ABSTRACT
Printing devices are known to emit chemicals into the indoor atmosphere. Understanding factors that influence release of chemical contaminants from printers is necessary to develop effective exposure assessment and control strategies. In this study, a desktop fused deposition modeling (FDM) 3-dimensional (3-D) printer using acrylonitrile butadiene styrene (ABS) or polylactic acid (PLA) filaments and two monochrome laser printers were evaluated in a 0.5 m3 chamber. During printing, chamber air was monitored for vapors using a real-time photoionization detector (results expressed as isobutylene equivalents) to measure total volatile organic compound (TVOC) concentrations, evacuated canisters to identify specific VOCs by off-line gas chromatography-mass spectrometry (GC-MS) analysis, and liquid bubblers to identify carbonyl compounds by GC-MS. Airborne particles were collected on filters for off-line analysis using scanning electron microscopy with an energy dispersive x-ray detector to identify elemental constituents. For 3-D printing, TVOC emission rates were influenced by a printer malfunction, filament type, and to a lesser extent, by filament color; however, rates were not influenced by the number of printer nozzles used or the manufacturer's provided cover. TVOC emission rates were significantly lower for the 3-D printer (49–3552 µg h−1) compared to the laser printers (5782–7735 µg h−1). A total of 14 VOCs were identified during 3-D printing that were not present during laser printing. 3-D printed objects continued to off-gas styrene, indicating potential for continued exposure after the print job is completed. Carbonyl reaction products were likely formed from emissions of the 3-D printer, including 4-oxopentanal. Ultrafine particles generated by the 3-D printer using ABS and a laser printer contained chromium. Consideration of the factors that influenced the release of chemical contaminants (including known and suspected asthmagens such as styrene and 4-oxopentanal) from a FDM 3-D printer should be made when designing exposure assessment and control strategies.
Introduction
Additive manufacturing (AM) is the process of joining materials using layer-upon-layer methodologies to make objects.Citation[1] Although AM technologies have been used for decades in industrial settings, inexpensive desktop fused deposition modeling (FDM) 3-dimensional (3-D) printers are becoming common in offices, libraries, schools, universities, and the home. With increased use of desktop and small-scale 3-D printers in non-industrial settings comes the concern for user health and safety.Citation[2]
In FDM printing, a solid thermoplastic filament is forced through a heated computer-controlled nozzle which melts the filament and deposits successive layers of plastic on a baseplate to form a solid 3-D shape. Thermoplastics are composed of a polymer that is mixed with a complex blend of materials known collectively as additives. As thermoplastics are heated, they undergo physical and chemical changes which can result in emission of gases and particulates.Citation[3–5]
Exposures to volatile organic compounds (VOCs) in indoor environments is of concern for workplaces, public venues, and private homes. Some VOCs are respiratory and mucous membrane irritantsCitation[2,6] or allergic asthmagens.Citation[7,8] Ozone is a lung irritant and a reactive gas that may alter indoor air chemistry by interacting with unsaturated VOCs to form secondary organic aerosols and reactive products such as carbonyl compounds.Citation[9–11] Carbonyl compounds such as aldehydes and ketones are associated with development of asthma.Citation[12,13]
To properly evaluate exposures from FDM 3-D printers and design control technologies, there needs to be an understanding of factors that influence emissions. summarizes several factors and their influence on emissions from FDM 3-D printers.Citation[14–19] Relevant factors include those of the printer itself and the properties of the thermoplastic filaments. Generally speaking, most emphasis has been placed on particle emissions; however, many of these same factors could also influence chemical emissions but they are not yet fully understood. Hence, the purpose of this study was to better understand factors that influence generation of airborne chemical contaminants from a desktop FDM 3-D printer. Specifically, we investigated printer- (number of nozzles, malfunction, controls) and consumable-related (filament type, color) factors to address existing knowledge gaps.
Table 1. Factors influencing emissions from desktop fused deposition modeling 3-D printers.
Materials and methods
Concentrations of airborne contaminants released from the printers were evaluated in a 0.5 m3 stainless steel chamber. A two-piece high efficiency particulate filter and activated carbon filter was attached to the chamber inlet to remove particles and organic chemicals from the room air prior to entering the chamber. This chamber has multiple sampling ports on the top connected to stainless steel sampling tubes that extend into the chamber to collect air from the center of the chamber. Conductive carbon tubing and stainless steel tubing without sharp bends were used for sampling; tubing lengths were less than 1 m to minimize particle line losses.Citation[20] The inlets of the sampling probes were placed approximately 10 cm from the printer for all trials. An upward air flow was generated through a perforated floor in the chamber, which in studies with titanium dioxide aerosols, when sampling at multiple locations in the chamber, reduced areas of stagnant air in the chamber and yielded relatively uniform contaminant concentrations, thereby minimizing bias relative to sampling positions.Citation[21] A vacuum leak test demonstrated that the leak rate was 0.05 L min−1 or ∼0.2% of the 25 L min−1 air flow through the chamber. The total sampling air flow rate of all instruments during sampling was 25 L min−1 which provided a chamber air change rate of 3.0 h−1 which is recommended for studies of office equipment.Citation[22] A carbon dioxide air exchange rate test was not performed because the chamber has negligible leakage, therefore the air exchange rate is equivalent to the air sampling flow rate. For more details on the experimental setup see Yi et al.Citation[19]
Concentrations of airborne contaminants were measured while printing a hair comb (100 mm × 33 mm × 3 mm) with a desktop FDM 3-D printer (MakerBot 2x, MakerBot, Brooklyn, NY) using acrylonitrile butadiene styrene (ABS) or polylactic acid (PLA) filaments. All tests were performed with the manufacturer-provided cover on the printer except where noted. Four colors of ABS filament (natural, blue, red, and black) and four colors of PLA filament (true red, army green, ocean blue, and transparent blue) were evaluated—see Figure S1 in the Supporting Information file for images of these colors. The time to print each comb was about 14 min. Only one 3-D printer nozzle was needed to print a hair comb. To evaluate the influence of using two printer nozzles, we printed a traffic cone (40 mm × 40 mm × 50 mm) using red and blue ABS, which took about 34 min. The manufacturer's recommended printer settings for ABS were: extruder temperature = 230°C and baseplate temperature = 110°C; while for PLA, extruder temperature = 215°C and baseplate heater was off. For comparison, we measured chamber air while printing with previously used laser printers (Laserjet P2055dn and Laserjet HP2600, Hewlett Packard, Palo Alto, CA). New manufacturer-specified toner cartridges were installed in each device prior to printing a standard 5% coverage standard patternCitation[22,23] on 216 mm × 279 mm white paper having weight 75 g/m2 (Office Depot, Boca Raton, FL). The print durations were 0.5–2.4 min for 10 and 80 pages, respectively, with HP2055dn and 1.5 and 9.7 min for 10 and 80 pages, respectively, with HP2600.
