Abstract
Gap junctions are among the most widely distributed cell structures involved in cell-to-cell communication. Recently completed genome sequencing projects including species from all major phyla have demonstrated the existence of three distinct gene families, the connexins, pannexins, and innexins, as molecular building blocks of gap junctional communication. In the present study, the authors have addressed the molecular complexity of gap junction gene expression in the zebrafish retina, a remarkably complex sensory organ built by diverse neuronal subtypes. Using a combination of cDNA library and genomic DNA library screening and/or RACE technology, the authors have cloned, in addition to the four previously reported connexins, seven novel connexins and four pannexin transcripts resembling two pannexin genes. This result demonstrates the presence of two distinct gap junction type gene families and indicates a remarkable molecular and functional diversity of gap junction–mediated coupling in the fish retina.
INTRODUCTION
Gap junctions are channel-forming structures at the sites of plasma membrane contacts allowing adjacent cells direct metabolic and electrical coupling. They belong to the most conserved structures in multicellular metazoans and can be found from hydra to man (Filshie and Flower Citation1977; Hertzberg and Skibbens Citation1984; Bauer et al. Citation2005; Fraser et al. Citation1987). Gap junctions are present between most cell types and actively participate in many aspects of the cellular life cycle, ranging from cell growth to cell death (for recent reviews see Vinken et al. Citation2006; Meier and Dermietzel Citation2006; Leithe et al. Citation2006).
Sequencing of the genomes of different species representing all major phyla has expanded the knowledge about the molecular diversity of gap junction type genes. To date, three distinct gene families are considered as the molecular building blocks of gap junctions. Connexins (Cx) and pannexins (Panx) are both found in the phylum chordata (Panchin Citation2005). Innnexins (Inx) form gap junctions in invertebrates (Bauer et al. Citation2005; Barbe et al. Citation2006). Although pannexins and innexins are distantly related, no sequence homology is found to the connexins (Barbe et al. Citation2006; Dahl and Locovei Citation2006).
The phylogenetic relationship between connexins of different species has been recently addressed in several studies. A comparison of the connexin gene families from human to zebrafish revealed an expanded family of ∼ 37 connexins in the zebrafish, with ∼ 23 zebrafish relatives of 16 mammalian connexins and 14 connexins apparently unique to the zebrafish (Eastman et al. Citation2006; Cruciani and Mikalsen Citation2007). The expanded gene family is most likely caused by a genome duplication in ancient teleost fish that happened during a short period of time ca. 200 million years (Myr) ago (Taylor et al. Citation2001, Citation2003). In contrast to connexins, the molecular diversity of pannexin genes is restricted and current evidence supports the existence of three members in higher vertebrates (Bruzzone et al. Citation2003; Baranova et al. Citation2004).
Because the complexity of the connexin and pannexin genes found in the fish genome has been established, it is evident that almost 40 genes encoding gap junction type proteins exist in the fish. Now it is of utmost importance to establish the physiological roles of expressed gap junction genes during the fish live cycle. One model to address this question is the analysis of neuronal networks coupled by gap junctions in the vertebrate retina. Here, gap junctional communication has been proposed to play a key role during the development of the retina where its roles may include control of neurogenesis, cell specification, synaptogenesis, and the synchronization of the spontaneous electrical activities required for the sharpening of central visual projections. In the adult, the plastic nature of gap junction channels is thought to contribute to the adaptation of the retinal circuitry to optimize visual processing (Becker et al. Citation1998; Weiler et al. Citation2000). Loss-of-function models in the mouse support a prominent role of gap junction coupling in processing visual information. Cx36 or Cx45 knockout mice show deficiencies in the rod or rod/cone pathway (Guldenagel et al. Citation2001; Demb and Pugh Citation2002; Robson et al. Citation2004; Maxeiner et al. Citation2005). The knock out of the Cx57 gene, the murine ortholog of the zebrafish drCx55.5, leads to a reduction of the receptive fields of horizontal cells (HCs) (Shelley et al. Citation2006). In a focal retinal lesion model, Cx36 has been shown to contribute to secondary cell loss after injury (Striedinger et al. Citation2005). Nevertheless, it is debatable whether the mouse is the appropriate animal model to study vision. Mice do not primarily rely on vision and color perception is restricted to dichromatic vision, which differs from the trichromatic vision of primates including humans (Haverkamp et al. Citation2005; Conway Citation2007; Jacobs et al. Citation2007). In contrast, the zebrafish is endowed with a rich color vision and largely relying on vision during its life cycle. This suggests that the fish could be a more comparable model to humans (Goldsmith and Harris Citation2003). Furthermore, the genetic characterization, easy genetic manipulation, and available visual behavior tests make the fish an attractive model to study the function of gap junction proteins in the neuronal plasticity of the retina.
As a first step towards this goal, we have reported the cloning of four connexin genes expressed in the retina in two previous studies (Dermietzel et al. Citation2000; Zoidl et al. Citation2004). In the present study, we extend our previous cloning work and demonstrate the expression of additional seven zebrafish (Danio rerio [dr]) connexin transcripts expressed in the retina aside of the well-known Cx35 (McLachlan et al. Citation2003) and Cx43 (Cheng et al. Citation2003). Furthermore, we demonstrate the cloning and expression of drPanx1 and drPanx2 isoforms expressed in the retina. Our results demonstrate a complex molecular and expression diversity of two families of gap junction type genes in the retina of the adult zebrafish Danio rerio.
MATERIAL AND METHODS
Animals
Zebrafish were kept at 28°C in aerated tanks filled with tap water circulating through a bacterial filter system. The fish were kept on a 14-h on/10-h off light-dark cycle. All animal experiments were carried out according to the guidelines of the German Animal Protection Law in its present version (1998). All experiments with animals were kept to the necessary minimum.
