Abstract
Multiple myeloma (MM) induced bone lesion is one of the most crippling characteristics, and the MM secreted Dickkopf-1 (DKK1) has been reported to play important role in this pathologic process. However, the underlying regulation mechanisms involved in DKK1 expression are still unclear. In this study, we validated the expression patterns of microRNA (miR) 15a, 34a, 152, and 223 in MM cells and identified that miR-152 was significantly downregulated in the MM group compared with the non-MM group, and that miR-152 level was negatively correlated with the expression of DKK1 in the MM cells. Mechanistic studies showed that manipulating miR-152 artificially in MM cells led to changes in DKK-1 expression, and miR-152 blocked DKK1 transcriptional activity by binding to the 3′UTR of DKK1 mRNA. Importantly, we revealed that MM cells stably expressing miR-152 improved the chemotherapy sensitivity, and counteracted the bone disruption in an intrabone-MM mouse model. Our study contributes better understanding of the regulation mechanism of DKK-1 in MM, and opens up the potential for developing newer therapeutic strategies in the MM treatment.
Abbreviations
MM | = | Multiple myeloma |
DKK1 | = | Dickkopf-1 |
miR-152 | = | MicroRNA 152 |
qPCR | = | Quantitative polymerase chain reaction |
3′UTR | = | Three prime untranslated region |
SCID | = | Severe combined immunodeficiency |
FDR | = | False discovery rate |
WT | = | Wild type |
MT | = | Mutated |
EV Ctrl | = | Empty vector control. |
Introduction
Multiple myeloma (MM) is a hematological neoplasm of B cells characterized by malignant proliferation of secretory plasma cells in bone marrow.Citation1 It is estimated that at least 86,000 new cases of MM are reported annually worldwide.Citation2 There are several combinations of drugs such as melphalan, prednisone, bortezomib, lenalidomide, dexamethasone and thalidomide that are available as front line agents for MM.Citation3-5 Only few patients are eligible for chemotherapy followed by stem-cell transplantation.Citation6 Often, high-dose therapies are associated with fatal complications and relapse of MM.Citation7 Thus, more robust therapies aimed at better disease control and chemotherapy resistance are necessary.
Severe bone destruction and decreased bone mass is a cardinal aspect of MM, occurring as a result of imbalance between osteoclast and osteoblast function.Citation8 Regulation of these activities involves Wnt signaling, controlled by antagonists such as dickkopf homolog 1 (DKK1) family of proteins. DKK1 is a secretory protein involved in the inhibition of Wnt/β-catenin pathway by interacting with the Frizzled co-receptor LRP6.Citation9 High expression of DKK1 is characterized by extensive bone disease in MM patients, because mesenchymal stem cells do not differentiate to osteoblasts.Citation10,11 However, bone marrow biopsies disclosed that not all patients with lytic bone lesions exhibit elevated DKK1 expression, which is in fact limited by specific disease stages.Citation11 However, deletion of a single allele of DKK1 in mouse osteoblasts led to increased bone formation and bone mass.Citation12 In a SCID-rab animal model, blockade of DKK1 with a neutralizing antibody reduced bone resorption and tumor growth.Citation13 Anti-DKK1 (BHQ880) antibody in a SCID–hu murine model decreased myeloma bone disease, and also indirectly inhibited tumor growth.Citation14 Taken together these reports indicate that DKK1 is a key player in the development of myeloma and blocking DKK1 activity may increase bone formation, reduce osteolytic bone resorption, and may even prevent myeloma tumor progression. Although it may seem like a tangible strategy to inhibit DKK1, the mechanism of regulation of DKK1 in MM is poorly understood.
Deregulation of miRNAs in various human cancers and their role in regulating tumor initiation and suppression by targeting oncogenes or tumor suppressors have been surmised.Citation15,16 MiR-148/152 family members are known to have several targets and whether they are relevant to function depends on their specific target mRNAs.Citation17,18 MiRNA-152 is reported to be associated primarily with cellular proliferation, invasion and angiogenesis.Citation19 Their predominant role being a tumor suppressor in a plethora of human cancers such as breast,Citation20 endometrial,Citation21,22 ovarian.Citation23 and cholangiocarcinoma.Citation17 have been reported. In MM, miRNA expression profiles of hyperdiploid and nonhyperdiploid types indicated that specific downregulation of miR-425, miR-152, and miR-24 was observed in hyperdiploid MM.Citation24 However, the function of miR-152 in MM, particularly in the regulation of DKK-1, has not yet been reported.
In the current study, we show for the first time the link between miR-152 and DKK1 in the pathogenesis of MM, which could be effectively antagonized by the overexpression of miR-152. In MM cell lines, miR-152 mimic in combination with melphalan synergistically induce PARP-mediated apoptosis. Further, in vivo studies demonstrate that over expression of miRNA-152 could reverse the bone destruction and enhance bone mineralization in MM mouse models.
Results
Expression of miR-152 is downregulated in MM patients
According to a previous study,Citation24 4 miRNAs, miR-152, miR-15a, miR34a, and miR-223 were found to be downregulated (False discovery rate, FDR < 0.05) in the MM group compared to the non-MM group using a miRNA array. To validate this finding in our set of experiments, we performed qRT-PCR to detect the change of expression of these 4 microRNAs in B cells from 16 healthy donors and 18 primary human multiple myeloma samples. The data indicated that among these 4 miRNAs, miR-15a, miR-34a, and miR-152 were downregulated significantly in MM group compared with the non-MM group, and among them, miR-152 was the one with the lowest level; however, we did not observe a significant difference between MM group and non-MM group in the expression of miR-223 (). For the better accuracy of results, expression of miR-152 was analyzed using log2(fold change) (ΔΔCt [MM/non-MM]) in all the 18 MM samples (). Results are integrated in a pie chart. ().
Figure 1. Gene expression of miR-152, miR-15a, miR-34a, and miR-223 in human multiple myeloma. (A) Expression of 4 candidate miRNAs was analyzed in MM patients (N = 18) and B cells from healthy donors (N = 16, non-MM group) by qPCR, after normalizing with the endogenous control U6. Among the 4 miRNAs, miR-152 showed the most significant downregulation compared to non-MM group (p < 0.004). (B) The expression of miR-152 in all the 18 MM samples were analyzed by log2(fold change)(ΔΔCt [MM/non-MM]). (C) Pie chart shows the percent distribution of miR-152 in downregulated, upregulated and unchanged samples from MM group.