Conditions inside the chamber (temperature, humidity, printer to chamber volume ratio, etc.) and our testing procedure followed RAL-UZ-171: Test Method for the Determination of Emissions from Hardcopy Devices.Citation[23] For testing the 3-D printer, the start of the operating phase was defined as the time the print command was sent to the 3-D printer. Air inside the chamber was monitored during the pre-operating phase (∼1 hr), printing phase, and post-operating phase using a suite of complementary real-time and time-integrated sampling techniques. During the pre-operating phase, the chamber was flushed with filtered air while the printer was on but not printing. During this phase, for the 3-D printer only, the nozzle and/or baseplate were heated to their set temperatures (no thermoplastic was extruded). No appreciable rise in VOC or particle concentration occurred in the pre-operating phase during the nozzle and baseplate heating. For all tests, temperature inside the chamber during printing was 21.0 ± 1.0°C and the relative humidity was 51.4 ± 4.4%. The post-operating phase began when the print job ended (all printers on; 3-D baseplate and nozzle cooling) and lasted for three air changes.
Chamber air monitoring
Total VOC (TVOC) concentration in the chamber was measured using a real-time photo-ionization detector with 10.6 eV ultraviolet discharge lamp (Model 3000 ppbRAE, RAE Systems, San Jose, CA) during all phases to calculate unit specific emission rates (SERu). This instrument was factory calibrated using isobutylene and span checked with isobutylene prior to use and is capable of measuring down to 1 ppb or 2.3 µg m−3 isobutylene equivalent. Ozone concentration was monitored using a real-time gas sensitive semiconductor sensor (Model S500, Ozone Solutions, Hull, IA) during all phases. The limit of detection for this monitor is 0.5 µg m−3. For determination of TVOC and ozone SERu, two replicate 3-D printer tests were performed and one test was performed for each laser printer.
Samples for specific VOCs were collected using whole-air 6 L Silonite®-coated canisters (Entech Instruments, Inc., Simi Valley, CA) followed by off-line analysis by gas chromatography-mass spectrometry (GC-MS) as described in the Supporting Information file. Canister samples are suitable for analyzing a range of VOCs (e.g., from alcohols to terpenes) at low levels in indoor atmospheres.Citation[24] Two canister samples were collected during each printing test, one during the pre-operating phase and the other at the mid-point of the printing phase. Collection took a period of about 1–2 min per sample. Two to five replicate tests (covering both the pre- and post-operating phases) were performed for the 3-D and laser printers. Samples for gas-phase carbonyls were obtained by pulling air from the test chamber using a calibrated (Model 4146, TSI Inc., Shorview, MN) pump (URG 3000-02Q, Chapel Hill, NC) at 4.0 L min−1 into 25 mL of deionized water in a 60 mL Teflon bubbler (Savillex, Eden Prairie, MN) during the pre-operating phase and again during the printing and post-operating phases. Samples were derivatized and analyzed using GC-MS (see Supporting Information file). Bubbler sampling followed by derivatization is a well-established method for measurement of low levels of carbonyls in indoor atmospheres.Citation[25–27] For identification of carbonyls, two tests (covering both the pre- and post-operating phases) were performed for the 3-D and laser printers.
Aerosol particles were collected on 47-mm track-etched polycarbonate filters with 2 µm pore size using a stainless-steel inline filter holder and pre-calibrated sampling pump (GilAir, Sensidyne, St. Petersburg, FL) with flow rate set to 3 L min−1 during the pre-operating phase and again during the printing phase. Collection efficiency of this type of filter ranges from 20–94% for 0.10–1 µm size particles.Citation[28] A section was cut from each filter, sputter coated with gold/palladium to enhance conductivity and imaged using field emission scanning electron microscopy (S-4800, Hitachi, Tokyo, Japan) with energy dispersive x-ray analysis (EDX, Quantax, Bruker Scientific Instruments, Berlin, Germany) to identify elemental constituents. The balance of the 25 L min−1 chamber air flow rate was accounted for by real-time particle monitors (see Supporting Information file).
Emissions from stock and printed thermoplastics
To evaluate potential for off-gassing, stock natural color ABS filament and printed 3-D combs made of natural color ABS were placed in a 375-mL glass chamber with an air exchange rate of 0.96 h−1. The glass chamber was not allowed to equilibrate. Two 450-mL fused-silica lined canisters equipped with capillary flow controllers were used to sample the chamber effluent at 0.48 mL min−1 for 6 hr. Samples were pressurized to 1.5 times atmospheric pressure and analyzed using a preconcentrator/GC-MS system (see Supporting Information file). Emission rates were calculated from the measured concentration, air exchange rate, and volume of the chamber.