Cloning
The original screening of a zebrafish retina cDNA (λ gt11) and a zebrafish genomic (λ FIX II; Stratagene, CA) library using the 32P-ATP radiolabeled probes frag26 and frag50, the subcloning and sequencing procedures have been described (Dermietzel et al. Citation2000). Incomplete sequences were completed using SMART RACE (Rapid Amplification of cDNA Ends) cDNA Amplification Kit technology (Clontech, CA). This method is used to incorporate synthetic adaptors into both the 5′ and 3′ ends of adult retina cDNA during synthesis, combining SMART first-strand cDNA synthesis technology with a powerful suppression PCR protocol, which vastly reduces the background amplification that is commonly associated with RACE protocols (Cai and Lytton Citation2004). The RNA extraction from freshly dissected animals used for SMART RACE was performed using the RNeasy Mini Kit or Oligotex Direct mRNA Mini Kit as described (Qiagen, Hilden, Germany). At least three independent cDNA clones were verified by overlapping sequencing for each connexin and pannexin prior submission to GenBank (http://www.ncbi.nlm.nih.gov/Genbank/submit.html).
Expression Analysis
The RNA extraction from freshly dissected animals using the RNeasy Mini Kit (Qiagen), the test of RNA integrity and the cDNA synthesis conditions of DNAseI-treated RNAs were performed as described previously (Ray et al. Citation2005). Quantitation reverse transcriptase–polymerase chain reaction (qRT-PCR) analysis was performed using the cDNA equivalent reverse transcribed from 20 ng total RNA in a 20-μ l reaction volume. CDNA synthesis setups omitting RT (RT− condition) served as controls for genomic DNA contaminations. Primers for qRT-PCR analysis were designed using the Vector NTI program (Invitrogen, Karlsruhe, Germany) and selected for universal reaction conditions. All primer pairs had a calculated Tm of 60°C to 64°C and produced single amplification products of a calculated Tm > 78°C as verified by melting point analysis. The amplicons generated by real-time RT-PCR are listed in . Quantification of 16s rRNA served as internal standard. Syber Green I reaction conditions were as recommended by the manufacturer for the SYBR Green I No ROX amplification kit (Eurogentec, Cologne, Germany). Real-time RT-PCR was performed on a DNA Engine Opticon2 detection system (Bio-Rad Laboratories, CA), applying the two-step amplification protocol mentioned in our previous studies (Zoidl et al. Citation2004; Ray et al. Citation2005). Data represent at least two independent sets of samples with each sample analyzed in triplicates. Relative expression ratios were calculated using the expression levels detected in the retina as reference. The statistical analysis was performed using the Relative Expression Software Tool (REST) software (Pfaffl et al. Citation2002).
Sequence Analysis
All sequences obtained by retrieval from the NCBI or ENSEMBL databases were directly processed using the Vector NTI software (Invitrogen, Karlsruhe, Germany). Sequences deriving from the on campus sequencing core facility (Department of Molecular Neurochemistry, Ruhr-University Bochum, Germany) were processed with Sequence Scanner 1.0 (Applied Biosystems, CA) prior to analysis with Vector NTI software. The same program was used for all sequence analysis, alignments, and phylogenetic tree calculations. BLAST analysis (BLASTN, BLASTP) was performed using the NCBI (http://www.ncbi.nlm.nih.gov/) or ENSEMBL gateway (http://www.ensembl.org/index.html). Sequence submission to the NCBI GenBank was performed using the BankIt gateway (http://www.ncbi.nlm.nih.gov/BankIt). Some of the primary sequence analyses were performed using the software tools implemented on the ExPASy Web site (http://www.expasy.org/). The analysis of real-time PCR data was performed using the Relative Expression Software Tool (REST-MCS; http://www.gene-quantification/) and MS-Excel.
RESULTS
Cloning of Seven Novel Connexin Genes Expressed in the Adult Zebrafish Retina
We have previously reported the cloning of neuronal connexins starting from an enriched preparation of fish horizontal cells followed by PCR amplification of ortholog genes of the murine connexins Cx26, Cx43, and Cx50 using degenerate primers (Dermietzel et al. Citation2000). This strategy was combined with the screening of a λ gt11 cDNA library prepared from zebrafish retina, a λ FIX II zebrafish genomic DNA library, and/or RACE technology to complete partial gDNA and/or cDNA sequences obtained during early stages of this screen procedure. A number of connexin genes were successfully isolated and we have initially reported the isolation and retinal expression of drCx27.5 (AF304049), drCx44.1 (AF304050), and drCx55.5 (AF304048). Sixteen incomplete sequences identified with the probes frag26 and frag50 (Dermietzel et al. Citation2000) were finished afterwards and after excluding duplicate genes deposited in the NCBI GenBank database (). The connexin coding regions deposited were drCx28.6 (AY135443), drCx33.8 (AY135444), drCx34.4 (AY135446), dr39.9 (AY135445), and drCx48.5 (AF465751). The full-length coding region of drCx34.4 encompasses 900 bp and encodes a protein of 299 amino acids (aa). The ortholog genes in higher mammals are the connexin genes Cx30.3/31.1, genes with no known expression in the mammalian retina but profound correlation with erythrokeratodermia variabilis (EKV), a mostly autosomal dominant disorder of keratinization (Plantard et al. Citation2003; Common et al. Citation2005).
TABLE 1 Summary of 13 connexin and 2 pannexin genes expressed in the zebrafish retina
Database searches using the BLAST algorithms implemented at the NCBI and ENSEMBL Web sites revealed the localization of the drCx34.4 gene locus on chromosome (Chr) 17 (). A single mRNA transcript is predicted and the coding region is located on a single coding exon, a property that is typical for most of the known connexins of higher vertebrates (Eiberger et al. Citation2001). Similarly, we retrieved the predicted positions of the other connexin gene loci by querying the public databases. It turned out that drCx28.6 (chromosome 17), drCx33.8 (chromosome 9), dr39.9 (chromosome 5), and drCx48.5 (chromosome 9) did not adhere to the general rule that connexin proteins derive from a single coding exon. This is still a finding worth to note because in higher vertebrates, only the Cx36 protein is encoded by two exons (Belluardo et al. Citation1999).
