Abstract
Lens connexins are phosphorylated in vivo; however, the function and regulation of the phosphorylation remain largely unknown. We have previously identified an in vivo phosphorylation site, Ser364, at the COOH terminus of lens connexin (Cx) Cx45.6 and phosphorylation appears to regulate connexin protein turnover. To assess the specific mechanism of Ser364 phosphorylation in Cx45.6, exogenous wild type and Ser364 mutant Cx45.6 were expressed in primary lens cultures through retroviral infection. Cx45.6 turnover was attenuated primarily by proteasomal inhibitors and to a lesser extent by lysosomal inhibitors. Furthermore, the level of Cx45.6 protein in ubiquitin co-expressed cells was significantly reduced as compared to the cells expressing Cx45.6 alone. Moreover, overexpression of ubiquitin led to a more significant decrease in wild type Cx45.6 than Cx45.6(S364A), a mutant deficient of phosphorylation site at Ser364, although we did not detect any difference in the levels of ubiquitination between wild type and mutant Cx45.6. Interestingly, the mutant mimicking constitutive phosphorylation, Cx45.6(S364D), partially prevented the cleavage of Cx45.6 by caspase-3. Together, our data suggest that phosphorylation of Cx45.6 at Ser364 appears to stimulate Cx45.6 turnover primarily through proteasome pathway and this phosphorylation inhibits the cleavage of Cx45.6 by caspase-3. These findings provide further insights into regulatory mechanism of the specific phosphorylation of connexins in the lens.
INTRODUCTION
Gap junctions are clusters of channels between two adjacent cells, which allow passage of small molecules (Mr ≤ 1000 Da), such as small metabolites, ions, and second messengers. Gap junctions are formed by a family of proteins called connexins. Most connexins are phosphoproteins. The phosphorylation of connexins has been shown to play important roles in regulating the function of gap junctions, including channel assembly (Musil and Goodenough Citation1991; Solan and Lampe Citation2005), gap junction channel gating (Cottrell et al. Citation2003; Moreno A.P. and Lau Citation2007; Kuroki et al. Citation1998), intracellular trafficking, internalization and turnover (Berthoud et al. Citation1999; Guan and Ruch Citation1996; Hertlein et al. Citation1998; Hossain et al. Citation1998; Laird Citation2005). However, few in vivo phosphorylation sites of connexin have been identified and the physiological role of connexin phosphorylation, particularly the direct correlation of a specific in vivo phosphorylation site to its function is largely uncharacterized.
Gap junction-mediated intercellular communication plays an important role in the lens. The vertebrate lens is an important model system in the study of the function and regulation of gap junctions. The lens is an avascular organ composed of an anterior epithelial cell layer and highly differentiated fibers ranging from the outer cortex toward the central core. The epithelial cells begin to differentiate into fibercells at the lens equator. As new fiber cells arise, older cells are pushed centrally and eventually become mature lens fibers (Bassnett Citation2002). The metabolic activity and protein synthesis are conducted by the epithelium and the differentiating fibers at the lens peripheral region. Lens differentiation shares a number of morphological and biochemical characteristics with apoptotic cells, such as nucleus degeneration, loss of organelles, and activation of caspases (Dahm Citation1999; Wride et al. Citation1999; Wride Citation2000; Goodenough Citation1992). However, unlike apoptotic cells, which are rapidly digested, the organelle-free lens fibers retain their basic cell integrity and metabolism throughout the lifetime of the organ. Survival of lens cells relies on the intercellular communications between these cells and the cells at the lens surface through a large network of gap junctions that facilitate the exchange of ions and metabolites throughout the organ (Donaldson et al. Citation2001).
Three different connexins are expressed in the lens. Cx43 is mainly expressed in lens epithelial cells (Musil et al. Citation1990). When epithelial cells migrate toward the lens equator and gradually differentiate into fibers, Cx43 is down-regulated and replaced by two fiber connexins, namely Cx50 and Cx46 in the mammalian lens (Paul et al. Citation1991; White et al. Citation1992) and Cx45.6 and Cx56, respectively (Jiang et al. Citation1994; Jiang et al. Citation1994; Rup et al. Citation1993; White et al. Citation1998) in the chick lens. Cx45.6, different from Cx43 and Cx56, plays a distinctive role in lens development and differentiation. Primary lens cultures closely mimic lens cell differentiation in vivo (Menko et al. Citation1984). We have shown that overexpression of Cx45.6 in lens primary cultures promotes fiber-like structure (“lentoid”) formation as well as upregulates the expression of differentiation markers (Gu et al. Citation2003). Mice deficient in Cx50 display a reduced lens size (Rong et al. Citation2002; White et al. Citation1998), a phenotype not observed in Cx46 knockout mice (Gong et al. Citation1997). Targeted replacement of Cx50 with Cx46 by genetic knock-in restores lens transparency, but does not restore normal growth (White Citation2002), suggesting that intrinsic properties of Cx50 are required for lens growth and differentiation. Cx45.6 is post-translationally modified by phosphorylation (Jiang et al. Citation1994). Protein kinase C (PKC) has been found to phosphorylate Cx45.6 (Jiang and Goodenough Citation1998a). Casein kinase (CK) I is another kinase responsible for the phosphorylation of Cx50, the ortholog of Cx45.6 in the ovine lens (Cheng and Louis Citation1999) and this phosphorylation results in reduction of intercellular coupling (Cheng and Louis Citation2001). We have previously identified that Cx45.6 is phosphorylated by CKII in vivo at Ser364 (formerly Ser363 by error) and this phosphorylation appears to accelerate Cx45.6 turnover (Yin et al. Citation2000). In addition to its phosphorylation, Cx50 has been shown to be gradually cleaved at its COOH terminus during lens development (Lin et al. Citation1997). We reported that Cx45.6 is cleaved by caspase-3-like protease (Yin et al. Citation2001).