![Figure 1. Gene expression of miR-152, miR-15a, miR-34a, and miR-223 in human multiple myeloma. (A) Expression of 4 candidate miRNAs was analyzed in MM patients (N = 18) and B cells from healthy donors (N = 16, non-MM group) by qPCR, after normalizing with the endogenous control U6. Among the 4 miRNAs, miR-152 showed the most significant downregulation compared to non-MM group (p < 0.004). (B) The expression of miR-152 in all the 18 MM samples were analyzed by log2(fold change)(ΔΔCt [MM/non-MM]). (C) Pie chart shows the percent distribution of miR-152 in downregulated, upregulated and unchanged samples from MM group.](/cms/asset/0ff452d6-0dbb-45d2-9f91-1bc999bb3ec9/krnb_a_1094600_f0001_c.gif)
Expression of miR-152 is negatively correlated with DKK-1 levels
As published studies suggest, DKK1 is highly expressed in most primary myeloma cells of patients with MM, which also plays important roles in the tumorigenesis, bone disruption, and metastasis.Citation11,25,26 We hypothesized that downregulation of miR-152 could have a close relationship with the upregulation of DKK1 in myeloma. The correlation analysis revealed that there is an inverse correlation between the expression of miR-152 and DKK1 in MM (p < 0.001, R2 =0.27) (). Moreover, we chose 2 samples with different levels of the DKK1 by immunohistochemistry, and detected the miR-152. We found that in one sample with high DKK1 protein, the miR-152 level was significantly lower than a non-MM control; whereas another sample with low DKK1 protein demonstrated no change in the miR-152 levels between the MM and non-MM (). To further investigate the correlation between DKK1 and miR-152, 6 normal B cells and 8 MM cell lines were used to compare the DKK1 protein/mRNA and miR-152 expression. Our data suggest that B cells express relatively low levels of DKK1 protein compared to most other MM cells. Likewise, DKK1 mRNA levels were also significantly lower in B cells compared to all other MM cell lines. Further, we used qRT-PCR and Western blot to detect the DKK1 levels in 8 multiple myeloma cell lines compared with 6 B cells isolated from healthy donors, and MM cell lines were classified into 2 groups based on differential expression of DKK1. As shown in the , U266, RPMI 8266, OPM-2 and MM.1S belonged to high-level group, while H929, OPM-1, MM144 and IM-9 constituted the intermediate level (). This expression of DKK1 is consistent with another study in cell lines and patients.Citation25 Interestingly, miR-152 were negatively associated with DKK-1 mRNA, which corroborate with our hypothesis that DKK1 may be regulated by miR-152.().
Figure 2. Expression of miR-152 negatively impacted DKK-1 in MM. (A) Spearman correlation analysis showing relative expression of miR-152 and DKK1 in 18 human multiple myeloma patients. An inverse correlation between miR-152 and DKK1 was observed (p < 0.001, R2 = 0.27). (B) Expression of miR-152 in one of the each representative MM and non-MM samples (upper panel). Significant difference at **p<0.01 in MM vs. non-MM from 3 independent experiments. Corresponding immunohistochemistry of DKK1 expression (lower panel) indicates an inverse relationship with miR-152. (C) Expression of DKK1 levels in 6 normal B cells and 8 MM cell lines as determined by Western blotting (upper panel). Levels of DKK1 were reduced in the B cells compared to the MM cell lines. β-actin was used as the loading control. Likewise, very low levels of DKK1 mRNA were found in the B-cells, while high and intermediate levels of DKK1 mRNA were found in the MM cell lines. (D) Expression of miR-152 in B cells and MM cell lines demonstrate high mRNA levels in the B cells compared to MM cell lines. Statistical significances at *p < 0.05 and **p < 0.001 vs. B cells in both (C) and (D) from 3 independent experiments. The band intensity of DKK1 was normalized against that of β-actin, and the ratios (Mean ± SD) were shown at the WB band bottom for 3 independent assay.
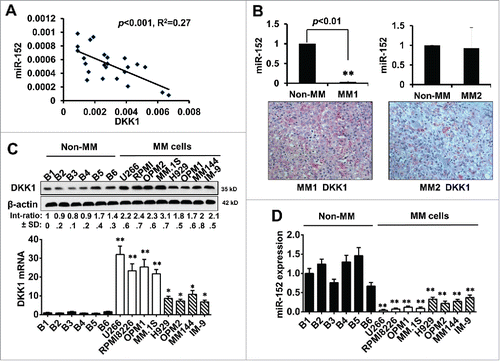
Manipulating miR-152 regulates DKK-1 expression
To study whether miR-152 indeed participates in the regulation of DKK-1, gain-of-function and loss-of-function experiments were designed. We utilized 4 cell lines, U266 and MM.1S - DKK1 high expression group, and OPM-2 and MM144 - intermediate expression group, to check whether ectopic expression of miR-152, or suppression of endogenous miR-152 influence DKK1 expression. Results show that DKK1 mRNA and proteins were significantly decreased compared with control (). On the other hand, upon knockdown of endogenous miR-152 by inhibitor, expression of DKK-1 mRNA and proteins were significantly upregulated. ().
Figure 3. Modulation of miR-152 governs DKK-1 expression in MM cell lines. Overexpression of miR-152 by transfection with a mimic for 48 h decreased (A) DKK1 mRNA and (B) protein levels compared with non-target control (NT Ctrl) in the MM cell lines. (C) Suppression of miR-152 with an inhibitor for 48 h resulted in the upregulation of DKK1 mRNA. Effect of NT control was negligible. (D) Expression of DKK1 in the above MM cells was increased corresponding with the downregulation of miR-152 by the inhibitor. Statistical significances at *p < 0.05 and **p < 0.001 vs. NT Ctrl in both (A) and (C) from 3 independent experiments. The band intensity of DKK1 was normalized against that of β-actin in 4 MM cell lins, and the ratios (Mean ± SD) were shown at the WB band bottom for 3 independent assay.