TABLE 2 Chromosomal localization of retinal connexin and pannexin genes and summary of PCR products generated by RT-PCR or real-time RT-PCR
The ENSEMBL database predicts three drCx33.8 transcripts with three proteins in the size range from 253 to 297 aa (). The cDNA sequence experimentally found in our analysis represented the 297-aa isoform. The predicted drCx33.8 proteins are orthologs of Cx26, a gene most recognized for its association with human inherited forms of deafness (Qu et al. Citation2007). Single transcripts of 1305 and 1062 nucleotides (nt) were isolated for the connexins drCx48.5 and drCx39.9. The two transcripts overlap with the transcripts predicted by ENSEMBL (Curwan et al. 2004) and the proteins of 435 and 354 aa are encoded by two exons similarly to Cx35/Cx36. The human ortholog of drCx48.5 is Cx46, a gap junction gene expressed in the murine lens (Paul et al. Citation1991; Pal et al. Citation2000). In contrast, orthologs of drCx39.9 are restricted to fish, amphibian, and birds but cannot be found in mammals (Cruciani and Mikalsen Citation2007). A single transcript was found for drCx28.6, but the predicted protein of 265 aa is predicted to be encoded by four exons. In our experiments we identified a shorter protein of 252 aa. This discrepancy between our experimental data and the predicted open reading frame needs further investigations. Ortholog proteins of drCx28.6 are only found in fish and Cruciani and Mikalsen have hypothesized recently (2007) that this connexin branch originated in fish only or was lost in higher vertebrates.
Comparison of Connexin-Specific Expression in Adult Zebrafish Tissues
In order to prove that the seven connexin sequences found originated from expressed transcripts, we performed tissue specific mRNA expression analysis using qRT-PCR methodology as described previously (Ray et al. Citation2005). The expression of drCx27.5, drCx35, carpCx43, drCx44.1, and dr48.5 in the developing or adult zebrafish has been shown previously (Dermietzel et al. Citation2000; Cason et al. Citation2001; Cheng et al. Citation2003; McLachlan et al. Citation2003), but quantitative expression data are elusive. Therefore, we included the novel and previously characterized connexins, including drCx35.1 and drCx43, in our analysis. The amplicon lengths generated for each connexin and the relative position of each amplicon with respect to the transcript sequences deposited in GenBank is summarized in .
Our qRT-PCR results demonstrated that all connexins were expressed in the retina but the expression pattern is variable with respect to tissue distribution (). DrCx35.1, drCx34.4, drCx44.1, and drCx48.5 expression was most abundant in the retina, with drCx35.1 having an expression restricted to nervous system tissues including retina, brain, and spinal cord. The restricted expression of drCx35.1 is consistent with the restricted expression previously reported for drCx35/Cx36 (Condorelli et al. Citation1998; O'Brien et al. Citation1998; Belluardo et al. Citation1999; McLachlan et al. Citation2003).
Figure 1 Tissue-specific expression of retinal connexins. QRT-PCR analysis of 9 connexins showed expression in the retina, whereas expression in the other organs varied. The brain was defined as reference condition and all other tissues as conditions I to VI. All cDNA preparations were assessed by PCR using primers specific for 16s rRNA. This analysis confirmed that comparative amounts of cDNA were included in all PCR reactions. No template controls were negative and a second PCR control reaction (with 16s rRNA primers or specific primers) omitting reverse transcription produced no amplification products, indicating the absence of genomic DNA contamination in the mRNA samples (data not shown). Ct values (retina) were in the range of 27.8 (drCx35.1) to 37.6 (drCx39.9), with a mean Ct value of 15.3 for 16s rRNA. Values are expressed on a 2-log scale. Error bars represent 2-log standard errors.
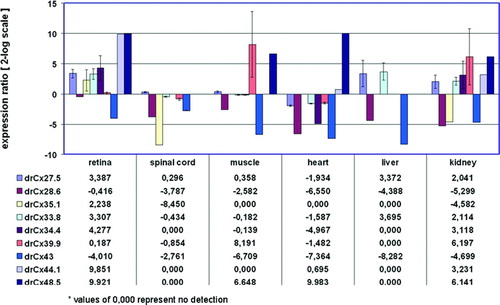
DrCx28.6 and drCx43 expression was up to 300-fold reduced in some tissues when compared to the brain. This result is not surprising taking into account that Cx43 resembles the major connexin expressed in astrocytes of the central nervous system (CNS) (Rash et al. Citation2001).
DrCx48.5 showed a prominent expression in the retina and heart (> 900-fold), with lower levels in kidney and muscle. The abundant expression found in the heart is consistent with the role of drCx48.5 in normal heart development (Cheng et al. Citation2004). It has been previously reported that drCx48.5 is expressed in the lens (Cheng et al. Citation2004). This result was confirmed by our analysis (data not shown).
DrCx39.9 was the only example of a protein found most abundant outside the nervous system, with high steady-state levels in muscle (> 220-fold) and kidney (> 70-fold). In summary, the expression analysis shown in confirmed the expression of all connexins in the retina and demonstrated that individual connexins have distinct expression patterns.
Identification of Orthologs of the Cx59/Cx62 Gene Families from Danio rerio
One of the results of our initial screening approach was the identification of two related connexins found in the retina when we tried to identify the Cx26 ortholog originally hypothesized by the works of Kamermans et al. (Citation2001) and Jansen-Bienhold et al. (Citation2001a, Citation2001b). The two genes found were termed drCx52.6 and drCx55.5. The ENSEMBL database predicted a localization onchromosomes 20 and 16. In case of drCx55.5 a single transcript of 1497 nt was retrieved containing a single coding exon of 498 aa (). In contrast, two transcripts are predicted for the drCx52.6 gene locus. Both transcript isoforms contain a total of five exons and encode for two distinct protein isoforms of 466 aa (two exons) and 256 aa (three exons). At present, our experimental data support the expression of a 1401-nt transcript encoding for the 466 aa protein only. Cx52.6 and Cx55.5 proteins have an amino acid identity score of 45% calculated using the full-length protein sequences. It is now considered that the two connexins are orthologous to either human Cx62 (drCx52.6) or Cx59 (drCx55.5) (Cruciani and Mikalsen Citation2007).