Data analysis
Unit specific emission rates (SERu) were calculated from the TVOC and ozone data for the 3-D and laser printers in accordance with RAL-UZ-171Citation[23] (see Supporting Information for details). Comparison of SERu between 3-D and laser printers is considered appropriate because: (1) both technologies use a thermoplastic feedstock (filament or toner powder); (2) both devices are used in indoor work spaces; (3) values of SERu are normalized to time which accounted for differences in printing duration among devices; and (4) in the absence of real-world data, chamber measurements, and comparison of emissions based on modeling is the method of choice for investigating factors that may influence emissions. The identities of airborne VOCs in the chamber were determined using whole-air canister samplers from 2–5 replicates tests.
One-way analysis of variance (ANOVA) models were fit in JMP (version 11.2.0, SAS Institute, Inc., Cary, NC) to investigate the impact of the fixed effects of color and filament on 3-D printer TVOC SERu and specific VOC concentration results. Tukey's test option was specified for multiple comparisons among colors within a filament type and Student's t-test was used to compare the effect of color between filament types. ANOVA F-statistics were used to note the overall differences in the means of colors within the filament types while Tukey's test was used to identify specific paired differences. For all comparisons, the significance level was set at 0.05. Note that emission rates were not calculated for individual VOCs from the canister samples because these results are only concentration measurements at a point in time during the print phase.
Results and discussion
TVOC emission rates
For the 3-D printer, the calculated TVOC SERu values were consistently higher for ABS filament compared to PLA (see ). Azimi et al. also reported higher TVOC SERu values for ABS compared to PLA.Citation[14] However, it is important to note that while the trend of SERu for ABS being higher than PLA is consistent between these studies, the absolute SERu values cannot be compared because we used a real-time monitor to measure TVOC concentration and Azimi et al. summed the concentrations of individual VOCs they quantified by GC-MS. In contrast to these results, Steinle, who calculated TVOC emission rates from individual GC-MS data, reported that SERu was higher for PLA compared to ABS.Citation[17] Looking at similar colors of these filament types, the calculated TVOC SERu was significantly higher (p < 0.05) for blue ABS (2385 ± 82 µg h−1) compared to transparent blue PLA (131 ± 37 µg h−1); TVOC levels were below the limit of detection for ocean blue PLA. SERu for red ABS (2383 ± 357 µg h−1) was significantly higher compared to true red PLA (49 µg h−1); p < 0.05.
Table 2. Average ± standard deviation of TVOC SERu values for 3-D and laser printers.
Within a given type of filament, color had a minor influence on TVOC SERu for ABS only, i.e., SERu for natural color ABS was significantly higher than black ABS (p < 0.05). There were no statistical difference among PLA filament colors. Kim et al. used the same type of real-time TVOC PID monitor as in our study and reported that levels were non-detectable when printing with two different PLA filaments.Citation[15] In our study, some tests with ocean blue, army green and true red PLA yielded TVOC concentrations below the instrument limit of detection. Interestingly, in our study the laser printers that consumed powdered toner had significantly higher TVOC SERu values than the FDM 3-D printer. However, it is important to note that presently there is insufficient toxicological data available to compare 3-D and laser printers on an absolute scale, i.e., higher emission rates by one device does not necessarily imply greater hazard.
To evaluate whether printing with two nozzles vs. one nozzle influenced emissions, real-time TVOC data were used to calculate yield, which accounts for differences in the mass of filament extruded during these print jobs. When printing with two nozzles to make a traffic cone, the average yield was 328 ± 41 µg TVOC g−1 printed filament. Average yield values from printing with one nozzle to make a hair comb were: 229 ± 64 µg TVOC g−1 printed filament (red comb), and 383 ± 16 µg TVOC g−1 printed filament (blue comb). Hence, the number of nozzles used for 3-D printing these objects with ABS filaments did not appear to influence TVOC emissions.
As summarized in , use of the manufacturer provided cover for the 3-D printer did not reduce TVOC SERu when we printed with natural color ABS; SERu were 3430 µg h−1 (cover off) vs. 3552 ± 549 µg h−1 (cover on). During a subsequent 3-D print job using natural color ABS with the cover off, there was a malfunction (object did not fully adhere to baseplate) and we continued to sample the chamber air. During this malfunction, the calculated SERu was 6454 µg h−1, which appears higher than when the printer was operating normally for the same type and color of filament. The printer malfunction was a random event which makes it difficult to reproduce to collect additional data and some caution is needed in generalizing our observation from this single event. Among all print jobs, the TVOC SERu followed the rank order: HP2600 (80 pages) ≈ HP2055dn (80 pages) > 3-D printing with ABS or PLA.
Ozone emission rates
is plots of ozone concentration in the chamber for the 3-D and laser printers; for simplicity, only one representative plot is shown per 3-D printer filament type. For natural color ABS, the background ozone concentration in the chamber was steady initially but began to decrease until reaching a minimum during the printing phase before slowly returning to background (). The decrease in ozone concentration was more pronounced when the cover of the 3-D printer was taken off. The time at which the ozone concentration began to decrease corresponded to the start of the 7-min period during which the baseplate was heated from ambient to 110°C. In contrast, for true red PLA the background ozone concentration was relatively constant throughout the pre-operating (baseplate heater off) and printing phases ().
Figure 1. Ozone concentrations for (a) 3-D printer using natural ABS with the printer cover on and off, (b) 3-D printer using true red PLA, (c) HP2055dn laser printer using monochrome toner (80 pages), and (d) HP 2600 laser printer using monochrome (80 pages) toner. Numbers for each vertical line denote: 0 = begin baseplate heating (ABS only), 1 = begin print job, and 2 = end print job.
