Abstract
Connexins and pannexins are vertebrate transmembrane proteins that form hexameric conduits termed hemichannels. Functional hemichannels allow the diffusional transport of ions and small molecules across the plasma membrane and serve as paracrine and autocrine communication pathways. During the last decade, interest in the hemichannel field increased substantially. Today, there is evidence for the existence of connexin hemichannels in vertebrate cells and bulk of information supports their function in diverse physiological and pathological responses. Controversy regarding the molecular identity of the hemichannel type mediating many responses arose recently with the identification of pannexin-based hemichannels. Here, the authors describe the most frequently used methods for studying hemichannels in living mammalian cells and focus on those with which they have more experience. Although the available in vitro evidence is substantial, further studies and possibly new experimental approaches are required to understand the role and properties of connexin and pannexin hemichannels in vivo.
INTRODUCTION: GENERAL FEATURES OF HEMICHANNELS
Animal cells interact with each other in complex networks formed by independent communication pathways including direct (intercellular) cell-cell contacts and paracrine/autocrine (extracellular) signaling systems (Evans et al. Citation2006). Two different protein families, connexins and pannexins, present in vertebrate cells are capable of forming gap junction channels in exogenous expression systems (Sáez et al. Citation2003a; Barbe et al. Citation2006; Litvin et al. 2006; Vanden Abeele et al. Citation2006; Lai et al. Citation2007). Connexins and pannexins have no sequence homology, but they share a common transmembrane topology. They oligomerize around a central pore to form hexameric plasma membrane conduits termed “hemichannels” (for hemi gap junction channels) (Evans et al. Citation2006; Barbe et al. Citation2006; Shestopalov and Panchin Citation2007). Searches of human gene libraries have identified 21 connexins ranging from Cx23 to Cx62 (Söhl and Willecke Citation2004). Pannexins comprise only three subtypes termed Px1 to Px3 (Litvin et al. 2006). Cx43 and Px1 are the most ubiquitous members of each family and they are often endogenously coexpressed in many cell types (Laird et al. Citation2006; Barbe et al. Citation2006; Shestopalov and Panchin Citation2007; Boassa et al. Citation2007).
In support of the notion that hemichannel openings are incompatible with cell life, it was demonstrated that hemichannels formed by most connexins display only limited spontaneous activity under resting culture conditions (Trexler et al. Citation1996; Gómez-Hernández et al. Citation2003; Contreras et al. Citation2003; Retamal et al. Citation2006, Citation2007a, Citation2007b; Bukauskas et al. Citation2006). However, human Cx26 hemichannels open at resting transmembrane potentials and in the presence of physiological extracellular [Ca2 +] in transfected oocytes and Neuro2A cells without compromising the cell viability (González et al. Citation2006).
During the last decade, interest in the hemichannel field increased substantially. Noteworthy, approximately 24% (44 out of 187) of the communications presented in the last International Gap Junction Conference held in Elsinore, Denmark in August of year 2007, included topics related to hemichannels (International Gap Junction Conference, Abstract Book, http://www.gapjunction2007.dk).
Increased activity of connexin hemichannels occurs in cells after stimulation with endogenous ligands such as sphingosine1-phosphate (Squecco et al. Citation2006), nerve growth factor (Belliveau et al. Citation2006) and fibroblast growth factors 1 and 2 (K. A. Schalper, N. Palacios-Prado, M. A. Retamal, K. F. Shoji, A. D. Martínez, and J. C. Sáez, unpublished data; De Vuyst et al. 2007). High Cx43 hemichannel activity occurs also under several pathological conditions, including metabolic inhibition of different cell types (John et al. Citation1999; Contreras et al. Citation2002; Vergara et al. Citation2003), following stimulation with inflammatory mediators (Retamal et al. Citation2007a) and as a result of connexin mutations (Dobrowolski et al. Citation2007). Less is known about modulation of pannexin hemichannels. Those formed by Px1 are activated by membrane depolarization, mechanical stimulation, free cytosolic [Ca2 +] increases, and ATP-mediated purinergic receptor transactivation (Bao et al. Citation2004; Locovei et al. Citation2006a, Citation2006b, Citation2007; Pelegrin and Surprenant Citation2006).
The independent role of Cx43 hemichannels from gap junction channels was recently demonstrated in vivo. A Cx43 mutant capable of forming hemichannels but not intercellular channels was retrovirally expressed in Cx43-deficient ovarian granulosa cells. After implants in host female mice kidney capsules, cells expressing wild-type Cx43, but not the mutant, were able to rescue folliculogenesis, indicating that intercellular channels and hemichannels play different functions (Tong et al. Citation2007). However, evidence of functional modulation and biological significance of hemichannels in tissues, where cells are tightly packed and have less unopposed plasma membrane than in culture conditions, is still limited. Therefore, phenomena attributed to hemichannels might be influenced by the experimental conditions.
Although the existence of hemichannels and their functions in cultured cells is becoming accepted, some controversy regarding the molecular identity of the hemichannels mediating many responses arose with the identification of pannexin-based hemichannels (Spray et al. Citation2006; Wang et al. Citation2007; Harris et al. Citation2007). This issue is even more conflicting, because many studies revealing the presence and function of connexin hemichannels were conducted using non-physiological experimental protocols that include the application of positive transmembrane potentials and exposure to extracellular solutions lacking divalent cations. In addition, the participation of one hemichannel type in a cellular response does not rule out the involvement of other hemichannel types. Evidence supporting the existence of connexin hemichannels includes (i) a close association between membrane diffusional transport of a small molecule and the expression of a specific connexin type; (ii) the presence of a transmembrane pathway with permeability, selectivity and pharmacological sensitivity of a connexin hemichannel; and (iii) the presence of the predicted unitary conductance and expected voltage dependence of connexin hemichannels. Similar criteria could be useful to identify and demonstrate the relevance of pannexin hemichannels. Evidence in favor of pannexin hemichannels as responsible for transmembrane transport in diverse systems is still accumulating and includes the association between membrane diffusional transport and the expression of Px1 protein (Locovei et al. Citation2006b; Pelegrin and Surprenant Citation2006). Further studies are required to understand the role of pannexin hemichannels and their functional differences with connexin hemichannels. Here we briefly comment on the methods most frequently used to study hemichannels in living mammalian cells and focus on those with which we have more experience.