Most recently, we have shown that the drCx52.6 and drCx55.5 proteins were expressed in HA-HCs but never HB-HCs of the retina (Shields et al. Citation2007). This result suggested that other gap junction–type genes may exist that could be potential candidates expressed in the second horizontal cell subtype of the retina. Thus, we reinvestigated unfinished sequencing data obtained during the early stages of our cloning strategy, recent entries deriving from the zebrafish genome project, and used RT-PCR and/or RACE technologies to obtain full-length coding regions of relatives of drCx52.6 and drCx55.5. This strategy was successful and we cloned two additional cDNA clones directly from retina cDNAs or retina cDNA libraries representing the recently reported zebrafish connexins drCx52.7 and drCx52.9 (). Our cDNA clones are highly homologous to the sequences found in the ENSEMBL database, except for the fact that the drCx52.9 cDNA cloned by us encoded for a protein of 477 aa and not 380 aa. Because the sequences deposited by us in GenBank are experimental probes derived from the overlapping sequencing of a minimum of three individual cDNA clones, we believe that the use of the PCR technology is not the cause for this difference. More likely, the apparent difference reflects an incomplete assembly of the zebrafish genome sequence.
Alignment of the four sequences with the human Cx59 and human Cx62 sequences confirmed a high degree of conservation in the amino-terminal domain, the first two transmembrane regions and the conserved cysteine motifs found in the extracellular domains EL1 and EL2 (). As expected, the carboxy-terminal domains were less conserved, supporting the idea that this part of the proteins may account for the major functional differences between the different connexin proteins. Amino acid identity scores calculated relative to drCx52.6 showed values of 60% for drCx52.7 and 46% and 45% for drCx52.9 and drCx55.5, respectively. DrCx52.6 (49%) and drCx52.7 (46%) were most similar to huCx62, drCx52.9 (44%) and drCx55.5 (45%) to huCx59 (). The identification of two pairs of related genes forming a subgroup of related connexins is consistent with the gene duplication event that teleost fish have undergone during evolution. However, in mouse, only the ortholog of human Cx62, the Cx57 gene (Hombach et al. Citation2004), was identified, suggesting that the mouse may have lost the ortholog of human Cx59 during evolution. The functional correlation of Cx57 with vision has been demonstrated formally recently when Shelley and coworkers proved that loss of Cx57 in horizontal cells leads to a reduction in receptive fields (Shelley et al. Citation2006).
Figure 2 Relationship of the human Cx59/Cx62 with zebrafish connexins. (A) The amino acid alignment was created using the Clustal W algorithm (Thompson et al. Citation1994) and the BLOSUM62mt2 matrix. The color code for identical amino acids, conservative amino acid exchanges, or blocks of similar amino acids are indicated. The position of the four transmembrane domains TM1 to TM4 were calculated using drCx52.6 as reference and the TMpred program implemented on the ExPasy.ch homepage. The conserved cysteine motifs in the extracellular loops EL1 and EL2 are marked by arrows. (B) A guide tree, resembling a phylogenetic tree, was built using the neighbor-joining method (NJ) (Battilana et al. Citation2002). The NJ method works on a matrix of distances between all pairs of sequence to be analyzed. These distances are related to the degree of divergence between the sequences. The guide tree is calculated after the sequences were aligned (see above). The values displayed in parenthesis following the molecule name displayed on the tree are the amino acids identity scores calculated by AlignX (VectorNTI).
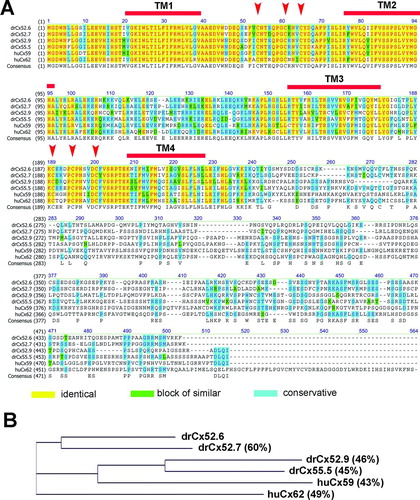
Tissue-Specific mRNA Expression of Orthologs of the Cx59/Cx62 Gene Families
Quantitative RT-PCR analysis shown in the relative expression ratio plot () demonstrated that drCx52.6 and drCx55.5 expression is most abundant in the retina. Due to the high sensitivity of the real-time PCR technology, we were able to detect the previously unrecognized low abundant expression of drCx52.6 in the brain (−787-fold) and a significantly reduced expression of drCx55.5 in a range from −13-fold (spinal cord) to −2318-fold (heart). This quantitative expression analysis supported earlier data generated on the basis of conventional RT-PCR technology showing expression of drCx52.6 and drCx55.5 in the retina only (Dermietzel et al. Citation2000; Zoidl et al. Citation2004b). The very low levels detected in other tissues may represent basal constitutive promoter activity as has been described for Cx32 (Bai et al. Citation1993, Citation1995) or leaky promoter activity (Uchida et al. Citation2006).
Figure 3 Relative expression ratio plot of Cx59/Cx62 related connexins in adult zebrafish tissues. The relative expression was calculated after real-time RT-PCR using the REST-MCS software based on the original concept introduced by Pfaffl et al. (Citation2002a). The retina was defined as reference condition and all other tissues as conditions I to VI. Crossing point values obtained for the 16s rRNA gene served as internal standards and all other genes as target genes 1 to 4. Ct values (retina) were in the range of 29.7 (drCx55.5) to 34.5 (drCx52.7). Values are expressed on a 2-log scale. Error bars represent 2-log standard errors.
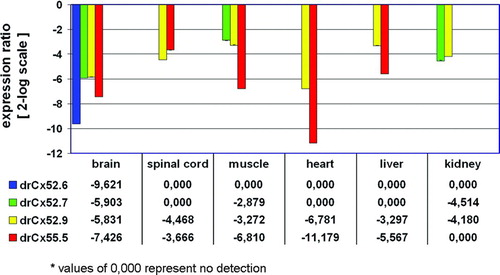
Next, we compared the expression profiles of drCx52.7 and drCx52.9 with those of the two other connexins in neuronal and non-neuronal tissues using real-time RT-PCR (). As shown, the expression of Cx52.7 and Cx52.9 is most abundant in the retina. The range of expression reduction found is similar to drCx52.6 and drCx55.5. A low level of drCx52.9 expression was found in all tissues. Cx52.7 was found at low levels in muscle and kidney. This result does not formally exclude the possibility that the four genes might be abundantly expressed elsewhere during development, in tissues not tested or at high levels in a small subset of cells within an organ and thus failing detection. Nevertheless, our data are in favor of a prominent and restricted mRNA expression in the retina. This result is remarkable and gives rise to important questions concerning the transcriptional regulation, sites of localization and physiological function(s) of drCx52.7 and drCx52.9 in the retina.