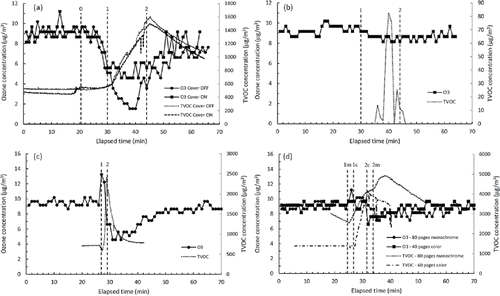
Both laser printers generated ozone ( and ). For the HP2055dn, when the print job was initiated (print command sent to device) the SERu for ozone was 0.5 µg hr−1 but began to decay rapidly to below background during the print job (80 pages) and slowly recovered to background thereafter. For the HP2600 printer, the rise in ozone concentration (SERu = 0.2 µg hr−1) corresponded to the output of the first page but decayed during the remainder of the 80 page print job to below background. Calculated SERu for ozone from the laser printers were quite low compared to previously published studies and may reflect improvements in technology relative to older studies.Citation[10,11,29]
Identification of carbonyl compounds
For both 3-D and laser printing, the organic compounds generated during operation may transform in the presence of ozone. Ozone can add to the carbon-carbon double bonds of airborne compounds (such as limonene) resulting in oxygenated species (i.e., aldehydes, ketones, carboxylic acids, etc.).Citation[9–11] These reactions can occur on a few second to few minute timescale which implies that printer generated compounds can be oxidized before they are removed by building air exchange. Several carbonyl compounds were qualitatively identified from samples collected during the print and post-print phases for all the 3-D and laser printers investigated. An example chromatogram for 4-oxopentanal formed during 3-D printing is provided as . As seen, the signal intensity is higher than background during the printing and post-printing phases indicating that 4-oxopentanal was formed by the printing process. An example of a mass spectrum for derivatized 4-oxopentanal is provided as Figure S2 in the Supporting Information. While the generation of these carbonyl compounds are not fully understood, they could be the result of intentional chemical reactions of the printing process and/or unintentional ozonolysis of alkene (carbon-carbon double bond) compounds from the printers (TVOC concentrations increased during all printing jobs as shown in ) or carbonaceous particulate matter. For the 3-D printer using ABS and PLA, regardless of color, we identified 4-oxopentanal, glyoxal, methyl glyoxal, and benzaldehyde. In addition, five other unidentified carbonyl compounds were detected when using ABS filaments. The carbonyl compounds identified during laser printing included: glyoxal, methyl glyoxal, m-tolualdehyde, and 4-oxopentanal. To our knowledge, these results are the first report of 4-oxopentanal being formed as a result of chemicals being released from printing systems. Exposure to 4-oxopentanal could potentially result in respiratory health effects.Citation[12,30–32] Additional work is planned for future investigations to quantify 4-oxopentanal levels to more completely characterize oxidation reactions from printing.
Figure 2. Chromatograms of the three peaks for TBOX-derivatized 4-oxopentanal from samples collected during background-, printing-, and post-printing phases—derivatization of non-symmetric carbonyls using TBOX typically results in multiple chromatographic peaks due to geometric isomers of the oximes.
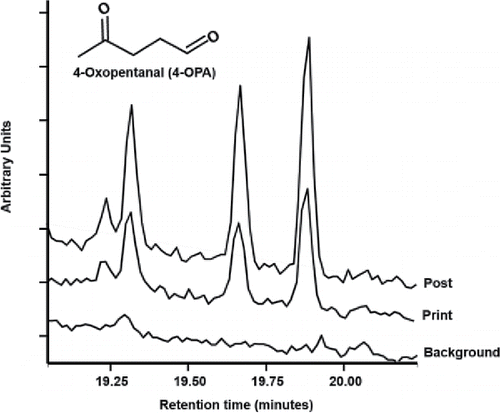
Identification of individual VOCs
summarizes the background (pre-operating phase)-corrected concentrations of individual VOCs detected in chamber air during FDM 3-D printing. Although 2–5 replicate tests (covering both the pre- and post-operating phases) were performed for the 3-D and laser printers, not all VOCs were identified in all samples. As such, when results are presented as an average in the table, the data represent at least two independent canister samples. Up to 13 different VOCs were above our analytical detection limits for ABS compared to a maximum of 9 for PLA. Four VOCs were common to both filament types: acetaldehyde, ethanol, acetone, and isopropyl alcohol. Concentrations of acetaldehyde for blue ABS were significantly higher than both blue PLA filaments and the same was true for red ABS relative to red PLA (p < 0.05). There were no differences in ethanol concentrations between filament types for blue and red colors. Acetone concentrations for blue ABS were higher than for blue PLA filaments (p < 0.05); however, the concentrations for red ABS and true red PLA were similar. Isopropyl alcohol concentrations did not differ between blue ABS and blue PLA filaments though concentrations from red ABS were significantly lower than from true red PLA (p < 0.05). Note that the data presented in are for a point in time during printing and are not the same as the emission rates calculated from the real-time TVOC data. Individual VOCs may be emitted at different times throughout the print process.Citation[10] Hence, depending on when certain VOCs are emitted during printing and the timing of the canister sample collection, the reported concentrations in may or may not reflect the highest concentrations in the chamber during printing, but were recorded by the PID and accounted for in the SERu calculations.
Table 3. Average ± standard deviation of background-corrected concentrations of individual VOCs measured by whole-air sampling with canisters during 3-D printing (µg m−3).
Of the six VOCs measured during 3-D printing with each color of ABS filaments, only acetone concentrations differed significantly (blue was higher compared to natural, red and black; p < 0.05). Seven VOCs were common to all colors of PLA filaments. The concentrations of acetone for true red and army green colors were significantly higher than either blue color (p < 0.05). Concentrations of isopropyl alcohol followed the rank order army green> true red> ocean blue> transparent blue (p < 0.05). Concentrations of acetaldehyde, ethanol, acetonitrile, hexane, and chloroform were similar. Based on the data presented in , filament type appears to have more influence on the identities of VOCs detected in chamber air than does color. According to the manufacturer's safety data sheet for the filaments, ABS is >98% acrylonitrile butadiene styrene co-polymer and < 0.1% styrene whereas PLA is >98% polylactide resin; however, no other specific information on ingredients is provided. Hence, the observed difference in identified VOCs between polymer types likely reflects differences in the basic ABS and PLA ingredients used to make the polymers.
