Abstract
Gated gap junction channels are important cellular conduits for establishing and maintaining intercellular communication. The three-dimensional structure of a mutant human connexin 26 (Cx26M34A) by electron cryocrystallography revealed a plug-like density in the channel pore suggesting that physical blockage of the pore may be one mechanism of closure (Citation, Proc Natl Acad Sci USA 104: 10034–10039). However, it remains to be determined what part of the sequence contributes to the plug. Here, we present the projection structure of an N-terminus deletion of Cx26M34A missing amino acids 2 to 7 (Cx26M34Adel2-7) crystallized in the same two-dimensional crystal form. A 10 Å resolution projection map of Cx26M34Adel2-7 revealed that the plug density was dramatically reduced in comparison with that found in full-length Cx26 channel. The difference map between the deletion and full-length Cx26M34A channels strongly suggests that the N-terminus of connexin contributes to the plug for the physical closure of gap junction channels.
INTRODUCTION
Elucidating the structure of gap junction channels at high resolution is essential to the understanding of the gating mechanisms that are regulated by cytoplasmic modulating proteins, transjunctional voltage, pH, ions, and chemical compounds (Harris Citation2001). Electron crystallography is a powerful tool for studying the three-dimensional (3D) structure of gap junction channels that often form two-dimensional (2D) crystals in lipid bilayers. Unwin and coworkers (Unwin and Zampighi Citation1980; Unwin and Ennis 1984) originally used this strategy to show that the tertiary structure of the gap junction channel undergoes a conformational change modulated by Ca2 +. Higher resolution studies using electron cryocrystallography of 2D crystals of a truncation mutant of Cx43 at residue 263 (Cx43-263T) also revealed that each hemichannel of gap junction is comprised of 24 α-helices (Unger et al. Citation1999), from which a model of α-helix assignment is derived (Fleishman et al. Citation2004).
The N-terminus has been described as a voltage sensor from the results of electrophysiological studies (Verselis et al. Citation1994; Oh et al. Citation2000, Citation2004). Mutagenesis of specific residues in the N-terminus of Cx26 and Cx32 reversed the gating polarity, thereby leading Verselis et al. (Citation1994) to propose that the N-terminus may be physically located in or close to the pore to sense the electric field. Further studies have also proposed that the first 10 amino acid residues of Cx32 interact with a transjunctional electric field located in the pore vestibule (Purnick et al. Citation2000b). Nuclear magnetic resonance (NMR) spectroscopy suggested that N-terminus of Cx26 has some helicity as well as a turn at residues 12 to 15 that allows the N-terminus to be moved in and out of the aqueous pore (Purnick et al. Citation2000a). It should also be noted that two hereditary mutations that result in nonsyndromic sensorineural deafness are found within these first 10 residues, a Gly to Asp point mutation at position 4 (Hwa et al. Citation2003) and a Thr to Met point mutation at position 8 (Kenna et al. Citation2001). Cx26 with the T8M mutation formed functional gap junction channels in paired Xenopus oocytes, but with a reduction in both the magnitude and rate of coupling compared with wild-type Cx26 (Mese et al. Citation2004).
Recently, we reported the 3D structure of a mutant Cx26 (Cx26M34A) gap junction channel contains an obstruction or “plug” in the pore vestibule, implying that channel closure may occur by physically blocking the channel opening at a position approximately three-fourths in from the cytoplasmic surface (Oshima et al. Citation2007). Because the plug was observed in all the hemichannels in the unit cell, we hypothesized that two interacting hemichannels can function independently and that channel activity may be regulated autonomously with a plug. However, because the 3D map is not at a resolution high enough to allow unambiguous assignment of the Cα backbone, the question of what primary sequence contributes to the plug density remains to be determined.
In order to address this issue, we created an N-terminal deletion mutant of Cx26M34A. We use Cx26M34A for our electron crystallographic analysis because it expresses in large amounts as compared to wild type. A recent report of N-terminal deletion mutants in Cx37 has shown that some deletion constructs are assembled into hemichannels, traffic to the membrane and form gap junction plaques in mammalian cells, but do not pass current in electrophysiological assays in paired Xenopus oocytes (Kyle et al. Citation2007). We were interested in constructing a deletion mutant missing a significant number of amino acids, yet still retaining the capability to form channels and based on the preliminary studies of Kyle et al. (Citation2007), we constructed an analogous Cx26 mutant missing amino acids 2 to 7 (Cx26M34Adel2-7) that formed gap junction plaques and hemichannels in both transfected mammalian and baculovirus-infected insect cells. The latter provides preparations suitable for 2D crystallization (Oshima et al. Citation2007). This mutant Cx26 was expressed, purified and crystallized as we previously reported.