Distinctive from other membrane proteins, connexin has a short half-life average between 1.5–5 hours (Laird et al. Citation1991; Saffitz et al. Citation2000). The highly dynamic property and high turnover rate of Cx43 are postulated to provide a regulatory mechanism in control of the levels of gap junctional communication (Musil et al. Citation2000; Saffitz et al. Citation2000); however, we know rather little about the turnover of other connexins. Increasing evidence suggests that phosphorylation is involved in connexin turnover. Phosphorylation has been shown to either promote or inhibit connexin turnover (Berthoud et al. Citation1999; Guan and Ruch Citation1996; Hertlein et al. Citation1998; Hossain et al. Citation1998; Yin et al. Citation2000), and treatment with proteasomal inhibitors results in an increase in phosphorylation of Cx43 (Girao and Pereira Citation2003; Qin et al. Citation2003). In the lens, phosphorylation stabilizes Cx56 (Berthoud et al. Citation1999). However, treatment of lens primary cell culture with PKC activator, 12-O-tetradecanoylphorbol-13-acetate (TPA), destabilizes Cx43, and degradation of Cx43 is inhibited by proteasomal inhibitors (Girao and Pereira Citation2003). Our previous report demonstrates that phosphorylation of Cx45.6 by CKII appears to regulate Cx45.6 turnover (Yin et al. Citation2000). However, the degradation pathway for lens connexins has not been resolved. Furthermore, little is known about the underlying mechanism between in vivo phosphorylation and regulation of connexin turnover and the cleavage by specific protease(s).
In this report, we showed that phosphorylation at Ser364 appeared to provide signals for Cx45.6 turnover and this turnover was likely to be mediated by the proteasomal pathway. Furthermore, the phosphorylation at Ser364 played a major role in the attenuation of the cleavage of Cx45.6 by caspase-3.
MATERIALS AND METHODS
Antibodies
Anti-flag M2 monoclonal antibody was from Sigma (St. Louis, MO). The affinity-purified anti-Cx45.6 antibody specific for the entire intercellular loop region of Cx45.6 was prepared as described previously (Jiang et al. Citation1994). Anti-myc antibody was obtained from BD Biosciences (Bedford, MA). Anti-β-actin antibody was obtained from Pierce (Rockford, IL). Peroxidase-conjugated goat anti-rabbit and anti-mouse secondary antibodies were from Amersham Pharmacia Biotech (Piscataway, NJ).
Preparation of DNA Constructs and High Titer Retroviruses Expressing Wild Type and Mutant Cx45.6
Retroviral constructs and high titer viruses were prepared based on our protocol described previously (Jiang Citation2001). Briefly, cDNA fragment containing the COOH-terminal flag-tagged wild type Cx45.6 was generated by polymerase chain reaction (PCR). NcoI/EcoRI restriction sites were created at NH2-and COOH-terminal ends of the DNA fragment, respectively. The DNA fragment was subcloned into an adapter vector, Cla12NCO, which shared the same ClaI restriction site as retroviral vector, RCAS(A) (Hughes et al. Citation1987). To ensure the correct sequence of the construct, the DNA fragment was sequenced at the University Of Texas Health Science Center at San Antonio (UTHSCSA) DNA Sequencing Facility. The connexin fragment was isolated following digestion with ClaI and subcloned into ClaI-linearized RCAS(A). The construct with the correct inserts was selected for retrovirus preparation. The high titer retroviruses (1–5 × 108 CFU/ml)) containing RCAS(A) Cx45.6 construct were prepared as described previously (Jiang and Goodenough Citation1998b). For expression in neuro2A mouse neuroblastoma (N2A) cells, flag-tagged wild type Cx45.6 was subcloned into the EcoRI site of the pIRE2-EGFP expression vector (kindly provided by Dr. David Paul from Harvard Medical School, Boston, MA). With wild type RCAS(A)-Cx45.6 and pIRE2-EGFP-Cx45.6 DNA constructs as templates, Cx45.6 mutants containing point mutations were generated with the QuikChange™ Site-directed Mutagenesis kit (Stratagene, La Jolla, CA) according to the manufacturer's instructions. The codon AGC encoding Ser364 was changed to GAC encoding Asp and GCC encoding Ala. The correctness of the sequences of all constructs generated was confirmed at the UTHSCSA DNA Sequencing Facility.
Preparation of Lens Primary Cell Culture and Immunoprecipitation Assay
Primary lens cultures were prepared according to the procedure described previously (Musil et al. Citation1990; Yin et al. Citation2000; Yin et al. Citation2001). Chick lenses dissected from embryonic day 11 embryos were ruptured, and the cells dissociated from the lenses were plated at 1 × 106 cells per 35-mm tissue culture plates in medium 199 containing 10% fetal bovine serum. At the second day after cell plating, high titer retroviruses (5 μ l/dish) carrying Cx45.6 cDNAs were added to primary lens cultures. The culture medium was changed every other day. Seven days after culturing, the cells were then lysed in lysis buffer (5 mM Tris, pH 8.0, and 5 mM EDTA/EGTA plus 2 mM phenylmethylsulfonyl fluoride, 5 mM N-ethylmaleimide, 100 μ M leupeptin and 1 mM Na3VO4), homogenized with a 26 gauge needle, and boiled in 0.6% SDS. The cell lysates were precleared by incubating in immunoprecipitation buffer (0.02 M sodium borate, 15 mM EDTA, 0.1 M NaCl, pH 8.5) and protein A-Sepharose beads at 4°C for 2 h. After centrifugation, the supernatant was then incubated with affinity-purified Cx45.6 antibody at 4°C overnight. Protein A-sepharose beads were then added to the solution containing cell lysates and anti-Cx45.6 antibody, and incubated for 1 h at 4°C. The beads were washed five times with wash solution (addition of 0.05% Triton-X-100, 0.1% SDS, 0.2 M sucrose in immunoprecipitation buffer) and the immunoprecipitated samples were eluted from the beads by boiling in electrophoresis sample buffer (50 mM Tris, pH 6.8, 1% SDS, 2% β-mercaptoethanol, and 15% glycerol) for 5 min.