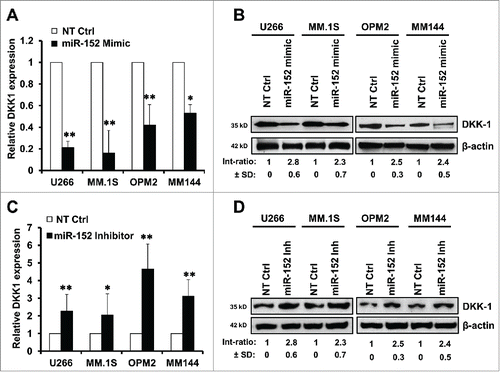
MiR-152 interrupts expression of DKK-1 by binding to 3′UTR of DKK-1 mRNA
To establish a functional relationship between miR-152 and DKK-1 regulation, we aligned the sequences of miR-152 with DKK-1 3′UTR on the website (http://www.microrna.org). Interestingly, there were 9 binding sites (at positions 263, 264, and 267-273) of miR-152 in DKK-1 3′UTR (, upper panel). Next, we cloned 851bp of 3′UTR of DKK1 mRNA into a pMIR-Report plasmid (pMIR-DKK1-3′UTR-wt), as well as mutant clone with 3 of the miR-152 binding sites changed (pMIR-DKK1-3′UTR-mt) to determine the transcriptional activity in MM.1S and OPM-2. Relative luciferase activity upon transfection of non-target control (NT Ctrl) or miR-152 mimic at different doses (0, 50, 100, 200nM) demonstrated that only the miR-152 mimic, but not the NT Ctrl, suppressed transcriptional activity of the 3′UTR DKK1 (, lower panel). On the other hand, in the mutant clone (as indicated in the , upper panel), inhibitory effect of miR-152 mimic on the 3′UTR DKK1 was not observed (, lower panel). Conversely, in the presence of miR-152 inhibitor, relative luciferase activity of pMIR-DKK1-3′UTR-wt was significantly increased (), while it was unaffected in the mutant (). These results indicate that DKK-1 gene is directly regulated by miR-152.
Figure 4. Binding of miR-152 to the 3′ UTR blocks DKK1 activity. (A) MM.1S and OPM-2 cells were infected with either DKK1 mRNA 3′UTR reporter plasmid (pMIR-DKK1-3′UTR-wt) or the mutant with 3 miR-152 binding sites changed (pMIR-DKK1-3′UTR-mt), and luciferase activity was evaluated. Upper panel shows the wild-type binding sites, while the lower panel demonstrates that the relative luciferase activity is declined in the presence of increasing concentration of the miR-152 mimic compared with non-target control (NT Ctrl), indicating overexpression of miR-152 by mimic decreased DKK1 activity considerably. (B) Upper panel shows mutated binding sites (the changed sites are shown in dotted lines). There was no change in the luciferase activity in presence of mimic after mutating the binding sites (lower panel), indicating feeble effects on the DKK1 activity. (C) Relative luciferase activity of DKK1 was upregulated in the absence of miR-152 overexpression or inhibitor treatment. (D) As observed previously, DKK1 activity was unaffected on mutating the binding sites, even in the presence of a miR-152 inhibitor. Statistical significances at *p < 0.05 and **p < 0.001 vs. respective NT Ctrl in (A) and (C) from 3 independent experiments.
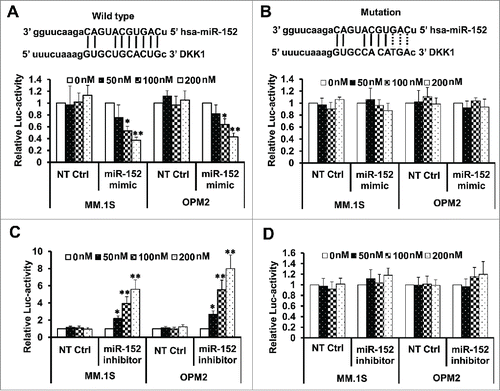
Overexpression of miR-152 and melphalan induces robust apoptosis
We determined whether miR-152 mimic either alone or in combination with melphalan indeed participates in the antitumor effects by induction of apoptosis in MM. The results demonstrate that miR-152 mimic alone did not induce apoptosis, and was similar to the NT Ctrl in both cells, while melphalan alone was slightly better than the mimic by itself. However, presence of miR-152 mimic and melphalan induced robust apoptosis in both MM.1S and OP-M2 cells, indicating a synergistic effect on apoptosis (). Quantitative data of the effects on percent apoptotic cells are shown in.
Figure 5. Targeting DKK-1 activity by the combination of miR-152 mimic and melphalan sensitizes MM cells to apoptosis. Flow cytometry assays were performed using Annexin V-FITC and PI to show the apoptotic cells in MM.1S or OPM-2 cells treated with NT control, miR-152 mimic or melphalan in different combinations. (A) miR-152 mimic in conjunction with melphalan induced noticeable apoptotic effects in the MM cells compared with either agent alone in both cell lines (B) Quantitative estimation of percent apoptotic cells from 3 independent experiments. Statistical significances at *p<0.05 vs. mimic or NT Ctrl and **p < 0.001 vs. mimic or melphalan and/or NT Ctrl. (C) MM.1S and OPM-2 cells were transfected with 100 nM of miR-152 mimic or NT Ctrl for 48 h, exposed to 5 µM of melphalan, cultured for 24 h, and the lysates were detected for the cleavage of PARP (c-PARP) proteins by Western blotting. Bands showing the cleavage of PARP in MM.1S or OPM-2 cells treated with specific agents are as indicated. The band intensity of c-PARP was normalized against that of β-actin and the ratios (Mean ± SD) were shown at the WB band bottom for 3 independent assay.
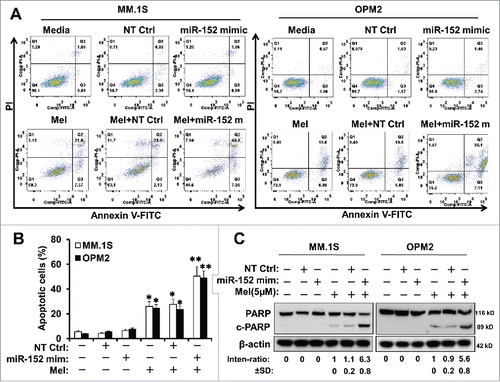
In order to investigate more detailed apoptotic mechanisms, cleavage of PARP was measured. Our results demonstrate that the cleavage of PARP was evident only in melphalan or melphalan+miR-152 mimic/NT Ctrl exposed cells (). Further, miR-152 mimic and melphalan combination induced severe PARP cleavage.
Overexpression of miR-152 alleviates bone destruction in vivo
Animal experiments were set up to determine whether miR-152 and DKK1 play key roles in the pathogenesis of MM induced bone lesions. Two stably expressing MM.1S cells infected with either miR-152 (miR-152MM.1S) or Vector control(vecMM.1S) was injected into the femur. As expected, the expression of miR-152 was significantly elevated in the bone marrow of miR-152MM.1S compared to vecMM.1S, and corresponding DKK1 proteins were decreased in the miR-152MM.1S compared to vecMM.1S (). H&E staining confirmed that the injections were consistent and successful in the mice bone marrow (). Next, immunohistochemical staining on the bone marrow cells confirmed the negative correlation as observed in vitro (). CD138+ expression confirmed the presence of MM in these animals.
