Abstract
Salivary gland adenoid cystic carcinoma (SACC) is one of the most common malignancies in the oral and maxillofacial region. Carcinoma-associated fibroblast (CAF) is an important component in the tumor microenvironment and participates in SACC progression. In this study, we established a CAF cell line derived from a human SACC and named it CAF-SA. It was identified that CAF-SA expressed typical CAF biomarkers. Then, we studied the cellular communications between CAF-SA, tumor cells and endothelial cells. It was found that CAF-SA promoted the migration, invasion, and proliferation of SACC tumor cells in vitro. In addition, tube formation by endothelial cells was enhanced by CAF-SA. In vivo experiment showed that SACC cells formed larger xenografts in nude mice when they were transplanted with CAF-SA. Overall, we demonstrated that CAF-SA exhibited the most important defining feature of CAF by promoting cancer progression.
Introduction
Salivary gland adenoid cystic carcinoma (SACC) is one of the most common malignancies in the oral and maxillofacial region (Bruzgielewicz et al. Citation2011; Wang et al. Citation2012; Mizrachi et al. Citation2017). It shows a strong capacity for local invasion and a high incidence of distant metastasis (Gao et al. Citation2013; Dantas et al. Citation2015). Our previous studies demonstrated that carcinoma-associated fibroblast (CAF) derived from SACC showed high levels of MMP2 and CXCL12 expression, which might be related to the aggressive growth behavior of SACC (Guan et al. Citation2015). Furthermore, we demonstrated that SACC-derived CAF led SACC invasion by creating an invasive track in the extracellular matrix (ECM) (Li et al. Citation2016).
CAFs are the most abundant stromal cells in the tumor microenvironment (Olumi et al. Citation1999; Liotta and Kohn Citation2001; Attieh and Vignjevic Citation2016). They are derived from various origins, such as normal fibroblasts, smooth muscle cells, endothelial cells, myoepithelial cells, or mesenchymal stem cells. CAFs support tumor growth by both secreting many growth factors and stimulating angiogenesis. Based on immunohistochemical data, several CAF markers were identified including vimentin (VIM), fibroblast-specific protein-1 (FSP-1), fibroblast-activation protein (FAP), α-smooth muscle actin (α-SMA) (Albrengues et al. Citation2014).
To our best knowledge, there is no report on the CAF cell line from patients with SACC. Here, we report on the first establishment of a CAF cell line from human SACC. Its characterizations and cellular communications with both SACC tumor cells and endothelial cells were studied.
Materials and methods
Establishment of a CAF cell line and collection of conditioned medium
Primary CAF cells were isolated from a case of SACC tumor tissues and characterized in our previous studies (Li et al. Citation2016). When the primary CAF cells were subcultured over 10 passages, they were trypsinized, diluted and seeded in a 96-well plate. The wells with only a single cell were selected and marked. The single cell was incubated with DMEM/F12 (HyClone, Waltham, MA, USA) supplemented with 10% fetal bovine serum (FBS, ScienCell, San Diego, CA, USA), 100 U/mL penicillin, 100 U/mL streptomycin, 1% hypoxanthine sodium and thymine supplementary (Gibco, Waltham, MA, USA), and epidermal growth factor (EGF, PeproTech, Rocky Hill, NJ, USA) for 10 days in an incubator with 5% CO2 at 37 °C. The culture medium was changed every 3 days. After the single cell expanded, they were moved to a dish and subcultured continuously. The cell line was named CAF-SA.
Conditioned medium (CM) of CAF-SA was collected. When CAF-SA reached 70% confluence in a 25 cm2 flask (∼2 × 106 cells), they were rinsed with phosphate-buffered saline (PBS) and cultured continuously in 5 mL FBS-free medium for another 3 days. Then, the medium was collected and centrifuged at 2500g for 10 min to deplete cell debris.