Here, we present the projection structure obtained from Cx26M34Adel2-7 2D crystals calculated at 10 Å resolution. In these 2D maps, the pore has a drastically reduced density as compared to full length Cx26M34A channel. The comparison of this initial projection map with the full-length Cx26M34A 3D map suggests that the N-terminus of connexin may function as a pivotal entity for one type of closure mechanism that uses a physical obstruction to prevent movement of molecules through the pore.
METHODS
Generation of Connexin Constructs
The N-terminus deletion mutant of human Cx26 cDNA was created using the following primer: Cx26M34Adel2-7: 5′-TTGAATTCCACCATGAC GATCCTGGGGGGTGTGAACAAACACTCC-3′.
Expression and Visualization in Mammalian Cells
For visualization with confocal microscopy, Cx26M34Adel2-7 was inserted into pEGFP-N3 (Clontech, Mountain View, CA). Transient transfections in HeLa cells were performed using the Fugene 6 transfection kit (Roche, Indianapolis, IN) following procedures described in Beahm et al. (Citation2006). Confocal images of cells expressing GFP fusion proteins were recorded on a BioRad MRC 1024 confocal microscope (Beahm et al. Citation2006). Dye coupling experiments were performed as described in Beahm et al. (Citation2006), except that Lucifer Yellow was used as the permeant.
Expression, Purification, and Crystallization of Mutants from Baculovirus-Infected Sf9 Cells
Recombinant baculoviruses of Cx26M34A and Cx26M34Adel2-7 were generated with linearized Bac-N-Blue DNA (Invitrogen, Carlsbad, CA). Protein expression with Sf9 insect cells, gap junction membrane isolation and protein purification were performed as described previously (Oshima et al. Citation2003). Reconstitution was performed by dialysis after the synthetic lipid dioleoylphosphatidylcholine (DOPC; Avanti Polar Lipids, Alabaster, AL) was mixed with purified proteins at a lipid-to-protein ratio of 0.8 (w/w) for Cx26M34Adel2-7, 1.0 (w/w) for Cx26M34A. Two-dimensional crystals were successfully obtained after 6 days dialysis, similar to those results previously reported for the full-length channels (Oshima et al. Citation2007). For Cx26M34Adel2-7 preparations, we observed that we obtained crystals that diffracted to higher resolution when carbenoxolone was not added to the outer dialysis solution or in the crystallization buffer.
Electron Cryomicroscopy and Three-Dimensional Crystallographic Reconstruction Methods
Two-dimensional crystals were embedded in dialysis buffer containing 10% trehalose and applied to the carbon support film attached on the molybdenum grid, then plunged into liquid nitrogen. Untilted images were recorded on films at 300 kV by cryo electron microscope, a liquid-helium stage equipped JEM-3000SFF electronmicroscope (JEOL) (Fujiyoshi et al. Citation1991). Images taken at a magnification of × 60,000 were analyzed and merged using the MRC image-processing package (Crowther et al. Citation1996). The amplitude data sets of Cx26M34A and Cx26M34Adel2-7 were scaled with SCALEIT (Collaborative Computational Project, Number 4 1994), and the phase information of Cx26M34A was used for the difference map calculation. For the cross-sectional view of Cx26M34A 3D structure, we confirmed that the autocorrelation function of the original twofold map revealed over 80% correlation at 60° and 120° rotational angles. Thus, it is reasonable to apply sixfold noncrystallographic rotational real space averaging to the p22121 structure. Symmetry averaging reinforced densities along the central channel pore axis, thereby strengthening the plug feature and eliminating other much weaker off-axis noise.