Western Blots
The crude membrane samples supposedly containing plasma, nuclear and organelle membranes or cell lysates were separated by 10% or 7.5% SDS-polyacrylamide gel electrophoresis (PAGE). Proteins on SDS-PAGE were transferred to nitrocellulose membranes and blotted with anti-Cx45.6 (1:500), anti-flag (1:2000), anti-myc (1:5000), or anti-β-actin (1:5000) antibody. The primary antibodies were detected by peroxidase-conjugated goat anti-rabbit or anti-mouse secondary antibody (1:5000) followed by chemiluminescence reagent kit (ECL) (Amersham Pharmacia Biotech, Piscataway, NJ) according to the manufacturer's instruction. The membranes were exposed to X-Omat AR films (Eastman Kodak, Rochester, NY) and detected by fluorography. The intensity of the bands on Western blot was quantified by densitometry (ImageJ software, National Institutes of Health, Bethesda, Maryland).
Treatment of Lens Primary Cell Cultures with Cycloheximide and/or Protease Inhibitors
Primary lens cells cultured for 7 days were subjected to the treatment by 20 μ g/ml cycloheximide with proteasomal or lysosomal inhibitors for various time periods. The proteasomal inhibitors used are: 10 μ M clasto-lactacystin β-lactone (Calbiochem, La Jolla, CA) and 0.5 μ M epoxomicin (Calbiochem). The lysosomal inhibitors used are 100 μ M leupeptin (Sigma) and 10 mM NH4Cl. Cells were collected in lysis buffer and centrifuged at 100,000 ×g for 30 min (Beckman, 28,000 rpm, SW60TI rotor). Crude cell membranes were resuspended in lysis buffer, boiled in 0.6% SDS, and immunoblotted with anti-Cx45.6 antibody.
Expression of Cx45.6 and Ubiquitin in N2A Cells
N2A cells were cultured in DMEM with 10% fetal calf serum in 6 well plates and transiently transfected with pIRE2-EGFP-Cx45.6 and mutants with or without PCS2-ubiquitin-myc (generously provided by Dr. Renee Yew at the UTHSCSA) using LIPOFECTAMINE PLUSTM reagent (Invitrogen, Carlsbad, CA). Twenty-four hours after transfection, cells were collected in lysis buffer (50 mM Hepes, pH 7.6, 150 mM NaCl, 1% Triton X-100, and 0.1% SDS), homogenized with a 26 gauge needle, and microcentrifuged at 13,000 rpm for 40 min at 4°C. The supernatant was used for either western blotting or immunoprecipitation using affinity-purified Cx45.6 antibody. The immunoprecipitation was performed as above described except that the affinity-purified Cx45.6 antibody was covalently conjugated to protein A beads. The immunoprecipitates were separated on a 7.5% SDS-PAGE and immunoblotted by anti-myc monoclonal antibody.
In vitro Cleavage of Wild Type Cx45.6 and Mutants by Caspase-3
Chicken embryonic fibroblast (CEF) cells were plated at 1 × 105 cells in 35-mm tissue culture plates and grown in DMEM containing 10% fetal calf serum and 2% chicken serum. At the second day of the culture, the cells were infected with high titer retroviruses (5 μ l/dish). The cells continued growth until they reached confluence. The crude CEF cell membranes were isolated and incubated at 37°C in 25 μ l reaction buffer (50 mM Tris-HCl, pH 8.0, 0.5 mM EDTA, 0.5 mM sucrose, and 5% glycerol) with and without 90 units of caspase-3 (Sigma) for 6 h. Reactions were terminated by boiling in the electrophoresis sample buffer for 5 min. The eluted samples were examined by western blot with affinity-purified anti-Cx45.6 antibody.
RESULTS
The Degradation of Cx45.6 was Mediated Primarily through the Proteasome Pathway in Lens Primary Cell Cultures
To determine the turnover pathway of Cx45.6, lens primary cells were treated with cycloheximide plus proteasomal or lysosomal inhibitors (). The proteasomal inhibitor, clasto-lactacystin β-lactone, efficiently blocked the degradation of Cx45.6, as shown by the retention of both phosphorylated and nonphosphorylated forms of Cx45.6 (). Interestingly, epoxomicin, another proteasomal inhibitor, appear to mainly stabilize the phosphorylated Cx45.6, the faster migrating band (). Lysosomal inhibitors, leupeptin () and NH4Cl (), could not effectively inhibit the Cx45.6 degradation. The intensity measurement of Cx45.6 protein bands from three separate experiments further confirmed this observation (). Interestingly, the inhibition by proteasomal inhibitors is not obvious at early time. One possible reason could be due to slow membrane permeablization of these drugs or/and inaccessibility to proteasome when exposed to drugs at short period of time. Together, our results suggest that Cx45.6 degradation in the lens is primarily mediated through the proteasome-dependent pathway.
Figure 1 Cx45.6 degradation is primarily through proteasomal pathway. The lens primary cultures were treated with 20 μ g/ml of cycloheximide alone (A and B, control), cycloheximide plus lysosomal inhibitor, 100 μ M leupeptin (A), proteasomal inhibitor, 10 μ M clasto-lactacystin β-lactone (A), lysosomal inhibitor 10 mM NH4Cl (B), or proteasomal inhibitor, 0.5 μ M epoxomicin (B) for 0 h (lane 1), 4 h (lane 2), 10 h (lane 3), and 24 h (lane 4). The Cx45.6 protein bands from three separate Western blot analyses were quantified by densitometry (C). The data are presented as the mean ± s.e.m. (n = 3).