General cell culture
Two SACC cell lines (SACC-LM and SACC-83) were used in this study. SACC-LM, SACC-83 and CAF-A1 were cultured in DMEM/F12 supplemented with 10% FBS, 100 U/mL penicillin, and 100 U/mL streptomycin. The human umbilical vein endothelial cells (HUVEC) were purchased from the American Type Culture Collection (ATCC, Manassas, VA, USA). They were cultured in endothelial cell medium (ScienCell, San Diego, CA, USA) supplemented with endothelial cell growth supplement, 7% FBS, 100 U/mL penicillin, and 100 U/mL streptomycin. All the cells were incubated in an incubator with 5% CO2 at 37 °C.
Short tandem repeat profiling
Genomic DNA was extracted from both CAF-A1 (third passage) and CAF-SA using commercial kit from Corning (AP-EMN-BL-GDNA-250G, Tewksbury, MA, USA) following the manufacturer’s instructions. Twenty STRs including amelogenin (for gender identification) were amplified by six multiplex PCR. The short tandem repeat (STR) analysis was performed using 3730xL DNA Analyzer (Applied Biosystems™, Thermo, Waltham, MA, USA) at the Shanghai Biowing Applied Biotechnology Co. Ltd. The signals were analyzed by GeneMapper (Shanghai, China).
Immunofluorescent staining
CAF-SA cells were cultured on a cover slide for 24 h. Then, the cells were fixed with 4% paraformaldehyde, washed by PBS, and blocked with 10% goat serum for 30 min. Cells were incubated with primary antibodies overnight at 4 °C. Primary antibodies included Pan cytokeratins (pan CK, IHCR2025-6, Merck Millipore, Temecula, CA, USA), VIM (1:200, 10366-1-AP, Proteintech, Wuhan, China), FSP-1 (1:200, ab124805, Abcam, Shanghai, China), FAP (1:200, ab53066, Abcam, Shanghai, China), α-SMA (1:100, ZM-0003, ZSGB-BIO, Beijing, China). Next day, cells were incubated with DyLight 594 conjugated goat anti-rabbit IgG, DyLight 488 conjugated goat anti-mouse IgG, or DyLight 488 conjugated donkey anti-goat IgG (Abbkine, Wuhan, China) at a dilution of 1:100, at room temperature for 30 min. Nuclei were counterstained with diamidino-2-phenylindole (DAPI). Images were recorded using an inverted fluorescent microscope (Olympus IX71, Tokyo, Japan).
Wound-healing assay
The lateral migration ability of cells was examined by a wound-healing assay. Cells in FBS-free medium were seeded into the two square wells (4 × 105 cells/well) of a polydimethylsiloxane (PDMS) membrane that was bound to the bottom of a small culture dish. The area of each well was 1 cm2 and the distance between the two wells was 1 mm. Cells reached confluency in the wells after 24 h. The PDMS membrane was removed and a wound of 1 mm length was formed. Then the cells were treated with CAF-SA CM for 24 h. Serum-free DMEM/F12 was used as a control. The wound area was recorded using an inverted microscope and calculated using Image-Pro Plus 6.0 (Rockville, MD, USA).
Cell invasion assay using Transwell® plates and a microfluidic model
Transwell® invasion assay was performed by coating 1:8 diluted Matrigel™ (Corning, Tewksbury, MA, USA) on the porous membrane in the upper chambers. SACC-83 and SACC-LM cells were seeded into the upper Transwell chambers (1 × 104 cells/chamber). The CAF-SA CM containing 0.5% FBS was added into the lower chamber to stimulate cells invasion. DMEM/F12 containing 0.5% FBS was used as a control. After incubating for 72 h at 37 °C, the medium in the chambers was removed and washed with PBS. The cells adhering to the upper surface of the porous membrane were removed using a cotton applicator. The cells on the lower side of the porous membrane were fixed in 4% paraformaldehyde, stained with 1% methylrosa (Solarbio, Beijing, China), and counted under an inverted microscope.
Cell invasion was further analyzed using a microfluidic model. Microfluidic operation was described in a previous article (Li et al. Citation2016). CAF-SA CM with 0.5% FBS was used as a stimulator. DMEM/F12 containing 0.5% FBS was used as a control. Phalloidin (Thermo, Waltham, MA, USA) was used to recognize cellular morphology. The images were recorded by an inverted microscope.