With regard to the use of the manufacturer provided cover, the same VOCs were detected while 3-D printing with natural color ABS whether the cover was on or off. Concentrations of VOCs measured with the cover on and off were generally similar, except for the following which appeared to increase (cover on vs. cover off): isopropyl alcohol (87 vs. 297 µg m−3), ethylbenzene (5 vs. 21 µg m−3), and styrene (250 vs. 396 µg m−3). Hence, the loose-fitting cover provided by the manufacturer did not control vapors generated during printing.
The generation of VOCs from 3-D printers is consistent with the decomposition of thermoplastic filament when it is heated by the extruder nozzle. Most of the chemical compounds detected during operation of the 3-D printer have been identified as pollutants released from various models of laser printers.Citation[33–37] Our data is consistent with reports that 3-D printing with various ABS filaments releases toluene, ethylbenzene, styrene, and acetophenone and that printing with PLA generates low amounts of toluene.Citation[14,15,17] We note that others have identified caprolactam, lactide, decane, cyclohexanol, methyl methacrylate, n-butanol, and other VOCs during 3-D printing with ABS or PLA filamentsCitation[14,17] although these compounds were not observed in our study. There may be several reasons for the observed differences in VOCs identified among studies such as the composition of the polymer filament, printer extrusion temperatures, and sampling methods used by investigators. Future studies would benefit from standardized emissions testing protocols.
summarizes the concentrations of individual VOCs detected during laser printing. Repeat samples were collected for each combination of printer and number of printed pages; however, several VOCs were identified in only one test which precluded statistical comparison of the data. For the HP2055dn printer, concentrations of seven VOCs increased from 10 pages to 80 pages. For the HP2600 printer, concentrations of acetaldehyde, isopropyl alcohol, and d-limonene increased from 10 pages to 80 pages; however, concentrations of toluene and m,p-xylene did not increase with the number of printed pages.
Table 4. Average ± standard deviation of background-corrected concentrations of individual VOCs Measured by Whole-air Sampling with Canisters during Laser Printing (µg m−3).
Comparison of and indicates that 3-D and laser printers generated numerous VOCs during operation, some of which are common to both technologies and others that are unique to one or the other. D-limonene was detected during most laser printing jobs but not with 3-D printing. In the presence of ozone, this compound may form oxidation products that are airway irritants.Citation[9] Acetone was detected in all 3-D printing samples but in only one laser printing sample. Benzene, toluene, ethylbenzene, and xylenes were detected during most laser printing jobs but only detected in a few samples during 3-D printing with PLA filament. Variations in identities of specific VOCs among printing devices are likely due to differences in thermoplastic composition and decomposition temperatures (200–230°C for the 3-D printer, compared to about 170°C for the laser printers) as well as other factors, not all of which completely understood at this time. Combustion, injection molding, extrusion, and recycling of ABS thermoplastics is known to emit benzene, benzaldehyde, ethylbenzene, ethylmethyl benzene, toluene, styrene, acetophenone, and m,p-xylene, o-xylene, and benzaldehyde.Citation[4,38,39] Consistent with the generation of organic chemicals from thermal degradation, we identified all of these compounds in chamber air during printing with ABS filaments. Note that some of the compounds identified in chamber air during 3-D and laser printing are associated with asthma. For example, styrene,Citation[7] and carbonyl compounds including 4-oxopentanal are known or suspected immune-mediated asthmagens.Citation[8,12,13,30–32] Further, ozone is known to transform styrene and unsaturated VOCs such as d-limonene into secondary organic aerosols.Citation[6,11]
Various compounds that were not in our calibration mixture were identified in chamber air during FDM 3-D and/or laser printing (Figure S3). A total of 33 different compounds were identified by spectral matching in at least two samples per tested device. Distinct differences existed between print technologies—14 compounds were identified during 3-D printing but not laser printing.
Elemental composition of airborne particulate
Particles released from the 3-D and laser printers differed in morphology and size (no particles were observed during inspection of filter samples collected during the pre-operating phases). 3-D printer aerosol were generally clusters of nanoscale particles or discrete nanoscale particles (Figures S4 and S5) whereas for laser printers the particles were micron-scale (Figure S6). For ABS, the following elements were detected: Cr, Ni, Si, Cl, Ca, Mg, Na, Al, and S. In general, the relative abundance of Cr was greater than any other element for particles collected during printing with natural, blue, and red ABS colors. Particles generated during 3-D printing with PLA contained Fe. Steinle reported that a nanoscale particle collected during 3-D printing (filament type not specified) contained Fe, though the presence of transition metals was not reported in that study.Citation[17] Particles emitted from laser printers contained Cr and/or S. Previous studies have reported that particles emitted from laser printers using monochrome toner contained all of these same elements identified in 3-D printer particles at levels less than 2%.[Citation34,37,40–43] In those studies, the presence of Ca was attributed to CaCO3 coating on paper while Fe was attributed to iron oxide (used to make toner) and Cr, Na, Si, and S were attributed to pigments and additives.Citation[34,40,41] Given that Fe is used to make powdered toner, its presence in 3-D printer filaments may reflect a basic composition of thermoplastics in general. The reason for the presence of transition metals in 3-D printer emitted particles is unknown (there is no mention of these metals on the safety data sheets), though based on characterization of laser printer emissions, they could be used as pigments and additives.