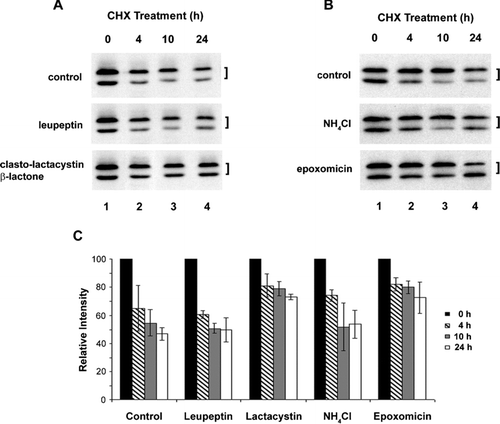
Cx45.6 was Degraded through a Phosphorylation-Mediated Ubiquitin-Dependent Pathway
We identified that Cx45.6 turnover was mediated by the proteasome-dependent pathway. The recognition of a protein substrate by proteasome normally requires polyubiquitination of the protein. Phosphorylation has been shown to regulate ubiquitination of a number of proteins (Hicke et al. Citation1998; Tedesco et al. Citation2002; Winston et al. Citation1999). To test whether phosphorylation at Ser364 is involved in the ubiquitination of Cx45.6, we initially attempted primary lens cells, but the ubiquitination was barely detectable (data not shown). To circumvent this problem, we expressed wild type Cx45.6 and Cx45.6(S364A) in connexin-deficient N2A cells with or without co-expression of c-myc-tagged ubiquitin. As compared to the levels of connexins in non-ubiquitin overexpressing cells (, lanes 1 and 2), co-expression of ubiquitin significantly reduced the levels of wild type and mutant Cx45.6 (, lanes 3 and 4). Although in the cells without co-expression of ubiquitin, the level of wild Cx45.6 was comparable to the mutant (, lanes 1–2), the level of wild type Cx45.6 was less than phosphorylation-deficient Cx45.6(S364A) in the ubiquitin-overexpressed cells (, lanes 3–4). Similar expression level of β-actin from each sample excluded the possibility that the altered level of Cx45.6 was due to loading variation. The ratio of Cx45.6 versus β-actin protein by densitomeric measurement further supported the observation (, right panel). These data indicate that phosphorylation at Ser364 appears to be involved in the regulation of ubiquitin-dependent degradation of Cx45.6.
Figure 2 Co-expression of ubiquitin and connexin constructs in N2A cells. N2A cells were transiently transfected with wild type and mutant Cx45.6 constructs with or without ubiquitin. Cells were lysed 24 h after transfection. (A) Same amount of proteins from wild type Cx45.6 (lanes 1 and 3) and Cx45.6(S364A) (lanes 2 and 4) transfected cells co-expressing ubiquitin (lanes 3 and 4) or without ubiquitin (lanes 1 and 2) were immunoblotted by anti-flag antibody and anti-β actin antibody. The protein band intensity was measured by densitometry and the intensity ratio of Cx45.6 versus β-actin was calculated (right panel). (n = 3). (B) The transfected N2A cell lysates were immunoprecipitated by anti-Cx45.6 antibody. The immunoprecipitates from ubiquitin only (lane 1), wild type Cx45.6 (lanes 2 and 4) and Cx45.6(S364A) (lanes 3 and 5) transfected cells co-expressing ubiquitin (lanes 4 and 5) or without ubiquitin (lanes 1–3) were analyzed on 7.5% SDS-PAGE and immunoblotted with anti-myc and anti-flag antibodies, respectively. (n = 3).
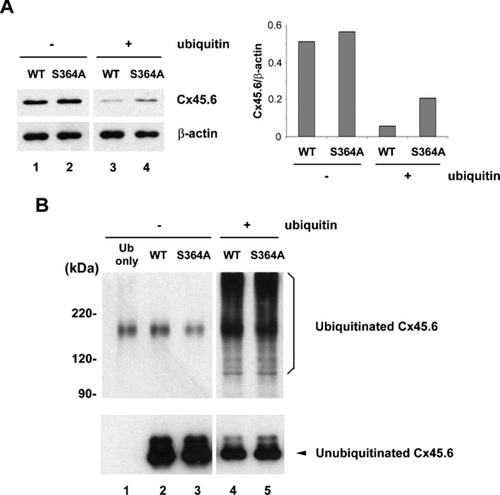
To further examine whether phosphorylation regulated ubiquitination process of Cx45.6, cell lysates were immunoprecipitated with anti-Cx45.6 antibody and the immunoprecipitates were analyzed by western blots. The portion of blots containing the ubiquitinated-Cx45.6 was blotted with anti-myc antibody, and the other portion containing the unubiquitinated Cx45.6 was blotted by anti-flag antibody. The results showed that both wild type and mutant Cx45.6 were polyubiquitinated () supporting the hypothesis that Cx45.6 was degraded through an ubiquitin-dependent pathway. However, no discernable difference in the levels of ubiquitination was observed between the wild type and the mutant.