METHODS FOR STUDYING HEMICHANNELS
Different methods have been useful in evaluating the functional state of hemichannels. Some of them are aimed at identifying and characterizing the hemichannel protein subunits and include biotinylation of surface proteins with associated immunoblot analysis, measurements of the relative protein content of plasma membrane fractions resistant to detergents, fluorescence visualization (e.g., immunofluorescence, fluorescent peptides that bind extracellular domains of hemichannels, or connexins tagged with a fluorescent protein), electron microscopy, and atomic force microscopy imaging. Other methods are based on detection and characterization of hemichannel functions and include the uptake and release of small molecules (i.e., fluorescent dyes and metabolically relevant compounds), whole-cell patch clamp, and transport-specific fractionation using sucrose or other permeable solutes.
Experimental models frequently used to study hemichannels express a known pattern of connexin/pannexin types or involve a connexin/ pannexin-deficient cell type transfected with the protein of interest. Genetic approaches using antisense oligodeoxynucleotides and other gene silencing strategies have also been successfully used (Li et al. Citation1996; Franco et al. Citation2001; Jiang and Cherian Citation2003; Cherian et al. Citation2005; Pelegrin and Surprenant Citation2006; Genetos et al. Citation2007).
So far, there is not a single method to unequivocally demonstrate the presence, properties or molecular identity of hemichannels. Therefore, a combination of several techniques is advisable for achieving a solid interpretation. Many of the aforementioned experimental paradigms are often complemented with the use of hemichannels blockers such as lanthanides (La3 + or Ga3 +), long-chain alcohols (octanol and heptanol), licorice-derived gap junction blockers (18-α-glycerrithinic acid, 18-β-glycerrithinic acid, and carbenoxolone), chloride channel blockers with hemichannel blocking actions (flufenamic and niflumic acid), and connexin/pannexin mimetic peptides (Gap26, Gap27, 10pxn1). A proper use of these pharmacological tools might be helpful in discriminating between connexin and pannexin hemichannels. A comprehensive description of stimuli and drugs affecting the functional state of connexin and pannexin hemichannels was recently published (Retamal et al. Citation2007c).
Biotinylation of Surface Proteins
This technique is based on the property of biotin to strongly and specifically bind its partner avidin, first used in the detection of surface connexins by Musil and Goodenough (Citation1991). This study demonstrated the presence of a free (extrajunctional) Cx43 pool soluble to detergents in communication competent cells (Musil and Goodenough Citation1991). Sulfo-NHS-SS-Biotin is a thiol-cleavable amine-reactive reagent with a molecular mass of 606.69 (Product Data Sheet, Pierce Biotechnology, IL). It allows labeling of any other primary amine-containing biomolecule in solution, including lysine residues of proteins (). It is particularly useful for labeling surface membrane proteins because it is membrane impermeant and therefore does not label intracellular proteins in healthy cells.
Figure 1 Diagram illustrating the surface protein biotinylation procedure for detection of hemichannels in living cells. Cultured cells are incubated at 4°C with the Sulfo-NHS-SS-Biotin (B), which binds connexins/pannexins forming hemichannels (HC) with respect to those forming intercellular channels at the junctional plaques (IC). After biotin removal, cells are washed with a glycine-containing solution and homogenized in the presence of proteases and phosphatases inhibitors. Total protein contents are measured and corrections are made to ensure that each sample has the same amount of proteins. Then, the same amount of avidin-agarose is added to each sample to pull down the biotin/surface protein complexes. After pull down, preparations are washed and eluted with an acid buffer, to release the surface proteins from the biotin/avidin complexes (BAC). Isolated surface proteins are blotted and probed with antibodies against the connexin/pannexin of interest.
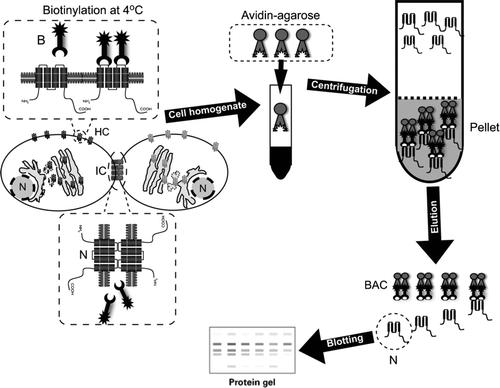
Cultured cells of interest are incubated with the Sulfo-NHS-SS-Biotin reagent for 30 to 60 min at 4°C to avoid biotin internalization through the endocytic pathway. To reduce or avoid degradation and/or aggregation of proteins, all of the following steps should be conducted at 4°C. A glycine-based solution is applied to quench unreacted biotin and cells are lysed and exposed to detergents (e.g., Triton X-100 and NP-40) to ensure complete removal of connexins from cell membrane fractions. Later on, samples are incubated with a solution containing a modified avidin derivative that specifically binds the biotin-surface proteins complexes. After pull-down, samples are eluted to separate the biotin attached to cell surface proteins. Finally, the protein content of each sample is measured and aliquots with the same amount of proteins are prepared and resolved in sodium dodecyl sulfate–polyacrylamide gel electrophoresis (SDS-PAGE). To confirm if each loaded sample contains the same amount of protein, membranes can be probed with general protein stains such as Ponceau's red prior to immunoblotting. The isolated proteins can be analyzed by Western blot using specific antibodies, allowing the detection of the relative amount of the target protein (). Recent studies by our group and others have used this approach to demonstrate changes in the relative amount of surface connexins forming hemichannels under different conditions (Cooper and Lampe Citation2002; Cherian et al. Citation2005; Lai et al. Citation2006; Retamal et al. Citation2006, Citation2007a, Citation2007b). A critical feature of the labeling is that the biotinylating reagent seems to have much less access to connexins found within the gap junction plaque with respect to the extrajunctional surface pool, so it provides a selective measure of nonjunctional connexins (Musil and Goodenough Citation1991). Indeed, only ∼ 15% of total surface biotinylated Cx43-eYFP is Triton X-100 resistant (Lai et al. Citation2006). Plaque-associated connexins are believed to be a poor substrate for the biotinylation reagent because of the restricted diffusion capacity of the molecule in the 2-nm intercellular space at the junction or to possible changes in the structure of connexins rendering the proteins reactive lysine residues inaccessible after plaque formation (Musil and Goodenough Citation1991).
