Abstract
Connexins and pannexins have been implicated in the formation of “hemichannels,” which may account for the uptake and release of membrane-impermeant molecules in single cells. The in vivo existence of “hemichannels” and their protein composition is still debated. Investigations on these matters are complicated by the lack of adequate negative controls. In search for such essential controls, the authors have investigated transformed (MIN6 line) and primary insulin-producing cells. Here, the authors report that these cells, which express Cx36 and pannexin1, cannot be shown to display functional “hemichannels,” as evaluated by (1) uptake of the membrane-impermeant tracer ethidium bromide, whether in the presence or absence of extracellular Ca2 +, following stimulation of P2X7 receptors, and after exposure to hypotonic medium; and (2) lack of exocytosis-independent release of endogenous ATP. Moreover, electrophysiological recordings indicated the absence of carbenoxolone-sensitive pannexin1 currents evoked by membrane potentials above +30 mV. Thus, insulin-producing cells are expected to provide a useful tool in the further characterization of hemichannel composition, properties, and physiological relevance.
INTRODUCTION
The cell membrane is poorly permeable to hydrophilic molecules, due to the presence of the two lipid leaflets. Accordingly, it may be rapidly permeated by small, current-carrying ions only in the presence of proteins that form ion-specific channels or transporters (Ashcroft Citation2000; Bröer and Wagner Citation2003). Typically, ion channels cannot be permeated by larger species, including most endogenous second messengers and metabolites of either the cytosol or the extracellular medium. However, increasing evidence indicates that some of these molecules, notably ATP and glutamate, may be rapidly released by living cells, including in the absence of exocytosis (Purpura et al. Citation2004; Burnstock Citation2007). Other experiments have indicated a possible, though slower, uptake by cells of even larger tracer molecules added to the extracellular medium, at least under certain experimental conditions (Harris Citation2001). These findings have led to the idea that the cell membrane may contain channels permeant to molecules larger than ions, which may functionally connect the cytosol to the immediate environment of cells, at least for short time periods (Stout et al. Citation2004; Saez et al. Citation2005; Spray et al. Citation2006).
Because the channels made by connexins (Cx) at gap junction domains of the cell membrane are permeated by both ions and larger molecules (Harris Citation2001), it was initially surmised that connexin proteins could also form single, unpaired connexons on the nonjunctional cell membrane, thereby providing the structural basis for the “hemichannels” that would account for the membrane permeation by hydrophilic molecules (Goodenough and Paul Citation2003; Stout et al. Citation2004; Saez et al. Citation2005; Spray et al. Citation2006). Consistent with this hypothesis, biochemical, immunolabeling, and expression studies revealed that connexons may be inserted in nonjunctional regions of the cell membrane (Musil et al. Citation1991; Paul et al. Citation1991; CitationDe Vries et al. 1992). Other studies showed that leakage of cytosolic molecules and uptake of extracellular, membrane-impermeant tracers may be abolished by drugs or conditions blocking gap junction channels (Liu et al. 1998; Li et al. Citation1996; Hofer and Dermietzel Citation1998; Kondo et al. Citation2000; Quist et al. Citation2000). Finally, electrophysiology of single cells identified currents with voltage sensitivity and unitary conductances approximatively as expected for half junctional channels, made of unpaired connexons (Harris Citation2001; Stout et al. Citation2004; Saez et al. Citation2005; Spray et al. Citation2006). Subsequently, however, the connexin hypothesis has been challenged by the evidence that proteins of the independent family of pannexins, discovered by Panchin et al. (Citation2000) and which do not form cell-to-cell channels, are prone to form the permeation pathway attributed to “hemichannels” (Bruzzone et al. Citation2003; Bao et al. Citation2004; Locovei et al. Citation2006b). Pannexin channels may be activated by the mechanical stretch of the membrane, and by the stimulation of purinergic receptors (Bao et al Citation2004; Locovei et al. Citation2006b; Pellegrin and Surprenant 2006; Locovei et al. Citation2007), two features that are not shared by connexin channels (Stout et al. Citation2004; Saez et al. Citation2005; Spray et al. Citation2006). Studies have further documented the presence of “hemichannels” in cells lacking connexin-dependent coupling, such as erythrocytes (Locovei et al. Citation2006a). Sorting these conflicting results is complicated by the usual coexpression of connexins and pannexins by a number of cell types, and by the lack of specific tools whereby to selectively block the channels made by either one of these proteins (Goodenough and Paul Citation2003; Bruzzone et al. Citation2005; Spray et al. Citation2006). Ultimately, because the models and conditions used to detect “hemichannels” are often non physiological, the question is still open whether functional “hemichannels” actually occur in vivo and, if so, what may be their physiological relevance (Goodenough and Paul Citation2003; Stout et al. Citation2004; Saez et al. Citation2005; Spray et al. Citation2006).