The Cleavage of Cx45.6 by Caspase-3 was Regulated by Phosphorylation at Ser364 Residue
We have previously demonstrated that in vitro phosphorylation by CKII prevents Cx45.6 from cleavage by caspase-3 (Yin et al. Citation2001). Since phosphorylation at Ser364 has a role in enhancing Cx45.6 turnover, it would be interesting to determine whether phosphorylation at Ser364 regulates the cleavage of Cx45.6 by caspase-3. Ser364 on Cx45.6 was mutated to Asp residue to mimic the constitutively phosphorylated Cx45.6. The wild type Cx45.6 and single site mutant Cx45.6(S364D) were expressed in CEF cells via recombinant retroviral infection and treated with caspase-3. Here we used CEF cells since we have previously shown that exogenous lens connexins can be effectively expressed in avian cell systems, such as CEF cells, using retroviral infection approach, and importantly Cx45.6 protein is adequately post-translationally modified in CEF cells (Yin et al. Citation2000; Yin et al. Citation2001). Mutation of Ser364 to asparate greatly attenuated the cleavage of Cx45.6 by caspase-3 (, compare lanes 2 to 4), which was further confirmed by the band intensity ratio of truncated versus full-length Cx45.6 (, lower panel). These results suggest that phosphorylation at Ser364 is required to block Cx45.6 from the cleavage by caspase-3.
Figure 3 Constitutively phosphorylated mutant, Cx45.6(S364D), was resistant to the cleavage by caspase-3 as compared to wild type Cx45.6. Exogenous wild type (WT) Cx45.6 (lanes 1 and 2) and Cx45.6 single phosphorylation site mutants, Cx45.6(S364D) (lanes 3 and 4) were expressed in CEF cells through retroviral infection. Crude membranes isolated from those infected cultures were treated in the absence (lanes 1 and 3) and presence (lanes 2 and 4) of caspase-3 in vitro at 37°C for 6 h. Arrows indicate the cleaved fragments. The protein band intensity was measured by densitometry and the intensity ratio of truncated versus full-length Cx45.6 was calculated (lower panel). (n = 3).
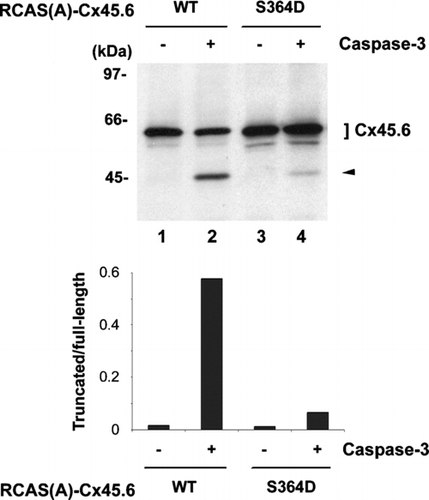
DISCUSSION
In this report, we showed that fast turnover of Cx45.6 by the phosphorylation at Ser364 appeared to be mediated through ubiquitin-proteasome pathway. The phosphorylation at Ser364 inhibited the cleavage of Cx45.6 by caspase-3. Together, as illustrated in , we have shown two different types of proteolysis of Cx45.6 occurring in the lens, which are alternatively regulated by the phosphorylation/dephosphorylation signals derived from Ser364. When a full-length Cx45.6 is phosphorylated at Ser364 (, A), which possibly provides signals for ubiquitination, Cx45.6 is likely to undergo degradation mediated through the ubiquitin-proteasome pathway. When Ser364 is not phosphorylated (, B), Cx45.6 renders itself into a substrate for caspase-3. Since caspase-3 has been shown to be activated in the region close to the border between the differentiating fibers and the mature fibers (Wride et al. Citation1999), the truncated Cx45.6 fragment eventually accumulates in the central core region during lens development.
Figure 4 A model for the regulation of proteolysis of Cx45.6 in the lens by phosphorylation at Ser364. (A) The phosphorylation at Ser364 renders Cx45.6 unstable and undergoes the degradation mediated by proteasome. (B) If Ser364 is not phosphorylated, Cx45.6 becomes a substrate for caspase-3, which cleaves behind Glu368. The truncated Cx45.6 mainly accumulates in the mature fibers at the center core region of the lens (Yin et al. Citation2001).
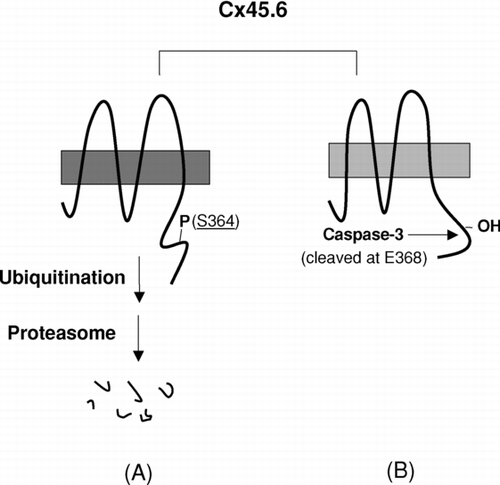
Lysosomes are generally thought to be the major machinery for degradation of the integral membrane proteins and proteasome is responsible for the turnover of most cytosolic, nuclear, and ER-retained misfolded or aggregated proteins. Lysosomal pathway has been demonstrated to be a major pathway in the turnover and degradation of connexins following internalization of gap junctions (reviewed by (Laird Citation2005)). Increasing evidence also reveals that the proteasome may participate in the turnover of plasma membrane proteins (Aviel et al. Citation2000; Hicke Citation1999) and connexins (Musil et al. Citation2000; VanSlyke and Musil Citation2002; Laing et al. Citation1998). The ubiquitin-proteasome pathway has been implicated for degradation of Cx43 (Laing and Beyer Citation1995). However, most of these studies were conducted in cells overexpressing connexins. Furthermore, several studies demonstrate that the treatment with proteasomal inhibitors accumulated the phosphorylated form of connexin in different types of the cell, while lysosomal inhibitors increase total connexins (Girao and Pereira Citation2003; Qin et al. Citation2003). Since the phosphorylation process of connexins occurs mainly after connexins reach plasma membrane (Musil and Goodenough Citation1991), the accumulation of the phosphorylated connexin by inactivation of proteasome implies that proteosomal pathway may play an important role in controlling the levels of connexin expressed on plasma membrane. Indeed, approximately one half of newly synthesized Cx43 and Cx32 molecules are reported to be degraded even if their exit from the ER is blocked (VanSlyke et al. Citation2000). A recent study by Kelly et al. (Citation2007) shows that cytosolic stress reduces ER-associated degradation by the proteasome (ERAD) at the level of substrate polyubiquitination, which in turn enhances surface expression of connexins. These studies suggest that proteasomal degradation possibly through polyubiquitination is one of the possible mechanisms in regulation of connexin levels on the plasma membrane and channel function. Our data further suggest that degradation of Cx45.6 by proteasome may provide an alternative in regulating the levels of the truncated Cx45.6, the functional form of the connexin, in the central core regions of lens fibers.