RESULTS AND DISCUSSION
In our earlier publication (Oshima et al. Citation2007), we speculated that the N-termini in a hemichannel might be the most likely candidate to form the plug domain. Previous studies have indicated that additions or deletions to the N-terminus can form gap junctions but are typically nonfunctional, indicating that the native conformation is critical to the channel functionality. For example, addition of a green fluorescent protein (GFP) or a tetracysteine domain to the N-terminus results in nonfunctional gap junctions, which at light microscopic resolution appear similar to wild type (see Sosinsky and Nicholson, Citation2005 for a more complete review). Here, we were interested in modifying or eliminating residues in the Cx26 N-terminus to see if the channel can assemble and if so, to test what structural changes in the tertiary structure ensue.
Cx26M34Adel2-7 Connexins Make Gap Junctions in Mammalian Cells
We generated the N-terminus deletion mutant Cx26, Cx26M34Adel2-7, in which amino acid residues from second to seventh (DWGTLQ) were removed (). A Cx26M34Adel2-7-GFP construct was created and expressed in HeLa cells to see whether the protein was trafficked to the cell surface. Confocal microscopy revealed that this construct exhibited a normal distribution of gap junction plaques between adjacent cells (, arrows). In preliminary functional assay experiments, Lucifer Yellow dye transfer was eliminated in all injected HeLa cells expressing wild type Cx26del2-7-GFP gap junctions (n = 5, time scale of > 5 min), whereas for the wild-type Cx26-GFP-4C (Beahm et al. Citation2006), there was extensive dye coupling over > 5 to 10 neighboring cells in all 5 trials within this same time period (data not shown). As a negative control, HeLa cells expressing Cx26M34Adel2-7-GFP gap junctions also did not exhibit dye transfer between adjoining cells. The M34A mutation has been shown to drastically reduce dye coupling to barely detectable levels (Oshima et al. Citation2003), so the expectation was that this double mutant should be incapable of Lucifer Yellow dye transfer. A more in depth analysis of whether these mutants completely eliminate or substantially reduce dye transfer or current is an avenue of our further investigations.
Figure 1 N-terminal sequences of human Cx26 genes studied in this work, and confocal and electron microscopy of Cx26M34Adel2-7 mutant. Sequence alignment of first 35 amino acid residues of two Cx26 N-termini, Cx26M34A and Cx26M34Adel2-7, is shown in (a). Both sequences contain the M34A point mutation. (b) Confocal micrograph of a transiently expressed Cx26M34Adel2-7-GFP in HeLa cells. Gap junction plaque formation between the adjacent cells was determined with the GFP fluorescence (arrows). The scale bar corresponds to 10 μ m. Isolated membrane fractions (c), purified channels (d), and 2D crystal (e) of Cx26M34Adel2-7 were negatively stained with uranyl acetate and observed by conventional EM. This deletion mutant appears to form gap junction plaques. Cx26M34Adel2-7 channels are stable in detergent solution and appear as homogeneous doughnut-shaped particles. The orthorhombic crystal lattice of Cx26M34Adel2-7 is the same packing as Cx26M34A 2D crystals (Oshima et al. Citation2007). Scale bars for (c to e) represents 100 nm.
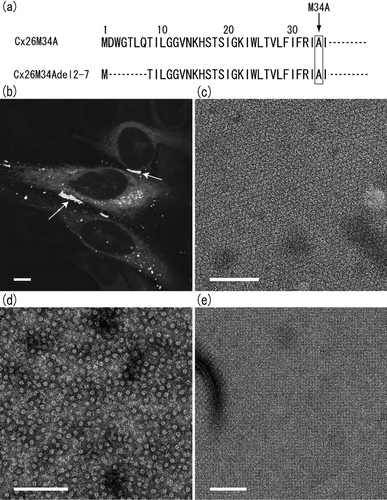
For our structural studies, a recombinant baculovirus of Cx26M34Adel2-7 was created and this mutant gap junction protein was overexpressed in Sf9 insect cells. In isolated membrane fractions, we confirmed that Cx26M34Adel2-7 forms oligomers that appear to form gap junction plaques () as is also observed in wild-type Cx26 or Cx26M34A preparations and is consistent with the idea that Cx26M34Adel2-7 gap junction channels expressed in Sf9 cells are identical to those comprised of double membranes in the native tissue or tissue culture cells.