The focus of the present study was on chemical contaminants generated by FDM 3-D printers; however, many types ultrafine particles (UFP, d<100 nm) can cause strong inflammatory responses and a variety of cardiovascular effects.Citation[44–46] We previously reported on particle emission rates (PER) for this 3-D-printer.Citation[19] For completeness, in the present study we determined PER from the laser printers using the same methods. summarizes the particle sizes and number-based PERs for the laser printers (this study) and the 3-D printer.Citation[19] Emitted particle sizes differed significantly and followed the rank order (from largest to smallest): HP2600 (80 pages) > HP2055dn (80 pages) ≈ all colors of ABS and PLA filaments. The average number-based PER (# min−1) followed the rank order (from highest to lowest): HP2055dn (80 pages) > 3-D printer (all colors of ABS and PLA filaments) > HP2600 (80 pages); differences were significant. He et al. reported PER based on SMPS measurements for a series of laser printers.Citation[29,47] In their first study of 3 monochrome printers, PER ranged from 4 × 107 to 7.6 × 1010 # min−1. In their subsequent more extensive study of 15 monochrome laser printers, all but one printer emitted more than 1010 # min−1 and were categorized as “high emitters.” Comparing our data in to that of He et al., the number-based PER of many laser printers can exceed those of a desktop 3-D printer.Citation[29] Note that the elemental composition of particles that was presented in the Supporting Information file was qualitative in nature (i.e., particle number concentration was not quantified from counts of particles on filters and sample collection volumes), as such, the emission rates determined from the non-specific real-time instruments may not correspond to emission rates of some identified constituent metals. Finally, the number-based PER for particles measured using an optical particle counter (>0.65 µm) and mass-based PER values are provided in Supporting Information Table S1.
Table 5. Average geometric mean (GM) particle size and average ± standard deviation particle emission rates (PER) for 3-D and laser printers.
Implications for exposure to vapor-phase pollutants
Based on our data, exposure may occur during pre-printing (e.g., handling stock filaments), printing, and post-printing (e.g., processing printed objects) tasks. From the glass chamber tests, stock ABS filament emitted 1.7 ng acetaldehyde g−1 h−1 (ng VOC per gram comb per hour) and 9.6 ng styrene g−1 h−1. ABS filament is sold in 1 kg spools; using our measured emission rates, if a 3 m3 closet without ventilation is used to store 50 spools, and a worker enters mid-shift (after 4 hours), the concentrations of acetaldehyde and styrene in the closet would be about 110 and 640 µg m−3, respectively. During printing, TVOC SERu ranged from 1085–3550 µg hr−1 for ABS filaments and from 50–130 µg hr−1 for PLA filaments (). Hence, for an 8-hr shift, the TVOC concentrations in a 40 m3 room without ventilation (typical of what we have observed in prototyping workplaces) where a single printer is operating would range from 215–710 µg m−3 (ABS) to 10–26 µg m−3 (PLA). In our experiences, up to 10 printers have been observed to be operating simultaneously in a 40 m3 room, indicating exposures could reasonably be 7100 µg m−3 (ABS) to 260 µg m−3 (PLA). Once printed, objects continue to off-gas VOCs. From the glass chamber tests, a printed ABS comb emitted 4.4–7.4 ng acetaldehyde g−1 h−1 and 5.1–5.9 ng styrene g−1 h−1. Using the average mass of a 3-D printed ABS comb, 3.7 g from Yi et al.,Citation[19] if a storage room (12 m3) without ventilation is used to store 1,000 combs (e.g., before packaging or shipment) and a worker enters the room mid-shift (after 4 hr), the concentrations of acetaldehyde and styrene in the room would be about 5–9 and 6–7 µg m−3, respectively. Note that these scenarios represent “worst case” situations in that we do not account for contaminant decay from air exchange or losses to walls, etc. in the rooms. Stephens et al. estimated that the combined effect of these factors may lower contaminant concentrations by 30–50%,Citation[1] indicating exposures would occur regardless. The current Threshold Limit Value (TLV®), a non-regulatory guidance limit, for acetaldehyde, expressed as a ceiling value (no full-shift time weighted TLV available), is 11 mg m−3. The full-shift time-weighted average TLV for styrene is 85 mg m−3 and the NIOSH Recommended Exposure Limit (REL), a non-regulatory level, is 215 mg m−3. These data indicate that low-level exposures to VOCs may occur during all steps of FDM processes (i.e., procurement/handling of feedstock, printing, and post-printing) in work environments, although the relative contributions differ among steps. Note that TLVs and RELs are not indoor air quality levels intended to protect the general public in the home environment (i.e., 24 hr per day). No standard exists for these compounds in home environments, as such, it is prudent to consider mitigating exposures when using FDM 3-D printers in the home.
Conclusions
Several printer- (number of nozzles, malfunction, controls) and consumable-related (filament type, color) factors were evaluated to understand their influence on chemicals generated by a desktop FDM 3-D printer. Results of our experiments indicate that TVOC emission rates from this 3-D printer were influenced by a printer malfunction, filament type, and to a lesser extent, by filament color. TVOC emission rates were not influenced by the number of printer nozzles used or the manufacturer's provided cover. Of interest is the observation that 14 different VOCs were identified during 3-D printing that were not present during laser printing. Further, carbonyl reaction products were likely formed from emissions of the 3-D printer, including 4-oxopentanal. 3-D printed objects continued to off-gas styrene, indicating potential for continued exposure after the print job is completed. Ultrafine particles generated by the 3-D printer using ABS and a laser printer contained chromium, a known toxicant. Our results indicate that both printer- and consumable-related factors influenced the release of chemical contaminants from a FDM 3-D printer and that understanding these factors can help to better design exposure assessment and control strategies.
Disclaimer
Mention of a specific product or company does not constitute endorsement by the Centers for Disease Control and Prevention. The findings and conclusions in this article are those of the authors and do not necessarily represent the views of the National Institute for Occupational Safety and Health.