The availability of models thoroughly documented to lack “hemichannels” may help clarify these issues. In the search for such essential controls, we have investigated transformed mouse insulin-producing cells (MIN6 line) and primary β-cells of pancreatic islets. Here, we document that these cells do not form functional “hemichannels” as measured by the influx of a fluorescent tracer, as well as the absence of carbenoxolone-sensitive “hemichannel”-like currents induced by strong membrane depolarization, in spite of the native expression of Cx36, pannexin1 and the purinergic receptor P2X7. These cells are expected to provide a useful tool to investigate the “hemichannel” composition, properties, and their physiological relevance.
METHODS
Cells and Pancreatic Islets
Wild-type (WT) murine neuroblastoma N2A cells (ATCC, Manassas, VA, USA) were cultured in Dulbecco's modified Eagle's medium (DMEM) containing 25 mmol/L glucose and 10% fetal calf serum. WT mouse insulinoma MIN6 cells and a stable clone of Cx36-null MIN-6 cells (Calabrese et al. Citation2003) were cultured in DMEM medium containing 15% heat-inactivated fetal calf serum and 70 μ M β-mercaptoethanol. Cells were kept at 37°C in a humidified incubator, gassed with air and 6% CO2, fed at 3-day intervals, and passed once a week.
Control C57/Bl6 (Charles River Laboratories, Lyon, France) and Cx36-null mice back-crossed with C57Bl6 partners for at least four generations at the animal facility of the University of Geneva (Ravier et al. Citation2005; Speier et al. Citation2007) were anesthetized (5% isoflurane) and sacrificed as per the regulations of our institutional and state committees on animal welfare. Islets of Langerhans were isolated from the mice pancreas, as previously reported (Ravier et al. Citation2005).
RNA Analysis
Total RNA was extracted from N2A and MIN6 cells using Trizol (Invitrogen), as recommended by the manufacturer. The RNA quality was controlled using a laser detection technique (Agilent 2100 bioanalyser). One microgram RNA was reverse-transcribed using 200 U Reverse Transcriptase Superscript II (Invitrogen), 10 ng/μ l random hexamer primers (Promega), and 500 μ M each dNTP mix (Invitrogen). The mixture was heated to 70°C for 10 min and quickly chilled on ice. First-strand buffer (1×), 10 mM dithiothreitol, and 200 U Superscript II Reverse transcriptase (all reagents from Invitrogen) were then added, and the samples were exposed for 10 min at 20°C, for 50 min at 42°C, and for 15 min at 70°C. RNase H (0.5 U; Roche) was then added for 20 min at 37°C. Sixty microliters total RNA were mixed with 10× buffer (Finnzymes), 200 μ M each dNTP, 300 nM each primer, and 0.02 U/μ l Dynazyme (Finnzymes). The following specific primers were used for amplification: Cx36 (559-bp amplicon): 5′-AGT GCC AGG GAG GTG TGA AT-3′ (forward [F]) and 5′-GAG CCC AGG CCA AGA GGA AGT C-3′ (reverse [R]); pannexin1 (94-bp amplicon): 5′-GAT CTC CAT CGG TAC CCA GA.-3′ (F) and 5′-TAC AGC AGC CCA GCA GTA T-3′ (R); pannexin2 (94-bp amplicon): 5′-AGA ACT TCG CAG AGG AAC CA-3′ (F) and 5′-AGC TCT GTC CAG CAG TAG CC-3′ (R); pannexin3 (104-bp amplicon): 5′-AGT TCA TTA CTG TGG GCT TTC C-3′ (F) and 5′-ACT GAA GTT GCT GGG AGA GAA A-3′ (R); P2X7r (69-bp amplicon): 5′-TGT GAA GTC TCT GCC TGG TG-3′ (F) and 5′-GCT CCT CAA GAG TGC AGG TC-3′ (R). All PCR amplifications were performed in a T Gradient Thermocycler 96 (Biometra GmbH, DE), using the following protocol: 1 min at 94°C, 40 s at 60°C, 35 cycles of 1 min at 72°C, and a 5-min final extension step at 72°C. PCR products were resolved by 2% agarose gel electrophoresis and visualized by ethidium bromide staining.
Western Blots
Confluent cultures of wild type MIN6 and Cx36-null MIN6 cells were lysed in Tris-buffered solution containing 1% Triton-X-100 (Sigma) and protease inhibitor cocktail (Sigma). Samples of whole-cell lysates and of enriched membrane fractions were subjected to electrophoresis using sodium dodecyl sulfate–polyacrylamide gel electrophoresis (SDS-Page) mini-gels (4% to 20%; BioRad). Proteins were transferred to nitrocellulose membranes (Schleider & Schuell) and blocked 1 h at room temperature with 2% non-fat dry milk and 0.05% Tween-20 in phosphate-buffered saline (PBS). Membrane were then blotted for 2 h at room temperature using either anti-P2X7R (1:500; Alomone Labs, Jerusalem, Israel) or anti-pannexin1 (1:2000; a gift from Dr. G. Dahl, University of Miami) antibodies After several washes in the PBS-Tween 20 solution, membranes were incubated for 2 h with goat anti-rabbit or rabbit anti-chicken horseradish peroxidase (HRP)-conjugated secondary antibodies (1:2000). Bands were visualized in x-ray films (Kodak) after incubation with the enhanced chemiluminescence reagent (Amersham).