Mutation of Ser364 to alanine stabilized Cx45.6 in primary lens cell cultures and in N2A cells co-expressing ubiquitin, implying that phosphorylation is involved in regulation of Cx45.6 turnover through ubiquitin-dependent pathway. The role of phosphorylation in protein degradation has been suggested in regulation of ubiquitination of a number of proteins (Hicke et al. Citation1998; Lin et al. Citation2002; Tedesco et al. Citation2002; Winston et al. Citation1999; Tedesco et al. Citation2002). Proteolysis of Iκ Bα and β-catenin requires phosphorylation at two specific serine residues for ubiquitination and proteasome degradation (Winston et al. Citation1999). A recent study reported that ubiquitin protein ligase Nedd4 binds to Cx43; however, Nedd4 can bind to both phosphorylated and unphosphorylated forms of Cx43 (Leykauf et al. Citation2006). Intriguingly, we failed to show any difference in the ubiquitination levels between wild type Cx45.6 and phosphorylation deficient-mutant. A possible interpretation is that there are different pools of the ubiquitinated Cx45.6 and phosphorylation of Cx45.6 at Ser364 may regulate ubiquitination in a small portion of Cx45.6; thereby, it is difficult to distinguish the differences in ubiquitination between wild type and phosphorylation-deficient mutants by comparing the total amount of the polyubiquitinated Cx45.6. The lack of difference in ubiquitination between wild type and phosphorylation-deletion mutants could also be explained by the fact that the ubiquitination reactions of Cx45.6 are relatively transient and the difference in the degree of ubiquitination is hardly detectable experimentally. Indeed, ubiquitination of Cx45.6 was barely detectable in lens primary cells. Therefore, this study was conducted using N2A cells expressing exogenous Cx45.6 and ubiquitin. The cellular environment in N2A cells could be distinct from that in lens cells although we have previously showed that post-translational modification regimes of Cx45.6 were reproduced in non-lens cells (Yin et al. Citation2000, Citation2001). Alternatively, phosphorylation at S364 may have no role in the ubiquitination of Cx45.6; intriguingly, we show the amount of S364A mutant is less than wild type in ubiquitin-overexpressing cells. Additionally, we cannot exclude the possibility that the polyubiquintination and degradation observed in transfected N2A cells could be a consequence of protein overexpression. Increased protein turnover may help reduce potential protein overload of secretory pathway.
The turnover of Cx45.6 mediated by phosphorylation may play a regulatory role in lens differentiation. Increasing evidence shows that Cx45.6 and its mammalian ortholog, Cx50, play an important role in lens growth and differentiation (Gu et al. Citation2003; Rong et al. Citation2002; White et al. Citation1998). However, the mechanism is less clear. Upregulation of ubiquitin pathway, CKII, and caspase-3 have been demonstrated in lens cortex region comprising of differentiating fibers (Pereira et al. Citation2003; Shang et al. Citation1999; Wride et al. Citation1999). It has been shown that gap junctional communication can be regulated at the level of connexin turnover (Musil et al. Citation2000). One possible mechanism could be that lens fiber cells selectively retain certain numbers of Cx45.6 molecules in the nucleus through its truncation by caspase 3. As long as Cx45.6 is phosphorylated, it has to undergo protein turnover regime. By using this kind of molecular switching system, lens cells regulate numbers of Cx45.6 molecules retained in the central nucleus region. Preliminary result showed that the channels formed by the caspase-3-truncated Cx45.6 exhibited less pH sensitivity than the channels formed by the full length connexin (our unpublished results). This is consistent with the evidence that truncation of Cx50 in ovine lens core region results in loss of pH sensitivity of channel gating property (Lin et al. Citation1998). The lens center core is mildly acidic (∼ pH 6.5) (Bassnett and Duncan Citation1985) due to anaerobic metabolism and production of lactic acid, while the peripheral region maintains normal pH levels around pH 7.2. The spatial differential distribution of the full-length Cx45.6 in differentiating lens fibers and truncated Cx45.6 in the mature fibers may accommodate the pH gradient in the lens to maintain the channels at open state for tissue homeostasis. Therefore, the cleavage of Cx45.6 by caspase-3 has to be strictly regulated. Phosphorylation/unphosphorylation of Cx45.6 at Ser364 may provide a unique mechanism for the regulation of the formation and accumulation of the caspase-3-truncated Cx45.6 in the lens fibers.