The biotinylation method also allows an estimation of the relative levels of surface Cx43 forming hemichannels as compared to total Cx43. In cortical astrocytes, this value turns out to be ∼ 15% (Retamal et al. Citation2006). Similarly, in HeLa cells stably transfected with Cx43, the surface hemichannels account for ∼ 11% of total Cx43 under resting conditions and no obvious differences in the distribution of phosphoforms are evident ().
Figure 2 Surface Cx43 corresponds to a small amount of total Cx43 in HeLa transfectants. (Upper panel) Representative immunoblots showing the relative levels of total (left) or biotinylated Cx43 (right) in HeLa cells stably expressing wild type Cx43 (HeLa-Cx43). (Lower panel) Normalized densitometric values; the band intensity detected in the biotinylated surface protein fraction relative to the total protein levels of connexin taken as 100%. Cells were studied under control conditions and in the presence of physiological concentrations of divalent cations (1.8 mM Ca2 + and 1 mM Mg2 +). Determination of surface Cx43 with respect to total protein was performed by blotting different amounts of total (1, 10, 20, 40, 80, and 160 μ g) and a sample of biotinylated proteins in the same gel. Densities were measured, a fitting curve was constructed and the protein amount of the biotinylated sample was determined by interpolation. Each bar represents average ± SEM of three independent experiments; **p < 0.01. NP and P2-P3 denote the unphosphorylated and phosphorylated forms of Cx43, respectively. Statistic analysis was performed using descriptive statistics and Microscoft Excel software.
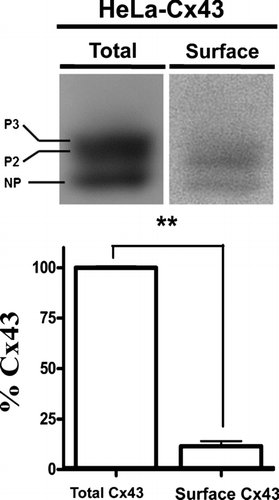
Sulfo-NHS-SS-Biotin has a linear shape and a molecular weight of 606.69 (), which is small enough to pass through open hemichannels or to block the hemichannel pore by forming complexes with the extracellular lysines lining the hemichannel pore. Data from our laboratory in which the biotin incubation was performed in the absence of divalent cations to open hemichannels () or in the presence of La3 + to close hemichannels () revealed that neither condition affects the amount of pulled down Cx43. Therefore, Sulfo-NHS-SS-Biotin does not enter through hemichannels opened by different stimuli as shown in and . Moreover, acute addition of the biotin reagent does not affect the ethidium bromide (Etd) uptake rate of HeLa cells expressing Cx43-eGFP (). Additional controls could be the blotting of biotinylated samples with antibodies against intracellular proteins known to be absent at the cell surface. In support of the interpretation that Sulfo-NHS-SS-Biotin does not label intracellular proteins; Cherian (2006) and Lai (Citation2006) showed an absence of actin labeling. In addition, studies of surface protein biotinylation and dye uptake for measuring hemichannels activity in cells submitted to metabolic inhibition or fibroblast growth factor (FGF)-1 treatment present a close correlation between the two parameters, indicating that biotinylation is possibly an appropriate method for determining the hemichannel content of cells under these conditions (Retamal et al. Citation2006; K. A. Schalper, N. Palacios-Prado, M. A. Retamal, K. F. Shoji, A. D. Martínez, and J. C. Sáez, unpublished data).
Figure 3 Sulfo-NHS-SS-Biotin neither enters cells through open hemichannels nor blocks the ethidium uptake. (A) Structure of Sulfo-NHS-SS-Biotin. (B) Surface Cx43 levels of mouse cortical astrocytes incubated with Sulfo-NHS-SS-Biotin in the presence of 1.8 mM Ca2 + and 1 mM Mg2 + (control) or in the absence of extracellular divalent cations (Ca2 +/Mg2 +-free). (C) Surface Cx43 levels of HeLa-Cx43 in control conditions (control), after treatment with 2.5 μ M 4-Br-A23187 for 50 min or after treatment with 2.5 μ M 4-Br-A23187 for 50 min and incubated with Sulfo-NHS-SS-Biotin in the presence of 200 μ M La3 + to block connexin hemichannels. NP and P2-P3 denote the unphosphorylated and phosphorylated forms of Cx43, respectively. (D) The Etd (5 μ M) uptake rate of HeLa-Cx43eGFP cells before and after addition of 500 μ g/ml Sulfo-NHS-SS-Biotin. Cells were recorded at room temperature in the presence of 1.8 mM Ca2 + and 1 mM Mg2 +, pH = 7.4. Each bar represents the mean ± SEM. Each experiment included time-lapse measurements of 20 cells and the number of independent experiments is indicated within each bar; ns = not significant (p > 0.05, U test).
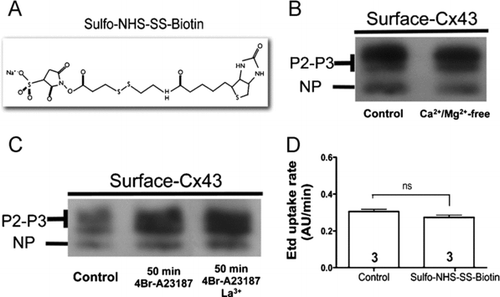
Dye Uptake Assays
In all functional studies, it is important to rule out the presence of hemichannel inhibitors in the water used to prepare saline solutions as well as specific reagents such as agonist applied to increase the activity of hemichannels. In our experience, distilled water deionized using milliQ system is not sufficient. In fact, using this water we did not see hemichannel activation in cortical astrocytes exposed to zero extracellular Ca2 + and Mg2 + (Contreras et al. Citation2002). Nonetheless, we detected a clear dye uptake increase induced by a Ca2 +/Mg2 +-free solution in different cell types, including astrocytes, when preparing all solutions and reagents with sterile-filtered, cell culture tested water obtained from Sigma-Aldrich (catalog no. W3500) ().