Figure 6. Over-expression of miR-152 alleviates bone destruction in MM in vivo. (A) Stably expressing miR-152 or empty vector infected MM.1S cells were injected into bone marrow of mice for the development of MM. Levels of miR-152 (left panel) and DKK1 mRNA and protein (right panel) are obtained from the mice bone marrow, when the disease is confirmed. Statistical significances at **p < 0.001 vs. empty vector from 3 independent experiments. (B) H&E staining showing equal and successful injection of 0.5×106 VecMM.1S and miR-152MM.1S cells into mice bone marrow. (C) Representative immunohistochemical staining of DKK1 (upper panel) and CD138+ (lower panel) in the VecMM.1S and miR-152MM.1S cells obtained from the mice bone marrow. (D) Representative fluorescent micrographs of dual calcein labeling of femur bone from 2 mice injected with 0.5×106 VecMM.1S and miR-152MM.1S cells. The distance between the 2 calcein labeling layers reflects the bone mineralization rate, and the analysis of the intensity ratio between the VecMM.1S group and the miR-152MM.1S groups were shown at the bottom. Bars represent the mean ± SEM of n = 10 mice per group (*P < 0.05).
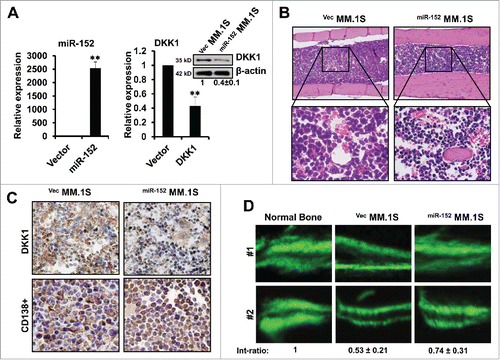
Our data on bone formation in vivo indicate that the distance between 2 consecutive labels in the calvaria by calcein labeling was dramatically increased in the vecMM.1S compared to miR-152MM.1S mice, indicating that over expression of miR-152 results in increased bone mineralization, as shown in 2 representatives of each group (). Furthermore, the bone formation in miR-152MM.1S mice was almost identical to the normal. Taken together, these data indicate that overexpression of miR-152 levels, could enhance the bone formation by way of suppressing DKK1 in MM.
Discussion
Myelomas are characterized by accumulation of monotypic plasma cells with strong bone marrow dependence contributing to osteolytic lesions, and skeletal devastation.Citation27 Despite substantial advances in the understanding of contextual molecular mechanisms, MM still remains highly incurable. Our study essentially addresses a novel role for miR-152 and its inverse relationship with DKK1 in the pathogenesis of MM. Expression of miR-152 was downregulated in the majority of MM patients and cell lines, which was negatively correlated with DKK1. Antagonizing or overexpressing miR-152 by targeting 3′UTR of DKK1, reversed the effects of DKK1 in MM cell lines. MiR-152 mimic and melphalan synergistically induced apoptosis in MM cells. Further, SCID mice models of MM carrying stably expressing miR-152, attenuated bone destruction and improved bone mineralization, providing supporting evidence for the role of miR-152 in the regulation of MM.
MiRNAs are ∼20-nucleotide genome-encoded RNAs that are critical in controlling cell proliferation, stem cell differentiation, apoptosis, and metabolism.Citation28 Literature advocates the deregulation of several miRNAs including miR- 15, 16, 17, 20, 135, 30, 150, 160 and so on, which are regarded as hallmarks of tumor progression, clinical staging, prognosis, and drug responses in MM.Citation29-34 Basic mechanisms of miRNAs include positive or negative regulation of critical oncogenes or tumor suppressors, epigenetic modifications etc. that predominantly determines cell survival and malignant transformation.Citation35-37 So far, a specific role for miR-152 and a relationship with DKK1 in MM has not been described.
The three mature members of the miR-148/152 family- miR-148a, miR-148b and miR-152 are about 21-22 nucleotides in length, with 6-7 nucleotides as same seed sequence, that serves as the prime region for binding to 3′UTR of target mRNAs.Citation19 In our study, we assessed 4 miRNAs (miR-152, 15a, 34, and 223) in MM and non-MM groups. Our results suggested that miR-152 expression was significantly downregulated in the majority of MM patients and cell lines compared with non-MM; consistent with the reports from other cancers. MiR-148/152 family members are predominantly downregulated in a variety of human cancers affecting liver, intestine, bile duct, pancreas, ovary, endometrium etc. as reviewed.Citation19 These point to the fact that they may act as tumor suppressor in MM as well. As known, microRNAs are regulated at transcriptional level and at post-transcriptional level,Citation38 and mature miRNA has 2 arms, the 5p and 3p.Citation39 The primary hairpin miRNA transcript (pri-miRNA) is generated by RNA polymerase II or III from microRNA gene or introns in nucleus, cleaved and digested by the microprocessor complex (Drosha-DGCR8) to the pre-miRNA, which is exported to the cytoplasm, and finally the pre-miRNA is cleaved and digest by Dicer, TRBP and Paz proteins to produce a mature duplex miRNA. The 3p arm is generally considered non-functional as it does not incorporate into the RISC complex during biogenesis, few authors have considered 3p arm to be functional because of binding to the different Agonaute proteins.Citation40 So far, very limited studies were found concerning the miR-152-3p and miR-152-5p, except an up-to-date report by Langhe R et al revealing that both 3p and 5p of miR-152 were found significantly down-regulated in ovarian cancer.Citation41 In our study, we presume that miR-152 was regulated at transcriptional level in MM cells, and examined the mature miR-152. The details in miR-152 regulation should be considered in further studies.