Immunofluorescence
N2A and MIN6 cells were grown on glass coverslips, fixed 10 min in 4% paraformaldehyde, incubated 30 min in 10 mM NH4Cl, rinsed in PBS containing 2% bovine serum albumin (BSA), and incubated for 2 h at room temperature in the presence of a chicken polyclonal antibody against pannexin1 (1:500; Diatheva, Fano, Italy). After repeated rinsing, cells were incubated a second time for 1 h at room temperature, using fluorescein-conjugated antibodies against chicken immunoglobulin G (Ig)ss (1:300; Abcam, Cambridge, UK). After additional rinsing, cells were counterstained with 0.03% Evan's blue (which gives a red background staining when viewed by fluorescence using the 543 nm excitation wavelength and the filter for rhodamine detection), coverslipped, and observed under ultraviolet (UV) illumination with 63× objective in an Axioplan I microscope (Zeiss, Oberkochen, Germany). Images were recorded using a color digital AxioCam camera (Zeiss).
Ethidium Bromide Uptake
Aliquots (3 × 104 cells) of N2A or MIN6 cells were plated on glass-bottomed dishes (MatTek, Ashland, MA) and cultured 1 to 3 days. Individual islets were also plated on these dishes and tested within 8 h after isolation. At the time of experimentation, the cells or islet medium was replaced by 200 μ l of one of the following solutions: Krebs-Ringer-bicarbonate buffer containing 20 mM glucose and supplemented with 20 μ M ethidium bromide (control KRB); control KRB containing 300 μ M BzATP (Sigma Chemical, St. Louis, MO); hypotonic control KRB, made by reducing by 15% the concentration of NaCl or by a 50% dilution of control KRB in distilled water; Ca2 +-free KRB, prepared without Ca2 +, and whose osmolarity was maintained by proportionally increasing the concentration of NaCl; Ca2 +-free KRB containing 300 μ M BzATP. The osmolarities of these solutions were 312, 273, 162, and 314 Osm in control, 15% hypotonic, 50% hypotonic, and Ca2 +-free KRB, respectively. The time course of dye uptake was monitored at room temperature, using an inverted Axiovert 100M microscope (Zeiss) equipped with a Ph1 Plan-Neofluar 20×/0.50 objective, filter sets to detect the intracellular fluorescence of ethidium bromide, and a high resolution black/white CCD camera (Hamamatsu Photonics, Massy, France) coupled to the OpenLab Software (Improvision, Waltham, MA). Time-lapse video recordings of this uptake were taken for 10 min, at 30-s intervals, and analyzed using the MetaMorph software (Molecular Devices, Sunnyvale, CA).
ATP Release
ATP levels in the bathing solution of MIN-6 cells were measured using a luciferin-luciferase assay, as previously described (Striedinger et al. Citation2007). Briefly, cell medium was replaced by 100 μ l of one of the following solutions: control KRB; control KRB plus 15 mM tetraethylammonium (TEA), Ca2 +-free KRB, Ca2 +-free KRB plus 15 mM TEA, and 200 μ M diazoxide (Sigma Chemical). After a 2-min incubation at 37°C, aliquots of bathing solution were rapidly collected and frozen at −20°C until measurements. Cells were then lysed with control KRB containing 0.1% triton-X100 for evaluation of total ATP content. Measurements of released ATP were performed by adding 2 μ l each sample to 20 μl Nucleotide Monitoring Reagent (NMR) of the ApoGlow Assay Kit (Cambrex Bio Science, Rockland, ME), and by reading the luminescence (RLU) using a Sirius Single Tube Luminometer (Berthold Detection Systems, Oak Ridge, TN), and a 10-s integration time. Background luminescence of the NMR solution was evaluated before adding the sample solutions, and subtracted from each sample reading. ATP concentration, expressed in nanomolar, was calculated according to standard curves obtained by plotting RLU as a function of serial dilutions of ATP (50 μ M, 5 μ M, 500 nM, 50 nM) prepared in the corresponding solution. Total amount of intracellular ATP was measured in the supernatant of cells lysed in the triton-containing solution.
Electrophysiology
N2A and MIN6 cells were plated on coverslips 24 to 48 h prior to recordings. The whole-cell patch clamp configuration was performed at room temperature on cells bathed in a solution containing (mM): NaCl 147, Hepes 10, glucose 13, CaCl2 2, MgCl2 1, and KCl 5, pH 7,4. The pipette solution contained (mM): CsCl 130, EGTA 10, Hepes 10, CaCl2 0.5. Activation of pannexin1 channels by voltage was performed using a ramp protocol that applied voltages from −60 to +100mV. Currents were measured during the first 3 ramps. To block the “hemichannels,” cells were acutely exposed to 50 μ m carbenoxolone (Sigma Chemical).