Two-Dimensional Crystallization of Cx26M34Adel2-7 Channels
As previously reported, we used His6-tag affinity purification methods to purify proteins for crystallization (Oshima et al. Citation2007). The proteins were solubilized in dodecyl-maltoside (DDM) detergent solution, and purified using Ni-NTA beads binding to a hexa-histidine tag. Using conventional negative-stain electron miscroscopy (EM), the purified proteins exhibited the typical doughnut shape particles that are indistinguishable from those of wild type (). In our recently published work (Oshima et al. Citation2007), we described these particles to be hemichannels, consistent with previous studies showing that purified protein preparations obtained with DDM contain hemichannels (Stauffer et al. Citation1991; Stauffer Citation1995; Buehler et al. Citation1995). It has been reported in the studies of sheep lens gap junctions that hemichannels and paired channels were likely to be mixed depending on the detergents, but side views of hemichannel pairs were clearly identified with conventional EM (Kistler et al. Citation1993, Citation1994). So far, we have not identified any side views of gap junction channels using conventional negative-staining methods. Even so, we cannot exclude the possibility that the purified protein preparations also include fully docked, dodecameric gap junction channels because it is hard to distinguish “en face” views of those two structures in projection if both stand upright on the carbon support film. Because the 2D crystals of Cx26M34A contain only full gap junction channels and no single hemichannels (Oshima et al. Citation2007), it is possible that this crystal form may be produced from purified full channels, although proof of this still remains to be determined.
Cx26M34Adel2-7 channels were crystallized into 2D orthorhombic crystals similar to those obtained with the full-length Cx26M34A (). The images recorded with cryo-EM of Cx26M34Adel2-7 and Cx26M34A were processed to correct distortions, and merged to 10 Å resolution. The space group was p22121, suggesting that Cx26M34Adel2-7 contained three lipid bilayers, as is the case for Cx26M34A orthorhombic crystals. The unit cell parameters of Cx26M34Adel2-7 are a = 113.4Å (±1.01Å), b = 112.2Å (± 1.43Å), γ = 90° determined from eight images, while those of Cx26M34A are a = 112.4 Å (± 0.41 Å), b = 111.2 Å (± 0.55 Å), γ = 90° from 13 images. The differences between Cx26M34Adel2-7 and Cx26M34A lattice parameters are within the standard deviations of experimental measurement, so that we conclude that these two Cx26 crystal forms are isomorphous. The details of crystallographic data are described in .
TABLE 1 Summary of electron crystallographic data
Projection Structure of Cx26M34Adel2-7 Channels Demonstrates a Decreased Central Density in the Channel
The projection maps of Cx26M34A and Cx26M34Adel2-7 are shown in . Both crystallographically averaged projection maps have a typical hexameric channel feature that is very similar to that of crystallographically averaged Cx43-263T projection images (Unger et al. Citation1997; Cheng et al. Citation2003), even though the crystal packing is different (p6 versus p22121). Given that Cx26M34A 2D crystals were comprised of three lipid bilayers (Oshima et al. Citation2007), the best-preserved hexameric features seen in projection mainly reflect the hemichannel molecules in the middle (the second) lipid bilayer of the three. These hemichannels are protected by the top and bottom lipid bilayers from distortions or defects caused by sample preparation for cryo electron microscopy. On the other hand, hemichannels in the flanking (the first and the third) layers are more prone to preparation artefacts (Oshima et al. Citation2007) and, therefore, deviate more from ideal crystallographic symmetry, especially when viewed in projection where channels in the first and third layers are superimposed. Deviations from higher symmetry space groups were also the case for the Cx43-263T projection maps where the superposition of the two interacting hemichannels had the lower symmetry space group of p6 rather than p622 (Unger et al. Citation1997; Cheng et al. Citation2003).
Figure 2 Projection maps of Cx26M34A (a) and Cx26M34Adel2-7 (b), and difference map (c) depicted by contours in combination with grey scale. The difference map (c) is drawn by subtraction of Cx26M34Adel2-7 density from Cx26M34A density. The represented area corresponds to one unit cell. The cell dimension of the difference map is consistent with Cx26M34A crystals. Solid and dashed lines represent positive and negative densities, respectively, with 0.2σ step intervals for (a) and (b), 0.5σ step intervals for (c). Asterisks represent the density peaks corresponding to the pore-lining helix C in 3D map (see ).