Figure 4 Removal of extracellular divalent cations increases the ethidium uptake in HeLa cells expressing Cx43 and the response is sensitive to a connexin hemichannel blocker. (A) Representative fluorescence photomicrography of the Etd (5 μ M) uptake in parental (HeLa-parental) or HeLa-Cx43 cells after 10 min exposure to a Ca2 +/Mg2 +-free solution (Ca2 +/Mg2 +-free). The Ca2 +/Mg2+-free solution was prepared in sterile-filtered Sigma water with no added divalent cations plus 5 mM EGTA, pH = 7.4. Bar = 20 μ m. (B) Representative time-lapse recording of the Etd (5 μ M) uptake in HeLa-parental or HeLa-Cx43 cells in control condition, bathed for 10 min with a solution lacking divalent cations (Ca2 +/Mg2 +-free) and after addition of 200 μ M La3 +. Cells were recorded at room temperature and pH = 7.4. Each point in the graph represents mean ± SEM of 20 cells.
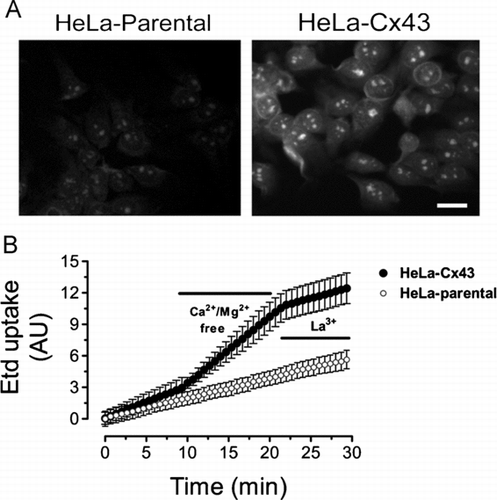
Analogous to the dye transfer experiments used to study gap junction channels, the transmembrane passage of small fluorescent permeability tracers has been widely used to study the functional state of hemichannels. This approach was first described using Xenopus oocytes injected with the RNA of Cx46 (Paul et al. 1991) and cultured solitary horizontal cells of the catfish retina (DeVries and Schwartz Citation1992). Later on, pioneer studies by Ross Johnson and collaborators validated this method in mammalian cells (Li et al. Citation1996). Basically, it consists of exposing cells to extracellular fluorescent permeability tracers with specific shape, mass and net charge such as the anionic dyes Lucifer yellow (LY; net charge = −1) and 5,6-carboxyfluorescein (CF; net charge = −1) and cationic probes ethidium bromide (Etd; net charge = +1), propidium iodide (PI; net charge = +2), and DAPI (net charge = +2). Then, the extracellular dye is washed-out at different time periods or after the application of stimuli to open hemichannels and the fluorescence intensity retained by each cell is quantified in snap shot images (). To prevent the nonspecific release of intracellular dye, a hemichannel blocker can be included in the washing solution or cells can be quickly fixed using an aldehyde. Membrane-impermeant cationic probes such as Etd and PI that become fluorescent when intercalated in nucleic acids strands allow real-time measurements and quantification of uptake rates using time-lapse imaging (). Either in a parallel experiment or at the end of the dye uptake assay, blockade with a hemichannel inhibitor helps to identify the membrane permeable pathway (). The use of an extracellular dye with molecular mass above the exclusion limit of hemichannels has been useful to rule out membrane damage as a possible nonspecific pathway of dye uptake (Li et al. Citation1996; Kondo et al. Citation2000; Contreras et al. Citation2002; De Vuyst et al. Citation2006). In addition, molecular shape could significantly influence the relative permeability measurement (Goldberg et al. Citation2004). In this regard, DAPI (molecular weight [MW] = 279.3) with a lower molecular mass and only one additional positive charge, than Etd (MW = 314.4), is less permeant to hemichannels formed by Cxs 26, 32, or 43 as compared to Cx45 hemichannels (J. A. Orellana, K. A. Schalper, and J. C. Sáez, unpublished data).
Release of ATP and Other Small Signaling Molecules
The opening of hemichannels formed by Cxs 26, 32, and 43 is reported to increase plasma membrane permeability to small bioactive molecules such as ATP, NAD+, ascorbic acid, glutamate, prostaglandin E2, glutathione, and the fluorescent 2-deoxyglucose (Cotrina et al. Citation1998; Franco et al. Citation2001; Ahmad and Evans Citation2002; Stout et al. Citation2002; Ye et al. Citation2003; Cherian et al. Citation2005; Pearson et al. Citation2005; Rana et al. 2006; De Vuyst et al. Citation2006; De Vuyst et al. Citation2007; Retamal et al. Citation2007a). Open Px1 hemichannels allow the passage of ATP and Ca2 + (Bao et al. Citation2004; Vanden Abeele et al. Citation2006), and likely many other unidentified small molecules also permeate them.
Under the same principle used for dye uptake experiments, the availability of high-sensitivity chemiluminiscence-based tests has permitted the study of biologically relevant molecules passing through hemichannels. A molecule virtually absent or present at very low concentration in the extracellular milieu under control conditions (e.g., ATP) is selected. After the application of a stimulus to open hemichannels, the molecule is either detected or its concentration increased in the bathing solution. As with dye uptake assays, pharmacological blockade is recommended. The use of La3 + to inhibit ATP release through hemichannels is discouraged because they precipitate, leading to results that are difficult to interpret. Alternatively, the use of connexin-expressing cells and equivalent connexin-deficient cells has been useful in identifying the membrane permeable pathway. One important drawback of this approach is that the relative permeability to such molecules is assumed to be similar or even identical among different hemichannels or cell types and permeability remains unaltered by different stimuli applied to activate hemichannels.
Electrophysiological Characterization of Hemichannels
Electrophysiological measurements, mainly using voltage clamp in the whole cell configuration, have been used for characterizing hemichannels. Both at the macroscopic and microscopic level, the current (I) versus voltage (V) curve crosses at zero, revealing the existence of a membrane pathway with permeability selectivity that is absent or limited, as expected for several connexin hemichannels. The hemichannel currents are measured in the presence of Ba2 + and Cs+ 2 to block most background inward and outward currents (e.g., inward rectifier and voltage-activated K+ channels). In addition, the low extracellular [Ca2 +]-induced currents are inhibited with connexin hemichannel blockers (Bennett et al. Citation2003; Retamal et al. Citation2007c), supporting the molecular identity of the hemichannels.