Multi-algorithm target prediction approach identified potential physiologically relevant targets of miR-152 contributing to the MM development. Computational prediction of targets relies on the pairing of the seed sequence of the miRNA to a complementary 3′UTR following strict base-pairing rules.Citation42 In the current study, several important binding sites of miR-152 to the 3′UTR of DKK1 mRNA were identified. DKK1 has been reported to be overexpressed in MM and in other cancers such as hepatoblastoma, Wilm's tumors and breast cancer.Citation11,43,44 Qian et al. showed that the primary tumor cells of all patients with MM express DKK1, and DKK1 peptide-specific cytotoxic T cells can effectively kill primary MM cells in vitro.Citation25 Whereas treatment with specific miR-152 mimic partly silenced the functioning of DKK1 mRNA transcripts, it was reversed in the presence of miRNA inhibitor, further confirming DKK1 as an authentic target of miR-152 in myeloma. To our knowledge, this is the first study that has defined a strategic link between miR-152 and DKK1 in any of the cancers. Previous studies have shown that other oncogenic miRNAs such as miR-372, 373 and 29a can directly target DKK1 promoting oncogenesis and chemoresistance in other cancers.Citation45,46
Of note, we also show that melphalan in the presence of miR-152 mimic sensitized MM cells to a more pronounced apoptotic cell death. Melphalan has been demonstrated to induce apoptosis either alone or in combination with other drugs through various canonical mechanisms.Citation47-49 Antimyeloma effect of proteasomal inhibitor bortezomib was enhanced in the presence of melphalan, and follow up clinical trials showed greater plasma cell apoptosis in MM patients.Citation50 Apoptosis of MM cells occurs through multiple mechanisms including down-regulation of NF-κB, intrinsic and extrinsic signaling, and inhibition of MM cell adhesion to the bone marrow stromal cells.Citation51 Although, not extensive with regard to apoptosis, our data demonstrate an effectual and synergistic strategy utilizing miR-152 to enhance the sensitivity of MM cells to apoptosis.
As elaborated earlier, since DKK1 is a direct target of miR-152, and increased DKK1 expression is a hallmark of MM, current study addressed the role of DKK1 and miR-152 in the bone mineralization using in vivo models of MM. Specifically, MM.1S cells containing empty vector developed decreased bone thickness, and lower mineralization as the distance between 2 calvaria consecutive labels increased; but on the other hand, miR-152MM.1S cells reversed the effects and was very much comparable to a normal bone. Thus, in vivo experiments conveyed that overexpression of miR-152 induces bone mineralization and also possibly repair lytic lesions associated with MM. Since DKK1 is a key molecule involved in the bone remodeling, it is logical to assume that MM cells-derived DKK1 may be responsible, at least in part, for the suppressed bone formation and bone destruction associated with MM as published earlier.Citation11 This possibly explains the decreased bone mineralization observed in the empty vector MM.1S animals, in the absence of overexpression of miR-152. Notably, this is in agreement with a previous study where inhibition of DKK1 activity increased osteoblastic differentiation, reduced osteoclast activity and MM growth in a myelomatous SCID-rab mice model.Citation13 Finally, these imply that miR-152 could be a novel entity regulating DKK1 expression in MM cells, which partially explains how deregulation of DKK-1 features a common pathogenic link in MM.
In conclusion, our study for the first time identified that miR-152 is a master regulator in the pathobiology of MM via targeting of DKK1. Developing tailored interventions and combination therapies by rational algorithms for both low-and high-risk patient subgroups, and identifying novel targets and therapies, although a herculean task is much needed. It is plausible that manipulation of miRNA could also lead to non-specific systemic effects; nevertheless, exploration of novel interaction network of molecules like miR-152 and DKK1 may open new avenues and novel strategies for the treatment of MM.
Methods
Cell lines and primary MM cells
The MM cell lines MM.1S, U266, OPM-2, RPMI 8266, H929, OPM-1, MM144, and IM-9 were purchased from the American Type Culture Collection (ATCC). Samples from MM patients (bone marrow aspirates) and healthy donors (blood) were collected at the Yongchuan Hospital Affiliated to Chongqing Medical University, and People's Hospital of Bishan District. Primary MM cells were isolated from bone marrow aspirates of MM patients using anti-CD138+ antibody-coated magnetic beads (Miltenyi Biotec Inc.., USA). B cells were isolated from peripheral blood mononuclear cells (PBMC) of healthy donors using EasySep™ Direct Human B Cell Isolation Kit (StemCell Technologies, Inc. Inc.., Canada). MM cells were maintained in RPMI-1640 medium, supplemented with 10% fetal bovine serum, penicillin (10,000 units/mL), and streptomycin (10 mg/mL) obtained from Life Technologies, USA. All patients gave their informed consent. The Institutional Review Board of the Chongqing Medical University approved this study.
RNA extraction and qRT-PCR
Total RNA was extracted using either the RNeasy kit or the miRNeasy mini kit (Qiagen Inc.., USA) following manufacturer's instructions). qRT-PCR was performed as previously reported.Citation52 The relative amount of each miRNA to U6 snRNA was calculated using the equation 2−△△Ct. Primers used were DKK1: 5′-ctcggttctcaattccaacg-3′ (F) and 5′-gcactcctcgtcctctg-3′ (R); U6: 5′-cgcaaggatgacacgcaaattc-3′ (F) and 5′-tagcagcacgtaaatattggcg-3′ (R); β-actin: 5′-aaggccaaccgcgag-3′ (F) and 5′-taatgtcacgcacgattcccg-3′ (R). Each experiment was performed in triplicates and the mean was determined.
MiRNA inhibition/overexpression and Western blotting
Inhibition or overexpression of miR-152 by using mimic or inhibitors was performed by electroporation under conditions of 1600V, 20Ω, and 1 pulse in MM cells. The control cells were infected with empty vector constructs. For stably expressing miR-152 in MM.1S cells, the lentivirus pEZX-miR-152 encoding miR-152 precursor was used for the infection (Genecopoeia, USA), and MM cells were selected using puromycin. After treatment, cells were collected for appropriate experiments. For Western blotting, cells were lysed in 1×lysate buffer (Cell Signaling, USA), and 50 μg of total protein was separated on a 4-12% gel (Life Technologies, USA). Primary antibodies, DKK1 and β-actin (Cell Signaling) were incubated at 4°C, followed by appropriate secondary antibody, and developed using chemiluminescence. Protein densitometry was performed using the ImageJ software.
Immunohistochemistry
Paraffin-embedded sections were deparaffinized, blocked, and incubated with 1:200 anti-DKK1 or anti-CD138+ (Millipore, USA) at 4°C overnight. Sections were incubated with HRP-conjugated secondary antibody at 1:500 for 1 h, developed using DAB, and counterstained with hematoxylin. Images were captured on an Olympus-BHS microscope attached to a QImaging Retiga 4000RV digital camera.