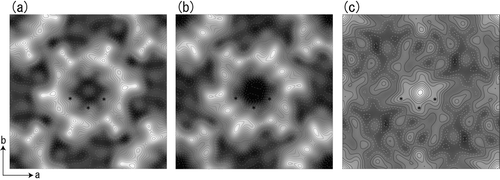
Comparison of the deletion mutant and Cx26M34A projection maps along with a difference map calculation provides a more quantitative analysis of the changes due to elimination of residues 2 to 7. The full-length Cx26M34A projection map displayed in has a significant peak in the channel pore. In contrast, Cx26M34Adel2-7 map shows considerably reduced peak inside the pore (). The difference map was calculated between Cx26M34A and Cx26M34Adel2-7 projection structures (). As is standard procedure in crystallographic analysis, this map was obtained by the subtraction of Cx26M34Adel2-7 amplitudes from Cx26M34A amplitudes and the phases from the Cx26M34A data set were used to synthesize the difference map. The highest difference peak, located right in the center of the pore, is equivalent to 4.5σ. This is consistent with the observation of a reduced density in the pore in Cx26M34Adel2-7 projection map (). Some peaks, as much as 2.0σ, were found between adjacent channels (). These may demonstrate different and unique interactions in the same crystal form across the two connexin mutants. However, without determining statistical confidence levels to establish the significance of these difference peaks, we can only conclude that the major difference is localized to the pore region.
This large structural change can be attributed to the deletion of the N-terminus because all other parts of the primary sequence are the same as the full-length construct (). It should be noted that the pore has an emptier appearance in the crystallographically averaged projection map, although a weak peak is sometimes observed in the pore for individual images of Cx26M34Adel2-7 (data not shown). Because crystallographic averaging enhances strong and consistent structural features, it is possible that a smaller, more flexible plug results in an average lower density value and, thus, enhances the difference peak. The cross-section of Cx26M34A 3D map (; also see Oshima et al. Citation2007) clearly shows that there is no density other than plug in each hemichannel along the central pore axis. The mass volume of the plug in this hemichannel () is 4402 Å3 when contoured at 2σ. Each hemichannel of Cx26M34Adel2-7 has lost 36 amino acid residues, which correspond to 5190 Å3 based on the volume/mass conversion coefficient of 1.35 g/cm3 (= 0.81 Da/Å3; Matthews 1968). This simplistic calculation is consistent with the volume measurement; however, it should be noted that the mass volume in the map depends on the contour threshold and does not provide further insight about which amino acids can be assigned to the plug density.
Figure 3 Cross-sectional view of the symmetrized 3D structure of Cx26M34A. Real space non-crystallographic six-fold symmetry has been applied to the original Cx26M34A 3D map (Oshima et al. Citation2007) to increase the signal to noise ratio, thereby enabling better visualization. At this contour level, the tertiary structure of the helices and extracellular domains is well-defined and a plug density is located in each hemichannel. The cross-sectional view of two docked hemichannels through the plugs (a) and the view of a hemichannel containing a whole plug (b) demonstrate that no other density is observed along the axis in the center of the pore. The central pore axis is represented by a perpendicular grey line. The pore-lining helices C are again labelled with asterisks as previously indicated in .
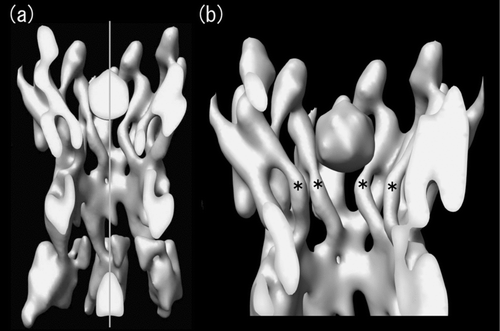
Taken together, these results indicate that the N-terminal sequence of Cx26 is likely to be part of the plug; however, other interpretations cannot be excluded. It is also possible that the conformations of the cytoplasmic loop and the C-terminus may be affected by the diminished sequence of the N-terminus. Interactions of the cytoplasmic loop and C-terminus have been proposed as a mechanism of pore closure for Cx43 (Delmar et al. Citation2004), and it has been shown that the interaction between the N-terminus and cytoplasmic loop of Cx26 may be essential for the junctional currents (Oh et al. Citation1999). A different part of the primary sequence may make up the plug but flexibility or variability in plug position or shape reduces the density in the vestibule seen in full-length channels. For example, individual channels or hemichannels in the crystal can have their plug at slightly different positions in the vestibule and with crystallographic averaging, the average density will be reduced. Given that the mass density of the plug is smaller than for the “core” (the tertiary structure of the transmembrane and extracellular domains), it is not possible to distinguish in a projection map at intermediate resolution between mass loss or positional mass variability.