Clonogenic assay
SACC-LM and SACC-83 were seeded into a six-well plate (500 cells/well). The CAF-SA CM with 10% FBS was added into the wells. DMEM/F12 containing 10% FBS was used as a control. After 5 days, cell culture medium was removed. Tumor colonies were rinsed with PBS, fixed with 4% paraformaldehyde, and stained with 0.1% crystal violet. Tumor colonies were recorded by an inverted microscope and counted using Image-Pro Plus 6.0 (Rockville, MD, USA).
HUVEC tube formation assay
HUVEC cells were used in tube formation assay. Cells (2 × 104 cells/well) were resuspended with CAF-SA CM and seeded in the wells of a 96-well plate pre-coated with Matrigel. FBS-free DMEM/F12 medium was used as a negative control and DMEM/F12 containing 10% FBS was used as a positive control. After 12 h, tube-like structures were observed and recorded by an inverted microscope.
Subcutaneous xenograft model
Animal experiments, transportation, and care were conducted in compliance with the relevant laws and the guidelines issued by the Ethical Committee of Dalian Medical University. BALB/c-nude mice (male, 18–20 g) were purchased from Institute of Genome Engineered Animal Models for Human Disease, Dalian Medical University, Dalian, China. Nude mice were divided into two groups according to the transplanted cells: the SACC-LM group (2.25 × 106 cells/mouse) and the SACC-LM with CAF-SA group (2.0 × 106 SACC-LM +2.5 × 105 CAF-SA). Cells were transplanted into the subcutaneous space on the back. Tumor size was measured every day. After 4 weeks, mice were sacrificed by 20% urethane and xenografts were collected and weighed. Tumor tissues were fixed with 10% formalin and embedded in paraffin. Tissue sections with 4 μm thickness were prepared and immunohistochemically stained with Ki-67 antibody (DAKO, Santa Clara, CA, USA) to assess cell proliferation ability. Immunohistochemical staining method was described previously (Guan et al. Citation2015).
Statistical analysis
Statistical analysis was carried out using the SPSS System 13.0 for Windows (Armonk, NY, USA). Nonparametric test was used to compare the statistical significance of differences. A p < .05 was considered to be statistically significant.
Results
Establishment of a CAF cell line
As shown in , a CAF cell line was established from a single primary CAF cell derived from a case of SACC. CAF-SA showed typical morphologic features of CAF with long processes. STR-profiling analysis detected multiple alleles in primary CAF and CAF-SA (). A 95% DNA match between primary CAF and CAF-SA was found, indicating that both cells were derived from the same patient.
Figure 1. Establishment of CAF-SA. (A) Left: A single cell in a well (white arrows). Middle: Expanded cells. Right: Subcultured CAF-SA. Scale bar =500 μm. (B) The biomarker expression of CAF-SA. Cell were negative for Pan CK and α-SMA and positive for VIM, FSP-1 and FAP. Scale bar =50 μm.
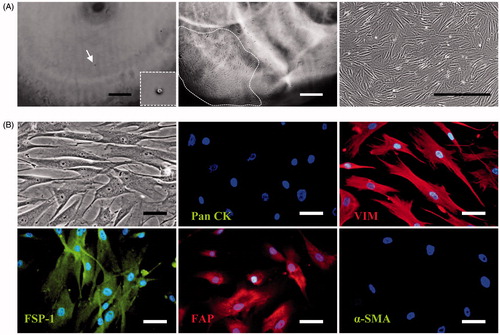
Table 1. STR profiling of CAF-A1 and CAF-SA.
We examined the expression of typical CAF biomarkers in CAF-SA, such as Pan CK, VIM, FSP-1, FAP, and α-SMA (). It was found that they were negative for Pan CK and α-SMA and positive for VIM, FSP-1 and FAP. It suggests that CAF-SA expressed the biomarkers of mesenchymal cells, but not epithelial cells.