Data Representation and Statistical Analyses
Data are shown as mean ± SEM values of the indicated number of experiments, anaylsis of variance (ANOVA) followed by Newman-Keuls' multiple comparison test or by unpaired t test were used for statistical analyses.
RESULTS
N2A and MIN6 Cells Differ in Connexin But Not Pannexin Expression
To validate our experimental approach, we used murine neuroblastoma cells of the N2A line, which have been reported to not express connexins (Srinivas et al. Citation1999). These cells were first compared to the murine insulin-producing cells of the MIN6 line for connexin and pannexin expression, as assessed by reverse transcriptase–polymerase chain reaction (RT-PCR). Using specific primers, we found that N2A cells express pannexin1 and pannexin2, as well as the P2X7 receptor, but not pannexin3 and either Cx36 or Cx43 transcripts (). In contrast, WT MIN6 cells were found to express pannexin1, minimal levels of pannexin2, the P2X7 receptor, and Cx36, but not pannexin3 and Cx43 transcripts (). Western blot analyses confirmed that MIN6 cells express the pannexin1 and P2X7R proteins, at levels that were comparable in wild-type and Cx36-null cells (). However, whereas pannexin1 was found in both whole-cell extracts and purified cell membranes ( and ), P2X7r was only detected in whole-cell extracts (). These results indicate that N2A and MIN6 cells share the same pannexin composition, but differ in terms of connexin expression and distribution of a purinergic receptor subtype.
Figure 1 N2A and MIN6 cells differ in connexin but not pannexin expression. (A) RT-PCR showed that N2A cells express pannexin1 (Px1) and pannexin2 (Px2), as well as the P2X7 receptor (P2X7r), but not pannexin3 (Px3) and either connexin36 (Cx36) or connexin43 (Cx43). In contrast, WT MIN6 cells express Px1, P2X7r, and Cx36, little Px2, but no Px3 and Cx43. (B) Western blot of whole extracts and purified membranes showed that both wild type (WT) and Cx36-null MIN6 cells (AS) expressed pannexin1 (Panx1), and inserted the protein into the cell membrane. Both cell types also expressed the P2X7 receptor, which, however, could not be detected on purified membrane extracts of MIN6 cells. (C) Immunofluorescence confirmed the presence of pannexin1 in both the cytoplasm and the membrane of N2A (right panel) and MIN6 cells (left panel). Bar, 20 μm.
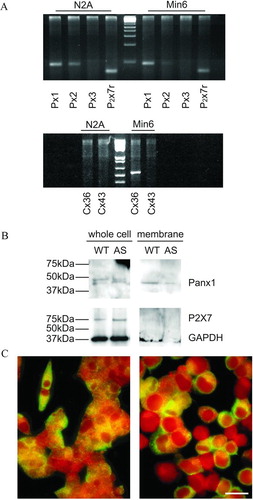
N2A Cells Express Functional “Hemichannels,” whereas MIN6 Cells Do Not
Using a high sensitivity video set-up, we monitored the time-dependent uptake of ethidium bromide, which was added to a variety of extracellular media. During stimulation of the P2X7 receptor by 300 μ M BzATP, we observed that all the single or clustered N2A cells we recorded progressively accumulated the dye, although at different levels, as indicated by the somewhat large standard deviations (). This uptake was more rapid, pronounced, and consistent in most, though not all N2A cells exposed to a Ca2 +-free KRB (). As expected for pannexin1 channels, mechanical strain, induced by a 50% hypotonic (162 mOsm) KRB, also increased the uptake of ethidium bromide (). Under the same conditions, WT MIN6 cells did not show a significant uptake of ethidium bromide (). Similar observations were made with MIN6 cells that had been transfected with an antisense Cx36 cDNA and failed to express the connexin at the cell membrane (data not shown). These data show that conditions revealing functional “hemichannels” in N2A cells did not reveal such channels in MIN6 cells.
Figure 2 N2A cells express functional “hemichannels.” Time course of ethidium bromide uptake by N2A cells (A) stimulated with 300 μM BzATP (n = 34 cells, 100% of the cells showed small uptake in a Ca2 +-containing medium; (B) exposed to a Ca2 +-free KRB (n = 34 cells, 81% of the cells showed uptake [solid symbols], but 19% did not [open symbols]); or (C) mechanically stressed by exposure to a 50% diluted KRB (n = 35 cells, 63% of the cells showed uptake [solid symbols], but 37% did not [open symbols]). Data are mean ± SEM of three experiments.