In addition to the reduction in plug density seen in the deletion mutant map, densities corresponding to the major pore lining C helices of the deletion mutant also became weaker than those of the full-length connexin (, , asterisks). Based on the small spacing between the plug and the constriction site, interactions with the C set of helices are possible (Oshima et al. Citation2007). Small rearrangements of the C set of helices could occur thereby changing the pore diameter because of its interacting counterpart being lost. A 3D map of the deletion mutant and the comparison with the full-length Cx26M34A will be used to identify the conformational change caused by the contribution of the N-terminus.
To summarize, we determined the projection structures of two Cx26 constructs that differ in the N-terminus length by six amino acids. In the N-terminus deletion mutant Cx26M34Adel2-7, the density residing in the pore of the full-length channels was drastically reduced. Thus, we propose that the N-terminus of connexin may play a pivotal role for the physical blocking of gap junction channels. It is worth noting that although some parts of the connexin channel like the transmembrane helical domains have sixfold noncrystallographic symmetry, the packing of the channels is not sixfold symmetric, but has inherent twofold symmetry as dictated by the p22121 space group. Therefore, it is possible that not all six subunits symmetrically contribute to a plug. Finally, the comparison of the 3D structure of Cx26M34Adel2-7 with the full-length Cx26M34A along with more in-depth correlated functional assays will provide information about of the state of this deletion mutant and will likely provide important insights into understanding the complicated gating mechanisms of gap junctions.
This article is not subject to United States copyright laws.
The authors thank Jenny Pranskevich for preparing transfected HeLa cells and recording the image in Figure1b. They are also grateful to Amy Smock and Eric Bushong for assisting in performing the dye transfer experiments. This work is supported by Grants-in-Aid for Specially Promoted Research, Grant-in-Aid for the Global Century Centers of Excellence, Kyoto University, Japan Science and Technology Agency (JST) and Japan New Energy and Industrial Technology Development Organization (NEDO) (to Y.F.), National Institutes of Health grant GM065937 (to G.E.S.), and National Science Foundation grants MCB-0543934 (to G.E.S.) and RR04050 (to Mark Ellisman, National Center for Microscopy and Imaging Research, University of California at San Diego).
REFERENCES
- Beahm D L, Oshima A, Gaietta G M, Hand G M, Smock A E, Zucker S N, Toloue M M, Chandrasekhar A, Nicholson B J, Sosinsky G E. Mutation of a conserved threonine in the third transmembrane helix of α-and β-connexins creates a dominant-negative closed gap junction channel. J Biol Chem 2006; 281: 7994–8009
- Buehler L K, Stauffer K A, Gilula N B, Kumar N M. Single channel behavior of recombinant β2 gap junction connexons reconstituted into planar lipid bilayers. Biophys J 1995; 68: 1767–1775
- Cheng A, Schweissinger D, Dawood F, Kumar N, Yeager M. Projection structure of full length connexin 43 by electron cryo-crystallography. Cell Commun Adhes 2003; 10: 187–191
- The CCP4 suite: Programs for protein crystallography. Acta Crys 1994; D50: 760–763, Collaborative Computational Project, Number 4
- Crowther R A, Henderson R, Smith J M. MRC image processing programs. J Struct Biol 1996; 116: 9–16
- Delmar M, Coombs W, Sorgen P, Duffy H S, Taffet S M. Structural bases for the chemical regulation of Connexin43 channels. Cardiovasc Res 2004; 62: 268–275
- Fleishman S J, Unger V M, Yeager M, Ben-Tal N. A Cα model for the transmembrane α helices of gap junction intercellular channels. Mol Cell 2004; 15: 879–888