Cell apoptosis and luciferase assay
Annexin V-binding assay was used to detect apoptosis, according to the manufacturer's instructions (BD Biosciences, USA). In brief, MM cells collected after 48 h of treatment with various agents and were used for the flow cytometric analysis (BD LSRFortessa flow cytometer). The 3′UTR sequence (851bp) from human DKK1 mRNA was cloned by RT-PCR with the primers containing Mlu I and Spe I restriction enzymes into the pMIR-Report-3′UTR plasmid (Life Technologies) using primers, 5′-cgacgcgtcgcaccatcaagccagtaattcttc-3′ (F) and 5′-gactagtcttcactctcattctgccatga-3′ (R). In addition, corresponding to the wild-type 3′UTR reporter plasmid (pMIR-DKK1-3′UTR-wt), variant constructs (pMIR-DKK1-3′UTR-mt) were produced using the QuikChange XL Kit (Life Technologies), in which 3 specific binding sites at the 5′ flanking of miR-152 (271G, 272A, 273C) were mutated using following primers:
F:5′-aaatttacataacaagaggaaaaataggtcatgcagcacctttagaaaaataattaaaagtttcattgc-3′
R: 5′-gcaatgaaacttttaattatttttctaaaggtgctgcatgacctatttttcctcttgttatgtaaattt-3′
For the luciferase assay, 1×106 MM cells were transfected with 5µg of pMIR-DKK1-3′UTR-wt or pMIR-DKK1-3′UTR-mt plasmids and 100 nM of miR-152 mimic, inhibitor or non-target controls (Life Technologies), and 0.5 µg of the Renilla luciferase vector pRL-TK (Promega, USA). Luciferase activity was measured post-transfection at 48 h using a Dual-Luciferase Kit (Promega).
Animal studies
Female C.B-17 SCID mice (n = 10/group, 5 mice per cage) for the experiments were inbred and maintained in the animal facility of Chongqing Medical University. To determine the effect of DKK1/miR-152 on the bone formation in vivo, we used an intra-bone injection model. In brief, 5×105 stable MM.1S cells [infected with either miR-152 (miR-152MM.1S) or Vec control (vecMM.1S)] were injected into the femur of each mouse. CD138+ staining was used to confirm MM after 4 weeks of cell injections. Calcein was injected subcutaneously twice at 4-day intervals prior to evaluating bone mineral apposition as described.Citation53 After 24 h of the last calcein injection, both femurs were extirpated, fixed in 70% ethanol, and further processed. The intensity of calcein signal was analysis by Photoshop 7.0 software. The Institutional Animal Care and Use Committee, Chongqing Medical University approved the animal protocols related to this study.
Statistical analysis
Statistical analysis was performed using GraphPad Prism 5 (GraphPad, USA). Data are presented as the mean ± standard deviation (SD) unless otherwise indicated. Independent-samples t-test or ANOVA (one-way analysis of variance) was used to determine the difference between or among groups. p<0.05 was considered statistically significant.
Disclosure of Potential Conflicts of Interest
No potential conflicts of interest were disclosed.
Author Contributions
Liu B and George SK designed the project and revised the manuscript; Xu Y performed experiments; Chen B collected samples and analyzed data.
Funding
This work was supported by Natural Science Foundation Project of CQ CSTC (No.2011BA5037).
References
- Kyle RA, Rajkumar SV. Multiple myeloma. New Eng J Med 2004; 351:1860-73; PMID:15509819; http://dx.doi.org/10.1056/NEJMra041875
- Becker N. Epidemiology of multiple myeloma. Recent Results Cancer Res 2011; 183:25-35
- Briani C, Berno T, Campagnolo M, Zambello R. Lenalidomide for bortezomib-resistant multiple myeloma. Nat Rev Clin Oncol 2010; 7:289-94; PMID:20824906
- Dimopoulos MA, Kastritis E, Christoulas D, Migkou M, Gavriatopoulou M, Gkotzamanidou M, Iakovaki M, Matsouka C, Mparmparoussi D, Roussou M, et al. Treatment of patients with relapsed/refractory multiple myeloma with lenalidomide and dexamethasone with or without bortezomib: prospective evaluation of the impact of cytogenetic abnormalities and of previous therapies. Leukemia 2010; 24:1769-78; PMID:20739955; http://dx.doi.org/10.1038/leu.2010.175
- Moreau P, Hulin C, Facon T. Frontline Therapy for Patients with Multiple Myeloma not Eligible for Stem Cell Transplantation. Hematol Oncol Clin North Am 2014; 28:829-38; PMID:25212885
- Kumar S. Stem cell transplantation for multiple myeloma. Curr Opin Oncol 2009; 21:162-70
- Jimenez-Zepeda VH, Reece DE, Trudel S, Chen C, Tiedemann R, Kukreti V. Lenalidomide (Revlimid), bortezomib (Velcade) and dexamethasone for heavily pretreated relapsed or refractory multiple myeloma. Leuk Lymphoma 2013; 54:555-60; PMID:22881043; http://dx.doi.org/10.3109/10428194.2012.719614
- Bataille R, Chappard D, Marcelli C, Dessauw P, Baldet P, Sany J, Alexandre C. Recruitment of new osteoblasts and osteoclasts is the earliest critical event in the pathogenesis of human multiple myeloma. J Clin Invest 1991; 88:62-6; PMID:2056131; http://dx.doi.org/10.1172/JCI115305
- Ren S, Johnson BG, Kida Y, Ip C, Davidson KC, Lin SL, Kobayashi A, Lang RA, Hadjantonakis AK, Moon RT, et al. LRP-6 is a coreceptor for multiple fibrogenic signaling pathways in pericytes and myofibroblasts that are inhibited by DKK-1. Proc Natl Acad Sci U S A 2013; 110:1440-5; PMID:23302695; http://dx.doi.org/10.1073/pnas.1211179110
- Qiang YW, Barlogie B, Rudikoff S, Shaughnessy JD, Jr. Dkk1-induced inhibition of Wnt signaling in osteoblast differentiation is an underlying mechanism of bone loss in multiple myeloma. Bone 2008; 42:669-80; PMID:18294945; http://dx.doi.org/10.1016/j.bone.2007.12.006