Functional hemichannels have been studied using electrophysiological techniques in exogenous expression systems such as Xenopus oocytes and transfected mammalian cells (Sáez et al. Citation2003b). Because a gap junction channel behaves as two series resistances, each one being a hemichannel composed of the same connexin type(s) and stoichiometry, the hemichannel unitary conductance was predicted to be twice that of a gap junction channel (Bennett et al. Citation2003). Using dual whole-cell voltage clamp for gap junction channels and single whole-cell voltage clamp for hemichannels, the recorded values confirmed this notion (Bennett et al. Citation2003). It should be kept in mind that the whole-cell patch clamp configuration might dialyze intracellular factors and metabolites that may influence the hemichannel activity. It remains unknown whether the unitary conductance values studied with whole-cell voltage clamp remain the same if measured with the cell-attached configuration.
Whole-cell voltage clamp studies of unitary events have also provided information on connexin hemichannel permeability selectivity, opening and closing kinetics, presence of subconductance states, and open probability (latency), among other properties (Valiunas and Weingart Citation2000; Kondo et al. Citation2000; Valiunas Citation2002, Bennett et al. Citation2003; Gómez-Hernandez et al. 2003; Contreras et al. Citation2003; Retamal et al. Citation2007b). In addition, these analyses are useful in distinguishing between connexin and pannexin hemichannels. For example, the single channel conductance of homomeric Cx43 and Px1 hemichannels measured under similar intra-and extracellular ionic environments are strikingly different being of ∼ 220 pS () and 475-550 pS, respectively (Contreras et al. Citation2003; Bao et al. Citation2004). The combined use of electrophysiology and dye uptake could be a promising approach to clearly distinguish connexin and pannexin hemichannels.
Figure 5 Records of unitary hemichannel currents in HeLa-Cx43 cells. Whole-cell voltage clamp recording in HeLa-Cx43 cells in the presence of extracellular divalent cations. The ionic composition of the extracellular and pipette solution was as described by Contreras et al. 2003. (A) A step from 0 to +80 mV elicited discrete current events in control cells. (B) Conductance values of record shown in A. The conductance value between the close state (c) and substate (s) or fully open state (o) correspond to ∼ 75 pS and ∼ 220 pS, respectively. (C) Frequency (counts) distribution of conductance values shown in B.
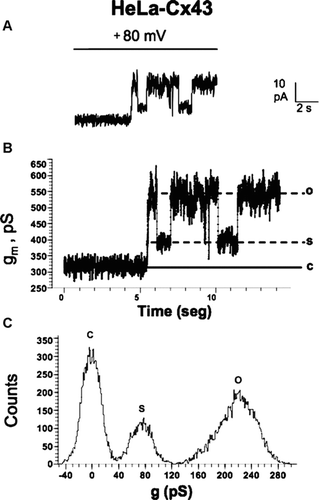
In most electrophysiological studies, opening of connexin hemichannels was observed at positive membrane potentials. Cx43 hemichannels expressed in HeLa cells open very infrequently at negative membrane potentials (Contreras et al. Citation2003). Nevertheless, Cx32 hemichannels expressed in HeLa cells open at negative membrane potential almost as frequent as they do at positive potentials (H. A. Sánchez, V. Verselis, and J. C. Sáez, unpublished data presented as poster in the International Gap Junction Conference held in Elsinore, Denmark, August 2007). Moreover, opening of Cx43 hemichannels at negative membrane potential was recently reported in astrocytes treated with proinflammatory agents (Retamal et al. Citation2007a). Under the latter condition, the increased hemichannels current was directly related to an increase in Etd uptake. It remains unknown why hemichannels constituted of certain connexins, such as mouse Cx32 or human Cx26, open at negative membrane potentials, whereas hemichannels formed by other connexin types, such as Cx43, stay preferentially closed in non-stimulated cells. Future studies shall elucidate whether these differences are explained by intrinsic connexin properties, changes in cell condition that change the covalent modification of connexins subunits or their interaction with intracellular regulatory molecules. An important aspect to be considered is the recording conditions. For instance, recording of Cx43 hemichannles at negative membrane potential in astrocytes treated with proinflamamtory cytokines required a pipette solution containing CsCl instead of KCl (Retamal et al. Citation2007a). Whether this protocol helps for recording unitary currents of hemichannels composed of other connexin types, in other cell types and under different conditions remains to be determined.
Pharmacology of Hemichannels
The pharmacological approach has been widely used in the study of hemichannels and constitutes a valuable tool to discriminate between connexin and pannexin hemichannels (Bruzzone et al. Citation2005; Pelegrin and Surprenant Citation2006; Retamal et al. Citation2006, Citation2007c). Diverse connexin hemichannel blockers have been shown to inhibit P2X7 purinergic receptors and could therefore negatively affect Px1 hemichannels function (Suadicani et al. Citation2006).
Hemichannels formed by most studied connexins, prominently increase their activity upon reduction or removal of extracellular divalent cations (Evans et al. Citation2006; Retamal et al. Citation2007c). Conversely, Px1 hemichannels are insensitive to variations in the extracellular Ca2 + concentration (Bruzzone et al. Citation2005; Pelegrin and Surprenant Citation2006). Px1 hemichannels are relatively insensitive to La3 +, heptanol, and flufenamic acid, whereas connexin hemichannels are effectively blocked by these agents (Bruzzone et al. Citation2005; Pelegrin and Suprenant Citation2006). The use of La3 + requires the absence of extracellular anions such as phosphates or sulphates, because they precipitate, leading to irreproducible hemichannel blockade. The use of heptanol or octanol as hemichannel blockers requires their preparation as stock solutions in organic solvents such as ethanol or DMSO (5% v/v) followed by dilutions to the final concentration in a physiological solution. If these long-chain alcohols are prepared at their final micromolar or millimolar concentration directly in a recording buffer, they come out of solution forming a toxic micellar mix.