- Fujiyoshi Y, Mizusaki T, Morikawa K, Yamagishi H, Aoki Y, Kihara H, Harada Y. Development of a superfluid helium stage for high-resolution electron microscopy. Ultramicroscopy 1991; 38: 241–251
- Harris A L. Emerging issues of connexin channels: Biophysics fills the gap. Q Rev Biophys 2001; 34: 325–472
- Hwa H L, Ko T M, Hsu C J, Huang C H, Chiang Y L, Oong J L, Chen C C, Hsu C K. Mutation spectrum of the connexin 26 (GJB2) gene in Taiwanese patients with prelingual deafness. Genet Med 2003; 5: 161–165
- Kenna M A, Wu B L, Cotanche D A, Korf B R, Rehm H L. Connexin 26 studies in patients with sensorineural hearing loss. Arch Otolaryngol Head Neck Surg 2001; 127: 1037–1042
- Kistler J, Bond J, Donaldson P, Engel A. Two distinct levels of gap junction assembly in vitro. J Struct Biol 1993; 110: 28–38
- Kistler J, Goldie K, Donaldson P, Engel A. Reconstitution of native-type noncrystalline lens fiber gap junctions from isolated hemichannels. J Cell Biol 1994; 126: 1047–1058
- Kyle J W, Haemmerling B, Budzik J, Lopez-Domowicz D, Berthoud V M, Hanck D A, Beyer E C. A full length N-terminus is required for connexin37 channel function but not gap junction plaque formation. Biophys J 2007; 444a, Abstract Issue
- Matthews B W. Solvent content of protein crystals. J Mol Biol 1968; 33: 491–497
- Mese G, Londin E, Mui R, Brink P R, White T W. Altered gating properties of functional Cx26 mutants associated with recessive non-syndromic hearing loss. Hum Genet 2004; 115: 191–199
- Oh S, Rubin J B, Bennett M V, Verselis V K, Bargiello T A. Molecular determinants of electrical rectification of single channel conductance in gap junctions formed by connexins 26 and 32. J Gen Physiol 1999; 114: 339–364
- Oh S, Abrams C K, Verselis V K, Bargiello T A. Stoichiometry of transjunctional voltage-gating polarity reversal by a negative charge substitution in the amino terminus of a connexin32 chimera. J Gen Physiol 2000; 116: 13–31
- Oh S, Rivkin S, Tang Q, Verselis V K, Bargiello T A. Determinants of gating polarity of a connexin 32 hemichannel. Biophys J 2004; 87: 912–928
- Oshima A, Doi T, Mitsuoka K, Maeda S, Fujiyoshi Y. Roles of Met-34, Cys-64, and Arg-75 in the assembly of human connexin 26. Implication for key amino acid residues for channel formation and function. J Biol Chem 2003; 278: 1807–1816
- Oshima A, Tani K, Hiroaki Y, Fujiyoshi Y, Sosinsky G E. Three-dimensional structure of a human connexin26 gap junction channel reveals a plug in the vestibule. Proc Natl Acad Sci U S A 2007; 104: 10034–10039
- Purnick P E, Benjamin D C, Verselis V K, Bargiello T A, Dowd T L. Structure of the amino terminus of a gap junction protein. Arch Biochem Biophys 2000a; 381: 181–190
- Purnick P E, Oh S, Abrams C K, Verselis V K, Bargiello T A. Reversal of the gating polarity of gap junctions by negative charge substitutions in the N-terminus of connexin 32. Biophys J 2000b; 79: 2403–2415
- Sosinsky G E, Nicholson B J. Structural organization of gap junction channels. Biochim Biophys Acta 2005; 1711: 99–125
- Stauffer K A, Kumar N M, Gilula N B, Unwin N. Isolation and purification of gap junction channels. J Cell Biol 1991; 115: 141–150
- Stauffer K A. The gap junction proteins β1-connexin (connexin-32) and β2-connexin (connexin-26) can form heteromeric hemichannels. J Biol Chem 1995; 270: 6768–6772
- Unger V M, Kumar N M, Gilula N B, Yeager M. Projection structure of a gap junction membrane channel at 7 Å resolution. Nat Struct Biol 1997; 4: 39–43
- Unger V M, Kumar N M, Gilula N B, Yeager M. Three-dimensional structure of a recombinant gap junction membrane channel. Science 1999; 283: 1176–1180
- Unwin P NT, Ennis P D. Two configurations of a channel-forming membrane protein. Nature 1984; 307: 609–613
- Unwin P NT, Zampighi G. Structure of the junction between communicating cells. Nature 1980; 283: 545–549
- Verselis V K, Ginter C S, Bargiello T A. Opposite voltage gating polarities of two closely related connexins. Nature 1994; 368: 348–351