2017_01_12_-_VOC_emissions_manuscript_-_Supporting_Information__redline___unlinked_refs_.docx
Download MS Word (1.1 MB)Acknowledgments
The authors wish to thank Mr. Dru Burns for performing some of the GC-MS analyses and Drs. Stephen Jackson and Leonard Zwack for critical review of this manuscript.
Funding
This work was funded by a NIOSH-intramural National Occupational Research Agenda (NORA) grant as well as NIH-R01-ES015022 (TRN), and NSF-DGE-1144676 (TRN). The funding sources had no involvement in the study design; data collection, analysis, and interpretation; writing of the report; and in the decision to submit the article for publication.
References
- International Organization for Standardization/American Society of Testing Materials (ISO/ASTM): Additive manufacturing — General principles — Terminology (ISO/ASTM 52900) [Standard] Geneva, Switzerland: ISO/ASTM, 2015.
- Short, D. B., A. Sirinterlikci, P. Badger, and B. Artieri: Environmental, health, and safety issues in rapid prototyping. Rapid Proto. J. 21(1):105–110 (2015).
- He, Z., G. Li, J. Chen, Y. Huang, T. An, and C. Zhang: Pollution characteristics and health risk assessment of volatile organic compounds emitted from different plastic solid waste recycling workshops. Environ. Int. 77:85–94 (2015).
- Rutkowski, J. V., and B. C. Levin: Acrylonitrile-butadiene-styrene copolymers (ABS): Pyrolysis and combustion products and their toxicity - A review of the literature. Fire Mat. 10(3–4):93–105 (1986).
- Unwin, J., M. R. Coldwell, C. Keen, and J. J. McAlinden: Airborne emissions of carcinogens and respiratory sensitizers during thermal processing of plastics. Ann. Occup. Hyg. 57(3):399–406 (2013).
- Wolkoff, P., C. K. Wilkins, P. A. Clausen, and G. D. Nielsen: Organic compounds in office environments - Sensory irritation, odor, measurements and the role of reactive chemistry. Indoor Air 16(1):7–19 (2006).
- Lee, J. S., H. S. Kwak, B. S. Choi, and S. Y. Park: A case of occupational asthma in a plastic injection process worker. Ann. Occup. Environ. Med. 25(1):25 (2013).
- Van Kampen, V., R. Merget, and X. Baur: Occupational airway sensitizers: An overview on the respective literature. Am. J. Ind. Med. 38(2):164–218 (2000).
- Clausen, P. A., C. K. Wilkins, P. Wolkoff, and G. D. Nielsen: Chemical and biological evaluation of a reaction mixture of R-(+)-limonene/ozone: Formation of strong airway irritants. Environ. Int. 26(7–8):511–522 (2001).
- Tuomi, T., B. Engström, R. Niemelä, J. Svinhufvud, and K. Reijula: Emission of ozone and organic volatiles from a selection of laser printers and photocopiers. Appl. Occup. Environ. Hyg. 15(8):629–634 (2000).
- Wang, H., C. He, L. Morawska, P. McGarry, and G. Johnson: Ozone-initiated particle formation, particle aging, and precursors in a laser printer. Environ. Sci. Technol. 46(2):704–712 (2012).
- Anderson, S. E., J. Franko, L. G. Jackson, J. R. Wells, J. E. Ham, and B. J. Meade: Irritancy and allergic responses induced by exposure to the indoor air chemical 4-Oxopentanal. Tox. Sci. 127(2):371–381 (2012).
- Jarvis, J., M. J. Seed, R. A. Elton, L. Sawyer, and R. M. Agius: Relationship between chemical structure and the occupational asthma hazard of low molecular weight organic compounds. Occup. Environ. Med. 62(4):243–250 (2005).
- Azimi, P., D. Zhao, C. Pouzet, N. E. Crain, and B. Stephens: Emissions of ultrafine particles and volatile organic compounds from commercially available desktop three-dimensional printers with multiple filaments. Environ. Sci. Technol. 50(3):1260–1268 (2016).
- Kim, Y., C. Yoon, S. Ham, J. Park, S. Kim, O. Kwon et al.: Emissions of Nanoparticles and Gaseous Material from 3D Printer Operation. Environ. Sci. Technol. 49(20):12044–12053 (2015).
- Stabile, L., M. Scungio, G. Buonanno, F. Arpino, and G. Ficco: Airborne particle emission of a commercial 3D printer: The effect of filament material and printing temperature. Indoor Air 27(2):398–408 (2017).
- Steinle, P.: Characterization of emissions from a desktop 3D printer and indoor air measurements in office settings. J. Occup. Environ. Hyg. 13(2):121–132 (2016).
- Stephens, B., P. Azimi, Z. El Orch, and T. Ramos: Ultrafine particle emissions from desktop 3D printers. Atmos. Environ. 79:334–339 (2013).
- Yi, J., R. F. LeBouf, M. G. Duling, et al.: Emission of particulate matter from a desktop three-dimensional (3-D) printer. J. Toxicol. Environ. Health A 79:453–465 (2016).
- Jankovic, J. T., S. M. Hollenbeck, and T. L. Zontek: Ambient air sampling during quantum-dot spray deposition. Int. J. Occup. Environ. Health 16(4):388–398 (2010).
- Yi, J., B. T. Chen, D. Schwegler-Berry, et al.: Whole-body nanoparticle aerosol inhalation exposures. J. Vis. Experiments (75):(2013).
- International Organization for Standardization/International Electrotechnical Commission (ISO/IEC): Information Technology - Office Equipment - Determination of Chemical Emission Rates from Electronic Equipment: (ISO/IEC 28360). [Standard] Geneva, Switzerland: ISO/IEC, 2007.
