanical ventilation in medical intensive care unit patients. Am J Respir Crit Care Med. 2017;195(1):57–66. doi: 10.1164/rccm.201602-0367OC.
- Matamis D, Soilemezi E, Tsagourias M, et al. Sonographic evaluation of the diaphragm in critically ill patients. Technique and clinical applications. Intensive Care Med. 2013;39(5):801–810. doi: 10.1007/s00134-013-2823-1.
- Zambon M, Greco M, Bocchino S, et al. Assessment of diaphragmatic dysfunction in the critically ill patient with ultrasound: a systematic review. Intensive Care Med. 2017;43(1):29–38. doi: 10.1007/s00134-016-4524-z.
- Boon AJ, Sekiguchi H, Harper CJ, et al. Sensitivity and specificity of diagnostic ultrasound in the diagnosis of phrenic neuropathy. Neurology. 2014;83(14):1264–1270. doi: 10.1212/WNL.0000000000000841.
- Gottesman E, McCool FD. Ultrasound evaluation of the paralyzed diaphragm. Am J Respir Crit Care Med. 1997;155(5):1570–1574. doi: 10.1164/ajrccm.155.5.9154859.
- Boussuges A, Brégeon F, Blanc P, et al. Characteristics of the paralysed diaphragm studied by M-mode ultrasonography. Clin Physiol Funct Imaging. 2019;39(2):143–149. doi: 10.1111/cpf.12549.
- Marchioni A, Castaniere I, Tonelli R, et al. Ultrasound-assessed diaphragmatic impairment is a predictor of outcomes in patients with acute exacerbation of chronic obstructive pulmonary disease undergoing noninvasive ventilation. Crit Care. 2018;22(1):109. doi: 10.1186/s13054-018-2033-x.
- Demoule A, Girou E, Richard J-C, et al. Benefits and risks of success or failure of noninvasive ventilation. Intensive Care Med. 2006;32(11):1756–1765. doi: 10.1007/s00134-006-0324-1.
- Cammarota G, Sguazzotti I, Zanoni M, et al. Diaphragmatic ultrasound assessment in subjects with acute hypercapnic respiratory failure admitted to the emergency department. Respir Care. 2019;64(12):1469–1477. doi: 10.4187/respcare.06803.
- Antenora F, Fantini R, Iattoni A, et al. Prevalence and outcomes of diaphragmatic dysfunction assessed by ultrasound technology during acute exacerbation of COPD: a pilot study. Respirology. 2017;22(2):338–344. doi: 10.1111/resp.12916.
- Kocyigit H, Gunalp M, Genc S, et al. Diaphragm dysfunction detected with ultrasound to predict noninvasive mechanical ventilation failure: a prospective cohort study. Am J Emerg Med. 2021;45:202–207. doi: 10.1016/j.ajem.2020.08.014.
- Patel NB, Jain G, Chauhan U, et al. Evaluating diaphragmatic dysfunction and predicting non-invasive ventilation failure in acute exacerbation of chronic obstructive pulmonary disease in India. Acute Crit Care. 2023;38(2):200–208. doi: 10.4266/acc.2022.01060.
- Farghaly S, Hasan AA. Diaphragm ultrasound as a new method to predict extubation outcome in mechanically ventilated patients. Aust Crit Care. 2017;30(1):37–43. doi: 10.1016/j.aucc.2016.03.004.
- Zhang X, Yuan J, Zhan Y, et al. Evaluation of diaphragm ultrasound in predicting extubation outcome in mechanically ventilated patients with COPD. Ir J Med Sci. 2020;189(2):661–668. doi: 10.1007/s11845-019-02117-1.
- Yoo JW, Lee SJ, Lee JD, et al. Comparison of clinical utility between diaphragm excursion and thickening change using ultrasonography to predict extubation success. Korean J Intern Med. 2018;33(2):331–339. doi: 10.3904/kjim.2016.152.
- Song J, Qian Z, Zhang H, et al. Diaphragmatic ultrasonography-based rapid shallow breathing index for predicting weaning outcome during a pressure support ventilation spontaneous breathing trial. BMC Pulm Med. 2022;22(1):337. doi: 10.1186/s12890-022-02133-5.
- Abbas A, Embarak S, Walaa M, et al. Role of diaphragmatic rapid shallow breathing index in predicting weaning outcome in patients with acute exacerbation of COPD. Int J Chron Obstruct Pulmon Dis. 2018;13:1655–1661. doi: 10.2147/COPD.S161691.
- Palkar A, Narasimhan M, Greenberg H, et al. Diaphragm excursion-time index: a new parameter using ultrasonography to predict extubation outcome. Chest. 2018;153(5):1213–1220. doi: 10.1016/j.chest.2018.01.007.
- Grosu HB, Lee YI, Lee J, et al. Diaphragm muscle thinning in patients who are mechanically ventilated. Chest. 2012;142(6):1455–1460. doi: 10.1378/chest.11-1638.
- Wagg K. Unravelling self-management for COPD: what next? Chron Respir Dis. 2012;9(1):5–7. doi: 10.1177/1479972311435910.
- Ries AL, Kaplan RM, Limberg TM, et al. Effects of pulmonary rehabilitation on physiologic and psychosocial outcomes in patients with chronic obstructive pulmonary disease. Ann Intern Med. 1995;122(11):823–832. doi: 10.7326/0003-4819-122-11-199506010-00003.
- Corbellini C, Boussuges A, Villafañe JH, et al. Diaphragmatic mobility loss in subjects with moderate to very severe COPD may improve after in-patient pulmonary rehabilitation. Respir Care. 2018;63(10):1271–1280. doi: 10.4187/respcare.06101.
- Chen Y, Li P, Wang J, et al. Assessments and targeted rehabilitation therapies for diaphragmatic dysfunction in patients with chronic obstructive pulmonary disease: a narrative review. Int J Chron Obstruct Pulmon Dis. 2022;17:457–473. doi: 10.2147/COPD.S338583.
- Crimi C, Heffler E, Augelletti T, et al. Utility of ultrasound assessment of diaphragmatic function before and after pulmonary rehabilitation in COPD patients. Int J Chron Obstruct Pulmon Dis. 2018;13:3131–3139. doi: 10.2147/COPD.S171134.
- Heijdra YF, Dekhuijzen PN, van Herwaarden CL, et al. Nocturnal saturation and respiratory muscle function in patients with chronic obstructive pulmonary disease. Thorax. 1995;50(6):610–612. doi: 10.1136/thx.50.6.610.
- Okura K, Kawagoshi A, Iwakura M, et al. Contractile capability of the diaphragm assessed by ultrasonography predicts nocturnal oxygen saturation in COPD. Respirology. 2017;22(2):301–306. doi: 10.1111/resp.12897.