![Figure 2 N2A cells express functional “hemichannels.” Time course of ethidium bromide uptake by N2A cells (A) stimulated with 300 μM BzATP (n = 34 cells, 100% of the cells showed small uptake in a Ca2 +-containing medium; (B) exposed to a Ca2 +-free KRB (n = 34 cells, 81% of the cells showed uptake [solid symbols], but 19% did not [open symbols]); or (C) mechanically stressed by exposure to a 50% diluted KRB (n = 35 cells, 63% of the cells showed uptake [solid symbols], but 37% did not [open symbols]). Data are mean ± SEM of three experiments.](/cms/asset/50069b5d-0170-4e09-8deb-2e7af050d918/icac_a_301591_uf0002_b.gif)
Figure 3 MIN6 cells do not express functional “hemichannels.” Time course of ethidium bromide uptake recorded under the same conditions used to screen N2A cells failed to reveal any uptake of ethidium bromide by MIN6 cells (A) stimulated with 300 μ M BzATP (n = 20 cells); (B) exposed to a Ca2 +-free KRB (n = 20 cells); or (C) mechanically stressed by a 50% hypotonic shock (n = 35 cells). Data are mean ± SEM of three experiments.
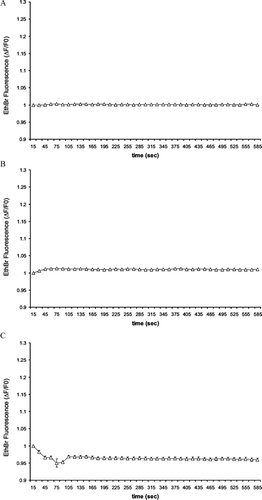
MIN6 Cells Release ATP Only via the Exocytotic Route
To evaluate whether MIN6 cells express “hemichannels” that could have escaped detection by the tracer uptake protocol, we tested their release of ATP under conditions favoring or preventing insulin release, since insulin secretory granules contain abundant ATP (Detimary et al. Citation1996). Stimulation of WT MIN6 cells by 20 mM glucose elicited a sizable release of ATP (), which was increased by TEA and even further in the absence of extracellular Ca2 +, but was prevented in the presence of the exocytosis blocker diazoxide (). Similar observations were made in Cx36-null MIN6 cells (), as a result of transfection with an antisense Cx36 cDNA. The two cell types had a similar content of ATP (data not shown). These data show that stimulated MIN6 cells release ATP mainly, and possibly exclusively, via the exocytotic pathway.
Figure 4 MIN6 cells release ATP only via the exocytotic route. Stimulation of wild-type MIN6 cells (MIN6) by glucose elicited an ATP release (solid column), which was increased by TEA (grey column) and even further in the absence of extracellular Ca2 + (hatched column), but was prevented by the exocytosis blocker diazoxide (open column). Similar observations were made in a clone of MIN6 cells that lacks Cx36 gap junctions (MIN6-AS), as a result of transfection with an antisense Cx36 cDNA.
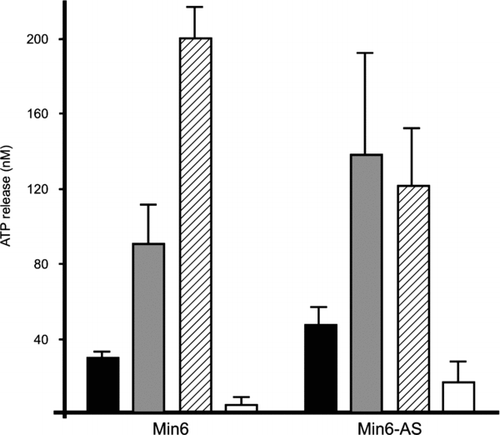
Membrane Depolarization Does Not Induce Carbenoxolone-Sensitive Currents in MIN6 Cells
Although the previous experiments failed to demonstrate the transfer of membrane-impermeant molecules, the presence of pannexin1 in membrane fractions of MIN6 cells prompted us to evaluate whether this protein could form “hemichannels” under conditions of membrane depolarization. Application of depolarizing ramps to single, patch clamped cells revealed outward currents in both N2A and MIN6 cells, whenever the depolarization exceeded +30 mV. At +100 mV, the mean value of total membrane conductance of channels allowing for this current in MIN6 cells (0.62 ± 0.12 nS; N = 13 cells) was not statistically different from that recorded from N2A cells (1.59 ± 0.65 nS; N = 13 cells) (). As indicated by the frequency distribution of the number of cells displaying outward currents in both cell lines cells (), 10 of 13 MIN6 cells displayed a conductance in the range of 500 pS, which is compatible with a single pannexin1 channel (Bao et al. Citation2004), whereas 7 of 13 N2A cells displayed conductance attributable to two or more Panx1 channels. To test whether these conductances were contributed by Panx1 channels, we tested for their sensitivity to a gap junction channel blocker. Superfusion of 50 μM carbenoxolone, a condition which preferentially blocks pannexin rather than connexin “hemichannels” (Bruzzone et al. Citation2005; Spray et al. Citation2006), reduced by 50% the total membrane conductance (0.71 ± 0.09 nS; N = 4 cells) of N2A cells, but did not affect membrane conductance (0.62 ± 0.08 nS; N = 6 cells) of MIN6 cells. Thus, these data indicate that MIN6 cells are devoid of functional “hemichannels.”