Short peptides with specific sequences equivalent to extracellular connexin domains, termed connexin mimetic peptides (e.g., Gap26, Gap27), have been widely used to demonstrate connexin hemichannel involvement in diverse cell responses (Evans et al. Citation2006). They are believed to selectively bind to and block surface hemichannels within the first hour of application and gap junction channels later, with a time window of a few hours (Evans et al. Citation2006). A Px1 mimetic peptide with apparent specific blocking effect was also recently reported (110pxn1) (Pelegrin and Surprenant Citation2006). A large number of publications demonstrate specific effects of some of these peptides on connexin hemichannels by using in parallel scrambled peptide versions, additional hemichannel blockers and/or studies of their effects on connexin-specific expression systems (Braet et al. Citation2003a, Citation2003b; Pearson et al. Citation2005; Gomes et al. Citation2005; Eltzschig et al. Citation2006; Takeuchi et al. Citation2006; De Vuyst et al. Citation2006; De Vuyst et al. Citation2007; Retamal et al. Citation2006, Citation2007b; Shintani-Ishida et al. Citation2007). However, it was recently reported that some Cx32, Cx43, and Px1 mimetic peptides cause non-specific blockade of hemichannels formed by Px1, Cx46, and a connexin Cx32 chimera carrying the first extracellular loop of Cx43 (Cx32E143) in the oocyte expression system (Wang et al. Citation2007). Because a similar blocking effect was observed with addition of polyethyleneglycol, the authors proposed that mimetic peptides might exert their actions through a steric block. Nevertheless, polyethyleneglycol might be affecting hemichannels through a mechanism unrelated to that of mimetic peptides. Further studies are required to conclusively prove the value of mimetic peptides as pharmacological tools in the study of hemichannels in mammalian cells.
The use of antibodies directed against extracellular connexin domains to specifically detect and block hemichannels has also been reported (Hofer and Dermietzel Citation1998). This is probably a promising and specific tool for detecting hemichannels in animal tissues and selective inhibition of hemichannels.
CONCLUSION AND PERSPECTIVES
Although the existence of hemichannels and their functions in living cells are becoming more accepted, there is still controversy regarding the molecular identity of the hemichannel type mediating many cellular responses. Because connexins and pannexins are coexpressed in many cell types, the existence of one hemichannel type does not exclude the involvement of the other hemichannel type in a particular cell function. Therefore, these autocrine/paracrine communication pathways might be redundant or complementary.
Combining techniques such as biotinylation of surface proteins, dye uptake assays, and electrophysiological measurements in cellular models with known connexin/pannexin expression patterns is a reasonable strategy to study hemichannels in cultured cells. Most methods currently used in the study of hemichannels require cautious implementation and careful interpretation. The use of hemichannel blockers seems like a valuable tool to discriminate between connexin and pannexin hemichannels. Further studies are required to assess the value of mimetic peptides as pharmacological tools in the study of hemichannels in mammalian cells.
New experimental approaches such as use of simultaneous dye uptake and electrophysiological measurements, hemichannel biosensors, and specific antibodies to detect and block hemichannels in vitro and in vivo are required to understand the role and specific properties of connexin and pannexin hemichannels.
REFERENCES
- Ahmad S, Evans W H. Post-translational integration and oligomerization of connexin 26 in plasma membranes and evidence of formation of membrane pores: Implications for the assembly of gap junctions. Biochem J 2002; 365: 693–699
- Bao L, Locovei S., Dahl G. Pannexin membrane channels are mechanosensitive conduits for ATP. FEBS Lett 2004; 572: 65–68
- Barbe M T, Monyer H, Bruzzone R. Cell-cell communication beyond connexins: The pannexin channels. Physiology 2006; 21: 103–114
- Belliveau D J, Bani-Yaghoub M, McGirr B, Naus C C, Rushlow W J. Enhanced neurite outgrowth in PC12 cells mediated by connexin hemichannels and ATP. J Biol Chem 2006; 281: 20920–20931
- Bennett M V, Contreras J E, Bukauskas F F, Sáez J C. New roles for astrocytes: Gap junction hemichannels have something to communicate. Trends Neurosci 2003; 26: 610–617
- Boassa D, Ambrosi C, Qiu F, Dahl G, Gaietta G, Sosinsky G. Pannexin1 channels contain a glycosylation site that targets the hexamer to the plasma membrane. J Biol Chem 2007; 282: 31733–31743
- Braet K, Aspeslagh S, Vandamme W, Willecke K, Martin P E, Evans W H, Leybaert L. Pharmacological sensitivity of ATP release triggered by photoliberation of inositol-1,4,5-trisphosphate and zero extracellular calcium in brain endothelial cells. J Cell Physiol 2003; 197: 205–213
- Braet K, Vandamme W, Martin P E, Evans W H, Leybaert L. Photoliberating inositol-1,4,5-trisphosphate triggers ATP release that is blocked by the connexin mimetic peptide gap 26. Cell Calcium 2003; 33: 37–48
- Bruzzone R, Barbe M T, Jakob N J, Monyer H. Pharmacological properties of homomeric and heteromeric pannexin hemichannels expressed in Xenopus oocytes. J Neurochem 2005; 92: 1033–1043