Cloning of Pannxin Genes Expressed in the Zebrafish Retina
RT-PCR and RACE technology was used to clone Pannexin1 (drPanx1) and Pannexin2 (drPanx2) transcripts from adult zebrafish retina. A single drPanx1 cDNA (identical to NM_200916) was successfully cloned when retina cDNA was used as template. The cDNA obtained was 99% identical to the previously reported pannexin1 sequences deposited in the NCBI and ENSEMBL databases. The single transcript encodes for a protein of 417 aa and is 54% and 55 % identical to the human or mouse ortholog. The protein is encoded by six exons as predicted by ENSEMBL () and the gene locus found on chromosome 15 ().
The cloning of the drPanx2 transcripts led to the discovery of three transcript isoforms. In order to gain insight into the origin and molecular diversity of these distinct transcripts, the cDNA cloning approach was extended into the 5′ and 3′ untranslated regions using SMART RACE technology. The names assigned for these transcripts were drPanx2-A (bankit1030489), drPanx2-B (bankit1030497), and drPanx2-C (bankit1030502). The transcript size for drPanx2-A, drPanx2-B, and drPanx2-C is 2630, 2385, and 2928 nt, respectively, and they encode for proteins of the following size: drPanx2-A (580 aa), drPanx2-B (604 aa), and drPanx2-C (652 aa). The transcripts were mapped individually on the zebrafish chromosome 18 using the BAC clone BX957245 as reference template (). As summarized in , we identified five exons for isoform A, six exons isoform B, and seven exons for isoform C. These exons are variable in length, although the various isoforms share common regions.
Figure 4 Schematic representation of the exon organization of the drPanx2 isoforms. The localization of drPanx2-IsoA,-IsoB and-IsoC exons was drawn relative to the sequence of the bacterial artificial chromosome (BAC) clone BX957245. The positions of individual exons were identified by alignment of Panx2 sequences with the BAC clone using by AlignX (VectorNTI). Note that the size of individual clones is not drawn to scale.
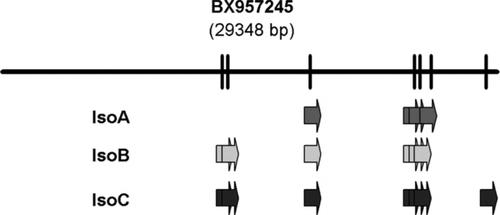
TABLE 3 Exon organization of the three drPanx2 transcript isoforms expressed in the retina and brain of the adult zebrafish
Next we aligned the protein sequences and calculated the identity values of the three drPanx2 isoforms relative to the known human Panx2 protein (). DrPanx2-A was most closely related to human Panx2, with an identity score of 71%. DrPanx2-B and drPanx2-C had scores of 68% and 66%. In comparison, human and mouse Panx2 were 88% identical at the amino acid level over the entire protein sequences. Most conserved regions between zebrafish and human Panx2 were the transmembrane regions as well as the first extracellular loop and the cyoplasmic loop. Prominent alterations were found in particular in the carboxy-terminal domain. The amino-terminal domain was expanded by 11 amino acids in frame with the previously defined translation start site of human or mouse Panx2. Furthermore, we were unable to identify the second conserved cysteine motif in the extracellular loop EL2 in all three transcript isoforms. These findings need further exploration.
Figure 5 Relationship of the human Panx2 with three zebrafish Panx2 transcript isoforms. The amino acid alignment was created using the Clustal W algorithm and the BLOSUM62mt2 matrix as described above. The color code for identical amino acids, conservative amino acid exchanges, or blocks of similar amino acids is indicated. The position of the four transmembrane domains TM1 to TM4 is indicated. The conserved cysteines in the extracellular loops EL1 and EL2 are marked by red arrows. Note the position of the second cysteine in EL2 (black arrow), which is replaced by a serine motif in all three transcript isoforms. The expansion of the amino terminal domain (11 amino acids) is highlighted.
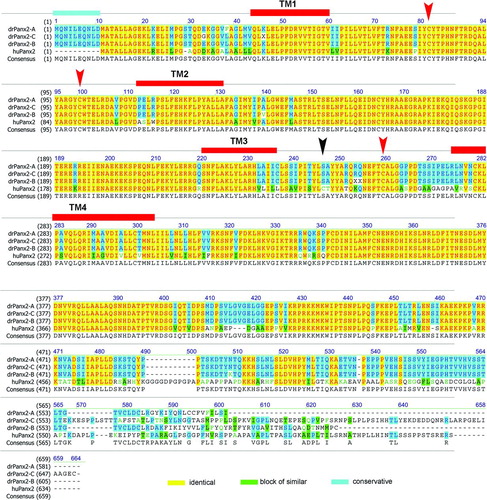
Finally, we used qRT-PCR to quantify pannexin expression in neuronal and non-neuronal tissues as shown above for the fish connexins (). Primers were selected to amplify unique amplicons listed in to distinguish between the different transcripts. The result of the qRT-PCR is presented as the expression ratio of the retina versus all other tissues. DrPanx1 was detected in all zebrafish tissues examined including the retina. This expression was slightly up-regulated in muscle (1.44 ± 0.66-fold) and kidney (1.21 ± 0.74-fold), and most reduced in the heart (−6.84 ± 0.06-fold). The pattern found was similar to that observed for the murine or human Panx1 genes (Bruzzone et al. Citation2003; Baranova et al. Citation2004; Ray et al. Citation2005a). In contrast to the widespread expression of drPanx1, the expression of the three drPanx2 isoforms was restricted to the nervous system. The drPanx2-A isoform was only found in the brain, which prevented the calculation of a ratio score as shown in . The drPanx2-B isoform was most widely expressed in the nervous system, with abundant levels in the brain (7.26 ± 2.12-fold). Isoform C was found exclusively in the retina and brain (2.94 ± 0.33-fold increase). Interestingly, we failed to obtain any transcript for isoform A in the retina, although two different primer pairs were used and a low level expression was detectable in the brain, thus excluding a possible amplification problem. At present, we cannot explain why drPanx2-A was represented in the retina cDNAs used during the cloning procedures. Our results prove the expression of drPanx1 and the two drPanx2 transcript isoforms B and C in the retina. In summary, we have shown that the zebrafish is endowed with a complex and divers pattern of gap junction gene expression.