Figure 5 N2A but not MIN6 cells show “hemichannels” after supra-physiological depolarization. (A) Bar histogram of the mean total membrane conductance measured at +100 mV during a voltage ramp from −60 to +100mV. Single N2A and wild-type MIN6 cells were patch-clamped and exposed to a ramp of increasing depolarization steps. For depolarizations above +30 mV, both N2A and MIN6 cell types showed outward currents, with total membrane conductance of about 0.7 nS in MIN6 cells and 1.5 nS in N2A cells. Currents recorded from N2A but not from MIN6 cells were reduced by carbenoxolone (see text), indicating the existence in N2A cells of functional “hemichannels” presumably made by pannexin1. (B) Frequency distribution of the number of MIN6 (black bars) and N2A (gray bars) cells displaying outward currents measured at +100 mV.
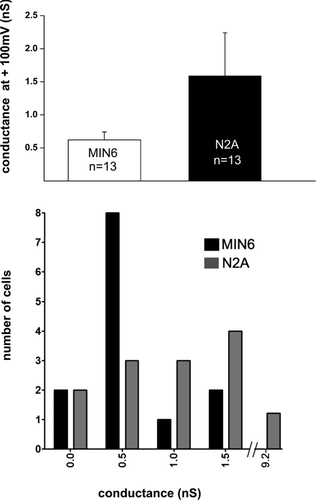
Primary β-Cells Do Not Express Functional “Hemichannels”
To assess whether the lack of detectable “hemichannels” as measured by uptake of tracers may be related to the transformed nature of MIN6 cells, we investigated primary insulin-producing β-cells within normal pancreatic islets, freshly isolated from mouse pancreas. After a 10-to 30-min exposure to ethidium bromide, a few β-cell nuclei were found stained by the dye (), presumably reflecting naturally dying cells and/or cells damaged during the isolation procedure. The proportion of these cells did not change when islets were exposed to 300 μ M Bz-ATP, a Ca2 +-free KRB, or a 50% hypotonic KRB, and none of these cells showed overtime a cytosolic accumulation of the exogenous tracer (). Similar observations were made in islets isolated from homozygous Cx36-null transgenic mice (), which lack gap junctions and are uncoupled (Ravier et al. Citation2005; Speier et al. Citation2007). These data show that ethidium bromide–permeable “hemichannels” are not detectable in primary insulin-producing cells.
Figure 6 Primary islet cells do not express functional “hemichannels.” Confocal microscopy views of pancreatic islets isolated from control (left column) and homozygous Cx36-null transgenic mice (right column), after a 10-min exposure to control KRB (A); stimulation by 300 μ M BzATP (B); exposure to Ca2 +-free KRB (C); or mechanical stress by a 50% hypotonic medium (D). In both types of islets, none of the experimental conditions significantly increased the number of ethidium bromide-stained cells, over the basal number observed under control conditions (A). In all cases, the tracer labeled only the cell nuclei of presumably damaged cells. Bar, 65 μ m.
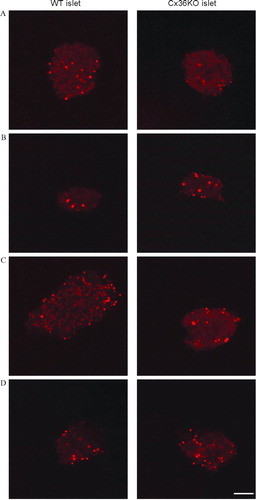
DISCUSSION
We have investigated transformed and primary insulin-producing cells, which feature Cx36 and pannexin1, for the presence of “hemichannel” activity that could lead to the membrane permeation by an exogenous tracer (ethidium bromide: molecular weight [MW] = 314, net charge = +1), and to release of an endogenous nucleotide (ATP: MW = 507, net charge −3). Under conditions of resting membrane potential, that easily showed the presence of such channels in N2A cells, we obtained no functional evidence for “hemichannels” in either MIN6 cells or primary pancreatic β-cells. This negative observation was made in spite of a sizable release of ATP during stimulation, which our data demonstrate to essentially occur via an exocytotic route, as anticipated by the presence of the nucleotide within the insulin-containing secretory granules (Detimary et al. Citation1996).