CAF-SA promotes the migration and invasion of SACC cells
To investigate whether CAF-SA can promote SACC cell migration, wound-healing assay was performed. As shown in F-SA CM significantly promoted the migration rates of both SACC-83 and SACC-LM cells, compared to controls.
Figure 2. CAF-SA promoted the migration and invasion of SACC cells. (A,B) Wound-healing assay. CAF-SA-CM promoted SACC-83 (A) and SACC-LM (B) migration significantly more than the control medium. (*p < .05. Scale bar = 200 μm) (C) SACC invasion assay using Transwell plates. CAF-SA-CM promoted SACC-83 and SACC-LM invasion significantly more than the control medium. (**p < .01. Scale bar = 200 μm). (D) SACC invasion assay using a microfluidic model. CAF-SA-CM promoted SACC-83 and SACC-LM invasion significantly more than the control medium. (**p < .01. Scale bar = 100 μm).
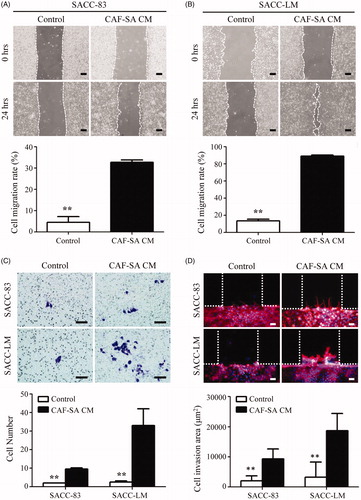
The effects of CAF-SA on tumor cell invasion were investigated by both Transwell® and microfluidic model assays. Transwell® assay showed that CAF-SA CM enhanced the invasions abilities of both SACC-83 and SACC-LM significantly (). On the microfluidic model, both SACC-83 and SACC-LM invaded into the Matrigel channel stimulated by CAF-SA CM. By contrast, both SACC-83 and SACC-LM cells cultured in the control medium rarely invaded into the Matrigel channel. The invasion areas of both tumor cells increased significantly by CAF-SA CM ().
CAF-SA promoted SACC proliferation and tube formation in vitro
It was known that CAFs support tumor growth in vivo by both promoting tumor cell proliferation (Gao et al. Citation2013; Luo et al. Citation2014) and stimulating angiogenesis (Zhang et al. Citation2011). Thus, we examined the effect of CAF-SA on the proliferation of SACC and angiogenesis of HUVEC. The clonogenic assay revealed that CAF-SA CM greatly promoted the proliferation of both SACC-83 and SACC-LM significantly ().
Figure 3. Clonogenic assay and tube formation assay. (A) Clonogenic assay. Representative images of colonies stained with crystal violet. (B) Quantification of clonogenic assay. CAF-SA-CM promoted SACC-83 and SACC-LM colony formation significantly more than the control medium. (*p < .05, **p < .01). (C) Tube formation assay. CAF-SA-CM promoted tube formation greatly. Scale bar = 100 μm.
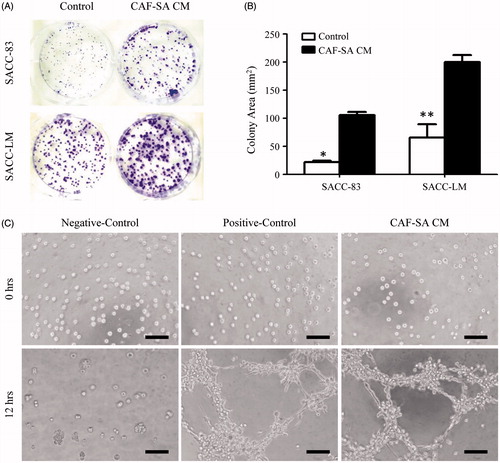
Angiogenesis is the indispensable way to provide nutrients and gas exchange for tumor (Bergers and Benjamin Citation2003; Potente et al. Citation2011). In order to study the role of CAF-SA on angiogenesis, tube formation assay was performed. Typical tube-like structures were detected in the CAF-SA CM group, but not in the control group ().