ACKNOWLEDGMENTS
We thank Dr. R. P. Yew at the UTHSCSA for providing the PCS2-ubiquitin-myc construct, and Dr. D. L. Paul at the Harvard Medical School for providing the pIRE2-EGFP vector. We also thank C. J. Villegas for technical assistance and the members of the Jiang laboratory for critical reading of the manuscript. This study was supported by the National Institutes of Health grant EY12085 (J.X.J) and the Welch Foundation grant AQ-1507 (J.X.J)
REFERENCES
- Aviel S, Winberg G, Massucci M, Ciechanover A. Degradation of the Epstein-Barr latent membrane protein 1 (LMP) by the ubiquitin-proteasome pathway-targeting via ubiquitination of the N-terminal residue. J Biol Chem 2000; 275: 23491–23499
- Bassnett S. Lens organelle degradation. Exp Eye Res 2002; 74: 1–6
- Bassnett S, Duncan G. Direct measurement of pH in the rat lens by ion-sensitive microelectrodes. Exp Eye Res 1985; 40: 585–590
- Berthoud V M, Bassnett S, Beyer E C. Cultured chicken embryo lens cells resemble differentiating fiber cells in vivo and contain two kinetic pools of connexin56. Exp Eye Res 1999; 68: 475–484
- Cheng H L, Louis C F. Endogenous casein kinase I catalyzes the phosphorylation of the lens fiber cell connexin49. Eur J Biochem 1999; 263: 276–286
- Cheng H L, Louis C F. Functional effects of casein kinaes I-catalyzed phosphorylation on lens cell-to-cell coupling. J Membr Biol 2001; 181: 21–30
- Cottrell G T, Lin R, Warn-Cramer B J, Lau A F, Burt J M. Mechanism of v-Src-and mitogen-activated protein kinase-induced reduction of gap junction communication. Am J Physiol 2003; 284: C511–C520
- Dahm R. Lens fibre cell differentiation—A link with apoptosis?. Ophthalmic Res 1999; 31: 163–183
- Donaldson P, Kistler J, Mathias R T. Molecular solutions to mammalian lens transparency. News Physiol 2001; 16: 118–123
- Girao H, Pereira P. Phosphorylation of connexin 43 acts as a stimuli for proteasome-dependent degradation of the protein in lens epithelial cells. Mol Vis 2003; 9: 24–30
- Gong X, Li E, Klier G, Huang Q, Wu Y, Lei H, Kumar N M, Horwitz J, Gilua N B. Disruption of α 3 connexin gene leads to proteolysis and cataractogenesis in mice. Cell 1997; 91: 833–843
- Goodenough D A. The crystalline lens: a system networked by gap junctional intercellular communication. Seminars in Cell Biology, N. B. Gilula. Sauders Scientific Pub./Academic Press, London 1992; 49–58
- Gu S, Yu X S, Yin X, Jiang J X. Stimulation of lens cell differentiation by gap junction protein connexin 45.6. Invest Ophthalmol Vis Sci 2003; 44: 2103–2111
- Guan X J, Ruch R J. Gap junction endocytosis and lysosomal degradation of connexin43-p2 in wb-f344 rat liver epithelial cells treated with ddt and lindane. Carcinogenesis 1996; 17: 1791–1798
- Hertlein B, Butterweck A, Haubrich S, Willecke K, Traub O. Phosphorylated carboxy terminal serine residues stabilize the mouse gap junction protein connexin45 against degradation. J Membr Biol 1998; 162: 247–257
- Hicke L. Gettin'down with ubiquitin: turning off cell-surface receptors, transporters and channels. Trends Cell Biol 1999; 9: 107–112
- Hicke L, Zanolari B, Riezman H. Cytoplasmic tail phosphorylation of the alpha-factor receptor is required for its ubiquitination and internalization. J Cell Biol 1998; 141: 349–358
- Hossain M Z, Ao P, Boynton A L. Rapid disruption of gap junctional communication and phosphorylation of connexin43 by platelet-derived growth factor in T51B rat liver epithelial cells expressing platelet-derived growth factor receptor. J Cell Physiol 1998; 174: 66–77
- Hughes S H, Greenhouse J J, Petropoulos C J, Sutrave P. Adaptor plasmids simplify the insertion of foreign DNA into helper-independent retroviral vectors. J Virol 1987; 61: 3004–3012
- Jiang J X. Use of retroviruses to express connexins. Connexin methods and protocols, R. Bruzzone, C. Giaume. Humana Press, Totowa 2001; 159–174
- Jiang J X, Goodenough D A. Phosphorylation of lens fiber connexins by protein kinase C. Eur J Biochem 1998a; 255: 37–44
- Jiang J X, Goodenough D A. Retroviral expression of connexins in embryonic chick lens. Invest Ophthalmol Vis Sci 1998b; 39: 537–543
- Jiang J X, White T W, Goodenough D A, Paul D L. Molecular cloning and functional characterization of chick lens fiber connexin45.6. Mol Biol Cell 1994; 5: 363–373
- Kelly S M, VanSlyke J K, Musil L S. Regulation of ubiquitin-proteasome system-mediated degradation by cytosolic stress. Mol. Biol. Cell 2007; 18: 4279–4291
- Kuroki T, Inoguchi T, Umeda F, Ueda F, Nawata H. High glucose induces alteration of gap junction permeability and phosphorylation of connexin-43 in cultured aortic smooth muscle cells. Diabetes 1998; 47: 931–936
- Laing J G, Beyer E C. The gap junction protein connexin43 is degraded via the ubiquitin proteasome pathway. J Biol Chem 1995; 270: 26399–26403
- Laing J G, Tadros P N, Green K, Saffitz J E, Beyer E C. Proteolysis of connexin43-containing gap junctions in normal and heat-stressed cardiac myocytes. Cardiovasc Res 1998; 38: 711–718
- Laird D W. Connexin phosphorylation as a regulatory event linked to gap junction internalization and degradation. Biochim Biiophys Acta 2005; 1711: 172–182