These data have at least two implications. First, they imply that neither Cx36 nor pannexin1 are per se sufficient to form functional “hemichannels” in insulin-producing cells. Cx36 makes β-to-β-cell channels that are permeated by a variety of tracers, including ethidium bromide (Ravier et al. Citation2005; Charpantier et al. Citation2007), and features one of the smallest unitary conductances (10 to 15 pS) yet observed between paired connexons (Srinivas et al. Citation1999). Even considering that Cx36 conductance would be expected to increase by about twofold in single, unpaired connexons (Harris Citation2001; Bukauskas and Verselis Citation2004, Spray et al. Citation2006), it is conceivable that Cx36 “hemichannels” may not provide a sufficient permeability to allow for a sizable passage of hydrophilic molecules across single cell membranes. The reason why pannexin1 channels are not active in MIN6 and pancreatic β-cells, in contrast to several other cell types (Bruzzone et al. Citation2005; Bao et al. Citation2004; Locovei et al. Citation2006a, Citation2006b, Citation2007), remains to be fully investigated. Interestingly, the P2X7 receptors of MIN6 cells are not detected at the cell membrane, suggesting that their cytoplasmic compartmentalization precludes pannexin1 activation. This may have important physiological implications given that high levels of ATP are co-released with insulin.
Second, our experiments document that the absence of “hemichannels” in cultured insulin-producing cells of the transformed MIN6 line reflects the situation in the primary endocrine pancreatic tissue. Thus, no functional “hemichannels” could be detected in freshly isolated islets of Langerhans, which, ex vivo, retain most of the normal features of primary β-cells in situ, notably with regard to insulin secretion and Cx36 channels (Ravier et al. Citation2005; Speier et al. Citation2007). The data provide evidence that, even if Cx36 were to form “hemichannels” in situ, these channels are unlikely to play a major physiological role. Thus, “hemichannels” are unlikely to account for the secretory alterations that have been observed in null and conditionally deleted transgenic mice that had lost β-cell Cx36 and gap junctions (Ravier et al. Citation2005; Speier et al. Citation2007, Wellershaus et al. 2007).
The in vivo existence of functional “hemichannels,” whether formed by connexin or pannexin proteins, and their potential physiological relevance are a matter of present excitement and debate (Goodenough and Paul Citation2003; Stout et al. Citation2004; Saez et al. Citation2005; Spray et al. Citation2006). The controversial views on these points cannot be unambiguously addressed at this time, because adequate tools to selectively interfere with these putative structures are lacking to sort the respective contributions, if any, of connexin and pannexin proteins. In this perspective, the finding that insulin-producing cells lack functional “hemichannels” in vitro and in vivo offers an opportunity to evaluate the mechanisms involved in the activation of these channels.
Work of our teams is supported by grants from the National Institute of Health (NS-41023 and NS-52245 to E.S., DK-060037 to D.S. and E.S., and HD-32573 and NS-41282 to D.S.), the Swiss National Science Foundation (310000-122430 to P.M.), the Juvenile Diabetes Research Foundation (1-2005-46 and 1-2007-158 to P.M.), Novo Nordisk (to P.M.), and the Geneva Program for Metabolic Disorders (to P.M.).
REFERENCES
- Ashcroft F M. Ion Channels and Disease. Academic Press, San Diego 2000
- Bao L, Locovei S, Dahl G. Pannexin membrane channels are mechanosensitive conduits for ATP. FEBS Lett 2004; 572: 65–68
- Bröer S, Wagner C A. Membrane Transporter Diseases. Kluwer Academic, New York 2003
- Bruzzone R, Barbe M T, Jakob N J, Monyer H. Pharmacological properties of homomeric and heteromeric pannexin hemichannels expressed in Xenopus oocytes. J Neurochem 2005; 92: 1033–1043
- Bruzzone R, Hormuzdi S G, Barbe M T, Herb A, Monyer H. Pannexins, a family of gap junction proteins expressed in brain. Proc Natl Acad Sci U S A 2003; 100: 13644–13649
- Burnstock G. Physiology and pathophysiology of purinergic neurotransmission. Physiol Rev 2007; 87: 659–797
- Bukauskas F F, Verselis V K. Gap junction channel gating. Biochim Biophys Acta 2004; 1662: 42–60
- Calabrese A, Zhang M, Serre-Beinier V, Caton D, Mas C, Satin L S, et al. Connexin36 controls synchronization of Ca2 + oscillations and insulin secretion in MIN6 cells. Diabetes 2003; 52: 417–424