- Bruzzone R, Hormuzdi S G, Barbe M T, Herb A, Monyer H. Pannexins, a family of gap junction proteins expressed in brain. Proc Natl Acad Sci U S A 2003; 100: 13644–13649
- Bukauskas F F, Kreuzberg M M, Rackauskas M, Bukauskiene A, Bennett M V, Verselis V K, Willecke K. Properties of mouse connexin 30.2 and human connexin 31.9 hemichannels: Implications for atrioventricular conduction in the heart. Proc Natl Acad Sci U S A 2006; 103: 9726–9731
- Cherian P P, Siller-Jackson A. J., Gu S, Wang X, Bonewald L F, Sprague E, Jiang J X. Mechanical strain opens connexin 43 hemichannels in osteocytes: A novel mechanism for the release of prostaglandin. Mol Biol Cell 2005; 16: 3100–3106
- Contreras J E, Sánchez H A, Eugenín E A, Speidel D, Theis M, Willecke K, Bukauskas F F, Bennett M V, Sáez J C. Metabolic inhibition induces opening of unapposed connexin 43 gap junction hemichannels and reduces gap junctional communication in cortical astrocytes in culture. Proc Natl Acad Sci U S A 2002; 99: 495–500
- Contreras J E, Sáez J C, Bukauskas F F, Bennett M V. Gating and regulation of connexin 43 (Cx43) hemichannels. Proc Natl Acad Sci U S A 2003; 100: 11388–11393
- Cooper C D, Lampe P D. Casein kinase 1 regulates connexin-43 gap junction assembly. J Biol Chem 2002; 277: 44962–44968
- Cotrina M L, Lin J H, Alves-Rodrigues A., Liu S, Li J, Azmi-Ghadimi H, Kang J, Naus C C, Nedergaard M. Connexins regulate calcium signaling by controlling ATP release. Proc Natl Acad Sci U S A 1998; 95: 15735–15740
- De Vuyst E, Decrock E, Cabooter L, Dubyak G R, Naus C C, Evans W H, Leybaert L. Intracellular calcium changes trigger connexin 32 hemichannel opening. EMBO J 2006; 25: 34–44
- De Vuyst E, Decrock E, De Bock M., Yamasaki H, Naus C C, Evans W H, Leybaert L. Connexin hemichannels and gap junction channels are differentially influenced by lipopolysaccharide and basic fibroblast growth factor. Mol Biol Cell 2007; 18: 34–46
- DeVries S H, Schwartz E A. Hemi-gap-junction channels in solitary horizontal cells of the catfish retina. J Physiol 1992; 445: 201–230
- Dobrowolski R, Sommershof A, Willecke K. Some oculodentodigitial dysplasia-associated Cx43 mutations cause increased hemichannel activity in addition to defecient gap junction channels. J Membr Biol 2007; 219: 9–17
- Eltzschig H K, Eckle T, Mager A, Kuper N, Karcher C, Weissmuller T, Boengler K, Schulz R, Robson S C, Colgan S P. ATP release from activated neutrophils occurs via connexin 43 and modulates adenosine-dependent endothelial cell function. Circ Res 2006; 99: 1100–1108
- Evans W H, De Vuyst E., Leybaert L. The gap junction cellular internet: Connexin hemichannels enter the signalling limelight. Biochem J 2006; 397: 1–14
- Franco L, Zocchi E, Usai C, Guida L, Bruzzone S, Costa A, De Flora A. Paracrine roles of NAD+ and cyclic ADP-ribose in increasing intracellular calcium and enhancing cell proliferation of 3T3 fibroblasts. J Biol Chem 2001; 276: 21642–21648
- Genetos D C, Kephart C J, Zhang Y, Yellowley C E, Donahue H J. Oscillating fluid flow activation of gap junction hemichannels induces ATP release from MLO-Y4 osteocytes. J Cell Physiol 2007; 212: 207–214
- Goldberg G S, Valiunas V, Brink P R. Selective permeability of gap junction channels. Biochim Biophys Acta 2004; 1662: 96–101
- Gomes P, Srinivas S P, Van Driessche W., Vereecke J, Himpens B. ATP release through connexin hemichannels in corneal endothelial cells. Invest Ophthalmol Vis Sci 2005; 46: 1208–1218
- Gómez-Hernández J M, de Miguel M., Larrosa B, González D, Barrio L C. Molecular basis of calcium regulation in connexin-32 hemichannels. Proc Natl Acad Sci U S A 2003; 100: 16030–16035
- Gonzalez D, Gómez-Hernandez J. M., Barrio L C. Species specificity of mammalian connexin-26 to form open voltage-gated hemichannels. FASEB J 2006; 20: 2329–2338
- Harris A L. Connexin channel permeability to cytoplasmic molecules. Prog Biophys Mol Biol 2007; 94: 120–143
- Hofer A, Dermietzel R. Visualization and functional blocking of gap junction hemichannels (connexons) with antibodies against external loop domains in astrocytes. Glia 1998; 24: 141–154
- Jiang J X, Cherian P P. Hemichannels formed by connexin 43 play an important role in the release of prostaglandin E(2) by osteocytes in response to mechanical strain. Cell Commun Adhes 2003; 10: 259–264
- John S A, Kondo R, Wang S Y, Goldhaber J I, Weiss J N. Connexin-43 hemichannels opened by metabolic inhibition. J Biol Chem 1999; 274: 236–240
- Kondo R P, Wang S Y, John S A, Weiss J N, Goldhaber J I. Metabolic inhibition activates a non-selective current through connexin hemichannels in isolated ventricular myocytes. J Mol Cell Cardiol 2000; 32: 1859–1872
- Lai A, Le D N, Paznekas W A, Gifford W D, Jabs E W, Charles A C. Oculodentodigital dysplasia connexin43 mutations result in non-functional connexin hemichannels and gap junctions in C6 glioma cells. J Cell Sci 2006; 119: 532–541
- Lai C P, Bechberger J F, Thompson R J, MacVicar B. A., Bruzzone R, Naus C C. Tumor-suppressive effects of pannexin 1 in C6 glioma cells. Cancer Res 2007; 67: 1545–5154
- Laird D W. Life cycle of connexins in health and disease. Biochem J 2006; 394: 527–543
- Li H, Liu T F, Lazrak A, Peracchia C, Goldberg G S, Lampe P D, Johnson R G. Properties and regulation of gap junctional hemichannels in the plasma membranes of cultured cells. J Cell Biol 1996; 134: 1019–1030
- Locovei S, Scemes E, Qiu F, Spray D C, Dahl G. Pannexin1 is part of the pore forming unit of the P2X(7) receptor death complex. FEBS Lett 2007; 581: 483–488