CAF-SA promotes SACC growth in the mouse model
To further investigate the effects of CAF-SA on tumor growth in vivo, SACC-LM cells with or without CAF-SA were subcutaneous transplanted in nude mice. Xenografts were collected and photographed (). Average tumor volumes were compared between SACC-LM and SACC-LM + CAF-SA groups. It was found that mice transplanted with tumor and CAF-SA cells commonly had a greater volume than that transplanted with tumour solely (). More Ki-67 positive cells were observed in the xenografts with both SACC-LM and CAF-SA than those with SACC-LM solely ().
Figure 4. CAF-SA promotes SACC growth in nude mouse models. (A) Photos of SACC-LM xenografts with or without CAF-SA. (B) Tumor volume analysis. CAF-SA-CM promoted SACC-LM growth significantly at Week 4, compared to controls (n = 5, two transplanted sites/nude mouse, *p < .05). (C) Typical xenografts of SACC-LM transplanted with or without CAF-SA after Ki67 immunostaining. Scale bar = 50 μm. (D) Quantification of Ki-67 staining. Positive cells significantly increased in the SACC-LM + CAF-SA group, compared to the SACC-LM group. (**p < .01).
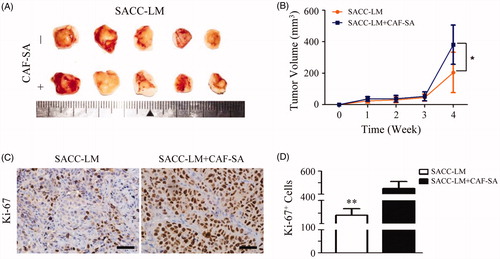
Discussion
In this study, we established a CAF cell line (CAF-SA) from a case of human SACC. CAF-SA expressed the typical CAF biomarkers. In addition, CAF-SA enhanced the migration and invasion abilities of SACC cells and the angiogenic ability of endothelial cells in vitro. CAF-SA promoted SACC growth in the xenograft mouse models.
It has been reported that CAFs were found in SACC tumor stroma and participated in the migration and invasion of SACC cells through variable soluble factors (Liu et al. Citation2010, Citation2012). In all the previous studies, primary CAF cells were used. No CAF cell line derived from SACC was reported. A cell line is a homogeneous cell population. They are widely used in basic scientific research. Compared to the primary cells, a cell line has many advantages, such as uniform cellular morphology, stable proliferation and biologic characteristics (Shin et al. Citation2001). To establish a stable cell line, various methods have been used, such as chemical methods (mutagenesis, viral transformation, genetic changes) (Kokubu et al. Citation2015) and physical dilution method (Pandrangi et al. Citation2014). In the study, we used the physical dilution method to establish CAF-SA. The cell line has specific characteristics of CAF according to their biomarker expression.
CAFs are one of the most abundant and important stromal cells in tumor microenvironment. The most important defining feature of CAFs compared to normal fibroblasts is their capacity to stimulate cancer invasion and metastasis. They regulate the growth, invasion and metastasis of tumor cells and stimulate angiogenesis, recruiting bone marrow-derived cells and immunocytes (Shiga et al. Citation2015). In liver cancer, CAF can promote the proliferation of tumor cells through secreting hepatocyte growth factor in vivo and in vitro (Jia et al. Citation2013). CAFs also secrete paracrine factors to promote the migration and invasion of tumor cells. For instance, Galectin-1 is involved in the process of fibroblast transforming into CAF and promotes the migration of glioma cells (Trylcova et al. Citation2015). In pancreatic ductal adenocarcinoma, CAF-derived IL-6 was induced by retinoic acid, which was related to epithelial–mesenchymal transition of tumor cells (Guan et al. Citation2014). In the study of oral squamous cell carcinoma, the expression of Notch3 in CAF was significantly correlated with the microvessel density in tumor stroma, suggesting that the Notch signal in CAF associated with tumor angiogenesis and the possibility of Notch3-targeted molecular therapy in this tumor (Kayamori et al. Citation2016). In this study, we confirmed that CAF-SA has the defining features of CAF by promoting SACC invasion and growth in vitro and in vivo.