- Tian E, Zhan F, Walker R, Rasmussen E, Ma Y, Barlogie B, Shaughnessy JD Jr. The role of the Wnt-signaling antagonist DKK1 in the development of osteolytic lesions in multiple myeloma. N Eng J Med 2003; 349:2483-94; PMID:14695408; http://dx.doi.org/10.1056/NEJMoa030847
- Roodman GD. Pathogenesis of myeloma bone disease. Blood Cells Mol Dis 2004; 32:290-2
- Yaccoby S, Ling W, Zhan F, Walker R, Barlogie B, Shaughnessy JD, Jr. Antibody-based inhibition of DKK1 suppresses tumor-induced bone resorption and multiple myeloma growth in vivo. Blood 2007; 109:2106-11; PMID:17068150; http://dx.doi.org/10.1182/blood-2006-09-047712
- Fulciniti M, Tassone P, Hideshima T, Vallet S, Nanjappa P, Ettenberg SA, Shen Z, Patel N, Tai YT, Chauhan D, et al. Anti-DKK1 mAb (BHQ880) as a potential therapeutic agent for multiple myeloma. Blood 2009; 114:371-9; PMID:19417213; http://dx.doi.org/10.1182/blood-2008-11-191577
- Di Leva G, Garofalo M, Croce CM. MicroRNAs in cancer. Ann Rev Pathol 2014; 9:287-314; PMID:24079833; http://dx.doi.org/10.1146/annurev-pathol-012513-104715
- Esquela-Kerscher A, Slack FJ. Oncomirs - microRNAs with a role in cancer. Nature Rev Cancer 2006; 6:259-69; PMID:16557279; http://dx.doi.org/10.1038/nrc1840
- Braconi C, Huang N, Patel T. MicroRNA-dependent regulation of DNA methyltransferase-1 and tumor suppressor gene expression by interleukin-6 in human malignant cholangiocytes. Hepatology 2010; 51:881-90; PMID:20146264
- Murata T, Takayama K, Katayama S, Urano T, Horie-Inoue K, Ikeda K, Takahashi S, Kawazu C, Hasegawa A, Ouchi Y, et al. miR-148a is an androgen-responsive microRNA that promotes LNCaP prostate cell growth by repressing its target CAND1 expression. Prostate Cancer Prostat Dis 2010; 13:356-61; PMID:20820187; http://dx.doi.org/10.1038/pcan.2010.32
- Chen Y, Song YX, Wang ZN. The microRNA-148/152 family: multi-faceted players. Mol Cancer 2013; 12:43; PMID:23683438; http://dx.doi.org/10.1186/1476-4598-12-43
- Xu Q, Jiang Y, Yin Y, Li Q, He J, Jing Y, et al. A regulatory circuit of miR-148a/152 and DNMT1 in modulating cell transformation and tumor angiogenesis through IGF-IR and IRS1. J Mol Cell Biol 2013; 5:3-13; PMID:22935141; http://dx.doi.org/10.1093/jmcb/mjs049
- Hiroki E, Akahira J, Suzuki F, Nagase S, Ito K, Suzuki T, Sasano H, Yaegashi N. Changes in microRNA expression levels correlate with clinicopathological features and prognoses in endometrial serous adenocarcinomas. Cancer Sci 2010; 101:241-9; PMID:19891660; http://dx.doi.org/10.1111/j.1349-7006.2009.01385.x
- Tsuruta T, Kozaki K, Uesugi A, Furuta M, Hirasawa A, Imoto I, Susumu N, Aoki D, Inazawa J. miR-152 is a tumor suppressor microRNA that is silenced by DNA hypermethylation in endometrial cancer. Cancer Res 2011; 71:6450-62; PMID:21868754; http://dx.doi.org/10.1158/0008-5472.CAN-11-0364
- Zhou X, Zhao F, Wang ZN, Song YX, Chang H, Chiang Y, Xu HM. Altered expression of miR-152 and miR-148a in ovarian cancer is related to cell proliferation. Oncol Rep 2012; 27:447-54; PMID:21971665
- Rio-Machin A, Ferreira BI, Henry T, Gomez-Lopez G, Agirre X, Alvarez S, Rodriguez-Perales S, Prosper F, Calasanz MJ, Martínez J, et al. Downregulation of specific miRNAs in hyperdiploid multiple myeloma mimics the oncogenic effect of IgH translocations occurring in the non-hyperdiploid subtype. Leukemia 2013; 27:925-31; PMID:23174883; http://dx.doi.org/10.1038/leu.2012.302
- Qian J, Xie J, Hong S, Yang J, Zhang L, Han X, Wang M, Zhan F, Shaughnessy JD Jr, Epstein J, et al. Dickkopf-1 (DKK1) is a widely expressed and potent tumor-associated antigen in multiple myeloma. Blood 2007; 110:1587-94; PMID:17515399; http://dx.doi.org/10.1182/blood-2007-03-082529
- Gunn WG, Conley A, Deininger L, Olson SD, Prockop DJ, Gregory CA. A crosstalk between myeloma cells and marrow stromal cells stimulates production of DKK1 and interleukin-6: a potential role in the development of lytic bone disease and tumor progression in multiple myeloma. Stem Cells 2006; 24:986-91; PMID:16293576; http://dx.doi.org/10.1634/stemcells.2005-0220
- Chng WJ, Lau LG, Yusof N, Mow BM. Targeted therapy in multiple myeloma. Cancer Control 2005; 12:91-104; PMID:15855892
- Sun K, Lai EC. Adult-specific functions of animal microRNAs. Nat Rev Genet 2013; 14:535-48; PMID:23817310; http://dx.doi.org/10.1038/nrg3471
- Bi C, Chng WJ. MicroRNA: important player in the pathobiology of multiple myeloma. Biomed Res Int 2014; 2014:521586; PMID:24991558; http://dx.doi.org/10.1155/2014/521586
- Ahmad N, Haider S, Jagannathan S, Anaissie E, Driscoll JJ. MicroRNA theragnostics for the clinical management of multiple myeloma. Leukemia 2014; 28:732-8; PMID:24714346; http://dx.doi.org/10.1038/leu.2013.262
- Dimopoulos K, Gimsing P, Gronbaek K. Aberrant microRNA expression in multiple myeloma. Eur J Haematol 2013; 91:95-105; PMID:23586898; http://dx.doi.org/10.1111/ejh.12124
- Gutierrez NC, Sarasquete ME, Misiewicz-Krzeminska I, Delgado M, De Las Rivas J, Ticona FV, Fermiñán E, Martín-Jiménez P, Chillón C, Risueño A, et al. Deregulation of microRNA expression in the different genetic subtypes of multiple myeloma and correlation with gene expression profiling. Leukemia 2010; 24:629-37; PMID:20054351; http://dx.doi.org/10.1038/leu.2009.274