Figure 6 Relative expression ratio plot of pannexin expression in adult zebrafish tissues. The relative expression of pannexins was calculated after real-ime RT-PCR using the REST-MCS software based on the original concept introduced by Pfaffl et al. (Citation2002a). The retina was defined as reference condition and all other tissues as conditions I to VI. Crossing point values obtained for the 16s rRNA gene served as internal standards and all other genes as target genes 1 to 4. Ct values (retina) were in the range of 28.8 (drPanx2-IsoC) to 33.5 (drPanx2-IsoB). Values are expressed on a 2-log scale. Error bars represent 2-log standard errors.
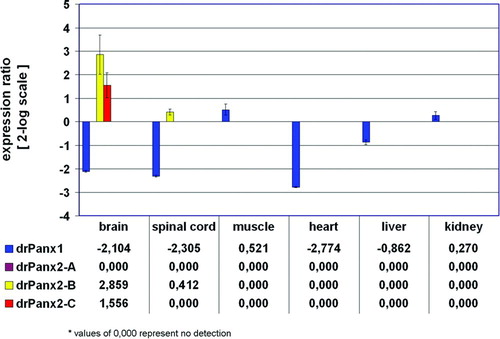
SUMMARY AND DISCUSSION
The evolutionary relationship of the connexin gene family of the zebrafish Danio rerio has been recently reported in great detail (Eastman et al. Citation2006; Cruciani and Mikalsen Citation2007). These studies demonstrated a particular complexity of connexins in the teleost, with ∼ 37 connexins found instead of ∼ 21 in human or the mouse genome. The cause for this diversity is most likely due to a genome duplication that the fish have undergone approximately 200 million years ago (Taylor et al. Citation2001, Citation2003). Aside from evolutionary constraints, one of the challenges of gap junction biology in the zebrafish is to place the large number of connexins into a physiological context. This study is a first step towards this goal, because we were able to allocate in this and two previous studies (Dermietzel et al. Citation2000; Zoidl et al. Citation2004b) the expression of 11 connexins in the retina. Together with the previously cloned Cx35 (O'Brien et al. Citation1998; McLachlan et al. Citation2003), at least 12 of the ∼ 37 known zebrafish connexin genes are expressed in the retina. This result might not be a big surprise taking into account the cellular complexity and high degree of electrical coupling by gap junctions of the retina. The molecular diversity identified with distinct expression patterns found ranging from a very restricted expression (drCx27.5, drCx52.6-drCx55.5) to a more general expression (drCx28.6) suggests that some connexins might have highly specialized functions, whereas others may serve more general functions.
Aside of connexin transcripts, we found the expression of two members of the second family of gap junction genes in the retina, confirming the retinal expression of murine Panx1 and Panx2 mRNA described previously (Dvoriantchikova et al. Citation2006). Recent evidences suggest that murine Panx1 may serve a function as a hemichannel forming (Zoidl et al. Citation2007), ATP sensitive conduit (Locovei et al. Citation2006a, Citation2006b; Huang et al. Citation2007) that can operate in cooperation with purinergic receptors (Pelegrin and Surprenant Citation2006). Thus it is suggestive to speculate that at least Panx1 may serve a function distinct from classical electrotonic coupling in the retina. On the basis of the molecular diversity of connexin and pannexin genes expressed in the retina, it will be now of utmost importance to perform localization studies as the next step towards an understanding of the functions of the connexins and pannexins described to gain insight into their contribution to the visual process.
This work was supported by funding of the DFG and SFB509 (RD, GZ) and a PhD grant of the Graduate School 726 (SB).
REFERENCES
- Bai S, Schoenfeld A, Pietrangelo A, Burk R D. Basal promoter of the rat connexin 32 gene: identification and characterization of an essential element and its DNA-binding protein. Mol Cell Biol 1995; 15: 1439–1445
- Bai S, Spray D C, Burk R D. Identification of proximal and distal regulatory elements of the rat connexin32 gene. Biochim Biophys Acta 1993; 1216: 197–204
- Baranova A, Ivanov D, Petrash N, Pestova A, Skoblov M, Kelmanson I, Shagin D, Nazarenko S, Geraymovych E, Litvin O, Tiunova A, Born T L, Usman N, Staroverov D, Lukyanov S, Panchin Y. The mammalian pannexin family is homologous to the invertebrate innexin gap junction proteins. Genomics 2004; 83: 706–716
- Barbe M T, Monyer H, Bruzzone R. Cell-cell communication beyond connexins: the pannexin channels. Physiology 2006; 21: 103–114
- Battilana J, Bonatto S L, Freitas L B, Hutz M H, Weimer T A, Callegari-Jacques S M, Batzer M A, Hill K, Hurtado A M, Tsuneto L T, Petzl-Erler M L, Salzano F M. Alu insertions versus blood group plus protein genetic variability in four Amerindian populations. Ann Hum Biol 2002; 29: 334–347
- Bauer R, Loer B, Ostrowski K, Martini J, Weimbs A, Lechner H, Hoch M. Intercellular communication: the Drosophila innexin multiprotein family of gap junction proteins. Chem Biol 2005; 12: 515–526
- Becker D, Bonness V, Mobbs P. Cell coupling in the retina: patterns and purpose. Cell Biol Int 1998; 22: 781–792
- Belluardo N, Trovato-Salinaro A, Mudo G, Hurd Y L, Condorelli D F. Structure, chromosomal localization, and brain expression of human Cx36 gene. J Neurosci Res 1999; 57: 740–752
- Bruzzone R, Hormuzdi S G, Barbe M T, Herb A, Monyer H. Pannexins, a family of gap junction proteins expressed in brain. Proc Natl Acad Sci U S A 2003; 100: 13644–13649