We applied a microfluidic model to investigate cell invasion. Recently, microfluidic chip has been proved to be ideal platform in biologic and medical research. It is also known as lab-on-a-chip (Bhatia and Ingber Citation2014). Microfluidic models have many advantages, such as requiring less time, reduced sample consumption, and low cost (Huh et al. Citation2011). Compared with the Transwell® invasion assay, the microfluidic model used in this study enable to observe the cell invasion processes in real time because the model is transparent and cells move horizontally.
In summary, we established a CAF cell line (CAF-SA) derived from human SACC. Both the characteristics of CAF-SA and cellular communications between CAF-SA and tumor cells were investigated. We demonstrated that CAF-SA did not only express typical CAF biomarkers, but also showed the most important CAF defining features of stimulating cancer progression. We hope that CAF-SA will promote the study of cellular communications between tumor and stromal cells in SACC.
Disclosure statement
No potential conflict of interest was reported by the authors.
Additional information
Funding
References
- Albrengues J, Meneguzzi G, Gaggioli C. 2014. Carcinoma-associated fibroblasts in cancer: the great escape. Med Sci. 30:391–397.
- Attieh Y, Vignjevic DM. 2016. The hallmarks of CAFs in cancer invasion. Eur J Cell Biol. 95:493–502.
- Bergers G, Benjamin LE. 2003. Tumorigenesis and the angiogenic switch. Nat Rev Cancer. 3:401–410.
- Bhatia SN, Ingber DE. 2014. Microfluidic organs-on-chips. Nat Biotechnol. 32:760–772.
- Bruzgielewicz A, Osuch-Wojcikiewicz E, Majszyk D, Checinski P, Nyckowska J, Zawadzka B, Skowronska-Gardas A, Niemczyk K. 2011. Adenoid cystic carcinoma of the head and neck – a 10 years experience. Otolaryngol Pol. 65:6–11.
- Dantas AN, Morais EF, Macedo RA, Tinoco JM, Morais Mde L. 2015. Clinicopathological characteristics and perineural invasion in adenoid cystic carcinoma: a systematic review. Braz J Otorhinolaryngol. 81:329–335.
- Gao M, Hao Y, Huang MX, Ma DQ, Luo HY, Gao Y, Peng X, Yu GY. 2013. Clinicopathological study of distant metastases of salivary adenoid cystic carcinoma. Int J Oral Maxillofac Surg. 42:923–928.
- Gao MQ, Kim BG, Kang S, Choi YP, Yoon JH, Cho NH. 2013. Human breast cancer-associated fibroblasts enhance cancer cell proliferation through increased TGF-α cleavage by ADAM17. Cancer Lett. 336:240–246.
- Guan H, Tan J, Zhang F, Gao L, Bai L, Qi D, Dong H, Zhu L, Li X, Liu T. 2015. Myofibroblasts from salivary gland adenoid cystic carcinomas promote cancer invasion by expressing MMP2 and CXCL12. Histopathology. 66:781–790.
- Guan J, Zhang H, Wen Z, Gu Y, Cheng Y, Sun Y, Zhang T, Jia C, Lu Z, Chen J. 2014. Retinoic acid inhibits pancreatic cancer cell migration and EMT through the downregulation of IL-6 in cancer associated fibroblast cells. Cancer Lett. 345:132–139.
- Huh D, Hamilton GA, Ingber DE. 2011. From 3D cell culture to organs-on-chips. Trends Cell Biol. 21:745–754.
- Jia CC, Wang TT, Liu W, Fu BS, Hua X, Wang GY, Li TJ, Li X, Wu XY, Tai Y, et al. 2013. Cancer-associated fibroblasts from hepatocellular carcinoma promote malignant cell proliferation by HGF secretion. PLoS One. 8:e63243.