- Tagliaferri P, Rossi M, Di Martino MT, Amodio N, Leone E, Gulla A, Neri A, Tassone P. Promises and challenges of MicroRNA-based treatment of multiple myeloma. Curr Cancer Drug Targets 2012; 12:838-46; PMID:22671926; http://dx.doi.org/10.2174/156800912802429355
- Palagani A, Op de Beeck K, Naulaerts S, Diddens J, Sekhar Chirumamilla C, Van Camp G, Laukens K, Heyninck K, Gerlo S, Mestdagh P, et al. Ectopic microRNA-150-5p transcription sensitizes glucocorticoid therapy response in MM1S multiple myeloma cells but fails to overcome hormone therapy resistance in MM1R cells. PLoS One 2014; 9:e113842
- Zhang YK, Wang H, Leng Y, Li ZL, Yang YF, Xiao FJ, Li QF, Chen XQ, Wang LS. Overexpression of microRNA-29b induces apoptosis of multiple myeloma cells through down regulating Mcl−1. Biochem Biophys Res Commun 2011; 414:233-9; PMID:21951844; http://dx.doi.org/10.1016/j.bbrc.2011.09.063
- Musilova K, Mraz M. MicroRNAs in B-cell lymphomas: how a complex biology gets more complex. Leukemia 2015; 29(5):1004-17; PMID:25541152
- Postberg J, Kanders M, Forcob S, Willems R, Orth V, Hensel KO, Weil PP, Wirth S, Jenke AC. CpG signalling, H2A.ZH3 acetylation and microRNA-mediated deferred self-attenuation orchestrate foetal NOS3 expression. Clin Epigenetics 2015; 7:9; PMID:25699114
- Winter J, Jung S, Keller S, Gregory RI, Diederichs S. Many roads to maturity: microRNA biogenesis pathways and their regulation. Nat Cell Biol 2009; 11:228-34; PMID:19255566; http://dx.doi.org/10.1038/ncb0309-228
- Slezak-Prochazka I, Durmus S, Kroesen BJ, van den Berg A. MicroRNAs, macrocontrol: regulation of miRNA processing. RNA 2010; 16:1087-95; PMID:20423980; http://dx.doi.org/10.1261/rna.1804410
- Czech B, Hannon GJ. Small RNA sorting: matchmaking for Argonautes. Nat Rev Genet 2011; 12:19-31; PMID:21116305 http://dx.doi.org/10.1038/nrg2916
- Langhe R, Norris L, Saadeh FA, Blackshields G, Varley R, Harrison A, Gleeson N, Spillane C, Martin C, O'Donnell DM, et al. A novel serum microRNA panel to discriminate benign from malignant ovarian disease. Cancer Lett 2015; 356:628-36; PMID:25451316; http://dx.doi.org/10.1016/j.canlet.2014.10.010
- Taipaleenmaki H, Bjerre Hokland L, Chen L, Kauppinen S, Kassem M. Mechanisms in endocrinology: micro-RNAs: targets for enhancing osteoblast differentiation and bone formation. Eur J Endocrinol 2012; 166:359-71; PMID:22084154
- Forget MA, Turcotte S, Beauseigle D, Godin-Ethier J, Pelletier S, Martin J, Tanguay S, Lapointe R. The Wnt pathway regulator DKK1 is preferentially expressed in hormone-resistant breast tumours and in some common cancer types. Br J Cancer 2007; 96:646-53; PMID:17245340; http://dx.doi.org/10.1038/sj.bjc.6603579
- Liddell K. Smell as a diagnostic marker. Postgrad Med J 1976; 52:136-8
- Kapinas K, Kessler C, Ricks T, Gronowicz G, Delany AM. miR-29 modulates Wnt signaling in human osteoblasts through a positive feedback loop. J Biol Chem 2010; 285:25221-31; PMID:20551325; http://dx.doi.org/10.1074/jbc.M110.116137
- Zhou AD, Diao LT, Xu H, Xiao ZD, Li JH, Zhou H, Qu LH. Beta-Catenin/LEF1 transactivates the microRNA-371-373 cluster that modulates the Wnt/β-catenin-signaling pathway. Oncogene 2012; 31:2968-78; PMID:22020335
- Gomez-Bougie P, Oliver L, Le Gouill S, Bataille R, Amiot M. Melphalan-induced apoptosis in multiple myeloma cells is associated with a cleavage of Mcl−1 and Bim and a decrease in the Mcl−1/Bim complex. Oncogene 2005; 24:8076-9; PMID:16091744; http://dx.doi.org/10.1038/sj.onc.1208949
- Landau HJ, McNeely SC, Nair JS, Comenzo RL, Asai T, Friedman H, Jhanwar SC, Nimer SD, Schwartz GK. The checkpoint kinase inhibitor AZD7762 potentiates chemotherapy-induced apoptosis of p53-mutated multiple myeloma cells. Mol Cancer Ther 2012; 11:1781-8; PMID:22653969; http://dx.doi.org/10.1158/1535-7163.MCT-11-0949
- Zheng Y, Cai Z, Wang S, Zhang X, Qian J, Hong S, Li H, Wang M, Yang J, Yi Q. Macrophages are an abundant component of myeloma microenvironment and protect myeloma cells from chemotherapy drug-induced apoptosis. Blood 2009; 114:3625-8; PMID:19710503; http://dx.doi.org/10.1182/blood-2009-05-220285
- Lonial S, Kaufman J, Tighiouart M, Nooka A, Langston AA, Heffner LT, Torre C, McMillan S, Renfroe H, Harvey RD, et al. A phase I/II trial combining high-dose melphalan and autologous transplant with bortezomib for multiple myeloma: a dose- and schedule-finding study. Clin Cancer Res 2010; 16:5079-86; PMID:20739431; http://dx.doi.org/10.1158/1078-0432.CCR-10-1662
- Chauhan D, Anderson KC. Mechanisms of cell death and survival in multiple myeloma (MM): Therapeutic implications. Apoptosis 2003; 8:337-43; PMID:12815276; http://dx.doi.org/10.1023/A:1024164700094
- George SK, Tovar-Sepulveda V, Shen SS, Jian W, Zhang Y, Hilsenbeck SG, Lerner SP, Smith CL. Chemoprevention of BBN-Induced Bladder Carcinogenesis by the Selective Estrogen Receptor Modulator Tamoxifen. Transl Oncol 2013; 6:244-55; PMID:23730403; http://dx.doi.org/10.1593/tlo.13247
- Furuya Y, Inagaki A, Khan M, Mori K, Penninger JM, Nakamura M, Udagawa N, Aoki K, Ohya K, Uchida K, et al. Stimulation of bone formation in cortical bone of mice treated with a receptor activator of nuclear factor-kappaB ligand (RANKL)-binding peptide that possesses osteoclastogenesis inhibitory activity. J Biol Chem 2013; 288:5562-71; PMID:23319583; http://dx.doi.org/10.1074/jbc.M112.426080