- Cai X, Lytton J. Molecular cloning of a sixth member of the K+-dependent Na+/Ca2+ exchanger gene family, NCKX6. J Biol Chem 2004; 279: 5867–5876
- Cason N, White T W, Cheng S, Goodenough D A, Valdimarsson G. Molecular cloning, expression analysis, and functional characterization of connexin44.1: A zebrafish lens gap junction protein. Dev Dyn 2001; 221: 238–247
- Cheng S, Christie T, Valdimarsson G. Expression of connexin48.5, connexin44.1, and connexin43 during zebrafish (Danio rerio) lens development. Dev Dyn 2003; 228: 709–715
- Cheng S, Shakespeare T, Mui R, White T W, Valdimarsson G. Connexin 48.5 is required for normal cardiovascular function and lens development in zebrafish embryos. J Biol Chem 2004; 279: 36993–37003
- Common J E, O'Toole E A, Leigh I M, Thomas A, Griffiths W A, Venning V, Grabczynska S, Peris Z, Kansky A, Kelsell D P. Clinical and genetic heterogeneity of erythrokeratoderma variabilis. J Invest Dermatol 2005; 125: 920–927
- Conway B R. Color vision: mice see hue too. Curr Biol 2007; 17: R457–460
- Condorelli D F, Parenti R, Spinella F, Trovato Salinaro A, Belluardo N, Cardile V, Cicirata F. Cloning of a new gap junction gene (Cx36) highly expressed in mammalian brain neurons. Eur J Neurosci 1998; 10: 1202–1208
- Cruciani V, Mikalsen S O. Evolutionary selection pressure and family relationships among connexin genes. Biol Chem 2007; 388: 253–264
- Curwen V, Eyras E, Andrews T D, Clarke L, Mongin E, Searle S M, Clamp M. The Ensembl automatic gene annotation system. Genome Res 2004; 14: 942–950
- Dahl G, Locovei S. Pannexin: to gap or not to gap, is that a question?. IUBMB Life 2006; 58: 409–419
- Demb J B, Pugh E N. Connexin36 forms synapses essential for night vision. Neuron 2002; 36: 551–553
- Dermietzel R, Kremer M, Paputsoglu G, Stang A, Skerrett I M, Gomes D, Srinivas M, Janssen-Bienhold U, Weiler R, Nicholson B J, Bruzzone R, Spray D C. Molecular and functional diversity of neural connexins in the retina. J Neurosci 2000; 20: 8331–8343
- Dvoriantchikova G, Ivanov D, Panchin Y, Shestopalov V I. Expression of pannexin family of proteins in the retina. FEBS Lett 2006; 580: 2178–2182
- Eastman S D, Chen T H, Falk M M, Mendelson T C, Iovine M K. Phylogenetic analysis of three complete gap junction gene families reveals lineage-specific duplications and highly supported gene classes. Genomics 2006; 87: 265–274
- Eiberger J, Degen J, Romualdi A, Deutsch U, Willecke K, Sohl G. Connexin genes in the mouse and human genome. Cell Commun Adhes 2001; 8: 163–165
- Filshie B K, Flower N E. Junctional structures in hydra. J Cell Sci 1977; 23: 151–172
- Fraser S E, Green C R, Bode H R, Gilula N B. Selective disruption of gap junctional communication interferes with a patterning process in hydra. Science 1987; 237: 49–55
- Goldsmith P, Harris W A. The zebrafish as a tool for understanding the biology of visual disorders. Semin Cell Dev Biol 2003; 14: 11–18
- Guldenagel M, Ammermuller J, Feigenspan A, Teubner B, Degen J, Sohl G, Willecke K, Weiler R. Visual transmission deficits in mice with targeted disruption of the gap junction gene connexin36. J Neurosci 2001; 21: 6036–6044
- Haverkamp S, Wassle H, Duebel J, Kuner T, Augustine G J, Feng G, Euler T. The primordial, blue-cone color system of the mouse retina. J Neurosci 2005; 25: 5438–5445
- Hertzberg E L, Skibbens R V. A protein homologous to the 27,000 dalton liver gap junction protein is present in a wide variety of species and tissues. Cell 1984; 39: 61–69
- Hombach S, Janssen-Bienhold U, Sohl G, Schubert T, Bussow H, Ott T, Weiler R, Willecke K. Functional expression of connexin57 in horizontal cells of the mouse retina. Eur J Neurosci 2004; 19: 2633–2640
- Huang Y J, Maruyama Y, Dvoryanchikov G, Pereira E, Chaudhari N, Roper S D. The role of pannexin 1 hemichannels in ATP release and cell-cell communication in mouse taste buds. Proc Natl Acad Sci U S A 2007; 104: 6436–6441
- Jacobs G H, Williams G A, Cahill H, Nathans J. Emergence of novel color vision in mice engineered to express a human cone photopigment. Science 2007; 315: 1723–1725
- Janssen-Bienhold U, Schultz K, Hoppenstedt W, Weiler R. Molecular diversity of gap junctions between horizontal cells. Prog Brain Res 2001a; 131: 93–107
- Janssen-Bienhold U, Schultz K, Gellhaus A, Schmidt P, Ammermuller J, Weiler R. Identification and localization of connexin26 within the photoreceptor-horizontal cell synaptic complex. Vis Neurosci 2001b; 18: 169–178
- Kamermans M, Fahrenfort I, Schultz K, Janssen-Bienhold U, Sjoerdsma T, Weiler R. Hemichannel-mediated inhibition in the outer retina. Science 2001; 292: 1178–1180
- Leithe E, Sirnes S, Omori Y, Rivedal E. Downregulation of gap junctions in cancer cells. Crit Rev Oncogene 2006; 12: 225–256
- Locovei S, Bao L, Dahl G. Pannexin 1 in erythrocytes: Function without a gap. Proc Natl Acad Sci U S A 2006a; 103: 7655–7659
- Locovei S, Wang J, Dahl G. Activation of pannexin 1 channels by ATP through P2Y receptors and by cytoplasmic calcium. FEBS Lett 2006b; 580: 239–244