- Charpantier E, Cancela J, Meda P. Beta cells preferentially exchange cationic molecules via connexin 36 gap junction channels. Diabetologia 2007; 50: 2332–2341
- Detimary P, Jonas J C, Henquin J C. Stable and diffusible pools of nucleotides in pancreatic islet cells. Endocrinology 1996; 137: 4671–4676
- DeVries S H, Schwartz E A. Hemi-gap-junction channels in solitary horizontal cells of the catfish retina. J Physiol 1992; 445: 201–230
- Goodenough D A, Paul D L. Beyond the gap: functions of unpaired connexon channels. Nat Rev Mol Cell Biol 2003; 4: 285–294
- Harris A L. Emerging issues of connexin channels: biophysics fills the gap. Q Rev Biophys 2001; 34: 325–472
- Hofer A, Dermietzel R. Visualization and functional blocking of gap junction hemichannels (connexons) with antibodies against external loop domains in astrocytes. Glia 1998; 24: 141–154
- Kondo R P, Wang S Y, John S A, Weiss J N, Goldhaber J I. Metabolic inhibition activates a non-selective current through connexin hemichannels in isolated ventricular myocytes. J Mol Cell Cardiol. 2000; 32: 1859–1872
- Li H, Liu T F, Lazrak A, Peracchia C, Goldberg G S, Lampe P D, Johnson R G. Properties and regulation of gap junctional hemichannels in the plasma membranes of cultured cells. J Cell Biol 1996; 134: 1019–1030
- Liu T F, Li H Y, Atkinson M M, Johnson R G. Comparison of lucifer yellow leakage and cell-to-cell transfer following intracellular injection in normal and antisense Novikoff cells under treatment with low extracellular Ca2 +. Methods Find Exp Clin Pharmacol 1996; 18: 493–497
- Locovei S, Bao L, Dahl G. Pannexin 1 in erythrocytes: function without a gap. Proc Natl Acad Sci U S A 2006a; 103: 7655–7659
- Locovei S, Scemes E, Qiu F, Spray D C, Dahl G. Pannexin1 is part of the pore forming unit of the P2X7 receptor death complex. FEBS Lett 2007; 581: 483–488
- Locovei S, Wang J, Dahl G. Activation of pannexin1 channels by ATP through P2Y receptors and by cytoplasmic calcium. FEBS Lett 2006b; 580: 239–244
- Musil L S, Goodenough D A. Biochemical analysis of connexin43 intracellular transport, phosphorylation, and assembly into gap junctional plaques. J Cell Biol 1991; 115: 1357–1374
- Panchin Y, Kelmanso I, Matz M, Lukyanov K, Usman N, Lukyanov S. A ubiquitous family of putative gap junction molecules. Curr Biol 2000; 10: R473–R474
- Paul D L, Ebihara L, Takemoto L J, Swenson K I, Goodenough D A. Connexin46, a novel lens gap junction protein, induces voltage-gated currents in nonjunctional plasma membrane of Xenopus oocytes. J Cell Biol 1991; 115: 1077–1089
- Pelegrin P, Surprenant A. Pannexin-1 mediates large pore formation and interleukin-1β release by the ATP-gated P2X7 receptor. EMBO J 2006; 25: 5071–5082
- Purpura V, Scemes E, Spray D C. Mechanisms of glutamate release from astrocytes: Gap junction “hemichannels”, purinergic receptors and exocytotic release. Neurochem Int 2004; 45: 259–264
- Quist A P, Rhee S K, Lin H, Lal R. Physiological role of gap-junctional hemichannels. Extracellular calcium-dependent isosmotic volume regulation. J Cell Biol 2000; 148: 1063–1074
- Ravier M A, Guldenagel M, Charollais A, Gjinovci A, Caille D, Sohl G, et al. Loss of connexin36 channels alters beta-cell coupling, islet synchronization of glucose-induced Ca2 + and insulin oscillations, and basal insulin release. Diabetes 2005; 54: 1798–1807
- Saez J C, Retamal M A, Basilio D, Bukauskas F F, Bennett M V. Connexin-based gap junction hemichannels: gating mechanisms. Biochim Biophys Acta 2005; 1711: 215–224
- Speier S, Gjinovci A, Charollais A, Meda P, Rupnik M. Cx36-mediated coupling reduces beta-cell heterogeneity, confines the stimulating glucose concentration range, and affects insulin release kinetics. Diabetes 2007; 56: 1078–1086
- Spray D C, YE Z C, Ramson B R. Functional connexin “hemichannels”: A critical appraisal. Glia 2006; 54: 758–773
- Srinivas M, Rozental R, Kojima T, Dermietzel R, Mehler M, Condorelli D F, Kessler J A, Spray D C. Functional properties of channels formed by the neuronal gap junction protein connexin36. J Neurosci 1999; 19: 9848–9855
- Stout C, Goodenough D A, Paul D L. Connexins: Functions without junctions. Curr Opin Cell Biol 2004; 16: 507–512
- Striedinger K, Meda P, Scemes E. Exocytosis of ATP from astrocyte progenitors modulates spontaneous Ca2 + oscillations and cell migration. Glia 2007; 55: 652–662
- Wellershaus K, Degen J, Deuchars J, Theis M, Charollais A, Caille D, Gauthier B, Janssen-Bienhold U, Meda P, Willecke K. A conditional mouse mutant with cyan fluorescent reporter protein reveals specific expression and functions of connexin36 in cerebral neurons and pancreatic beta-cells. Exp Cell Res 2008; 314: 997–1012