- Kayamori K, Katsube K, Sakamoto K, Ohyama Y, Hirai H, Yukimori A, Ohata Y, Akashi T, Saitoh M, Harada K, et al. 2016. NOTCH3 is induced in cancer-associated fibroblasts and promotes angiogenesis in oral squamous cell carcinoma. PLoS One. 11:e0154112.
- Kokubu Y, Asashima M, Kurisaki A. 2015. Establishment and culture optimization of a new type of pituitary immortalized cell line. Biochem Biophys Res Commun. 463:1218–1224.
- Li J, Jia Z, Kong J, Zhang F, Fang S, Li X, Li W, Yang X, Luo Y, Lin B, et al. 2016. Carcinoma-associated fibroblasts lead the invasion of salivary gland adenoid cystic carcinoma cells by creating an invasive track. PLoS One. 11:e0150247.
- Liotta LA, Kohn EC. 2001. The microenvironment of the tumour-host interface. Nature. 411:375–379.
- Liu T, Lin B, Qin J. 2010. Carcinoma-associated fibroblasts promoted tumor spheroid invasion on a microfluidic 3D co-culture device. Lab Chip. 10:1671–1677.
- Liu W, Zhu E, Wang R, Wang L, Liu T. 2012. CXCL12 G801A polymorphism is associated with an increased risk of benign salivary gland tumors in the Chinese population. Med Oncol. 29:677–681.
- Luo H, Yang G, Yu T, Luo S, Wu C, Sun Y, Liu M, Tu G. 2014. GPER-mediated proliferation and estradiol production in breast cancer-associated fibroblasts. Endocr Relat Cancer. 21:355–369.
- Mizrachi A, Bachar G, Unger Y, Hilly O, Fliss DM, Shpitzer T. 2017. Submandibular salivary gland tumors: Clinical course and outcome of a 20-year multicenter study. Ear Nose Throat J. 96:E17–E20.
- Olumi AF, Grossfeld GD, Hayward SW, Carroll PR, Tlsty TD, Cunha GR. 1999. Carcinoma-associated fibroblasts direct tumor progression of initiated human prostatic epithelium. Breast Cancer Res. 59:5002–5011.
- Pandrangi SL, Raju Bagadi SA, Sinha NK, Kumar M, Dada R, Lakhanpal M, Soni A, Malvia S, Simon S, Chintamani C, et al. 2014. Establishment and characterization of two primary breast cancer cell lines from young Indian breast cancer patients: mutation analysis. Cancer Cell Int. 14:14.
- Potente M, Gerhardt H, Carmeliet P. 2011. Basic and therapeutic aspects of angiogenesis. Cell. 146:873–887.
- Shiga K, Hara M, Nagasaki T, Sato T, Takahashi H, Takeyama H. 2015. Cancer-associated fibroblasts: their characteristics and their roles in tumor growth. Cancers. 7:2443–2458.
- Shin KH, Choe G, Park YJ, Jang JH, Jung HW, Park JG. 2001. Establishment and characterization of nine human brain tumor cell lines. In Vitro Cell Dev Biol Anim. 37:625–628.
- Trylcova J, Busek P, Smetana K, Jr., Balaziova E, Dvorankova B, Mifkova A, Sedo A. 2015. Effect of cancer-associated fibroblasts on the migration of glioma cells in vitro. Tumor Biol. 36:5873–5879.
- Wang YL, Zhu YX, Chen TZ, Wang Y, Sun GH, Zhang L, Huang CP, Wang ZY, Shen Q, Li DS, et al. 2012. Clinicopathologic study of 1176 salivary gland tumors in a Chinese population: experience of one cancer center 1997–2007. Acta Otolaryngol. 132:879–886.
- Zhang Y, Tang H, Cai J, Zhang T, Guo J, Feng D, Wang Z. 2011. Ovarian cancer-associated fibroblasts contribute to epithelial ovarian carcinoma metastasis by promoting angiogenesis, lymphangiogenesis and tumor cell invasion. Cancer Lett. 303:47–55.