- Maxeiner S, Dedek K, Janssen-Bienhold U, Ammermuller J, Brune H, Kirsch T, Pieper M, Degen J, Kruger O, Willecke K, Weiler R. Deletion of connexin45 in mouse retinal neurons disrupts the rod/cone signaling pathway between AII amacrine and ON cone bipolar cells and leads to impaired visual transmission. J Neurosci 2005; 25: 566–576
- McLachlan E, White T W, Ugonabo C, Olson C, Nagy J I, Valdimarsson G. Zebrafish Cx35: cloning and characterization of a gap junction gene highly expressed in the retina. J Neurosci Res 2003; 73: 753–764
- Meier C, Dermietzel R. Electrical synapses—gap junctions in the brain. Results Probl Cell Differ 2006; 43: 99–128
- O'Brien J, Bruzzone R, White T W, Al-Ubaidi M R, Ripps H. Cloning and expression of two related connexins from the perch retina define a distinct subgroup of the connexin family. J Neurosci 1998; 18: 7625–7637
- Pal J D, Liu X, Mackay D, Shiels A, Berthoud V M, Beyer E C, Ebihara L. Connexin46 mutations linked to congenital cataract show loss of gap junction channel function. Am J Physiol Cell Physiol 2000; 279: C596–C602
- Panchin Y V. Evolution of gap junction proteins—the pannexin alternative. J Exp Biol 2005; 208: 1415–1419
- Paul D L, Ebihara L, Takemoto L J, Swenson K I, Goodenough D A. Connexin46, a novel lens gap junction protein, induces voltage-gated currents in nonjunctional plasma membrane of Xenopus oocytes. J Cell Biol 1991; 115: 1077–1089
- Pelegrin P, Surprenant A. Pannexin-1 mediates large pore formation and interleukin-1beta release by the ATP-gated P2X7 receptor. EMBO J 2006; 25: 5071–5082
- Pfaffl M W, Horgan G W, Dempfle L. Relative expression software tool (REST) for group-wise comparison and statistical analysis of relative expression results in real-time PCR. Nucleic Acids Res 2002; 30: e36
- Plantard L, Huber M, Macari F, Meda P, Hohl D. Molecular interaction of connexin 30.3 and connexin 31 suggests a dominant-negative mechanism associated with erythrokeratodermia variabilis. Hum Mol Genet 2003; 12: 3287–3294
- Qu Y, Tang W, Dahlke I, Ding D, Salvi R, Sohl G, Willecke K, Chen P, Lin X. Analysis of connexin subunits required for the survival of vestibular hair cells. J Comp Neurol 2007; 504: 499–507
- Rash J E, Yasumura T, Davidson K G, Furman C S, Dudek F E, Nagy J I. Identification of cells expressing Cx43, Cx30, Cx26, Cx32 and Cx36 in gap junctions of rat brain and spinal cord. Cell Commun Adhes 2001; 8: 315–320
- Ray A, Zoidl G, Weickert S, Wahle P, Dermietzel R. Site-specific and developmental expression of pannexin1 in the mouse nervous system. Eur J Neurosci 2005; 21: 3277–3290
- Robson J G, Maeda H, Saszik S M, Frishman L J. In vivo studies of signaling in rod pathways of the mouse using the electroretinogram. Vision Res 2004; 44: 3253–3268
- Shelley J, Dedek K, Schubert T, Feigenspan A, Schultz K, Hombach S, Willecke K, Weiler R. Horizontal cell receptive fields are reduced in connexin57-deficient mice. Eur J Neurosci 2006; 23: 3176–3186
- Shields C R, Klooster J, Claassen Y, Ul-Hussain M, Zoidl G, Dermietzel R, Kamermans M. Retinal horizontal cell-specific promoter activity and protein expression of zebrafish connexin 52.6 and connexin 55.5. J Comp Neurol 2007; 501: 765–779
- Striedinger K, Petrasch-Parwez E, Zoidl G, Napirei M, Meier C, Eysel U T, Dermietzel R. Loss of connexin36 increases retinal cell vulnerability to secondary cell loss. Eur J Neurosci 2005; 22: 605–616
- Taylor J S, Van de Peer Y, Braasch I, Meyer A. Comparative genomics provides evidence for an ancient genome duplication event in fish. Philos Trans R Soc Lond B Biol Sci 2001; 356: 1661–1679
- Taylor J S, Braasch I, Frickey T, Meyer A, Van de Peer Y. Genome duplication, a trait shared by 22000 species of ray-finned fish. Genome Res 2003; 13: 382–390
- Thompson J D, Higgins D G, Gibson T J. CLUSTAL W: improving the sensitivity of progressive multiple sequence alignment through sequence weighting, position-specific gap penalties and weight matrix choice. Nucleic Acids Res 1994; 22: 4673–4680
- Uchida S, Sakai S, Furuichi T, Hosoda H, Toyota K, Ishii T, Kitamoto A, Sekine M, Koike K, Masushige S, Murphy G, Silva A J, Kida S. Tight regulation of transgene expression by tetracycline-dependent activator and repressor in brain. Genes Brain Behav 2006; 5: 96–106
- Vinken M, Vanhaecke T, Papeleu P, Snykers S, Henkens T, Rogiers V. Connexins and their channels in cell growth and cell death. Cell Signal 2006; 18: 592–600
- Weiler R, Pottek M, He S, Vaney D I. Modulation of coupling between retinal horizontal cells by retinoic acid and endogenous dopamine. Brain Res Brain Res Rev 2000; 32: 121–129
- Zoidl G, Bruzzone R, Weickert S, Kremer M, Zoidl C, Mitropoulou G, Srinivas M, Spray D C, Dermietzel R. Molecular cloning and functional expression of zfCx52.6: A novel connexin with hemichannel-forming properties expressed in horizontal cells of the zebrafish retina. J Biol Chem 2004; 279: 2913–2921
- Zoidl G, Petrasch-Parwez E, Ray A, Meier C, Bunse S, Habbes H W, Dahl G, Dermietzel R. Localization of the pannexin1 protein at postsynaptic sites in the cerebral cortex and hippocampus. Neuroscience 2007; 146: 9–16