- Bundesanstalt für Materialforschung und-prüfung (BAM): Basic Criteria for Award of the Environmental Lable: Office Equipment with Printing Function (Printers, Copiers, Multifunction Devices) (RAL-UZ-171). [Standard] St. Augustin, Germany: BAM, 2012.
- LeBouf, R. F., M. A. Virji, R. Saito, P. K. Henneberger, N. Simcox, and A. B. Stefaniak: Exposure to volatile organic compounds in healthcare settings. Occup. Environ. Med. 71(9):642–650 (2014).
- Ham, J. E., S. R. Jackson, J. C. Harrison, and J. R. Wells: Gas-phase reaction products and yields of terpinolene with ozone and nitric oxide using a new derivatization agent. Atmos. Environ. 122:513–520 (2015).
- Jackson, S. R., J. E. Ham, J. C. Harrison, and J. R. Wells: Identification and quantification of carbonyl-containing α-pinene ozonolysis products using O-tert-butylhydroxylamine hydrochloride. J. Atmos. Chem. DOI:10.1007/s10874-016-9344-6.
- Wells, J. R., and J. E. Ham: A new agent for derivatizing carbonyl species used to investigate limonene ozonolysis. Atmos. Environ. 99:519–526 (2014).
- Liu, B. Y. H., D. Y. H. Pui, and K. L. Rubow: Characteristics of air sampling filter media. In Aerosols in the Mining and Industrial Work Environments, V. A. Marple and B. Y. H. Liu (eds.). Ann Arbor, MI: Ann Arbor Science, 1983. pp. 989–1038.
- He, C., L. Morawska, H. Wang, et al.: Quantification of the relationship between fuser roller temperature and laser printer emissions. J. Aerosol Sci. 41(6):523–530 (2010).
- Nørgaard, A. W., V. Kofoed-Sørensen, C. Mandin, et al.: Ozone-initiated terpene reaction products in five European offices: Replacement of a floor cleaning agent. Environ. Sci. Technol. 48(22):13331–13339 (2014).
- Nørgaard, A. W., J. D. Kudal, V. Kofoed-Sørensen, I. K. Koponen, and P. Wolkoff: Ozone-initiated VOC and particle emissions from a cleaning agent and an air freshener: Risk assessment of acute airway effects. Environ. Int. 68:209–218 (2014).
- Wisthaler, A., and C. J. Weschler: Reactions of ozone with human skin lipids: sources of carbonyls, dicarbonyls, and hydroxycarbonyls in indoor air. Proc. Natl. Acad. Sci. USA. 107(15):6568–6575 (2010).
- Brown, S. K.: Assessment of pollutant emissions from dry-process photocopiers. Indoor Air 9(4):259–267 (1999).
- Castellano, P., S. Canepari, R. Ferrante, and N. L'Episcopo: Multiparametric approach for an exemplary study of laser printer emissions. J. Environ. Monit. 14(2):446–454 (2012).
- Lee, S. C., S. Lam, and H. Kin Fai: Characterization of VOCs, ozone, and PM10 emissions from office equipment in an environmental chamber. Build. Environ. 36(7):837–842 (2001).
- Leovic, K. W., L. S. Sheldon, D. A. Whitaker, R. G. Hetes, J. A. Calcagni, and J. N. Baskir: Measurement of Indoor Air Emissions from Dry-Process Photocopy Machines. J. Air Waste Manag. Assoc. 46(9):821–829 (1996).
- Wang, Z. M., J. Wagner, and S. Wall: Characterization of laser printer nanoparticle and VOC emissions, formation mechanisms, and strategies to reduce airborne exposures. Aerosol Sci. Technol. 45(9):1060–1068 (2011).
- Contos, D. A., M. W. Holdren, D. L. Smith, R. C. Brooke, V. L. Rhodes, and M. L. Rainey: Sampling and analysis of volatile organic compounds evolved during thermal processing of acrylonitrile butadiene styrene composite resins. J. Air Waste Manag. Assoc. 45(9):686–694 (1995).
- Forrest, M. J., A. M. Jolly, S. R. Holding, and S. J. Richards: Emissions from processing thermoplastics. Ann. Occup. Hyg. 39(1):35–53 (1995).
- Barthel, M., V. Pedan, O. Hahn, et al.: XRF-analysis of fine and ultrafine particles emitted from laser printing devices. Environ. Sci. Technol. 45(18):7819–7825 (2011).
- Morawska, L., C. He, G. Johnson, R. Jayaratne, T. Salthammer, H. Wang et al.: An investigation into the characteristics and formation mechanisms of particles originating from the operation of laser printers. Environ. Sci. Technol. 43(4):1015–1022 (2009).
- Pirela, S. V., G. A. Sotiriou, D. Bello, et al.: Consumer exposures to laser printer-emitted engineered nanoparticles: A case study of life-cycle implications from nano-enabled products. Nanotoxicology 9(6):760–768 (2015).
- Salthammer, T., T. Schripp, E. Uhde, and M. Wensing: Aerosols generated by hardcopy devices and other electrical appliances. Environ. Pollut. 169:167–174 (2012).
- LeBlanc, A. J., J. L. Cumpston, B. T. Chen, D. Frazer, V. Castranova, and T. R. Nurkiewicz: Nanoparticle inhalation impairs endothelium-dependent vasodilation in subepicardial arterioles. J. Toxicol. Environ. Health A 72(24):1576–1584 (2009).
- Nurkiewicz, T. R., D. W. Porter, A. F. Hubbs, et al.: Nanoparticle inhalation augments particle-dependent systemic microvascular dysfunction. Part. Fibre Toxicol. 5:1 (2008).
- Oberdorster, G.: Pulmonary effects of inhaled ultrafine particles. Int. Arch. Occup. Environ. Health 74(1):1–8 (2001).
- He, C., L. Morawska, and L. Taplin: Particle emission characteristics of office printers. Environ. Sci. Technol. 41(17):6039–6045 (2007).