- Laird D W, Puranam K L, Revel J P. Turnover and phosphorylation dynamics of connexin43 gap junction protein in cultured cardiac myocytes. Biochem J 1991; 273: 67–72
- Leykauf K, Salek M, Bomke J, Frech M, Lehmann W-D, Durst M, Alonso A. Ubiquitin protein ligase Nedd4 binds to connexin43 by a phosphorylation-modulated process. J Cell Sci 2006; 119: 3634–3642
- Lin H K, Wang L, Hu Y C, Altuwaijri S, Chang C. Phosphorylation-dependent ubiquitylation and degradation of androgen receptor by Akt require Mdm2 E3 ligase. EMBO J 2002; 21: 4037–4048
- Lin J S, Eckert R, Kistler J, Donaldson P. Spatial differences in gap junction gating in the lens are a consequence of connexin cleavage. Eur J Cell Biol 1998; 76: 246–250
- Lin J S, Fitzgerald S, Dong Y, Knight C, Donaldson P, Kistler J. Processing of the gap junction protein connexin50 in the ocular lens is accomplished by calpain. Eur J Cell Biol 1997; 73: 141–149
- Menko A S, Klukas K A, Johnson R G. Chicken embryo lens cultures mimic differentiation in the lens. Dev Biol 1984; 103: 129–141
- Moreno A P, Lau A F. Gap junction channel gating modulated through protein phosphorylation. Prog Biophys Mol Biol 2007; 94: 107–119
- Musil L S, Beyer E C, Goodenough D A. Expression of the gap junction protein connexin43 in embryonic chick lens: molecular cloning, ultrastructural localization, and post-translational phosphorylation. J Membr Biol 1990; 116: 163–175
- Musil L S, Goodenough D A. Biochemical analysis of connexin43 intracellular transport, phosphorylation, and assembly into gap junctional plaques. J Cell Biol 1991; 115: 1357–1374
- Musil L S, Le A-CN, VanSlyke J K, Roberts L M. Regulation of connexin degradation as a mechanism to increase gap junction assembly and function. J Biol Chem 2000; 275: 25207–25215
- Paul D L, Ebihara L, Takemoto L J, Swenson K I, Goodenough D A. Connexin46, a novel lens gap junction protein, induces voltage-gated currents in nonjunctional plasma membrane of Xenopus oocytes. J Cell Biol 1991; 115: 1077–1089
- Pereira P, Shang F, Hobbs M, Girao H, Taylor A. Lens fibers have a fully functional ubiquitin-proteasome pathway. Exp Eye Res 2003; 76: 623–631
- Qin H, Shao Q, Igdoura S A, Alaoui-Jamali M A, Laird D W. Lysosomal and proteasomal degradation play distinct roles in the life cycle of Cx43 in gap junctional intercellular communication-deficient and-competent breast tumor cells. J Biol Chem 2003; 278: 30005–30014
- Rong P, Wang X, Niesman I, Wu Y, Benedetti L E, Dunia I, Levy E, Gong X. Disruption of Gja8 (α 8 connexin) in mice leads to microphthalmia associated with retardation of lens growth and lens fiber maturation. Development 2002; 129: 167–174
- Rup D M, Veenstra R D, Wang Z, Brink P R, Beyer E C. Chick connexin-56, a novel lens gap junction protein. J Biol Chem 1993; 268: 706–712
- Saffitz J E, Laing J G, Yamada K A. Connexin expression and turnover-implications for cardiac excitability. Cir Res 2000; 86: 723–728
- Shang F, Gong X, McAvoy J W, Chamberlain C, Nowell T R, Taylor A. Ubiquitin-dependent pathway is up-regulated in differentiating lens cells. Exp Eye Res 1999; 68: 179–192
- Solan J L, Lampe P D. Connexin phosphorylation as a regulatory event linked to gap junction channel assembly. Biiochim Biophys Acta 2005; 1711: 154–163
- Tedesco D, Lukas J, Reed S I. The pRb-related protein p130 is regulated by phosphorylation-dependent proteolysis via the protein-ubiquitin ligase SCF (Skp2). Genes Dev 2002; 16: 2946–2957
- VanSlyke J K, Deschenes S M, Musil L S. Intracellular transport, assembly, and degradation of wild-type and disease-linked mutant gap junction protein. Mole Biol Cell 2000; 11: 1933–1946
- VanSlyke J K, Musil L S. Dislocation and degradation from the ER are regulated by cytosolic stress. J Cell Biol 2002; 157: 381–394
- White T W. Unique and redundant connexin contributions to lens development. Science 2002; 295: 319–320
- White T W, Bruzzone R, Goodenough D A, Paul D L. Mouse Cx50, a functional member of the connexin family of gap junction proteins, is the lens fiber protein MP70. Mol Biol Cell 1992; 3: 711–720
- White T W, Goodenough D A, Paul D L. Targeted ablation of connexin50 in mice results in microphthalmia and zonular pulverulent cataracts. J Cell Biol 1998; 143: 815–825
- Winston J T, Strack P, Beer-Romero P, Chu C Y, Elledge S J, Harper J W. The SCFβTRCP- ubiquitin ligase complex associates specifically with phosphorylated destruction motifs in Iκ Bα and β-catenin and stimulates Iκ Bα ubiquitination in vitro. Genes Dev 1999; 13: 270–283
- Wride M A. Minireview: apoptosis as seen through a lens. Apoptosis 2000; 5: 203–209
- Wride M A, Parker E, Sanders E J. Members of the Bcl-2 and caspase families regulate nuclear degeneration during chick lens fire differentiation. Dev Biol 1999; 213: 142–156
- Yin X, Gu S, Jiang J X. The development-associated cleavage of lens connexin 45.6 by caspase-3-like protease is regulated by casein kinase II-mediated phosphorylation. J Biol Chem 2001; 276: 34567–34572
- Yin X, Jedrzejewski P T, Jiang J X. Casein kinase II phosphorylates lens connexin 45.6 and is involved in its degradation. J Biol Chem 2000; 275: 6850–6856