- Locovei S, Wang J, Dahl G. Activation of pannexin 1 channels by ATP through P2Y receptors and by cytoplasmic calcium. FEBS Lett 2006a; 580: 239–244
- Locovei S, Bao L, Dahl G. Pannexin 1 in erythrocytes: Function without a gap. Proc Natl Acad Sci U S A 2006b; 103: 7655–7659
- Musil L S, Goodenough D A. Biochemical analysis of connexin43 intracellular transport, phosphorylation, and assembly into gap junctional plaques. J Cell Biol 1991; 115: 1357–1374
- Cherian P P, Siller-Jackson A. J., Gu S, Wang X, Bonewald L F, Sprague E, Jiang J X. Mechanical strain opens connexin 43 hemichannels in osteocytes: A novel mechanism for the release of prostaglandin. Mol Biol Cell 2005; 16: 3100–3106
- Pearson R A, Dale N, Llaudet E, Mobbs P. ATP released via gap junction hemichannels from the pigment epithelium regulates neural retinal progenitor proliferation. Neuron 2005; 46: 731–744
- Pelegrin P, Surprenant A. Pannexin-1 mediates large pore formation and interleukin-1beta release by the ATP-gated P2X7 receptor. EMBO J 2006; 25: 5071–5082
- Rana S, Dringen R. Gap junction hemichannel-mediated release of glutathione from cultured rat astrocytes. Neurosci Lett 2007; 415: 45–48
- Retamal M A, Cortes C J, Reuss L, Bennett M V, Sáez J C. S-nitrosylation and permeation through connexin 43 hemichannels in astrocytes: Induction by oxidant stress and reversal by reducing agents. Proc Natl Acad Sci U S A 2006; 103: 4475–4480
- Retamal M A, Froger N, Palacios-Prado N, Ezan P, Sáez P J, Sáez J C, Giaume C. Cx43 hemichannels and gap junction channels in astrocytes are regulated oppositely by proinflammatory cytokines released from activated microglia. J Neurosci 2007a; 27: 13781–13792
- Retamal M A, Schalper K A, Shoji K F, Bennett M V, Sáez J C. Opening of connexin 43 hemichannels is increased by lowering intracellular redox potential. Proc Natl Acad Sci U S A 2007b; 104: 8322–8327
- Retamal M A, Schalper K A, Shoji K F, Orellana J A, Bennett M V, Sáez J C. Possible involvement of different connexin43 domains in plasma membrane permeabilization induced by ischemia-reperfusion. J Membr Biol 2007c; 218: 49–63
- Sáez J C, Berthoud V M, Brañes M C, Martínez A D, Beyer E C. Plasma membrane channels formed by connexins: Their regulation and functions. Physiol Rev 2003a; 83: 1359–1400
- Sáez J C, Contreras J E, Bukauskas F F, Retamal M A, Bennett M V. Gap junction hemichannels in astrocytes of the CNS. Acta Physiol Scand 2003b; 179: 9–22
- Shestopalov V I, Panchin Y. Pannexins and gap junction protein diversity. Cell Mol Life Sci 2007; 65: 376–394
- Shintani-Ishida K, Uemura K, Yoshida K. Hemichannels in cardiomyocytes open transiently during ischemia and contribute to reperfusion injury following brief ischemia. Am J Physiol Heart Circ Physiol 2007; 293: 1714–1720
- Sohl G, Willecke K. Gap junctions and the connexin protein family. Cardiovasc Res 2004; 62: 228–232
- Spray D C, Ye Z C, Ransom B R. Functional connexin “hemichannels”: A critical appraisal. Glia 2006; 54: 758–773
- Squecco R, Sassoli C, Nuti F, Martinesi M, Chellini F, Nosi D, Zecchi-Orlandini S, Francini F, Formigli L, Meacci E. Sphingosine 1-phosphate induces myoblast differentiation through Cx43 protein expression: A role for a gap junction-dependent and-independent function. Mol Biol Cell 2006; 17: 4896–4910
- Stout C E, Costantin J L, Naus C C, Charles A C. Intercellular calcium signaling in astrocytes via ATP release through connexin hemichannels. J Biol Chem 2002; 277: 10482–10488
- Suadicani S O, Brosnan C F, Scemes E. P2X7 receptors mediate ATP release and amplification of astrocytic intercellular Ca2 + signaling. J Neurosci 2006; 26: 1378–1385
- Takeuchi H, Jin S, Wang J, Zhang G, Kawanokuchi J, Kuno R, Sonobe Y, Mizuno T, Suzumura A. Tumor necrosis factor-alpha induces neurotoxicity via glutamate release from hemichannels of activated microglia in an autocrine manner. J Biol Chem 2006; 281: 21362–21368
- Tong D, Li T Y, Naus K E, Bai D, Kidder G M. In vivo analysis of undocked connexin43 gap junction hemichannels in ovarian granulosa cells. J Cell Sci 2007; 120: 4016–4024
- Trexler E B, Bennett M V, Bargiello T A, Verselis V K. Voltage gating and permeation in a gap junction hemichannel. Proc Natl Acad Sci U S A 1996; 93: 5836–5841
- Franco L, Zocchi E, Usai C, Guida L, Bruzzone S, Costa A, De Flora A. Paracrine roles of NAD+ and cyclic ADP-ribose in increasing intracellular calcium and enhancing cell proliferation of 3T3 fibroblasts. J Biol Chem 2001; 276: 21642–21648
- Valiunas V. Biophysical properties of connexin-45 gap junction hemichannels studied in vertebrate cells. J Gen Physiol 2002; 119: 147–164
- Valiunas V, Weingart R. Electrical properties of gap junction hemichannels identified in transfected HeLa cells. Pflugers Arch 2000; 440: 366–379
- Vanden Abeele F, Bidaux G, Gordienko D, Beck B, Panchin Y V, Baranova A V, Ivanov D V, Skryma R, Prevarskaya N. Functional implications of calcium permeability of the channel formed by pannexin 1. J Cell Biol 2006; 174: 535–546
- Vergara L, Bao X, Cooper M, Bello-Reuss E, Reuss L. Gap-junctional hemichannels are activated by ATP depletion in human renal proximal tubule cells. J Membr Biol 2003; 196: 173–184
- Wang J, Ma M, Locovei S, Keane R W, Dahl G. Modulation of membrane channel currents by gap junction protein mimetic peptides: Size matters. Am J Physiol Cell Physiol 2007; 293: 1112–1119
- Ye Z C, Wyeth M S, Baltan-Tekkok S., Ransom B R. Functional hemichannels in astrocytes: A novel mechanism of glutamate release. J Neurosci 2003; 23: 3588–3596